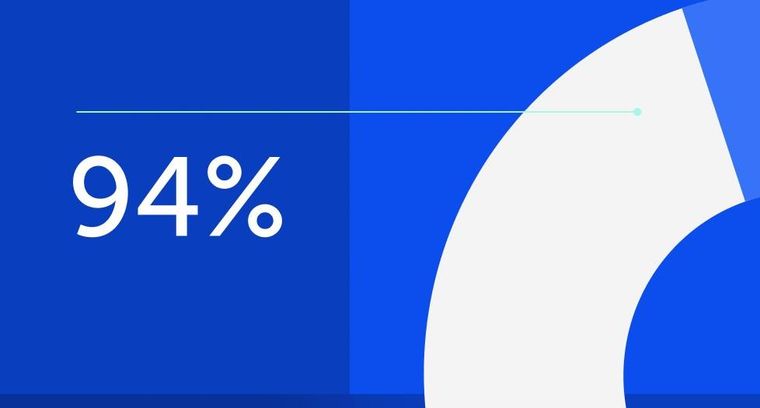
94% of researchers rate our articles as excellent or good
Learn more about the work of our research integrity team to safeguard the quality of each article we publish.
Find out more
ORIGINAL RESEARCH article
Front. Endocrinol., 26 August 2021
Sec. Systems Endocrinology
Volume 12 - 2021 | https://doi.org/10.3389/fendo.2021.719920
Observational studies report some association between circulating bilirubin levels and osteoporosis, but it is unknown if this association is causal or confounded. In this two-sample Mendelian randomization (MR) study, we included a large genome-wide association study (GWAS) associated with total bilirubin levels among 317,639 people, a large meta-analysis to identify genetic variants associated with bone mineral density (BMD) estimated by heel quantitative ultrasound (eBMD) among 426,824 individuals and fracture among 1.2 million individuals. The results revealed that circulating bilirubin levels had no causal influence on eBMD (beta-estimate: 0.004, 95% confidence interval [CI]: -0.019 to 0.028, SE:0.012, P-value=0.705) or the risk of fracture (beta-estimate: -0.009, 95% CI: -0.035 to 0.017, SE:0.013, P-value=0.488), which were both confirmed by multiple sensitivity analyses. Our results confirm that circulating bilirubin levels have no causal role in eBMD or the incidence of fracture, indicating that circulating bilirubin levels is unlikely to be a causal risk factor for osteoporosis or fracture.
As one common and aging-related disease, osteoporosis is featured by decreased bone mineral density (BMD) and increased risk of fracture (1–4). The treatment of osteoporosis remains a big challenge and public health problem in the world (5–7). Osteoporosis is a common complication of liver diseases such as chronic cholestasis and primary biliary cholangitis (8, 9). Bone loss is caused by deficient osteoblast activity and increased bone resorption (10). High concentrations of circulating bilirubin levels were documented to result in the abnormal osteoblast function and serum bilirubin had detrimental effects on bone-forming cells (11). Emerging evidence suggested that total bilirubin levels participated in multiple biological activities such as immunomodulatory processes (12, 13).
The association between bilirubin levels and osteoporosis has not been well established, and several studies have reported the conflicting results (8, 14–16). For instance, one observational study included 918 postmenopausal individuals without potential liver diseases, and revealed that total bilirubin level was independently associated with BMD [beta-estimate=0.41, 95% CI (0.35–0.47), P<0.001 for lumbar spine BMD and beta-estimate=0.44, 95% CI (0.36–0.48), P<0.001 for femur neck BMD] (16). However, these observational studies may be subject to confounding factors and reverse causality.
Genome-wide association studies (GWASs) demonstrate that osteoporosis is one highly polygenic trait (17–19). Mendelian randomization (MR) study is effective and powerful to establish the causal relationship between exposure phenotype and exposure phenotype through using the GWAS summary statistics (20–22). These genetic variants are randomly allocated before birth and fixed at conception, and benefit to prevent reverse causation and potential confounding factors (23, 24).
The two-sample MR analysis has emerged as an important approach to greatly increase the scope and statistical power of MR based on the published summary data from GWASs (22, 25, 26). In this study, we use single nucleotide polymorphisms (SNPs) strongly associated with circulating bilirubin levels as the instrumental variables. To our knowledge, this is the first two-sample MR study to explore the causal effect of total bilirubin levels on BMD and fracture.
A large-scale GWAS associated with circulating bilirubin levels included 317,639 individuals of European ancestry (27). Total bilirubin levels in serum was measured using a colorimetric assay (2,4-dicholorani-line reaction). Each SNP was tested after adjusting for age, sex, recruitment center, indicators of socioeconomic status, the top 40 principal components for population stratification, and potential technical confounders (i.e. fasting time, sample dilution factor, blood and urine sampling time) (27).
Initially, 115 SNPs with genome-wide significance (P<5×10−8) were found to have robust association with total bilirubin levels (Supplementary Table 1). Then, SNPs were ideally expected to not be in linkage disequilibrium (LD), because SNPs in strong LD may produce some bias. We measured LD between selected SNPs using European samples from the 1000 Genomes project. 27 SNPs were excluded due to high LD (r2≥0.001). Finally, 88 SNPs associated with circulating bilirubin levels were used as instrumental variables (Supplementary Table 2). If SNPs were unavailable in the outcome dataset, the proxy SNPs in linkage disequilibrium (LD, r2>0.9) were used as the instrumental variables. Thus, rs72831372 was used as a proxy for rs7185774 among eBMD, but no proxy SNPs were used for rs11601507 or rs157595 among eBMD (Supplementary Table 2).
The large-scale GWAS summary data was calculated among 426,824 people of European decent for bone mineral density (BMD) and up to 1.2 million individuals of European decent for fracture. BMD was estimated by heel quantitative ultrasound (eBMD, [g/cm2]), which was derived as a linear combination of speed of sound (SOS) and bone ultrasound attenuation (BUA) (i.e. eBMD = 0.002592 * (BUA + SOS) − 3.687). Fracture cases were defined as any fracture apart from the fracture of skull, face, hands, feet, pathological fractures due to malignancy, atypical femoral fractures, periprosthetic and healed fracture. These GWAS summary data were analyzed after adjusting for age, sex and genotyping (1).
To determine MR estimates of total bilirubin levels on eBMD and fracture, we conducted the inverse variance weighted (IVW) meta-analysis of the Wald ratio. The weighted median and MR-Egger regression methods were also applied to estimate the effects. We evaluated the directional pleiotropy based on the intercept obtained from MR-Egger analysis (28). MR pleiotropy residual sum and outlier test (MR-PRESSO) was also used to assess the presence of pleiotropy and the effect estimates were recalculated after outlying SNPs were excluded (29).
The ethical approval for each study included in this investigation can be found in the original publications (including informed consent from each participant). The differences with P<0.05 were considered statistically significant. All of these analyses were conducted in R V.4.0.4 by using the R packages of ‘MendelianRandomization’ (30), ‘TwoSampleMR’ (31) and ‘MR-PRESSO’ (32).
We evaluated the causal effect of circulating bilirubin levels on eBMD in the MR analysis (Table 1). Circulating bilirubin levels demonstrated no obvious MR association with eBMD according to IVW analysis (beta-estimate: 0.004, 95% CI: -0.019 to 0.028, SE:0.012, P-value=0.705) or weighted-median analysis (beta-estimate: 0.002, 95% CI: -0.004 to 0.008, SE:0.003, P-value=0.511), which was also confirmed by MR-Egger analysis (beta-estimate: 0.009, 95% CI: -0.017 to 0.034, SE:0.013, P-value=0.500, Table 1 and Figure 1).
Figure 1 Mendelian randomization analysis for the association between circulating bilirubin levels and eBMD.
Circulating bilirubin levels showed no causal effect on the risk of fracture according to the IVW analysis (beta-estimate: -0.009, 95% CI: -0.035 to 0.017, SE:0.013, P-value=0.488), weighted-median analysis (beta-estimate: -0.009, 95% CI: -0.031 to 0.013, SE:0.011, P-value=0.436) or MR-Egger analysis (beta-estimate: -0.007, 95% CI: -0.035 to 0.021, SE:0.014, P-value=0.618, Table 1). MR association between circulating bilirubin levels and fracture was presented in Figure 2.
Figure 2 Mendelian randomization analysis for the association between circulating bilirubin levels and fracture.
There was little evidence of directional pleiotropy for all models (MR-Egger intercept P-values >0.05) (Table 1). Significant heterogeneity remained for eBMD and fracture based on Cochran’s Q (Heterogeneity P-heterogeneity<0.05), and thus MR-PRESSO test was conducted to identify 27 outliers (rs2375279, rs17513135, rs556107, rs4671605, rs6431625, rs2267846, rs1482852, rs61791066, rs151450, rs1126673, rs6869704, rs12515233, rs853684, rs12210538, rs58699591, rs3118753, rs2519093, rs34755157, rs174554, rs76895963, rs4149056, rs4760682, rs61984409, rs17184256, rs4575545, rs7222046, rs4820091) for eBMD and one outlier (rs174554) for fracture among the 88 SNP instrumental variables.
After excluding these outlying SNP variants, circulating bilirubin levels showed no MR association with eBMD (beta-estimate: 0.035, 95% CI: -0.006 to 0.076, SE:0.021, P-value=0.094, Table 2 and Figure 1) or fracture (beta-estimate: -0.010, 95% CI: -0.035 to 0.014, SE:0.012, P-value=0.402, Table 2 and Figure 2).
Table 2 Mendelian randomization estimates between bilirubin levels and osteopross after excluding outliers detected by PRESSO.
Overall, our large multi-instrument approaches found no causal effect of circulating bilirubin levels on eBMD or the risk of fracture. This two-sample MR estimates had great robustness to support no MR association between circulating bilirubin levels and osteoporosis based on the results of various MR methods and sensitivity analyses. These indicated that circulating bilirubin levels is not the causal risk factor for osteoporosis or fracture.
Circulating bilirubin level is one robust indicator of hepatic dysfunction, and in vitro study reveals that bilirubin from jaundiced patients can inhibit the proliferative capacity of osteoblast (11, 33, 34). Total bilirubin has a detrimental effect on cell viability, cell differentiation and mineralization of primary human osteoblasts in a dose-dependent manner (11). In addition, total bilirubin is an end metabolic product of heme degradation and servers as a potent antioxidant to inhibit the oxidization of lipids and lipoprotein (especially low density lipoprotein) and eliminate radical oxygen species in the various forms including free, albumin-bound, unconjugated and conjugated forms (16, 35). Osteoporosis caused by oxidative stress may be also associated with endothelial dysfunction and decreased blood flow for bone tissue (36, 37). These provide strong theoretical support for the association between circulating bilirubin levels and osteoporosis.
Several epidemiological studies have demonstrated the significant inverse association between total bilirubin levels and BMD in patients with underlying liver diseases, but other studies reported conflicting association between them (8, 14–16). This inconsistent association may be caused by the methodological limitations (i.e. confounding, reverse causation and measurement error) of traditional observational study and small patient sample (38). Because total bilirubin level is a highly informative indicator of hepatic dysfunction, it is infeasible to identify whether bilirubin level is the direct cause of osteoporosis (16, 33, 39). It remains uncertain whether circulating bilirubin level has the casual effect on osteoporosis or fracture.
Randomized controlled trial (RCT) is the gold standard in causal inference, but it is not feasible to explore the association between total bilirubin levels and osteoporosis due to the unethical approaches of raising bilirubin levels. The MR study is widely used to evaluate causal inferences between risk factors and disease outcomes with the features of preventing confounding and reverse causation (40). To date, our work is the first two-sample MR study to explore the causal effect of circulating bilirubin levels on eBMD and fracture.
Our study included the large GWAS for total bilirubin levels among 317,639 individuals and the large meta-analysis to identify genetic variants associated with BMD among 426,824 individuals and fracture among 1.2 million individuals. This two-sample MR study confirmed no casual role of circulating bilirubin levels in eBMD or the risk of fracture based on the multiple MR methods and sensitivity analyses.
There are several important strengths in this study. This is the first two-sample MR study to investigate the causal effect of circulating bilirubin levels on BMD and fracture. This study design can prevent some limitations (e.g. reverse causation and potential confounding factors) of conventional observational studies. Our study include large sample sizes of GWAS summary data associated with circulating bilirubin levels, BMD and fracture. The intercepts for the MR-Egger analyses suggest that all causal associations are not affected by directional pleiotropy. We also conduct multiple sensitivity analyses to test the influence of pleiotropy on our causal estimates, and our results are consistent and robust according to various MR tests and sensitivity analyses.
Several limitations should be taken into consideration. Firstly, all the included participants are of European decent, and more studies are needed to explore whether our findings are generalizable to other populations. Secondly, it is not feasible to perform the MR analysis based on different age stratums because of the limitation of GWAS summary statistics. Thirdly, significant heterogeneity remains for the MR association between bilirubin levels and BMD/fracture, which may be caused by different patient populations and unknown confounding factors.
This two-sample MR provides strong evidence to confirm that circulating bilirubin levels is unlikely to be a causal risk factor of osteoporosis or fracture.
The original contributions presented in the study are included in the article/Supplementary Material. Further inquiries can be directed to the corresponding authors.
JZ, MZ, BH, and ZQ conducted Study Design, Data Collection, and Statistical Analysis. BH, MZ, LD, YL, and JZ conducted Data Interpretation, Manuscript Preparation, and Literature Search. LD, BH, and JZ conducted Funds Collection. All authors contributed to the article and approved the submitted version.
This study was funded by Chongqing Yuzhong Nature Science Foundation of China (Grant No. 2018114), National Natural Science Foundation of China (82070626) and Natural Science Foundation of Chongqing (cstc2019jcyj-msxmX0836).
The authors declare that the research was conducted in the absence of any commercial or financial relationships that could be construed as a potential conflict of interest.
All claims expressed in this article are solely those of the authors and do not necessarily represent those of their affiliated organizations, or those of the publisher, the editors and the reviewers. Any product that may be evaluated in this article, or claim that may be made by its manufacturer, is not guaranteed or endorsed by the publisher.
The authors acknowledged the GEnetic Factors for OSteoporosis Consortium and the UK Biobank for contributing the data used in this work. We thank all the genetics consortiums for making the GWAS summary data publicly available.
The Supplementary Material for this article can be found online at: https://www.frontiersin.org/articles/10.3389/fendo.2021.719920/full#supplementary-material
1. Morris JA, Kemp JP, Youlten SE, Laurent L, Logan JG, Chai RC, et al. An Atlas of Genetic Influences on Osteoporosis in Humans and Mice. Nat Genet (2019) 51(2):258–66. doi: 10.1038/s41588-018-0302-x
2. Liu J, Curtis EM, Cooper C, Harvey NC. State of the Art in Osteoporosis Risk Assessment and Treatment. J Endocrinol Invest (2019) 42(10):1149–64. doi: 10.1007/s40618-019-01041-6
3. Black DM, Geiger EJ, Eastell R, Vittinghoff E, Li BH, Ryan DS, et al. Atypical Femur Fracture Risk Versus Fragility Fracture Prevention With Bisphosphonates. N Engl J Med (2020) 383(8):743–53. doi: 10.1056/NEJMoa1916525
4. Leder BZ, Mitlak B, Hu MY, Hattersley G, Bockman RS. Effect of Abaloparatide vs Alendronate on Fracture Risk Reduction in Postmenopausal Women With Osteoporosis. J Clin Endocrinol Metab (2020) 105(3):938–43. doi: 10.1210/clinem/dgz162
5. Reid IR. A Broader Strategy for Osteoporosis Interventions, Nature Reviews. Endocrinology (2020) 16(6):333–9. doi: 10.1038/s41574-020-0339-7
6. Khosla S, Hofbauer LC. Osteoporosis Treatment: Recent Developments and Ongoing Challenges. Lancet Diabetes Endocrinol (2017) 5(11):898–907. doi: 10.1016/S2213-8587(17)30188-2
7. Compston JE, McClung MR, Leslie WD. Osteoporosis. Lancet (Lond Engl) (2019) 393(10169):364–76. doi: 10.1016/S0140-6736(18)32112-3
8. Menon KV, Angulo P, Weston S, Dickson ER, Lindor KD. Bone Disease in Primary Biliary Cirrhosis: Independent Indicators and Rate of Progression. J Hepatol (2001) 35(3):316–23. doi: 10.1016/S0168-8278(01)00144-1
9. Guañabens N, Parés A, Ros I, Caballería L, Pons F, Vidal S, et al. Severity of Cholestasis and Advanced Histological Stage But Not Menopausal Status Are the Major Risk Factors for Osteoporosis in Primary Biliary Cirrhosis. J Hepatol (2005) 42(4):573–7. doi: 10.1016/j.jhep.2004.11.035
10. Guichelaar MM, Malinchoc M, Sibonga J, Clarke BL, Hay JE. Bone Metabolism in Advanced Cholestatic Liver Disease: Analysis by Bone Histomorphometry. Hepatology (2002) 36(4 Pt 1):895–903. doi: 10.1053/jhep.2002.36357
11. Ruiz-Gaspà S, Martinez-Ferrer A, Guañabens N, Dubreuil M, Peris P, Enjuanes A, et al. Effects of Bilirubin and Sera From Jaundiced Patients on Osteoblasts: Contribution to the Development of Osteoporosis in Liver Diseases. Hepatology (2011) 54(6):2104–13. doi: 10.1002/hep.24605
12. Kirkby KA, Adin CA. Products of Heme Oxygenase and Their Potential Therapeutic Applications. Am J Physiol Renal Physiol (2006) 290(3):F563–71. doi: 10.1152/ajprenal.00220.2005
13. Ali SS, Zia MK, Siddiqui T, Ahsan H, Khan FH. Bilirubin Binding Affects the Structure and Function of Alpha-2-Macroglobulin. J Immunoassay Immunochem (2020) 41(5):841–51. doi: 10.1080/15321819.2020.1783290
14. Ormarsdóttir S, Ljunggren O, Mallmin H, Michaëlsson K, Lööf L. Increased Rate of Bone Loss at the Femoral Neck in Patients With Chronic Liver Disease. Eur J Gastroenterol Hepatol (2002) 14(1):43–8. doi: 10.1097/00042737-200201000-00008
15. Newton J, Francis R, Prince M, James O, Bassendine M, Rawlings D, et al. Osteoporosis in Primary Biliary Cirrhosis Revisited. Gut (2001) 49(2):282–7. doi: 10.1136/gut.49.2.282
16. Bian LQ, Li RZ, Zhang ZY, Jin YJ, Kang HW, Fang ZZ, et al. Effects of Total Bilirubin on the Prevalence of Osteoporosis in Postmenopausal Women Without Potential Liver Disease. J Bone Mineral Metab (2013) 31(6):637–43. doi: 10.1007/s00774-013-0452-y
17. Richards JB, Zheng HF, Spector TD. Genetics of Osteoporosis From Genome-Wide Association Studies: Advances and Challenges, Nature Reviews. Genetics (2012) 13(8):576–88. doi: 10.1038/nrg3228
18. Trajanoska K, Rivadeneira F. The Genetic Architecture of Osteoporosis and Fracture Risk. Bone (2019) 126:2–10. doi: 10.1016/j.bone.2019.04.005
19. Yang TL, Shen H, Liu A, Dong SS, Zhang L, Deng FY, et al. A Road Map for Understanding Molecular and Genetic Determinants of Osteoporosis. Nat Rev Endocrinol (2020) 16(2):91–103. doi: 10.1038/s41574-019-0282-7
20. Burgess S, Dudbridge F, Thompson SG. Combining Information on Multiple Instrumental Variables in Mendelian Randomization: Comparison of Allele Score and Summarized Data Methods. Stat Med (2016) 35(11):1880–906. doi: 10.1002/sim.6835
21. Dalbeth N, Topless R, Flynn T, Cadzow M, Bolland MJ, Merriman TR. Mendelian Randomization Analysis to Examine for a Causal Effect of Urate on Bone Mineral Density. J Bone Mineral Res (2015) 30(6):985–91. doi: 10.1002/jbmr.2434
22. He B, Lyu Q, Yin L, Zhang M, Quan Z, Ou Y. Depression and Osteoporosis: A Mendelian Randomization Study. Calcified Tissue Int (2021). doi: 10.1007/s00223-021-00886-5
23. Davey Smith G, Ebrahim S. What Can Mendelian Randomisation Tell Us About Modifiable Behavioural and Environmental Exposures? BMJ (2005) 330(7499):1076–9. doi: 10.1136/bmj.330.7499.1076
24. Davies NM, Holmes MV, Davey Smith G. Reading Mendelian Randomisation Studies: A Guide, Glossary, and Checklist for Clinicians. BMJ (2018) 362:k601. doi: 10.1136/bmj.k601
25. Pierce BL, Burgess S. Efficient Design for Mendelian Randomization Studies: Subsample and 2-Sample Instrumental Variable Estimators. Am J Epidemiol (2013) 178(7):1177–84. doi: 10.1093/aje/kwt084
26. Burgess S, Scott RA, Timpson NJ, Davey Smith G, Thompson SG. Using Published Data in Mendelian Randomization: A Blueprint for Efficient Identification of Causal Risk Factors. Eur J Epidemiol (2015) 30(7):543–52. doi: 10.1007/s10654-015-0011-z
27. Sinnott-Armstrong N, Tanigawa Y, Amar D, Mars N, Benner C, Aguirre M, et al. Genetics of 35 Blood and Urine Biomarkers in the UK Biobank. Nat Genet (2021) 53(2):185–94. doi: 10.1038/s41588-020-00757-z
28. Burgess S, Thompson SG. Interpreting Findings From Mendelian Randomization Using the MR-Egger Method. Eur J Epidemiol (2017) 32(5):377–89. doi: 10.1007/s10654-017-0255-x
29. Burgess S, Thompson SG. Multivariable Mendelian Randomization: The Use of Pleiotropic Genetic Variants to Estimate Causal Effects. Am J Epidemiol (2015) 181(4):251–60. doi: 10.1093/aje/kwu283
30. Yavorska OO, Burgess S. MendelianRandomization: An R Package for Performing Mendelian Randomization Analyses Using Summarized Data. Int J Epidemiol (2017) 46(6):1734–9. doi: 10.1093/ije/dyx034
31. Hemani G, Zheng J, Elsworth B, Wade KH, Haberland V, Baird D, et al. The MR-Base Platform Supports Systematic Causal Inference Across the Human Phenome. eLife (2018) 7:e34408. doi: 10.7554/eLife.34408
32. Verbanck M, Chen CY, Neale B, Do R. Detection of Widespread Horizontal Pleiotropy in Causal Relationships Inferred From Mendelian Randomization Between Complex Traits and Diseases. Nat Genet (2018) 50(5):693–8. doi: 10.1038/s41588-018-0099-7
33. Malinchoc M, Kamath PS, Gordon FD, Peine CJ, Rank J, ter Borg PC. A Model to Predict Poor Survival in Patients Undergoing Transjugular Intrahepatic Portosystemic Shunts. Hepatology (2000) 31(4):864–71. doi: 10.1053/he.2000.5852
34. Kunutsor SK, Frysz M, Verweij N, Kieneker LM, Bakker SJL, Dullaart RPF. Circulating Total Bilirubin and Risk of Non-Alcoholic Fatty Liver Disease in the PREVEND Study: Observational Findings and a Mendelian Randomization Study. Eur J Epidemiol (2020) 35(2):123–37. doi: 10.1007/s10654-019-00589-0
35. Kwon YJ, Lee HS, Lee JW. Direct Bilirubin Is Associated With Low-Density Lipoprotein Subfractions and Particle Size in Overweight and Centrally Obese Women. Nutrition Metabol Cardiovasc Dis: NMCD (2018) 28(10):1021–8. doi: 10.1016/j.numecd.2018.05.013
36. Sánchez-Rodríguez MA, Ruiz-Ramos M, Correa-Muñoz E, Mendoza-Núñez VM. Oxidative Stress as a Risk Factor for Osteoporosis in Elderly Mexicans as Characterized by Antioxidant Enzymes. BMC Musculoskelet Disord (2007) 8:124. doi: 10.1186/1471-2474-8-124
37. Zhou Q, Zhu L, Zhang D, Li N, Li Q, Dai P, et al. Oxidative Stress-Related Biomarkers in Postmenopausal Osteoporosis: A Systematic Review and Meta-Analyses. Dis Markers (2016) 2016:7067984. doi: 10.1155/2016/7067984
38. Boyko EJ. Observational Research–Opportunities and Limitations. J Diabetes Its Complicat (2013) 27(6):642–8. doi: 10.1016/j.jdiacomp.2013.07.007
39. Parés A, Rodés J. Natural History of Primary Biliary Cirrhosis. Clinics Liver Dis (2003) 7(4):779–94. doi: 10.1016/S1089-3261(03)00100-4
Keywords: osteoporosis, circulating bilirubin levels, BMD, fracture, Mendelian randomization study
Citation: Zhao J, Zhang M, Quan Z, Deng L, Li Y and He B (2021) Systematic Influence of Circulating Bilirubin Levels on Osteoporosis. Front. Endocrinol. 12:719920. doi: 10.3389/fendo.2021.719920
Received: 10 June 2021; Accepted: 02 August 2021;
Published: 26 August 2021.
Edited by:
Marcus M. Seldin, University of California, Irvine, United StatesReviewed by:
Eusebio Chiefari, University Magna Graecia of Catanzaro, ItalyCopyright © 2021 Zhao, Zhang, Quan, Deng, Li and He. This is an open-access article distributed under the terms of the Creative Commons Attribution License (CC BY). The use, distribution or reproduction in other forums is permitted, provided the original author(s) and the copyright owner(s) are credited and that the original publication in this journal is cited, in accordance with accepted academic practice. No use, distribution or reproduction is permitted which does not comply with these terms.
*Correspondence: Liang Deng, ZGVuZ2xpYW5nbGlvbkAxNjMuY29t; Yongguo Li, bGl5b25nZ3VvZG9jdG9yQDE2My5jb20=
†These authors have contributed equally to this work
Disclaimer: All claims expressed in this article are solely those of the authors and do not necessarily represent those of their affiliated organizations, or those of the publisher, the editors and the reviewers. Any product that may be evaluated in this article or claim that may be made by its manufacturer is not guaranteed or endorsed by the publisher.
Research integrity at Frontiers
Learn more about the work of our research integrity team to safeguard the quality of each article we publish.