- 1Obstetrics and Gynecology Hospital, Fudan University, Shanghai, China
- 2Shanghai Key Laboratory of Female Reproductive Endocrine Related Diseases, Obstetrics and Gynecology Hospital, Fudan University, Shanghai, China
As a rate-limiting step in pregnancy, embryo implantation is highly dependent on intercellular communication. Extracellular vesicles (EVs) are newly identified to be important in the course of intercellular communication. EVs have been isolated from a wide variety of biofluids and tissues, including plasma, liver, uterine, semen, embryo, etc. The present and future use of EVs not only as biomarkers, but also as targeting drug delivery system, is promisingly pave the way for advanced comprehension of implantation failure in reproductive diseases. However, as the precise mechanisms of EVs in embryo implantation has not been elucidated yet. Herein, we summarize the current knowledge on the diverse effects of EVs from various sources and their cargos such as microRNA, long non-coding RNA, protein, etc. on embryo implantation, and the potential mechanisms of EVs in reproductive diseases such as recurrent implantation failure, polycystic ovary syndrome and endometriosis. It is essential to note that many of the biologically plausible functions of EVs in embryo implantation discussed in present literatures still need further research in vivo.
Introduction
Implantation is a continuous dynamic process during which the blastocyst is implanted in the receptive endometrium in the mid-luteal phase. Embryo implantation is the starting point and a rate-limiting step of pregnancy. The success of embryo implantation mainly depends on two factors: zygote and corresponding establishment of endometrial receptivity. The essential processes of embryo implantation involve “location”, “adhesion” and “invasion”. Endometrial receptivity is present only for a very short time in the mid-secretory phase of each menstrual cycle, typically occurring in the 22th to 24th days of the cycle (1). This fleeting moment is called “windows of implantation (WOI)”.
Contrary to the widespread belief, an article proposed that “embryo implantation” should more properly be regarded as the “war” between embryo and endometrium (2). The embryo uses a variety of coercive tactics to force its acceptance by endometrium. It’s not a cooperation or an accommodation, but an aggression and a conquest. This metaphor is not exaggerated because the natural conception rate of human is very low (only 30-40%) (3). Additionally, 75% of pregnancy losses are due to failed embryo implantation (4). Even with the help of assisted reproductive technology, the pregnancy rate is still low, the culprit remains implantation failure (5, 6). Take polycystic ovary syndrome (PCOS) as an example, even we are able to obtain high-quality oocytes by controlled ovarian hyperstimulation and ideal blastocytes by in vitro fertilization, the rate of clinical pregnancy and live birth is still lower in women with PCOS comparing with that of women without PCOS, which is mainly due to the impaired endometrial receptivity (7, 8). At present, embryo implantation has obviously become an unsolved hot issue in the field of reproductive medicine research, and the mechanisms of embryo implantation need further study. Embryo implantation only occurs when the development of embryo coordinate with the station of endometrium, which is highly dependent on intercellular communication (9–11). Intercellular communication in traditional sense relies on intimate physiology contact or soluble mediators in microenvironment such as hormones, growth factors, cytokines, chemokines and proteases (12–16). In recent years, a series of papers have revealed a bran-new communication mechanism that modulates embryo implantation, which is called intercellular communication mediated by extracellular vesicles (EVs) (13, 17, 18).
The aim of this review is to summarize the current knowledge about the physiological roles of EVs produced by maternal tissues, embryo, semen as intercellular messengers in embryo implantation process. We have also reviewed how EVs affect embryo implantation in reproductive diseases.
Methods
The present review includes three strategies: literature search, study selection, and results summary. A systematic review was performed using the PubMed, Medline (Ovid), Embase (Ovid), and Web of Science databases without additional limits (Figure 1). We used the following query: (‘extracellular vesicles’ or ‘exosomes’ or ‘microparticles’ or ‘microvesicles’) and (‘embryo implantation’ or ‘embryo development’ or ‘endometrium’ or ‘polycystic ovary syndrome’ or ‘recurrent implantation failure’ or ‘endometriosis’). The last search was run on 25 April 2021. Both animal and human studies were considered suitable for this review. Research studies on EVs (“apoptotic bodies” and “apoptotic vesicles” are not included) or their regulation in embryo implantation, discussing either the endometrial receptivity, embryo, or both, were eligible for inclusion.
We identified 12121 articles after a primary search by the databases (Figure 1), immediately excluded 3577 records because they were duplicated. Then two Reviewers independently screened articles by title and abstract, any discrepancies were resolved by consensus. 8338 records were excluded (including irrelevant topics, reviews, comments, replies or letters to the Editor). The remaining 206 articles were collected as full-texts. Finally, after full-text screening, 65 articles were used for qualitative analysis.
Extracellular Vesicles
EVs are cell-derived membranous vesicles without specific targets. The cargos of EVs are heterogeneous, including nucleic acids (DNA, mRNA, microRNAs and long non-coding RNAs), proteins, lipids and so on. Almost all cells can produce and release EVs. Once released into extracellular space, EVs can produce local effects through autocrine and paracrine methods, Or be transported to distant organs or tissues through body fluids such as blood and lymph as important carriers for molecular exchange between different kinds of cells in short or long distance (19). However, there is still a lack of consensus on the nomenclature of EVs (19). We propose to distinguish three different types of EVs on the basis of biogenetic pathway and physical characteristics: 1. exosomes, 2. microparticles (MPs) (or microvesicles, MVs) and 3. apoptotic bodies (17, 20). Exosomes are 40-120 nm size homogenous vesicles, which originated from multivesicular bodies (21, 22) and enriched in major histocompatibility complex class I (MHC class I), MHC class II and tetraspanins such as CD6, CD9, CD63, CD81, as well as protein markers like Alix, TSG101 and chaperones (23, 24).
EVs and Embryo Implantation
Embryo implantation is divided into three steps (25): (1) Establishment of endometrial receptivity (2), Endometrial decidualization regulated by embryonic signals (3), Trophoblast invasion. Production of EVs by various tissues/cells such as endometrium, decidua, embryo, seminal fluid, oviduct as well as diverse stem cells has been reported to have an impact on the three steps of embryo implantation (summarized in Table 1). There are 30 EVs-related references in Section 4.
Endometrium-Derived EVs
Endometrial epithelial cell-derived exosomes can fuse with developing embryos and functionally affect the process of adhesion and invasion of blastocysts (26, 27, 48, 49), which partially mediated by active focal adhesion kinase (FAK) signaling (28, 29). Endometrial epithelial cell-derived exosomes can enhance human trophectodermal spheroid adhesion and outgrowth capacity (30). Besides, endometrial epithelial-exosomes-treated embryos have an increased implantation rate in vivo (30). Exosomal microRNAs from endometrial epithelial cells, including miR-218, ensure trophoblast cell development by targeting sFRP2 and regulating the WNT signaling pathway under conditions of endometritis (31). In addition, Embryo ATP production can be modulated by maternal mitochondrial DNA secreted from endometrial fluid-derived-EVs (50).
Proteome of uterine lavage-EVs may provide novel insights into biological processes critical for embryo development, implantation, and successful pregnancy (51). These EVs are dynamically regulated for their protein composition throughout menstrual cycle, transfer invasive properties and antioxidant function to trophectoderm cells (51). Moreover, these EVs carry proteins that regulate embryo implantation and predict WOI, thus highlighting their potential as a minimally invasive biomarker (51).
Decidual Stromal Cell (DSC)-Derived EVs
Decidualization is the epithelioid transformation of endometrial stromal cells (eSC) during embryo implantation (23). Both endometrial stromal and epithelial cells can intake embryo-derived EVs (28), while DSCs-derived EVs can also be taken up by trophoblast cells and induce invasion through SMAD2/3-N-cadherin signaling pathway (32).
Embryo-Derived EVs
Based on the latest research, bovine embryos secrete EVs with microRNA content according to embryonic competence and developmental stage. Similar to what was observed with EVs generated by other cell types, embryonic EVs are also involved in cellular signal transduction, and thereby regulating embryo implantation (21, 33). Supplementation of outgrowth embryo-derived EVs to the culture medium improved the development (52) and implantation capacity of preimplantation embryo (21). MVs produced by embryonic stem (ES) cells play an important role in stimulating trophoblast migration through the activation of FAK and c-Jun N-terminal kinase (JNK) (18). The injection of MVs isolated from ES cells into blastocysts increases the efficiency of embryo implantation (18). Human embryo-derived EVs have effects on endometrium by altering the expression of specific transcripts in endometrial epithelial cells (33). Interestingly, only good-prognosis embryos induced the observed effects while degenerated embryos failed to initiate any changes (33). Recently, an encouraging study reported that the size of EVs from culture medium of human embryos might be an alternative for evaluating their developmental competence (53). In the process of in vitro culture, the mean diameter of MVs/Exo from top quality embryos was higher (112.17 nm) than that of fair (108.02 nm) and poor quality embryos (102.78 nm) (P < 0.05) (53).
Seminal Exosomes (SE)
Seminal fluid is not only the carrier for sperm delivery, but also a signaling agent that interact with female reproductive tissues to facilitate conception (54, 55). EVs are indispensable bioactive signaling factors in the crosstalk between seminal fluid and female reproductive system (34–36).
SEs of humans and pigs have been demonstrated to participate in the immuno-inflammatory responses of endometrium, and regulate uterine microenvironment related to embryo implantation through the changes of chemokines and cytokines (34, 35). In addition, a recent study has confirmed that SEs can promote the prolactin secretion of eSCs during WOI and enhance in vitro decidualization of human eSCs (36). This excited finding suggests that mechanisms by which SEs influence embryo implantation may be diverse.
Oviductal EVs (oEVs)
Early embryonic development occurs in the oviduct, where an ideal microenvironment is provided by epithelial cells and by the oviductal fluid produced by these cells (56). OEVs are emerging as key players in the embryo-maternal interactions (37). During the process of bovine embryonic development, microRNA cargos of oEVs induce changes in embryonic gene expression which lead to a decrease of apoptosis of the embryonic cells and improve embryo viability which contribute to successful pregnancy (37, 38). The passage of gametes and the presence of embryo modulate microRNAs contents of oEVs (56), while the oviduct epithelial cell-derived exosomes improve the mitochondrial heath of in vitro-produced bovine embryos (39). Exosomes-treatment significantly upregulated the pyruvate dehydrogenase and glutamate dehydrogenase expression, required for metabolic fine-tuning of the TCA-cycle in the developing embryos (39). Melatonin was proved to be present in oviduct fluids and oEVs (40). The treatment of oEVs and melatonin enhances the in vitro development of embryo by regulating ROS and 5-mC levels (40).
Mesenchymal Stem Cell (MSC) -Derived EVs
As an advanced therapeutic strategy, MSC therapy have been applied in many fields. MSCs play therapeutic and recovery roles not only through cell differentiation but also by secreting various paracrine signaling factors into the environment (46). However, low survival rate, immunological rejection and inevitable risk of tumor transformation limit its promise (44).
As a cell-free structure, MSC-derived EVs seem to be more promising due to its advantages of higher biological stability and easier perfusion into tissues (18, 41, 47, 57). As a new paradigm for endometrial-embryo crosstalk, EVs light the path to the research of embryo implantation and bring hope for the therapy of infertility in the future.
The proteomic characteristics of EVs derived from human endometrial mesenchymal stem cells (endMSC) are related to embryonic development and implantation (58). It was reported that endMSC-EVs exert an exogenous ROS scavenger activity during embryo culture (41), increase the developmental ability of IVF-derived embryos of elderly women, presumably by modulating the expression of antioxidant enzymes and promoting pluripotent activity (41). EndMSC-EVs enhance embryo quality reflected by a significant increase in total cell number per blastocyst and embryo hatching, and support angiogenesis, vascularization, immunoregulation, differentiation and tissue remodeling of the endometrium after embryo hatching (42, 43).
In rats with intrauterine adhesion (IUA), exosomes derived from adipose-derived mesenchymal stem cells (ADSC-exo) has an angiogenic effect on endometrial regeneration. Besides, ADSC-exo upregulates the expression of integrin and leukemia inhibitory factor (LIF) which are recognized as classic markers of endometrial receptivity (44). Bone marrow mesenchymal stem cell (BMSC)-derived exosomes may promote endometrial repair by the TGF-β1/Smad signaling pathway (45). Exosomes derived from umbilical cord-derived mesenchymal stem cell (UC-MSC) can also promote endometrial regeneration and fertility recovery through immunomodulation (47).
EVs from various tissues can promote implantation function by participating in intercellular communication, which is beneficial to embryo implantation process (shown as Table 1). However, it’s also important to note that these results are mostly obtained through in vitro tests and subsequent in vivo experiments are needed for further verification.
Given their fundamental role in regulating intercellular communication, it is not surprising that in some pathological contexts EVs can also play a negative role in embryo implantation. For example, endometrium-derived EVs from women with recurrent implantation failure (RIF) attenuate the growth and invasion of embryos (59). Therefore, EVs play a dual role in the process of embryo implantation, which may be attributed to the heterogeneity of EVs contents. Similar situations will be discussed in the following sections.
RNA Cargos of EVs and Their Roles in Implantation
MicroRNAs (miRNAs)
MiRNAs are a class of small non-coding RNAs that regulate gene expression either negatively by inhibition of translational repression or positively through the targeting of gene promoters (60). EV is one of the main carriers of miRNA in vivo (60, 61). The bilayer phospholipid membrane structure of EVs protects miRNAs from degradation and contributes to their stability (26). Evidences suggested that heterogeneous nuclear ribonucleoprotein C1 (hnRNPC1) may be involved in the internalization of endometrial miR-30d into exosomes to prepare for its subsequent incorporation into trophectoderm cells (62, 63). However, it is still unknown whether this protein is generally involved in miRNAs integration in EVs. Emerging evidences suggest the considerable role of EVs-derived miRNAs in embryo implantation events (27, 60). Among them, members of lethal-7, miR-30, miR-21 families are especially remarkable (64). Other miRNAs, such as miRNA-17-92 cluster, miR-29a, etc., have been proved to be closely related to embryo implantation process despite the temporary lack of EVs-related evidences (64, 65). There are 18 EVs-related references in this section.
Lethal-7 Family
The let-7 family, which has 12 members up to now, is important for cell development and proliferation inhibition (66). The diversity of let-7 is particularly noticeable for the establishment of endometrial receptivity and embryonic development (66–69).
During the whole event of embryo implantation, the expression level of let-7 family is dynamic (66, 67). In the preparation stage of embryo implantation, up-regulated let-7a/g in endometrial epithelial cells enhance endometrial receptivity by inhibiting the classical Wnt signaling pathway (68). It is well known that the acquisition of endometrial receptivity mainly depends on the precise control of estrogen and progesterone (70, 71). The level of miR-let-7a is regulated by estrogen and progesterone (68), which suggests that let-7 may be involved in the regulation of steroid hormones on embryo implantation (68). Interestingly, the expression level of let-7 family members (including let-7a/g) was significantly decreased in the developed blastocysts during implantation (67), which is contrary to what is found in endometrial epithelial cells.
A recent study has shown that placental exosome-derived bta-miR-499-5p is involved in the inhibition of NF-κB through the Lin28B/let-7 axis (72). As a member of the let-7 family, EVs-derived miR-98 regulates the maternal immune system of endometrium during the period of peri-implantation by regulating immune-related genes such as CTSC, IL6, CASP4 and IKBKE (69). The effect of let-7 on the regulation of immune response may be related to the apoptosis of endometrial cells and embryo implantation (69, 72), but further experiments are needed. A recent study has expanded the understanding of the regulatory role of let-7 family on embryo. Let-7 is a major factor that induces diapause in embryos. Let-7-containing EVs from uterine fluid induce mouse embryonic diapause by inhibiting c-myc/mTORC1 and mTORC2 signaling pathways (73, 74). Over-expression of EVs-derived let-7 potentially hamper trophoblast differentiation and the implantation capacity of embryo (73, 74).
MicroRNA-30 Family
As mentioned above, hnRNPC1 may be involved in the internalization of endometrial miR-30d into exosomes to prepare for its subsequent incorporation into trophectoderm cells (62, 63). During WOI, up-regulated miR-30 family in human endometrial epithelium is secreted into uterine fluid as exosome-associated molecule (75, 76). Hsa-miR-30d, secreted by human endometrium and taken up by the pre-implantation embryo, might modify its transcriptome, increase the adhesion rate via indirect overexpression of genes encoding for certain molecules involved in embryonic adhesion phenomenon, such as Itgb3, Itga7 and Cdh5 (75).
MicroRNA-21 Family
The miR-21 family has anti-apoptotic effects on many cellular biological processes, including regulating anti-apoptotic ability of preimplantation embryos (77). In pregnant mice, increased EVs and miR-21 in uterine luminal fluid regulate the growth of fertilized eggs and embryo development via apoptosis-related gene (Bax, Bcl-2, etc.) (78). Sus scrofa (ssc)-miR-21-5p regulates endometrial epithelial cell proliferation, apoptosis and migration via programmed cell death 4 (PDCD4)/AKT pathway (79).
Of note, the research progress of EVs-related miRNAs in embryo implantation is not limited to the above-mentioned families. During implantation, the expression of EVs-derived miRNAs, such as miR-34c-5p, miR-210 are significantly up-regulated in extracellular environment of uterine (80). Exosomal miR-100-5p not only promotes angiogenesis during implantation, but also activates both FAK and JNK to enhance the implant potency of trophoblasts (81). Moreover, despite the temporary lack of EVs-related evidences, numerous miRNAs have also been proved to play a regulatory role in embryo implantation (64, 65). It’s worthy to study whether EVs participate in the interaction between these miRNAs and embryo implantation. The specific discussion is as follows.
Other Implantation-Related MiRNAs
MiRNA-17-92 cluster is up-regulated at implantation site during WOI (64). Similarly, miR-29a is highly expressed in uterus to control implantation events (65), which may be achieved by inhibiting the apoptosis of eSC via targeting the pro-apoptotic factor genes Bak1, Bmf and the anti-apoptotic factor gene Bcl-w. As we all know, Cox-2-derived prostaglandins are critical to implantation, and a research have found that miR-101a and miR-199a regulate the implantation process by regulating Cox-2 post transcriptionally (82). MiR-31 targets immunoregulatory factors like FOXP3, CXCL12 and so on to achieve optimal endometrial receptivity through immunosuppression mechanisms (83).
However, miRNAs are complex and precise regulatory factor, and some miRNAs play negative regulatory roles during the process of embryo implantation. Higher expression of miR-200 family members is found in the serum of infertility and abortion women compared with that of healthy women (84). In vitro experiments have demonstrated that miR-200c inhibits proliferation and receptive ability of uterine epithelial cells via miR-200c/FUT4/α-1,3-fucosylation (LeY)/CD44/Wnt/β-catenin signal pathway (84). In addition, miR-661, which is specifically secreted by implantation-incompetent blastocysts, negatively regulates the adhesion of trophoblasts onto epithelium via PVRL1 in vitro, and may be involved in the breakdown of intercellular contact and loss of epithelial cell polarity in endometrium (85). Higher expression of miR-181b is also found in degenerated bovine embryos compared with fine blastocysts (86).
The above results (summarized in Table 2) strongly support the important role of miRNAs in embryo implantation, and the analysis of miRNAs describe a promising picture of the future in assisted reproduction. But the expression profiles of miRNAs are very variable in these studies mainly because of the complexity of miRNA signals, different species of experimental animals and individual heterogeneity (60). So, the emergence of new methodologies for miRNA extraction and quantification is urgently needed, and the role of EVs in embryo implantation needs to be discussed in categories. In the seventh section of this review, we will focus on the adverse effects of EVs on pathological embryo implantation.
Long Non-Coding RNAs (lncRNAs)
As the most heterogeneous class of non-protein-coding RNA, with lengths ranging from 200 to 100,000 nt, lncRNAs are involved in almost all biological processes (87). EVs may be involved in the process of lncRNAs avoiding the degradation of ubiquitous RNase in body fluids, so as to reach target cells and play a regulatory role (87, 88). So far, there are few studies on EVs-derived lncRNAs in embryo implantation, but the research progress of EVs-derived lncRNAs in some endometrium-related diseases has brought clues for future research. EVs from endometriosis women are characterized by a unique miRNA-lncRNA signature (89), which may affect endometrial receptivity during WOI result in implantation failure (90). Decidualization and angiogenesis are typical changes in the endometrium during implantation, and antisense hypoxia-inducible factor (aHIF) is a well-known angiogenesis-related lncRNA (87). Endometriotic cyst stromal cells-derived exosomal aHIF induces angiogenesis by regulating angiogenesis-related genes in human umbilical vein endothelial cells (87). Some other specific lncRNAs are associated with endometrial physiopathology and embryo implantation (91, 92). LIF is a kind of cytokine secreted by endometrial glands and plays an amazingly important role in embryo implantation during WOI (93, 94). As a competing endogenous RNA for miR‐15b, lncRNA882 regulates LIF by sponging miR‐15b in endometrial epithelium cells of dairy goat (95). (There are 3 EVs-related references in this section.)
Protein Cargos of EVs and Their Roles in Implantation
Proteomic profiling of endometrium has revealed that endometrial exosomes contain a number of unique exosomal proteins not previously identified in exosomes from any other tissues (29). These endometrium-derived exosomal proteins are primarily regulated by estrogen and progesterone during menstrual cycle (29), which is consistent with the establishment of endometrial receptivity (70, 71). Importantly, proteomic changes in human trophectoderm function are demonstrated after endometrial EVs are internalized by human trophectoderm cells, which probably due to the transfer of EV protein cargos (26). Therefore, the expression differences of exosomal protein during the various stages of menstrual cycle may be closely related to embryo implantation. There are 5 EVs-related references in Section 6.
Among the numerous exosomal proteins, matrix metalloproteinase (MMP), which may have potential roles in embryo-maternal crosstalk during implantation has attracted much attention (22, 29). MMPs are widely expressed on maternal-fetal interface, responsible for extracellular matrix degradation and regulated by tissue inhibitors of MMPs (TIMPs) (96, 97). Different expression of MMP2, MMP14, and TIMP2 is observed in endometrium during various phases of the estrous cycle (98). MMPs contribute to the spatial and temporal matrix remodeling in bovine endometrium and may be related to the invasive ability of trophoblast cell (96). Some MMPs are relevant to endometrial angiogenesis (99), which is essential for vascularized receptive endometrium.
HLA-G is a key molecule in the process of embryo implantation, avoids the maternal immune rejection of the embryo by regulating the maternal-fetal immune response, and mediates communication with target cells in a variety of ways (100). The expression of HLA-G protein at the maternal-fetal interface is critical to the success of pregnancy, and HLA-G can be secreted with EVs (100).
So far, researches on the role of EVs-derived proteins in embryo implantation process are still not in-depth. In vitro experiments have discovered that 254 and 126 proteins are uniquely enriched in endometrial-derived exosomes during the proliferation and receiving phases, respectively (29). Whether these numerous and diverse proteins can provide appropriate conditions for embryo needs further explosion.
EVs and Embryo Implantation: A New Viewpoint of Reproductive Diseases
It is widely accepted that women with a history of reproductive diseases such as recurrent implantation failure (RIF), polycystic ovary syndrome (PCOS), and endometriosis, are associated with future impaired embryo implantation (101–105). EVs-based intercellular communication plays an important role in female reproductive microenvironments and is involved in the pathogenesis of these disorders (59, 89, 106–109). The regulatory disorders caused by abnormal EVs may provide a new viewpoint of implantation failure in women with reproductive diseases (summarized in Table 3). There are 9 EVs-related references in Section 7 (3 for endometriosis; 4 for PCOS; 2 for RIF).
EVs in Endometriosis
Endometriosis is defined by the presence of viable endometrial tissue outside the uterine cavity (103). A number of implantation markers such as αvβ3 integrin, LIF, homeobox A10 (HOXA10) and HOXA11 are aberrantly expressed in patients with endometriosis and may contribute to infertility in some women with endometriosis (103). Endometrial defects and defective endometrial-embryo cross-talk are both proposed mechanisms of implantation failure in endometriosis (103, 111). In recent years, the role of EVs in the process of implantation failure in endometriosis women is attracting increasing attention, and changes in the expression of certain proteins, lncRNAs and mRNAs may affect endometrial receptivity in rats with endometriosis during WOI, probably resulting in implantation failure of the embryo (89, 90, 108, 110).
EVs, including exosomes obtained from tissues (including endometrium, eutopic and ectopic endometriotic lesions, peritoneal fluid) and plasma samples of endometriosis women have unique miRNA-lncRNA characteristics (89). Forty-nine differentially expressed miRNAs are identified in eutopic endometrial stromal cells exosomes compared with that of normal endometrial stromal cells exosomes, and 12 miRNAs are predicted to target HOXA10, which is also a hopeful predictor of endometrial receptivity (112, 113), and/or the LIF 3’ untranslated region (110). In addition, higher levels of angiogenic and inflammatory cytokines are present in the human umbilical vein endothelial cells cocultured with the endometriotic epithelial exosomes (89, 108). The proinflammatory microenvironment stimulated by eutopic endometriotic lesions-derived EVs may also responsible for the disorders of embryo implantation in endometriosis (89, 108).
EVs in PCOS
PCOS, one of the most common endocrine disorders in women, affects 8-13% of women of reproductive age (114). Nowadays, sufficient evidences have proved that hormonal disturbances as well as metabolic changes in PCOS women can both affect endometrial receptivity and embryo implantation (101, 102).
At gene level, there is a differential gene expression in endometrium of PCOS detected by microarray evaluation (115, 116). It is noteworthy that most of these genes are involved in steroid hormone synthesis, inflammation and oxidative stress, which are indispensable for the establishment of endometrial receptivity (116). The proteome pattern of endometrium during WOI in PCOS women is significantly different with that of normal female (117, 118). These differences in transcription, post-transcriptional modification and translation are often related to metabolism, cell cycle, DNA repair, apoptosis and signal transduction (116), and may consequently cause impaired endometrial receptivity in PCOS women. But the specific mechanisms are still unknown. Researches on EVs may provide new insights into the further understanding of implantation failure in PCOS.
RNA sequencing microarray and proteomic analysis have shown differentially expressed small RNAs, circRNAs, and 86 proteins in follicular fluid exosomes of PCOS women (106, 107, 119, 120). The alterations of the proteomic profile of PCOS women are related to the inflammation, reactive oxygen species metabolic process, cell migration and proliferation (106), which are closely related to embryo implantation. S100‐A9 protein in exosomes derived from follicular fluid promotes inflammation and causes disorders of ovarian steroidogenesis via activation of NF‐κB pathway (106). The down-regulated exosomal circLDLR in follicle fluid of PCOS women is proposed to involve in abnormal estrogen secretion as well (107).
Chronic inflammation is an acknowledged cause of endometrial physiological dysfunction (121, 122). Excessive inflammation results in the adverse pregnancy outcomes in PCOS and endometriosis. The articles above (106, 107) specialize in local inflammation and steroidogenesis of the ovary, and the disorders of ovarian steroidogenesis and abnormal inflammatory state caused by follicle fluid exosomal cargos may also be the potential mechanisms of the disorders of embryo implantation in PCOS.
EVs in RIF
RIF refers to the repeated transfer of morphologically good embryos to a normal uterus without achieving successful implantation and a clinical pregnancy (123). Traditionally, the failure to achieve a clinical pregnancy after transfer of at least four good-quality embryos in a minimum of three fresh or frozen cycles in a woman under the age of 40 years is defined as RIF (105). The etiologies and pathogenesis of RIF is unknown, and clarifying the mechanisms of EVs in RIF may provide benefits to the treatments of embryo implantation disorders.
Altered miRNA profiles in RIF-EVs might be involved in the pathogenesis of RIF. The up-regulated miR-1246 and miR-1290 in the RIF-EVs may indicate the inadequate endometrial receptivity of RIF women (124). In vitro experimental studies have demonstrated that endometrial EVs from RIF women attenuate embryonic development and implantation capacity by inhibiting blastocyst formation, decreasing the total cell number of embryos as well as inhibiting the proliferation, migration, and invasion of trophoblast cells (59, 109).
Prospects and Conclusions
In conclusion, as one of the important communication mechanisms that modulate blastocyst and endometrial functions, EVs play an important role in promoting embryo implantation, which mainly owes to EVs’s unique ability in transferring heterogeneous cargos (summarized in Figure 2) (125). Abnormal EVs play a negative role in some pathological conditions.
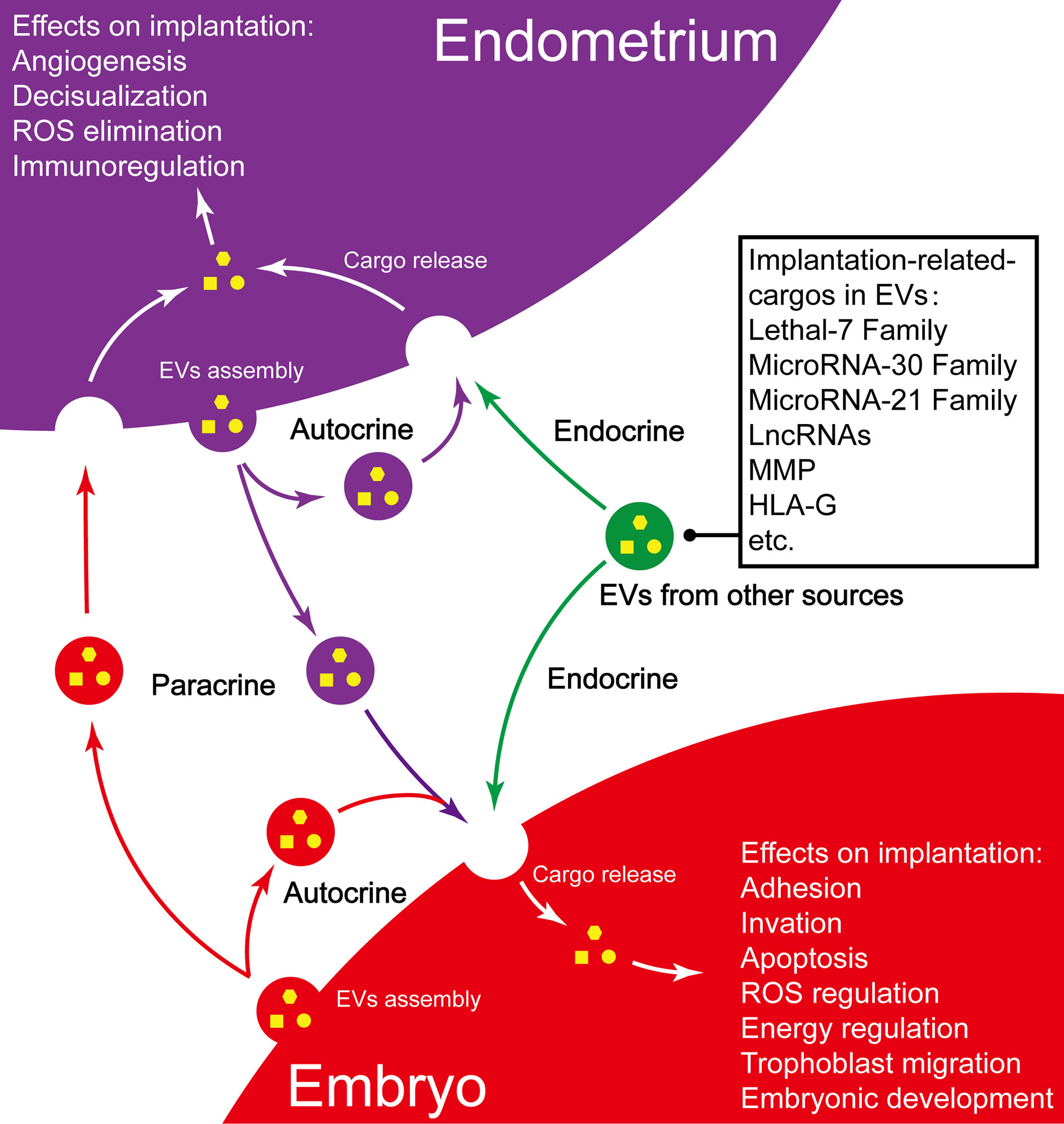
Figure 2 Summary of EVs and their implication in embryo implantation. Purple vesicles, endometrium-derived EV; Red vesicles, embryo-derived EVs; Green vesicles, EVs from other sources.
EVs have shown great potential as molecular biomarkers in diagnosis, prognosis and also as possible therapeutic targets. Currently, endometrial dysfunction is the biggest obstacle to the treatment of embryo implantation disorders. EVs derived from serum and uterine fluid may be used, as a noninvasive and accurate marker of endometrial station, to identify women with implantation defects, demonstrate the optimal timing for embryo transfer, and replace the traditional endometrial biopsy (83, 126, 127). EVs-based preparation may be a promising approach to endometrial regeneration and improving pregnancy outcomes (128).
But the precise mechanisms of EVs regulating embryo implantation have not been elucidated enough. The following points deserve attention and improvement particularly: 1. the data of EVs cargos are mainly derived from immortalized or long-term passaged cell lines. 2. there is still no golden standard of EVs separation, concentration and purification, and no acknowledged nomenclature of subclassing EVs with various biophysical properties. 3. the experimental data of effects of EVs in embryo implantation is largely based on in vitro trophoblast adhesion/invasion assays, but the corresponding in vivo evidence has not been rigorously established. 4. current understanding of EVs has been limited to protein and RNA cargos, but the role of EVs-derived DNA and lipid molecules in embryo implantation remains unknown. So further exploration of the effects of EVs and their cargos on embryo implantation is still needed.
Author Contributions
N-XJ wrote the manuscript. X-LL designed the topic and critically revised our work. All authors contributed to the article and approved the submitted version.
Funding
This study was funded by Natural Science Foundation from Science and Technology Commission of Shanghai Municipality (grant No. 17ZR1403100 to X-LL).
Conflict of Interest
The authors declare that the research was conducted in the absence of any commercial or financial relationships that could be construed as a potential conflict of interest.
Acknowledgments
The authors would like to thank the support from Natural Science Foundation from Science and Technology Commission of Shanghai Municipality.
References
1. Wilcox AJ, Baird DD, Wenberg CR. Time of Implantation of the Conceptus and Loss of Pregnancy. N Engl J Med (1999) 340(23):1796–9. doi: 10.1056/NEJM199906103402304
2. Ashary N, Tiwari A, Modi D. Embryo Implantation: War in Times of Love. Endocrinology (2018) 159(2):1188–98. doi: 10.1210/en.2017-03082
3. Macklon NS, Geraedts JPM, Fauser B. Conception to Ongoing Pregnancy: The ‘Black Box’ of Early Pregnancy Loss. Hum Reprod Update (2002) 8(4):333–43. doi: 10.1093/humupd/8.4.333
4. Wilcox AJ, Weinberg CR, Oconnor JF, Baird DD, Schlatterer JP, Canfield RE, et al. Incidence Of Early Loss of Pregnancy. N Engl J Med (1988) 319(4):189–94. doi: 10.1056/NEJM198807283190401
5. Miller PB, Parnell BA, Bushnell G, Tallman N, Forstein DA, Higdon HL, et al. Endometrial Receptivity Defects During IVF Cycles With and Without Letrozole. Hum Reprod (2012) 27(3):881–8. doi: 10.1093/humrep/der452
6. Norwitz ER, Schust DJ, Fisher SJ. Mechanisms of Disease - Implantation and the Survival of Early Pregnancy. N Engl J Med (2001) 345(19):1400–8. doi: 10.1056/NEJMra000763
7. Adam H, Balen1 S-LT, MacDougall J, Jacobs HS. Miscarriage Rates Following In-Vitro Fertilization Are Increased in Women With Polycystic Ovaries and Reduced by Pituitary Desensitization With Buserelin. Hum Reprod (1993) 8:959–64. doi: 10.1093/oxfordjournals.humrep.a138174
8. Steiner N, Ates S, Shaulov T, Shrem G, Volodarsky-Perel A, Dahan SY, et al. A Comparison of IVF Outcomes Transferring a Single Ideal Blastocyst in Women With Polycystic Ovary Syndrome and Normal Ovulatory Controls. Arch Gynecol Obstet (2020) 302(6):1479–86. doi: 10.1007/s00404-020-05699-9
9. Psychoyos A. Uterine Receptivity for Nidation. Ann NY Acad Sci (1986) 476:36–42. doi: 10.1111/j.1749-6632.1986.tb20920.x
10. Egashira M, Hirota Y. Uterine Receptivity and Embryo–Uterine Interactions in Embryo Implantation: Lessons From Mice. Reprod Med Biol (2013) 12(4):127–32. doi: 10.1007/s12522-013-0153-1
11. Aplin JD, Ruane PT. Embryo–Epithelium Interactions During Implantation at a Glance. J Cell Sci (2017) 130(1):15–22. doi: 10.1242/jcs.175943
12. Dey SK, Lim H, Das SK, Reese J, Paria BC, Daikoku T, et al. Molecular Cues to Implantation. Endocrine Rev (2004) 25(3):341–73. doi: 10.1210/er.2003-0020
13. Salamonsen LA, Evans J, Nguyen HP, Edgell TA. The Microenvironment of Human Implantation: Determinant of Reproductive Success. Am J Reprod Immunol (2016) 75(3):218–25. doi: 10.1111/aji.12450
14. Poon CE, Madawala RJ, Dowland SN, Murphy CR. Nectin-3 Is Increased in the Cell Junctions of the Uterine Epithelium at Implantation. Reprod Sci (2016) 23(11):1580–92. doi: 10.1177/1933719116648216
15. Wu JJ, Taylor RN, Sidell N. Retinoic Acid Regulates Gap Junction Intercellular Communication in Human Endometrial Stromal Cells Through Modulation of the Phosphorylation Status of Connexin 43. J Cell Physiol (2013) 228(4):903–10. doi: 10.1002/jcp.24241
16. Grund S, Grummer R. Direct Cell-Cell Interactions in the Endometrium and in Endometrial Pathophysiology. Int J Mol Sci (2018) 19(8):30. doi: 10.3390/ijms19082227
17. Liu Y, Shen Q, Zhang L, Xiang W. Extracellular Vesicles: Recent Developments in Aging and Reproductive Diseases. Front Cell Dev Biol (2020) 8:577084. doi: 10.3389/fcell.2020.577084
18. Desrochers LM, Bordeleau F, Reinhart-King CA, Cerione RA, Antonyak MA. Microvesicles Provide a Mechanism for Intercellular Communication by Embryonic Stem Cells During Embryo Implantation. Nat Commun (2016) 7:11958. doi: 10.1038/ncomms11958
19. Jaiswal R, Sedger LM. Intercellular Vesicular Transfer by Exosomes, Microparticles and Oncosomes - Implications for Cancer Biology and Treatments. Front Oncol (2019) 9:125. doi: 10.3389/fonc.2019.00125
20. van der Pol E, Boing AN, Harrison P, Sturk A, Nieuwland R. Classification, Functions, and Clinical Relevance of Extracellular Vesicles. Pharmacol Rev (2012) 64(3):676–705. doi: 10.1124/pr.112.005983
21. Kim J, Lee J, Lee TB, Jun JH. Embryotrophic Effects of Extracellular Vesicles Derived From Outgrowth Embryos in Pre- and Peri-Implantation Embryonic Development in Mice. Mol Reprod Dev (2019) 86(2):187–96. doi: 10.1002/mrd.23093
22. Latifi Z, Fattahi A, Ranjbaran A, Nejabati HR, Imakawa K. Potential Roles of Metalloproteinases of Endometrium-Derived Exosomes in Embryo-Maternal Crosstalk During Implantation. J Cell Physiol (2018) 233(6):4530–45. doi: 10.1002/jcp.26259
23. Kurian NK, Modi D. Extracellular Vesicle Mediated Embryo-Endometrial Cross Talk During Implantation and in Pregnancy. J Assisted Reprod Genet (2019) 36(2):189–98. doi: 10.1007/s10815-018-1343-x
24. Jankovicova J, Secova P, Michalkova K, Antalikova J. Tetraspanins, More Than Markers of Extracellular Vesicles in Reproduction. Int J Mol Sci (2020) 21(20):1–30. doi: 10.3390/ijms21207568
25. Mishra A, Ashary N, Sharma R, Modi D. Extracellular Vesicles in Embryo Implantation and Disorders of the Endometrium. Am J Reprod Immunol (2020) 85(2):e13360. doi: 10.1111/aji.13360
26. Evans J, Rai A, Nguyen HPT, Poh QH, Elglass K, Simpson RJ, et al. Human Endometrial Extracellular Vesicles Functionally Prepare Human Trophectoderm Model for Implantation: Understanding Bidirectional Maternal-Embryo Communication. Proteomics (2019) 19(23):e1800423. doi: 10.1002/pmic.201800423
27. Ng YH, Rome S, Jalabert A, Forterre A, Singh H, Hincks CL, et al. Endometrial Exosomes/Microvesicles in the Uterine Microenvironment: A New Paradigm for Embryo-Endometrial Cross Talk at Implantation. PLoS One (2013) 8(3):e58502. doi: 10.1371/journal.pone.0058502
28. Giacomini E, Vago R, Sanchez AM, Podini P, Zarovni N, Murdica V, et al. Secretome of In Vitro Cultured Human Embryos Contains Extracellular Vesicles That are Uptaken by the Maternal Side. Sci Rep (2017) 7(1):5210. doi: 10.1038/s41598-017-05549-w
29. Greening DW, Nguyen HPT, Elgass K, Simpson RJ, Salamonsen LA. Human Endometrial Exosomes Contain Hormone-Specific Cargo Modulating Trophoblast Adhesive Capacity: Insights Into Endometrial-Embryo Interactions. Biol Reprod (2016) 94(2):38. doi: 10.1095/biolreprod.115.134890
30. Gurung S, Greening DW, Catt S, Salamonsen L, Evans J. Exosomes and Soluble Secretome From Hormone-Treated Endometrial Epithelial Cells Direct Embryo Implantation. Mol Hum Reprod (2020) 26(7):510–20. doi: 10.1093/molehr/gaaa034
31. Wang X, Li Q, Xie T, Yuan M, Sheng X, Qi X, et al. Exosomes From Bovine Endometrial Epithelial Cells Ensure Trophoblast Cell Development by miR-218 Targeting Secreted Frizzled Related Protein 2. J Cell Physiol (2021) 236(6):4565–79. doi: 10.1002/jcp.30180
32. Liu M, Chen X, Chang QX, Hua R, Wei YX, Huang LP, et al. Decidual Small Extracellular Vesicles Induce Trophoblast Invasion by Upregulating N-Cadherin. Reproduction (2020) 159(2):171–80. doi: 10.1530/REP-18-0616
33. Es-Haghi M, Godakumara K, Haling A, Lattekivi F, Lavrits A, Viil J, et al. Specific Trophoblast Transcripts Transferred by Extracellular Vesicles Affect Gene Expression in Endometrial Epithelial Cells and May Have a Role in Embryo-Maternal Crosstalk. Cell Commun Signal (2019) 17(1):146. doi: 10.1186/s12964-019-0448-x
34. Bai R, Latifi Z, Kusama K, Nakamura K, Shimada M, Imakawa K. Induction of Immune-Related Gene Expression by Seminal Exosomes in the Porcine Endometrium. Biochem Biophys Res Commun (2018) 495(1):1094–101. doi: 10.1016/j.bbrc.2017.11.100
35. Paktinat S, Hashemi SM, Ghaffari Novin M, Mohammadi-Yeganeh S, Salehpour S, Karamian A, et al. Seminal Exosomes Induce Interleukin-6 and Interleukin-8 Secretion by Human Endometrial Stromal Cells. Eur J Obstetrics Gynecol Reprod Biol (2019) 235:71–6. doi: 10.1016/j.ejogrb.2019.02.010
36. Rodriguez-Caro H, Dragovic R, Shen M, Dombi E, Mounce G, Field K, et al. In Vitro Decidualisation of Human Endometrial Stromal Cells is Enhanced by Seminal Fluid Extracellular Vesicles. J Extracellular Vesicles (2019) 8(1):1565262. doi: 10.1080/20013078.2019.1565262
37. Bauersachs S, Mermillod P, Alminana C. The Oviductal Extracellular Vesicles’ RNA Cargo Regulates the Bovine Embryonic Transcriptome. Int J Mol Sci (2020) 21(4):1303. doi: 10.3390/ijms21041303
38. Lopera-Vasquez R, Hamdi M, Maillo V, Gutierrez-Adan A, Bermejo-Alvarez P, Angel Ramirez M, et al. Effect of Bovine Oviductal Extracellular Vesicles on Embryo Development and Quality In Vitro. Reproduction (2017) 153(4):461–70. doi: 10.1530/REP-16-0384
39. Sidrat T, Khan AA, Joo MD, Wei Y, Lee KL, Xu L, et al. Bovine Oviduct Epithelial Cell-Derived Culture Media and Exosomes Improve Mitochondrial Health by Restoring Metabolic Flux During Pre-Implantation Development. Int J Mol Sci (2020) 21(20):1–22. doi: 10.3390/ijms21207589
40. Qu P, Luo S, Du Y, Zhang Y, Song X, Yuan X, et al. Extracellular Vesicles and Melatonin Benefit Embryonic Develop by Regulating Reactive Oxygen Species and 5-Methylcytosine. J Pineal Res (2020) 68(3):e12635. doi: 10.1111/jpi.12635
41. Marinaro F, Macias-Garcia B, Sanchez-Margallo FM, Blazquez R, Alvarez V, Matilla E, et al. Extracellular Vesicles Derived From Endometrial Human Mesenchymal Stem Cells Enhance Embryo Yield and Quality in an Aged Murine Model. Biol Reprod (2019) 100(5):1180–92. doi: 10.1093/biolre/ioy263
42. Alvarez V, Sanchez-Margallo FM, Macias-Garcia B, Gomez-Serrano M, Jorge I, Vazquez J, et al. The Immunomodulatory Activity of Extracellular Vesicles Derived From Endometrial Mesenchymal Stem Cells on CD4+ T Cells Is Partially Mediated by Tgfbeta. J Tissue Eng Regener Med (2018) 12(10):2088–98. doi: 10.1002/term.2743
43. Blazquez R, Sanchez-Margallo FM, Alvarez V, Matilla E, Hernandez N, Marinaro F, et al. Murine Embryos Exposed to Human Endometrial MSCs-derived Extracellular Vesicles Exhibit Higher VEGF/PDGF AA Release, Increased Blastomere Count and Hatching Rates. PLoS One (2018) 13(4):e0196080. doi: 10.1371/journal.pone.0196080
44. Zhao S, Qi W, Zheng J, Tian Y, Qi X, Kong D, et al. Exosomes Derived From Adipose Mesenchymal Stem Cells Restore Functional Endometrium in a Rat Model of Intrauterine Adhesions. Reprod Sci (2020) 27(6):1266–75. doi: 10.1007/s43032-019-00112-6
45. Yao Y, Chen R, Wang G, Zhang Y, Liu F. Exosomes Derived From Mesenchymal Stem Cells Reverse EMT Via TGF-beta1/Smad Pathway and Promote Repair of Damaged Endometrium. Stem Cell Res Ther (2019) 10(1):225. doi: 10.1186/s13287-019-1332-8
46. Ebrahim N, Mostafa O, El Dosoky RE, Ahmed IA, Saad AS, Mostafa A, et al. Human Mesenchymal Stem Cell-Derived Extracellular Vesicles/Estrogen Combined Therapy Safely Ameliorates Experimentally Induced Intrauterine Adhesions in a Female Rat Model. Stem Cell Res Ther (2018) 9(1):175. doi: 10.1186/s13287-018-0924-z
47. Xin L, Lin X, Zhou F, Li C, Wang X, Yu H, et al. A Scaffold Laden With Mesenchymal Stem Cell-Derived Exosomes for Promoting Endometrium Regeneration and Fertility Restoration Through Macrophage Immunomodulation. Acta Biomaterialia (2020) 113:252–66. doi: 10.1016/j.actbio.2020.06.029
48. Nguyen HPT, Greening DW, Elgass K, Simpson RJ, Salamonsen LA. Proteomic and Functional Characterization of Human Endometrial Epithelial Exosomes Reveal Cargo Proteins Essential for Embryo-Maternal Interactions. Clin Endocrinol (2016) 1):49. doi: 10.1111/cen.13010
49. Nguyen H, Elgass K, Simpson R, Salamonsen L, Greening D. Endometrial Extracellular Vesicles Are Important for Maternal-Embryo Communications. J Extracellular Vesicles (2016) 5:31. doi: 10.3402/jev.v5.31552
50. Bolumar D, Amadoz A, Moreno I, Simon C, Vilella F. Embryo ATP Production can be Modulated by Maternal Mitochondrial DNA Secreted From the Human Endometrium in Extracellular Vesicles. Fertility Sterility (2019) 112:e314. doi: 10.1016/j.fertnstert.2019.07.912
51. Rai A, Poh QH, Fatmous M, Fang H, Gurung S, Vollenhoven B, et al. Proteomic Profiling of Human Uterine Extracellular Vesicles Reveal Dynamic Regulation of Key Players of Embryo Implantation and Fertility During Menstrual Cycle. Proteomics (2021) e2000211. doi: 10.1002/pmic.202000211
52. Qu P, Qing S, Liu R, Qin H, Wang W, Qiao F, et al. Effects Embryo-Derived Exosomes on the Development of Bovine Cloned Embryos. PLoS One (2017) 12(3):e0174535. doi: 10.1371/journal.pone.0174535
53. Veraguas D, Aguilera C, Henriquez C, Velasquez AE, Melo-Baez B, Silva-Ibanez P, et al. Evaluation of Extracellular Vesicles and gDNA From Culture Medium as a Possible Indicator of Developmental Competence in Human Embryos. Zygote (Cambridge England) (2021) 29(2):138–49. doi: 10.1017/S0967199420000593
54. Saint-Dizier M, Mahe C, Reynaud K, Tsikis G, Mermillod P, Druart X. Sperm Interactions With the Female Reproductive Tract: A Key for Successful Fertilization in Mammals. Mol Cell Endocrinol (2020) 516:110956. doi: 10.1016/j.mce.2020.110956
55. Schjenken JE, Robertson SA. The Female Response To Seminal Fluid. Physiol Rev (2020) 100(3):1077–117. doi: 10.1152/physrev.00013.2018
56. Mazzarella R, Bastos NM, Bridi A, del Collado M, Andrade GM, Pinzon J, et al. Changes in Oviductal Cells and Small Extracellular Vesicles miRNAs in Pregnant Cows. Front Veterinary Sci (2021) 8:639752. doi: 10.3389/fvets.2021.639752
57. El Andaloussi S, Maeger I, Breakefield XO, Wood MJA. Extracellular Vesicles: Biology and Emerging Therapeutic Opportunities. Nat Rev Drug Discov (2013) 12(5):348–58. doi: 10.1038/nrd3978
58. Marinaro F, Alvarez V, Blazquez R, Lopez Nieto E, MacIas-Garcia B, Gomez Serrano M, et al. Proteomic Profiling of the Exosomes Released by Endometrial Mesenchymal Stem Cells: Possible Relations With Embryo Development and Implantation. Hum Reprod (2018) 33:i506–i7. doi: 10.26226/morressier.5af300b4738ab10027aa9b23
59. Liu C, Yao W, Yao J, Li L, Yang L, Zhang H, et al. Endometrial Extracellular Vesicles From Women With Recurrent Implantation Failure Attenuate the Growth and Invasion of Embryos. Fertility Sterility (2020) 114(2):416–25. doi: 10.1016/j.fertnstert.2020.04.005
60. Paul ABM, Sadek ST, Mahesan AM. The Role of microRNAs in Human Embryo Implantation: A Review. J Assisted Reprod Genet (2019) 36(2):179–87. doi: 10.1007/s10815-018-1326-y
61. Sun Z, Shi K, Yang S, Liu J, Zhou Q, Wang G, et al. Effect of Exosomal miRNA on Cancer Biology and Clinical Applications. Mol Cancer (2018) 17(1):147. doi: 10.1186/s12943-018-0897-7
62. Balaguer N, Moreno I, Herrero M, Gonzalez M, Simon C, Vilella F. Heterogeneous Nuclear Ribonucleoprotein C1may Control miR-30d Levels in Endometrial Exosomes Affecting Early Embryo Implantation. Mol Hum Reprod (2018) 24(8):411–25. doi: 10.1093/molehr/gay026
63. Balaguer N, Moreno I, Herrero M, Simon C, Vilella F. Maternal Endometrial HSA-MIR-30D Is Internalized and Secreted in Exosomes by Binding With Hnrnpc1. Reprod Sci (2016) 1):66A–7A. doi: 10.1177/1933719116641257
64. Liu W, Niu Z, Li Q, Pang RTK, Chiu PCN, Yeung WSB. MicroRNA and Embryo Implantation. Am J Reprod Immunol (2016) 75(3):263–71. doi: 10.1111/aji.12470
65. Xia HF, Jin XH, Cao ZF, Hu Y, Ma X. MicroRNA Expression and Regulation in the Uterus During Embryo Implantation in Rat. FEBS J (2014) 281(7):1872–91. doi: 10.1111/febs.12751
66. Li Q, Chiu PCN, Yeung WSB, Liu WM. Regulation of Human Trophoblast Surrogate Jeg-3 Spheroids Implantation Potential by Wnt/Beta-Catenin Pathway and lin28a/let-7a Axis. Exp Cell Res (2020) 388(1):111718. doi: 10.1016/j.yexcr.2019.111718
67. Li Q, Shi JZ, Liu WM. The Role of Wnt/beta-catenin-lin28a/let-7 Axis in Embryo Implantation Competency and Epithelial-Mesenchymal Transition (EMT). Cell Communication Signaling (2020) 18(1):108. doi: 10.1186/s12964-020-00562-5
68. Li Q, Liu WM, Chiu PCN, Yeung WSB. Mir-Let-7a/G Enhances Uterine Receptivity Via Suppressing Wnt/Beta-Catenin Under the Modulation of Ovarian Hormones. Reprod Sci (2020) 27(5):1164–74. doi: 10.1007/s43032-019-00115-3
69. Nakamura K, Kusama K, Ideta A, Kimura K, Hori M, Imakawa K. Effects of miR-98 in Intrauterine Extracellular Vesicles on Maternal Immune Regulation During the Peri-Implantation Period in Cattle. Sci Rep (2019) 9(1):20330. doi: 10.1038/s41598-019-56879-w
70. Griffiths RM, Pru CA, Behura SK, Cronrath AR, McCallum ML, Kelp NC, et al. AMPK is Required for Uterine Receptivity and Normal Responses to Steroid Hormones. Reproduction (2020) 159(6):707–17. doi: 10.1530/REP-19-0402
71. Paulson RJ. Introduction: Endometrial Receptivity: Evaluation, Induction and Inhibition. Fertil Steril (2019) 111(4):609–10. doi: 10.1016/j.fertnstert.2019.02.029
72. Zhao G, Yang C, Yang J, Liu P, Jiang KF, Shaukat A, et al. Placental Exosome-Mediated Bta-miR-499-Lin28B/let-7 Axis Regulates Inflammatory Bias During Early Pregnancy. Cell Death Dis (2018) 9(6):704. doi: 10.1038/s41419-018-0713-8
73. Liu W, Niu Z, Yeung W. The Roles of Let-7 Contained in Extracellular Vesicles (EV) in, Embryo Implantation in Human. Hum Reproduction (2018) 33:i363–i4. doi: 10.1093/humrep/33.Supplement_1.1
74. Liu WM, Cheng RR, Niu ZR, Chen AC, Ma MY, Li T, et al. Let-7 Derived From Endometrial Extracellular Vesicles is an Important Inducer of Embryonic Diapause in Mice. Sci Adv (2020) 6(37):eaaz7070. doi: 10.1126/sciadv.aaz7070
75. Vilella F, Moreno-Moya JM, Balaguer N, Grasso A, Herrero M, Martinez S, et al. Hsa-miR-30d, Secreted by the Human Endometrium, is Taken Up by the Pre-Implantation Embryo and Might Modify its Transcriptome. Development (2015) 142(18):3210–U232. doi: 10.1242/dev.124289
76. Moreno-Moya JM, Vilella F, Martinez S, Pellicer A, Simon C. The Transcriptomic and Proteomic Effects of Ectopic Overexpression of miR-30d in Human Endometrial Epithelial Cells. Mol Hum Reprod (2014) 20(6):550–66. doi: 10.1093/molehr/gau010
77. Shen XH, Han YJ, Zhang DX, Cui XS, Kim NH. A Link Between the Interleukin-6/Stat3 Anti-Apoptotic Pathway and MicroRNA-21 in Preimplantation Mouse Embryos. Mol Reprod Dev (2009) 76(9):854–62. doi: 10.1002/mrd.21048
78. Lv C, Yu WX, Wang Y, Yi DJ, Zeng MH, Xiao HM. MiR-21 in Extracellular Vesicles Contributes to the Growth of Fertilized Eggs and Embryo Development in Mice. Biosci Rep (2018) 38(4):BSR20180036. doi: 10.1042/BSR20180036
79. Hua RW, Zhang XL, Li WC, Lian WS, Liu QR, Gao DY, et al. Ssc-miR-21-5p Regulates Endometrial Epithelial Cell Proliferation, Apoptosis and Migration Via the PDCD4/AKT Pathway. J Cell Sci (2020) 133(23):jcs248898. doi: 10.1242/jcs.248898
80. Tan Q, Shi S, Liang J, Zhang X, Cao D, Wang Z. Micrornas in Small Extracellular Vesicles Indicate Successful Embryo Implantation During Early Pregnancy. Cells (2020) 93):645. doi: 10.21203/rs.2.21948/v1
81. Tan Q, Shi S, Liang J, Cao D, Wang S, Wang Z. Endometrial Cell-Derived Small Extracellular Vesicle miR-100-5p Promotes Functions of Trophoblast During Embryo Implantation. Mol Ther - Nucleic Acids (2021) 23:217–31. doi: 10.1016/j.omtn.2020.10.043
82. Chakrabarty A, Tranguch S, Daikoku T, Jensen K, Furneaux H, Dey SK. MicroRNA Regulation of Cyclooxygenase-2 During Embryo Implantation. Proc Natl Acad Sci USA (2007) 104(38):15144–9. doi: 10.1073/pnas.0705917104
83. Kresowik JDK, Devor EJ, Van Voorhis BJ, Leslie KK. Microrna-31 Is Significantly Elevated in Both Human Endometrium and Serum During the Window of Implantation: A Potential Biomarker for Optimum Receptivity. Biol Reprod (2014) 91(1):17. doi: 10.1095/biolreprod.113.116590
84. Zheng Q, Zhang DD, Yang Y, Cui XY, Sun JQ, Liang CX, et al. MicroRNA-200c Impairs Uterine Receptivity Formation by Targeting FUT4 and Alpha 1,3-Fucosylation. Cell Death Differ (2017) 24(12):2161–72. doi: 10.1038/cdd.2017.136
85. Cuman C, Van Sinderen M, Gantier MP, Rainczuk K, Sorby K, Rombauts L, et al. Human Blastocyst Secreted Microrna Regulate Endometrial Epithelial Cell Adhesion. Ebiomedicine (2015) 2(10):1528–35. doi: 10.1016/j.ebiom.2015.09.003
86. Lange-Consiglio A, Lazzari B, Pizzi F, Idda A, Cremonesi F, Capra E. MicroRNA Profile of In Vitro Bovine Embryos Cultured in the Presence of Amniotic Extracellular Vesicles Shifts Toward In Vivo-Collected Blastocysts. Reproduction Fertility Dev (2020) 321-2):170. doi: 10.1071/RDv32n2Ab88
87. Qiu JJ, Lin XJ, Zheng TT, Tang XY, Zhang Y, Hua KQ. The Exosomal Long Noncoding Rna aHIF is Upregulated in Serum From Patients With Endometriosis and Promotes Angiogenesis in Endometriosis. Reprod Sci (2019) 26(12):1590–602. doi: 10.1177/1933719119831775
88. Qiu JJ, Lin YY, Tang XY, Ding Y, Yi XF, Hua KQ. Extracellular Vesicle-Mediated Transfer of the lncRNA-TC0101441 Promotes Endometriosis Migration/Invasion. Exp Cell Res (2020) 388(1):111815. doi: 10.1016/j.yexcr.2020.111815
89. Khalaj K, Miller JE, Lingegowda H, Fazleabas AT, Young SL, Lessey BA, et al. Extracellular Vesicles From Endometriosis Patients are Characterized by a Unique miRNA-lncRNA Signature. JCI Insight (2019) 4(18):19. doi: 10.1172/jci.insight.128846
90. Cai H, Zhu X, Li Z, Zhu Y, Lang J. lncRNA/mRNA Profiling of Endometriosis Rat Uterine Tissues During the Implantation Window. Int J Mol Med (2019) 44(6):2145–60. doi: 10.3892/ijmm.2019.4370
91. Hong L, Hu Q, Zang X, Xie Y, Zhou C, Zou X, et al. Analysis and Screening of Reproductive Long Non-coding Rnas Through Genome-Wide Analyses of Goat Endometrium During the Pre-Attachment Phase. Front Genet (2020) 11:568017. doi: 10.3389/fgene.2020.568017
92. Maduro MR. A Role for Long Noncoding RNAs in Implantation Failure. Reprod Sci (2019) 26(1):5. doi: 10.1177/1933719118818726
93. Bhatt H, Brunet LJ, Stewart CL. Uterine Expression of Leukemia Inhibitory Factor Coincides With the Onset of Blastocyst Implantation. Proc Natl Acad Sci USA (1991) 88(24):11408–12. doi: 10.1073/pnas.88.24.11408
94. Chen JR, Cheng JG, Shatzer T, Sewell L, Hernandez L, Stewart CL. Leukemia Inhibitory Factor can Substitute for Nidatory Estrogen and is Essential to Inducing a Receptive Uterus for Implantation But is Not Essential for Subsequent Embryogenesis. Endocrinology (2000) 141(12):4365–72. doi: 10.1210/endo.141.12.7855
95. Zhang L, Liu X, Cui J, Che S, Liu Y, An X, et al. LncRNA882 Regulates Leukemia Inhibitory Factor (LIF) by Sponging miR-15b in the Endometrial Epithelium Cells of Dairy Goat. J Cell Physiol (2019) 234(4):4754–67. doi: 10.1002/jcp.27272
96. Anacker J, Segerer SE, Hagemann C, Feix S, Kapp M, Bausch R, et al. Human Decidua and Invasive Trophoblasts are Rich Sources of Nearly All Human Matrix Metalloproteinases. Mol Hum Reprod (2011) 17(10):637–52. doi: 10.1093/molehr/gar033
97. Bai SX, Wang YL, Qin L, Xiao ZJ, Herva R, Piao YS. Dynamic Expression of Matrix Metalloproteinases (MMP-2, -9 and -14) and the Tissue Inhibitors of MMPs (Timp-1, -2 and -3) at the Implantation Site During Tubal Pregnancy. Reproduction (2005) 129(1):103–13. doi: 10.1530/rep.1.00283
98. Ulbrich SE, Meyer SU, Zitta K, Hiendleder S, Sinowatz F, Bauersachs S, et al. Bovine Endometrial Metallopeptidases MMP14 and MMP2 and the Metallopeptidase Inhibitor TIMP2 Participate in Maternal Preparation of Pregnancy. Mol Cell Endocrinol (2011) 332(1-2):48–57. doi: 10.1016/j.mce.2010.09.009
99. Plaisier M, Koolwijk P, Hanemaaijer R, Verwey RA, van der Weiden RMF, Risse EKJ, et al. Membrane-Type Matrix Metalloproteinases and Vascularization in Human Endometrium During the Menstrual Cycle. Mol Hum Reprod (2006) 12(1):11–8. doi: 10.1093/molehr/gah257
100. Nardi FS, Slowik R, Michelon T, Manvailer LFS, Wagner B, Neumann J, et al. High Amounts of Total and Extracellular Vesicle-Derived Soluble HLA-G are Associated With HLA-G 14-Bp Deletion Variant in Women With Embryo Implantation Failure. Am J Reprod Immunol (2016) 75(6):661–71. doi: 10.1111/aji.12507
101. Giudice LC. Endometrium in PCOS: Implantation and Predisposition to Endocrine CA. Best Pract Res Clin Endocrinol Metab (2006) 20(2):235–44. doi: 10.1016/j.beem.2006.03.005
102. Giordano MV, Giordano LA, Teixeira Gomes RC, Simoes RS, Nader HB, Giordano MG, et al. The Evaluation of Endometrial Sulfate Glycosaminoglycans in Women With Polycystic Ovary Syndrome. Gynecolog Endocrinol (2015) 31(4):278–81. doi: 10.3109/09513590.2014.989980
103. Cakmak H, Taylor HS. Implantation Failure: Molecular Mechanisms and Clinical Treatment. Hum Reprod Update (2011) 17(2):242–53. doi: 10.1093/humupd/dmq037
104. Valdes CT, Schutt A, Simon C. Implantation Failure of Endometrial Origin: it is Not Pathology, But Our Failure to Synchronize the Developing Embryo With a Receptive Endometrium. Fertil Steril (2017) 108(1):15–8. doi: 10.1016/j.fertnstert.2017.05.033
105. Makrigiannakis A, Makrygiannakis F, Vrekoussis T. Approaches to Improve Endometrial Receptivity in Case of Repeated Implantation Failures. Front Cell Dev Biol (2021) 9:613277. doi: 10.3389/fcell.2021.613277
106. Li H, Huang X, Chang X, Yao J, He Q, Shen Z, et al. S100-A9 Protein in Exosomes Derived From Follicular Fluid Promotes Inflammation Via Activation of NF-kappaB Pathway in Polycystic Ovary Syndrome. J Cell Mol Med (2020) 24(1):114–25. doi: 10.1111/jcmm.14642
107. Huang X, Wu B, Chen MX, Hong L, Kong PC, Wei ZY, et al. Depletion of Exosomal circLDLR in Follicle Fluid Derepresses miR-1294 Function and Inhibits Estradiol Production Via CYP19A1 in Polycystic Ovary Syndrome. Aging-Us (2020) 12(15):15414–35. doi: 10.18632/aging.103602
108. Sun H, Li D, Yuan M, Li Q, Li N, Wang G. Eutopic Stromal Cells of Endometriosis Promote Neuroangiogenesis Via Exosome Pathway. Biol Reprod (2019) 100(3):649–59. doi: 10.1093/biolre/ioy212
109. Liu C, Li L, Wang M, Shui S, Yao H, Sui C, et al. Endometrial Extracellular Vesicles of Recurrent Implantation Failure Patients Inhibit the Proliferation, Migration, and Invasion of HTR8/SVneo Cells. J Assisted Reprod Genet (2021) 38(4):825–33. doi: 10.1007/s10815-021-02093-5.
110. Zhou W, Lian Y, Jiang J, Wang L, Ren L, Li Y, et al. Differential Expression of microRNA in Exosomes Derived From Endometrial Stromal Cells of Women With Endometriosis-Associated Infertility. Reprod BioMedicine Online (2020) 41(2):170–81.
111. Garrido N, Navarro J, García-Velasco J, Remoh J, Pellice A, Simón C. The Endometrium Versus Embryonic Quality in Endometriosis-Related Infertility. Hum Reprod Update (2002) 8(1):95–103. doi: 10.1093/humupd/8.1.95
112. Akbas GE, Taylor HS. HOXC and HOXD Gene Expression in Human Endometrium: Lack of Redundancy With HOXA Paralogs. Biol Reprod (2004) 70(1):39–45. doi: 10.1095/biolreprod.102.014969
113. Chen H, S-g S, Qu L-r, Wang X-x, Hui M. Expression of Hoxa-10 mRNA in the Endometrium of Women With Unexplained Infertile. J China Med Univ (2003) 32(3):252–3. doi: 10.1093/humrep/dep306
114. Bozdag G, Mumusoglu S, Zengin D, Karabulut E, Yildiz BO. The Prevalence and Phenotypic Features of Polycystic Ovary Syndrome: A Systematic Review and Meta-Analysis. Hum Reprod (2016) 31(12):2841–55. doi: 10.1093/humrep/dew218
115. Qiao J, Wang L, Li R, Zhang XW. Microarray Evaluation of Endometrial Receptivity in Chinese Women With Polycystic Ovary Syndrome. Reprod Biomedicine Online (2008) 17(3):425–35. doi: 10.1016/S1472-6483(10)60228-3
116. Rashid N, Nigam A, Jain SK, Naqvi SH, Wajid S. Proteomic Sift Through Serum and Endometrium Profiles Unraveled Signature Proteins Associated With Subdued Fertility and Dampened Endometrial Receptivity in Women With Polycystic Ovary Syndrome. Cell Tissue Res (2020) 380(3):593–614. doi: 10.1007/s00441-020-03171-3
117. Matsuzaki S, Canis M, Darcha C, Pouly JL, Mage G. HOXA-10 Expression in the Mid-Secretory Endometrium of Infertile Patients With Either Endometriosis, Uterine Fibromas or Unexplained Infertility. Hum Reprod (2009) 24(12):3180–7. doi: 10.1093/humrep/dep306
118. Amjadi F, Mehdizadeh M, Ashrafi M, Nasrabadi D, Taleahmad S, Mirzaei M, et al. Distinct Changes in the Proteome Profile of Endometrial Tissues in Polycystic Ovary Syndrome Compared With Healthy Fertile Women. Reprod Biomedicine Online (2018) 37(2):184–200. doi: 10.1016/j.rbmo.2018.04.043
119. Hu JH, Tang T, Zeng Z, Wu J, Tan XS, Yan J. The Expression of Small RNAs in Exosomes of Follicular Fluid Altered in Human Polycystic Ovarian Syndrome. Peerj (2020) 8:e8640. doi: 10.7717/peerj.8640
120. Wang LP, Peng XY, Lv XQ, Liu L, Li XL, He X, et al. High Throughput circRNAs Sequencing Profile of Follicle Fluid Exosomes of Polycystic Ovary Syndrome Patients. J Cell Physiol (2019) 234(9):15537–47. doi: 10.1002/jcp.28201
121. Piltonen TT, Chen JC, Khatun M, Kangasniemi M, Liakka A, Spitzer T, et al. Endometrial Stromal Fibroblasts From Women With Polycystic Ovary Syndrome Have Impaired Progesterone-Mediated Decidualization, Aberrant Cytokine Profiles and Promote Enhanced Immune Cell Migration In Vitro. Hum Reprod (2015) 30(5):1203–15. doi: 10.1093/humrep/dev055
122. Long X, Li R, Yang Y, Qiao J. Overexpression of IL-18 in the Proliferative Phase Endometrium of Patients With Polycystic Ovary Syndrome. Reprod Sci (2017) 24(2):252–7. doi: 10.1177/1933719116653681
123. Das M, Holzer HE. Recurrent Implantation Failure: Gamete and Embryo Factors. Fertil Steril (2012) 97(5):1021–7. doi: 10.1016/j.fertnstert.2012.02.029
124. Liu C, Wang M, Zhang H, Sui C. Altered Microrna Profiles of Extracellular Vesicles Secreted by Endometrial Cells From Women With Recurrent Implantation Failure. Reprod Sci (2021). doi: 10.1007/s43032-020-00440-y
125. Ranjbaran A, Latifi Z, Nejabati HR, Abroon S, Mihanfar A, Sadigh AR, et al. Exosome-Based Intercellular Communication in Female Reproductive Microenvironments. J Cell Physiol (2019) 234(11):19212–22. doi: 10.1002/jcp.28668
126. Giacomini E, Sanchez AM, Vigano P, Giardina P, Papaleo E, Scaruffi P, et al. Getting Insight of Uterine Fluid-Derived Extracellular Vesicles. J Extracellular Vesicles (2016) 5:38. doi: 10.3402/jev.v5.31552
127. Zhang Y, Wang Q, Wang H, Duan E. Uterine Fluid in Pregnancy: A Biological and Clinical Outlook. Trends Mol Med (2017) 23(7):604–14. doi: 10.1016/j.molmed.2017.05.002
Keywords: extracellular vesicles, exosomes, embryo implantation, intercellular communication, microRNA
Citation: Jiang N-X and Li X-L (2021) The Complicated Effects of Extracellular Vesicles and Their Cargos on Embryo Implantation. Front. Endocrinol. 12:681266. doi: 10.3389/fendo.2021.681266
Received: 16 March 2021; Accepted: 18 May 2021;
Published: 04 June 2021.
Edited by:
Qi Chen, The University of Auckland, New ZealandReviewed by:
Mancy Tong, Yale University, United StatesSeok Hee Lee, University of California, San Francisco, United States
Copyright © 2021 Jiang and Li. This is an open-access article distributed under the terms of the Creative Commons Attribution License (CC BY). The use, distribution or reproduction in other forums is permitted, provided the original author(s) and the copyright owner(s) are credited and that the original publication in this journal is cited, in accordance with accepted academic practice. No use, distribution or reproduction is permitted which does not comply with these terms.
*Correspondence: Xue-Lian Li, xllifc@fudan.edu.cn