- 1Department of Orthopaedics, The Second Xiangya Hospital of Central South University, Changsha, China
- 2Hunan Key Laboratory of Tumor Models and Individualized Medicine, The Second Xiangya Hospital of Central South University, Changsha, China
The clinical need for effective osteoporotic fracture therapy and prevention remains urgent. The occurrence and healing of osteoporotic fracture are closely associated with the continuous processes of bone modeling, remodeling, and regeneration. Accumulating evidence has indicated a prominent role of exosomes in mediating multiple pathophysiological processes, which are essential for information and materials exchange and exerting pleiotropic effects on neighboring or distant bone-related cells. Therefore, the exosomes are considered as important candidates both in the occurrence and healing of osteoporotic fracture by accelerating or suppressing related processes. In this review, we collectively focused on recent findings on the diagnostic and therapeutic applications of exosomes in osteoporotic fracture by regulating osteoblastogenesis, osteoclastogenesis, and angiogenesis, providing us with novel therapeutic strategies for osteoporotic fracture in clinical practice.
Introduction
Bone is an essential component of the musculoskeletal system, providing structural support for tendons and ligaments as well as protection to vital internal organs. Bone fracture is a worldwide public health problem and often leads to dire consequences, exerting a strong threat on life quality and even mortality (1). It results from injury, or a mild stress under certain pathological conditions that weakens the bones, like osteoporosis (OP), bone cancer, or osteogenesis imperfecta (2). In particular, OP is a major systemic musculoskeletal disorder characterized by deterioration of micro-architecture and bone loss, with an inclination to high risks of bone fragility and even fracture (3). As a matter of fact, osteoporotic fracture accounts for a large proportion of all fractures. Osteoporotic fracture-related impaired healing, especially non-union, is another critical problem, resulting in prolonged treatment and aggravated socio-economic burden.
Exosomes were first identified in 1981 as exfoliated membranes by Trams (4). At present, exosomes are defined as cell-derived spherical lipid bilayer vesicles (EVs) with a diameter around 40-160nm (5). Exosomes can be secreted by nearly all sorts of cells and are found in various biological fluids, such as plasma, serum, and cerebral spinal fluid (6). Previous studies have demonstrated that the constituents of exosomes are macromolecules including DNAs, RNAs (mRNA, microRNA, and other non-coding RNA), proteins, lipids, and cytokines (7, 8). Size and content are two major manifestations of the heterogeneity for exosomes, which vary with cellular origin, metabolic status, and environment of cells (5). Size heterogeneity is beneficial to distinguish exosomes and other EV subtypes. This property is also highly relevant to exosome isolation. Nowadays, some researchers usually use differential centrifugation to purify exosomes, but these exosome pellet fractions contain more than just exosomes. Hence, we require modified novel techniques for exosome purification, which is directly associated with the results of many exosomes-related experiments. Content heterogeneity determines the functions of exosomes since exosomes can convey their contains into recipient cells via endocytosis, receptor- ligand interaction, or fusion of membranes (9), thus mediating lots of responses of these recipient cells. Moreover, numerous studies have demonstrated that exosomes were obviously related to immune responses (10), pregnancy (11), and the occurrence and development of many disease, such as cardiovascular disease (12), central nervous system-associated diseases (13), and cancer (9). For example, exosomes secreted by apoptotic vascular endothelial cells were rich in miR-126, which could suppress the apoptosis of endothelial cells by activating chemokine ligand 12 (CCL12). Moreover, miR-126 containing exosomes inhibited the penetration of macrophages into the blood vessel wall, thereby stabilizing the hardened plaque and exerting the anti- atherosclerosis effect (14). Exosomes derived from prostate cancer cells could induce neoplastic reprogramming and tumor formation of adipose stem cells via transferring their cargos, like miR-125b, miR-130, miR-155, HRas, and Kras mRNAs (15). Apoptotic glioblastoma cells could secret spliceosomal proteins such as RBM11 and small nuclear RNAs (snRNAs) containing exosomes to modify mRNA splicing (MDM4, CCND1) of recipient cells, resulting in tumor aggressiveness and drug resistance (16). Significantly, translation of more comprehensive understanding of exosomes in various diseases into diagnosis and therapeutic applications has already occurred. First, the intrinsic properties of exosomes have exhibited their great potential in diagnosis of multiple diseases. Lewis et al. presented a simple method which integrated and analyzed exosomes as well as other extracellular vesicles directly from whole blood, plasma, or serum onto an AC electrokinetic microarray chip. They detected the samples from pancreatic ductal adenocarcinoma (PDAC) patients as well as healthy objects, finding that glypican-1 and CD63 were useful biomarkers to predict the occurrence of PDAC. These researchers also developed a bivariate model to detect PDAC with 99% sensitivity and 82% specificity (17). In addition, exosomes have been implicated as therapeutic targets in many fields, with potential utility in delivering therapeutic payloads directly to the desired place (18). Exosomal microRNA (miRNA) could target mRNA and modify related gene expression in the recipient cells, and some laboratories have attempted to use exosomes for the delivery of miRNA or small interfering RNA (siRNA) to treat mammary carcinoma (19), glioma (20), and pancreatic cancer (21). Moreover, clinical-grade MSC-derived exosomes with KrasG12D siRNA payload (iExosomes) have been applied to the treatment of pancreatic cancer in various animal models (22). Taken together, exosomes play an important role in mediating a variety of physiological and pathological processes through the special intracellular communication, which provides us a promising avenue to conquer numerous disorders, including osteoporotic fractures (23).
In this review, we aimed to illustrate the relationship between the exosomes and osteoporotic fracture, while referring to the complicated occurrence and healing processes. Moreover, we summarized the applications of exosomes in preventing and treating osteoporotic fracture, which may be an invaluable tool for the intervention of osteoporotic fracture and other related musculoskeletal disorders.
Bone Physiology and Exosomes
Bone consists of minerals and organic material. The minerals include crystals of hydroxyapatite Ca10(PO4)6(OH)2 and other ions, while the organic material comprises osteogenic cells and extracellular matrixes like collagen fibers (24). Osteogenic cells mainly include three types of cells: the osteoblasts, osteoclasts, and osteocytes (25). In fact, exosomes exerted a dominant effect on bone turnover and remodeling by mediating these important elements of bone. On the one hand, exosomes played a key role in the process of production of bone extracellular matrixes. For instance, the exosomes membrane rich in phosphatidylserine (PS) participated in the formation of hydroxyapatite crystal during osteogenesis, a process that was also accelerated by the calcium stored in exosomal annexins and the phosphate generated by exosomal ATPases, nucleotidases, phosphatases, pyrophosphatases, and membrane transporters (24). On the other hand, exosomes acted as messengers to mediate signals transmitting in the same cluster (autocrine) or different cluster (paracrine) of osteogenic cells, thus participating in their differentiations and activations. Wei et al. found let-7 was contained in both osteoblast precursors and differentiated osteoblasts-derived exosomes, which could promote osteogenesis through mediating high-mobility group AT-hook 2 (HMGA2) and Axin 2 (26). Another study by Li et al. showed osteoclasts-derived exosomal miR-214-3p suppressed osteoblastic bone formation (27). Further, bone modeling and remodeling were tightly correlated with the endocrine system. And parathyroid hormone (PTH), estrogen hormone, and glucocorticoid were important factors in mediating the bone microenvironment for osteoanabolism (28). However, the specific relationships between exosomes and steroid or protein hormones in bone physiology is still not fully understood and needs further exploration. Overall, exosomes exert an indispensable role in these processes and future research analyzing their properties and functions may help to build a multi-targeted system to maintain the balance of bone resorption and formation.
Relationships Between Exosomes and the Occurrence of Osteoporotic Fracture
Bone loss and a fall bias lead to great susceptibility to fractures for the aging and menopausal population (29). Clinically, osteoporotic fractures are common, have become a serious public health issue worldwide, and cause an ever-increasing burden to the healthcare system. OP has an obvious clinical and public health impact which is estimated to affect 75 million people worldwide (30). Moreover, the number of annual incident fragility fractures is about 9 million (31).
Mechanically, OP is a result of excessive bone resorption and inadequate formation of new bone upon aberrant activities of osteoclasts and osteoblasts (32). Under normal circumstances, bone metabolism follows the strict control of various factors. And among them, receptor activator of NF-κB (RANK)/receptor activator of NF-κB ligand (RANKL), Wnt/β-catenin, and Jagged1/Notch1 are the three best studied pathways which exert a strong influence on bone mass density (33). Strikingly, exosomes have been widely studied for their roles in OP, which make great contributions to the imbalance of osteoblasts and osteoclasts, hence promoting the prevalence of fragility fracture. Therefore, we comprehensively reviewed the role of exosomes in OP initiating bone fracture and presented their potential in retarding the occurrence and progress of osteoporotic fracture.
The Functions of Exosomes in OP
As mentioned above, exosomes functioned as “the carrier pigeons of the cell” in intercellular communication and materials exchange to mediate a series of responses of adjacent or distant recipient cells (34, 35). Multiple studies have shown that bone-related exosomes were tightly associated with bone modeling and remodeling (27, 36) by transferring biologically essential molecules to interfere with the activities of osteoblasts and osteoclasts (Figure 1). Besides, the circulatory exosomes possessed pathophysiological functions in the development of senile OP and thus would be helpful for its diagnosis and therapy.
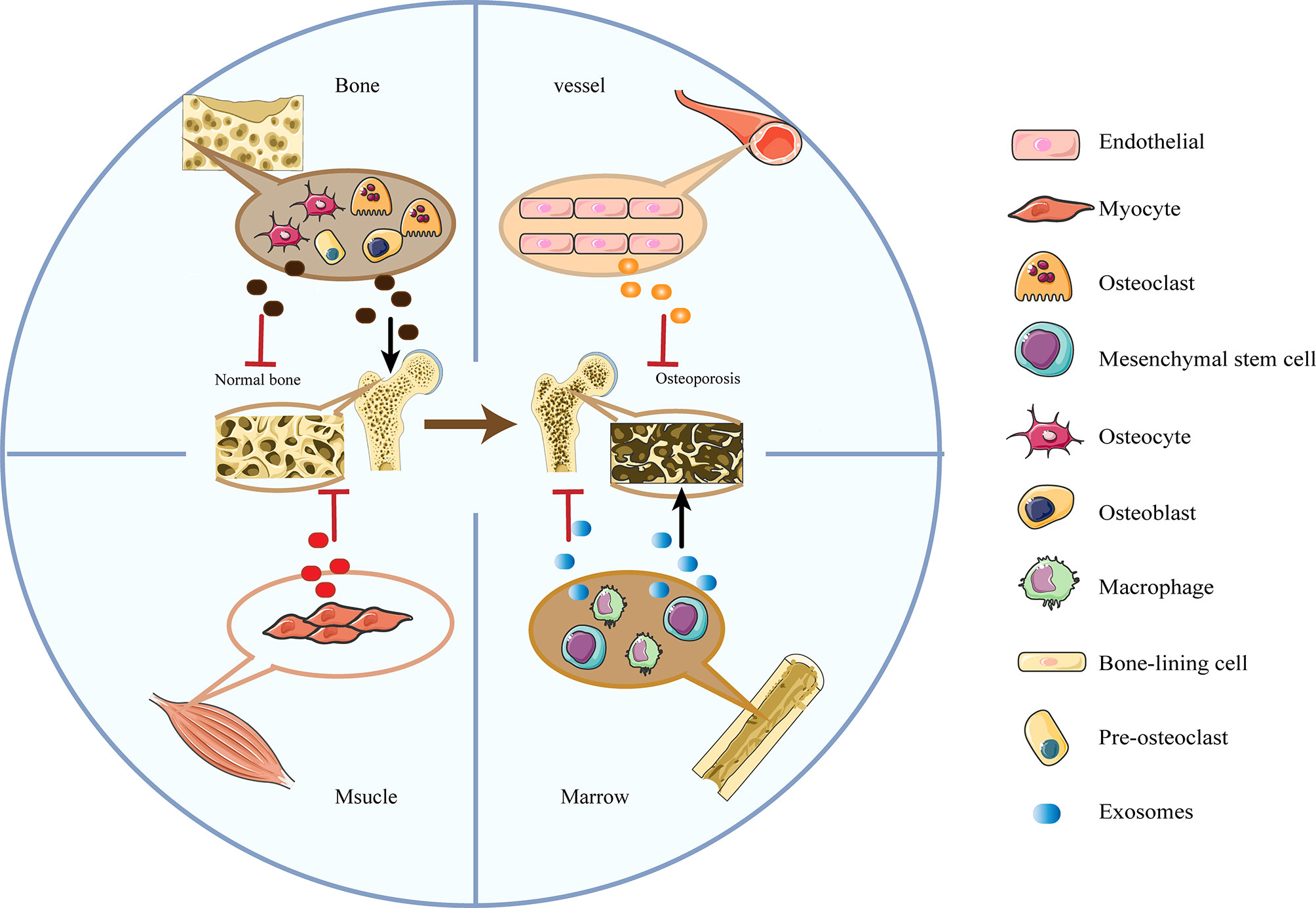
Figure 1 The diplex functions of bone-related cells-derived exosomes in OP. Exosomes secreted by osteoblasts, osteoblasts and mesenchymal stem cells have bilateral effects in promoting and suppressing OP; Exosomes derived from myocytes and vascular endothelial cells mainly inhibit the process of OP.
Circulatory Exosomes in OP
A large number of reports detected the main differences of serum-derived exosomes (SDEs) between OP or osteopenia and normal bone mass by related techniques to get a more comprehensive understanding of the functions of exosomes in OP (Table 1). Xie et al. identified 1371 proteins from SDEs, especially, 585 differentially expressed proteins (DEPs) of OP. These DEPs not only participated in inhibiting integrin-related activation and function of osteoblasts, but also strengthened the capacity of osteoclasts. Notably, the integrin β1 (ITGβ1), integrin β3 (ITGβ3), and hematopoietic progenitor cell antigen CD34 (CD34) were three representatives of downregulated proteins, which acted as hubs in suppressing bone mineralization of osteoblasts (37). Chen et al. utilized the PLAGH Hip Fracture Database, finding a total of 45 significant DEPs and verifying four exosomal proteins, namely PSMB9, PCBP2, VSIR, and AARS, with an AUC of 0.805 in the classification of OP (38). Zhang et al. investigated the functions of transfer RNA‐derived fragments (tRFs), a novel type of small non‐coding RNAs derived from tRNAs contained in plasma exosomes in OP. They found 29 differentially expressed tRFs, which played an active role in some important pathways related to OP, including Wnt, PI3K‐Akt, MAPK, TGF‐β, and calcium signaling pathway. More importantly, plasma exosomal tRF‐25‐R9ODMJ6B26 (tRF‐25), tRF‐38‐QB1MK8YUBS68BFD2 (tRF‐38), and tRF‐18‐BS68BFD2 (tRF‐18) were at highly-expressed levels in OP patients compared to normal controls and were utilized to develop a more accurate model for OP diagnosis with an average AUC of 0.815 (39). Furthermore, a study by Teng et al. detected 393 differentially expressed lncRNAs and found co-located mRNAs were highly enriched in OP-associated processes, such as MAPK pathway, insulin secretion, cellular response to metal ions, fucosylation, and proteolysis (40). More importantly, Shao et al. isolated serum exosomes from menopausal females with or without OP and detected 191 aberrant miRNAs, which were related to Wnt, MAPK, and Hippo pathways (41). All of these findings offered forceful evidence to illustrate exosomes from the circulation could be potential biomarkers of evaluating bone status for OP diagnosis and analyzing the results of therapy.
Bone-Related Cell-Derived Exosomes in OP
Bone-related cells like osteoblasts, osteoclasts, and endothelial cells, could achieve mutual interaction through exosomes, the cell-cell communicators (Table 2). Over the past few years, many researchers have drawn attention to the relationship between bone-related cells-derived exosomes and OP. First, some laboratories have extensively investigated exosomes derived from bone-related cells by using techniques including Nanoparticle Tracking Analysis (NTA) (69), Transmission electron microscopy (TEM) (50), western blotting (WB), immuno-EM, or bead-based fluorescence-activated cell sorting (FACS) (63). More significantly, these scientists not only identified the classical bone-related cell-derived exosomal markers of OP, but also revealed the ability of exosomes to mediate osteogenic cells differentiation and activity as well as matrix formation in OP. Overall, this evidence indicated the essential role of bone-related cell-derived exosomes in OP and provided us serviceable tools in the treatment of OP.
MSCs-Derived Exosomes in OP
MSCs are pluripotent stem cells with the capability to proliferate extensively and maintain the potential to differentiate into various types of cells (70). In 2010, MSCs-derived exosome was first isolated from conditioned medium of human embryonic-derived MSCs (hESC-MSCs) (71). Nowadays, MSCs have been identified as the most ferocious producer of exosomes (72). Moreover, Chen et al. detected more than 850 gene products and 150 miRNAs in MSCs-derived exosomes (73, 74), indicating their potential clinical efficacy for a variety of diseases, including OP. In fact, a considerable amount of research has confirmed that BM-MSCs-derived exosomes could improve OP by triggering osteoblasts proliferation, differentiation, and activation as well as inhibiting cell apoptosis (42, 43) through miR-196a (44), miR-150-3p (45), miR‐181a (46), miR‐218 (47), lncRNA MALAT1 (48), and let‐7 (26). In addition, miR-29a loaded in BM-MSCs-derived exosomes could not only promote angiogenesis and osteogenesis but also restrain osteoclastogenesis, which was down-regulated in the aged populations, providing us a novel way to improve therapeutic strategy for OP (49). BM-MSCs-derived exosomes also have been identified as inducers to motivate undifferentiated MSCs toward osteogenic differentiation both in vivo and in vitro (50–52), showing its potential as a bone regenerative drug for OP. Moreover, Zuo et al. expounded that BM-MSCs-derived exosomes could promote bone formation by banishing reactive oxygen species (ROS), assisting in DNA repair, rescuing cell (mainly osteoblasts) proliferation and differentiation ability, suppressing the senescence-related protein and adipogenic gene expression, and accelerating osteogenic expression, thus ameliorating OP (53).
However, some studies also showed that BM-MSCs-derived exosomes could be helpful to OP with the function of promoting maturation and activity of osteoclasts. Previous studies have proven that miR‐31a‐5p was over-expressed in aged BM-MSCs- derived exosomes, which could reduce osteoblastogenesis by the SATB2 and E2F2 pathways and promote osteoclastogenesis by the RhoA pathway (54). Hence miR‐31a‐5p contained in BM-MSCs exosomes would lead to osteoporotic bone loss in aging bone tissue. Cheng et al. also detected that miR‐148a from BM-MSCs-derived exosomes could stimulate osteoclasts differentiation via targeting V‐maf musculoaponeurotic fibrosarcoma oncogene homolog B (55). Besides, a recent study by Jiang and colleagues demonstrated miR-21 was at a high level in BM-MSCs derived exosomes from OP patients, which inhibited osteogenesis by targeting SMAD7 (56).
Therefore, exosomes secreted by BM-MSCs are capable of mediating proliferation and differentiation of osteoblasts and osteoclasts, suggesting a novel method to improve therapeutic strategy for OP. For instance, researchers have successfully used exosomes from processed BM-MSCs, like miR-935-modified BM-MSCs and circ-Rtn4-modified BM-MSCs, to treat OP (75, 76).
Osteoblasts-Derived Exosomes in OP
Paracrine/autocrine in communication between osteoblasts and other bone-related cells in the field of OP has provided us with new knowledge and evoked further detection in this area. Ge et al. isolated osteoblasts-derived exosomes and analyzed their cargos, finding they were tightly combined with osteogenesis (57). Consistently, accumulating studies indicated that the contents of osteoblasts-derived exosomes, like miR-677-3p (59) or miR-378 (58), could accelerate osteogenesis, while RANKL (61), miR-30d-5p, miR-133-3p (59), and miR-140-5p (60) from osteoblast-derived exosomes possessed the ability to suppress this progress. Niedermair et al. recently revealed osteoblasts-derived exosomes from OP patients could also hamper osteogenic differentiation of BM-MSCs (77). Therefore, it remains a mystery for us as to what the specific functions of osteoblasts-derived exosomes in OP are.
Osteoclasts-Derived Exosomes in OP
Osteoclasts-derived exosomes have positive as well as negative feedback in the progress of osteogenesis, which played dual roles in OP. A recent study showed that exosomes secreted by osteoclast precursors facilitated vitamin D-dependent osteoclast formation in whole mouse marrow cultures, while exosomes from osteoclast-enriched cultures suppressed osteoclastogenesis in the same cultures which referred to an important factor-RANK (62). Furthermore, miR-214-containing exosomes from osteoclasts have been reported to have a significant effect on osteogenesis. On one hand, it could restrain osteoblasts via targeting EphrinA2/EphA2 (63) and activating transcription factor 4 (ATF4) (27). On the other hand, it could promote osteoclastogenesis through the PI3K/Akt pathway (64). Therefore, miR-214-3p may be a potential clinical target to reverse established OP. In fact, another study performed by Zhu et al. also found that the magnetic hydroxyapatite scaffold (MHA) facilitated osteoblasts’ proliferation in a model of OP through altering the osteoclasts-derived exosomal cargos and suppressing exosomes intussuscept by osteoblasts (78), indicating exosomes could act as tools to modify the interactions of bone cells or direct drugs to treat OP.
Osteocytes-Derived Exosomes in OP
Osteocytes are vital components of bone tissue that originated from osteoblasts and play multifaceted roles in bone remodeling. Emerging studies found osteocytes-derived exosomes could enter into circulation and carry some miRNAs, such as miR-29, miR-484, and miR-221 (79). Besides, Qin et al. offered evidence that exosomes from osteocytes could co-localize with the nucleus of MC3T3-E1 cells and reduce osteoblastogenesis. More significantly, they expounded the specific mechanism that myostatin possessed the ability to suppress osteocytes-derived exosomal miR-218, which was an unprecedented method for muscle-bone communication (65).
Muscle-Derived Exosomes in OP
Muscle has a mechanical crosstalk with bone. As aforementioned, muscle is identified as a secretory endocrine organ that takes part in biochemical interplay and influences the functions mutually. Bone-derived factors compromise fibroblast growth factor (FGF)-2, prostaglandin E2, osteocalcin, and sclerostin, while the muscle secreted factors are described as “myokines” (80), such as myostatin, interleukin (IL)-6, irisin, and RANKL. Recently, Fulzele et al. found that miR-34a-5p was overexpressed in muscle and muscle-secreted exosomes with aging and with myoblast exposure to oxidation (66). Further research showed that muscle-derived exosomes containing miR-34a could suppress Sirt1 expression in BM-MSCs and accelerate BM-MSCs senescence (81). The evidence represented a potential pathway by which muscle could affect bone physiology and provide us a different perspective to understand OP.
Endothelial Cells-Derived Exosomes in OP
Blood vessels occupy vital positions in bone homeostasis. Endothelial cells are stationed at the inner layer of vascular vessels, which actively participate in the progress of internalizing and secreting substances like exosomes. Song et al. found that miR-155 loaded in endothelial cells-derived exosomes suppressed osteoclasts’ differentiation and activation by several targets, like Spi1, microphthalmia-associated transcription factor (Mitf), and suppressor of cytokine signaling 1 (Socs1) (67). Furthermore, endothelial cells-derived exosomes could rescue the glucocorticoid-induced osteogenic suppression of osteoblasts by inhibiting ferritinophagy-dependent ferroptosis (68). Hence, endothelial cells-derived exosomes might be promising and biocompatible nanomedicine for OP.
Macrophages-Derived Exosomes in OP
Osteal macrophages are a subtype of bone-resident macrophages, which are close to the bone surface and adjacent to osteoblasts, with the function of mediating bone formation (82). Current knowledge about macrophage-derived exosomes is limited. A study by Wei et al. showed that BMP2/macrophage-derived exosomes could up-regulate the expression of osteogenesis-associated genes such as ALP, Runx2, BMP-2, BMP-7, and osteopontin, playing essential roles in OP (83). Collectively, the explicit mechanism of the macrophage-derived exosomes in OP remains unclear. However, with more in-depth study, it may show great potential acting as the therapeutic target for OP in the future.
Relationships Between Exosomes and Osteoporotic Fracture Healing
Bone is a unique form of tissue that can heal without a fibrous scar (84). Similar to the process of bone remodeling, bone regeneration is highly orchestrated and precisely controlled, and is influenced by multiple extrinsic and intrinsic factors (85). Extrinsic factors include smoking, alcohol, and certain use of medicines While intrinsic factors cover injury to the periosteum or endosteum and poor vascularization at the injury site (86). Although we have achieved great improvement in surgical techniques nowadays, sometimes osteoporotic fractures-related impaired bone regeneration like non-union would happen, which greatly affects the life quality of a number of patients (87). Therefore, it is of great need to do intensive investigations to fill the clinical gaps and develop effective therapeutic approaches for these affected patients.
In general, fracture healing is subdivided into four biological phases followed in a chronological order: hematoma formation and inflammatory response, proliferation and differentiation, ossification, and remodeling (88). These phases are involved in many physiological processes, like inflammation, angiogenesis, stem cell differentiation, osteogenesis, and chondrogenesis with the involvement of various types of bone-related cells (89). Exosomes are closely connected with these processes through mediating cell-to-cell communications (Table 3). In fact, exosomes could promote osteoblasts’ differentiation, suppress osteoclasts formation and differentiation, and accelerate angiogenesis. In addition, some important intrinsic factors mentioned above could modify the functions of exosomes to regulate the healing process. BM-MSCs could be induced to recruit, proliferate, migrate, and differentiate into osteoblasts and chondrocytes during intramembranous ossification and endochondral ossification, which played important roles in osteoporotic fracture healing (99). Reliable evidence has illustrated the capacities of osteogenic differentiation for BM-MSCs-derived exosomes. For example, Furuta et al. used the CD9–/– mouse, an established model with reduced levels of exosomes, and found there was an obvious delay of endochondral ossification and fracture healing compared with the wild-type mouse. More interestingly, the delay could be rescued by injecting BM-MSCs-exosomes (90). Besides, Narayanan et al. incubated exosomes isolated from osteogenic differentiated BM-MSCs with BM-MSCs, finding BM-MSCs internalized these exosomes and gave rise to an extensive upregulation of several important genes like bone morphogenetic protein 9 (BMP9), transforming growth factor β1 (TGFβ1), transcription factors, and ECM molecules (50). Xu et al. showed similar results and detected this impact was involved in the modulatory effect of miRNAs on target genes and pathways (100). Among these, miR-196a acted as one of the most significant molecules in the modulatory process and miR-21 and miR-25 have also proven to possess the ability to accelerate osteogenesis and angiogenesis (90, 91). More importantly, BM-MSCs-exosomes contained a variety of bone repair-related cytokines such as monocyte chemotactic protein 1 (MCP-1), monocyte chemotactic protein 3 (MCP-3), stromal cell-derived factor-1 (SDF-1), and angiogenic factors, which could promote fracture healing (101). Recently, some researchers transplanted BM-MSCs-derived exosomes into the fracture site in a rat model of femoral non-union, obtaining the results that these exosomes could significantly trigger osteogenesis and angiogenesis to promote bone healing process though activating the BMP-2/Smad1/RUNX2 and the HIF-1α/VEGF signaling pathways (92). Furthermore, some studies also revealed that exosomes secreted by human umbilical cord mesenchymal stem cells (uMSCs) could enhance angiogenesis to accelerate bone healing by overexpressing VEGF, HIF‐1α (93), or Wnt signaling pathway (94), which provided a new view for us regarding uMSCs-related bone fracture. As we mentioned above, some intrinsic factors could change the functions of exosomes and thus affect the osteoporotic fracture healing. Xu et al. observed that aged exosomes had obvious attenuated effects on MSCs osteogenic differentiation in vitro and facture healing in vivo. Under further investigation, they found miR-128-3p was dramatically over-expressed in aged-BM-MSCs-derived exosomes, which functioned as a suppressor in the process of fracture healing by directly targeting the 3’-UTR of Smad5 (95). Hence, exosomal miR-128-3p antagomir could be an ideal treatment for bone fracture healing, especially for the elderly. Wang et al. observed that the healing time was longer in obese fracture patients than normal weight patients. Interestingly, using bioinformatics analysis and related assays, they further found that high-fat treatment could decrease the secretion of BM-MSCs-derived exosomes and reduce the carried lncRNA H19 via miR-467/HoxA10 axis, hence affecting osteogenic differentiation and fracture (96). Liu et al. performed a serious of in vivo and in vitro experiments to verify that hypoxia preconditioning BM-MSCs-derived exosomes exerted a greater effect on promoting fracture healing. Hypoxic MSC-derived exosomes were enriched with miR-126, which possessed the abilities of being pro-angiogenesis, pro-proliferative, and pro-migratory by suppressing SPRED1/Ras/Erk signaling pathway. Therefore, hypoxia-induced BM-MSCs transplantation might have great potential as a therapy for fracture, but several challenges remain to be overcome to achieve clinical applications (97). Moreover, a study by Xiong et al. explored the function of M2 macrophages in bone formation and its underlying mechanisms. They co-cultured M2 macrophages and BM-MSCs and isolated the exosomes secreted by M2 macrophages, finding M2 macrophages-derived exosomes (M2D-Exos) were rich in miR-5106, which could be internalized by BM-MSCs and promote osteoblasts’ differentiation to accelerate healing via targeting the osteogenic related genes, like Salt-inducible kinase 2 and 3 (SIK2 and SIK3). Therefore, local injection of M2D-Exos might be a significant therapeutic strategy to accelerate bone fracture healing (98).
Current studies provided powerful evidence that exosomes appeared to show the ability to enhance osteogenesis and angiogenesis to promote fracture healing via multiple pathways, implying a novel insight to comprehend the process of bone regeneration. Therefore, we need to spare no effort to do related research and detect an effective treatment for osteoporotic fracture.
Summary and Perspectives
The number of people with OP is increasing rapidly among generations, coupled with a growing number of osteoporotic fractures (102). Moreover, osteoporotic fractures increase the incidence of abnormal fracture healing, like delayed healing or non-union, which aggravates the heavy burden placed on healthcare systems as well as the economy. Hence, we should endeavor to look for more effective ways to prevent and treat osteoporotic fractures. Nowadays, the application of exosomes in osteoporotic fractures has received much attention, and lots of scientists have revealed multiple functions of exosomes in this field. As outlined above, exosomes played an essential role in mediating osteoporotic fractures. First, many researchers found obvious dysregulations of certain contents in circulatory exosomes from patients with OP or related impaired fracture healing, which could be promising biomarkers for the diagnosis of OP. Second, exosomes were a double-edged sword for OP by transporting their cargos to modify the activities of surrounding or distant bone-related cells. Third, exosomes have been applicated as a brilliant drug delivery system to treat osteoporotic fractures by accelerating osteogenesis and angiogenesis (Figure 2). However, the specific mechanisms of intercellular communications via exosomes is still not fully understood. A recent study by Ge et al. detected 1536 proteins contained in osteoblasts-derived exosomes and 172 among them overlapped with proteins in the bone database, such as ephrinB1 (EFNB1), transforming growth factor beta receptor 3 (TGFBR3), lipoprotein receptor-related protein (LRP6), bone morphogenetic protein receptor type-1 (BMPR1), and Smad ubiquitylation regulatory factor-1 (SMURF1) (103). Besides, increasing data unveiled there were nine overexpressed miRNAs (let-7a, miR-199b, miR-218, miR-148a, miR-135b, miR-203, miR-219, miR-299-5p, and miR-302b) and four down-regulated (miR-221, miR-155, miR-885-5p, miR-181a, and miR-320c) in MSCs-derived exosomes (100). However, these complicated mechanisms and the therapeutic potential for osteoporotic fractures remain a mystery and there is a clear need for us to perform further investigations. On the other hand, Xie et al. found that BM-MSCs-derived exosomes could promote bone formation when mixed with decalcified bone matrix scaffolds (104). But it is still a serious challenge to quantify and separate different exosome subpopulations as well as modify these exosomes for us. Overall, it should be noted that several significant developments are expected to occur, including: (a) clarifying the specific mechanisms of cargos within bone-related cells-derived exosomes in OP and osteoporotic fracture; (b) elucidating the functions of exosomes in the occurrence and healing of osteoporotic fractures, which possess both positive and negative effects; (c) discovering credible biomarkers contained in circulatory exosomes of clinical significance in the early diagnosis of OP; (d) improving the methodologies of extraction and separation of exosomes; and (e) propelling clinical application in the treatment of osteoporotic fracture through utilizing them as therapeutic agents or drug carriers. Advances in these areas will likely require new experimental techniques, superior creativity, and much work. But at the same time, such advances will help us to get a more comprehensive understanding of osteoporotic fractures and allow scientists to translate this knowledge into exosome-based therapies and diagnosis in clinic. Therefore, exosomes provide a promising method to improve the therapeutic effects of osteoporotic fractures, even though an increase in research is demanded.
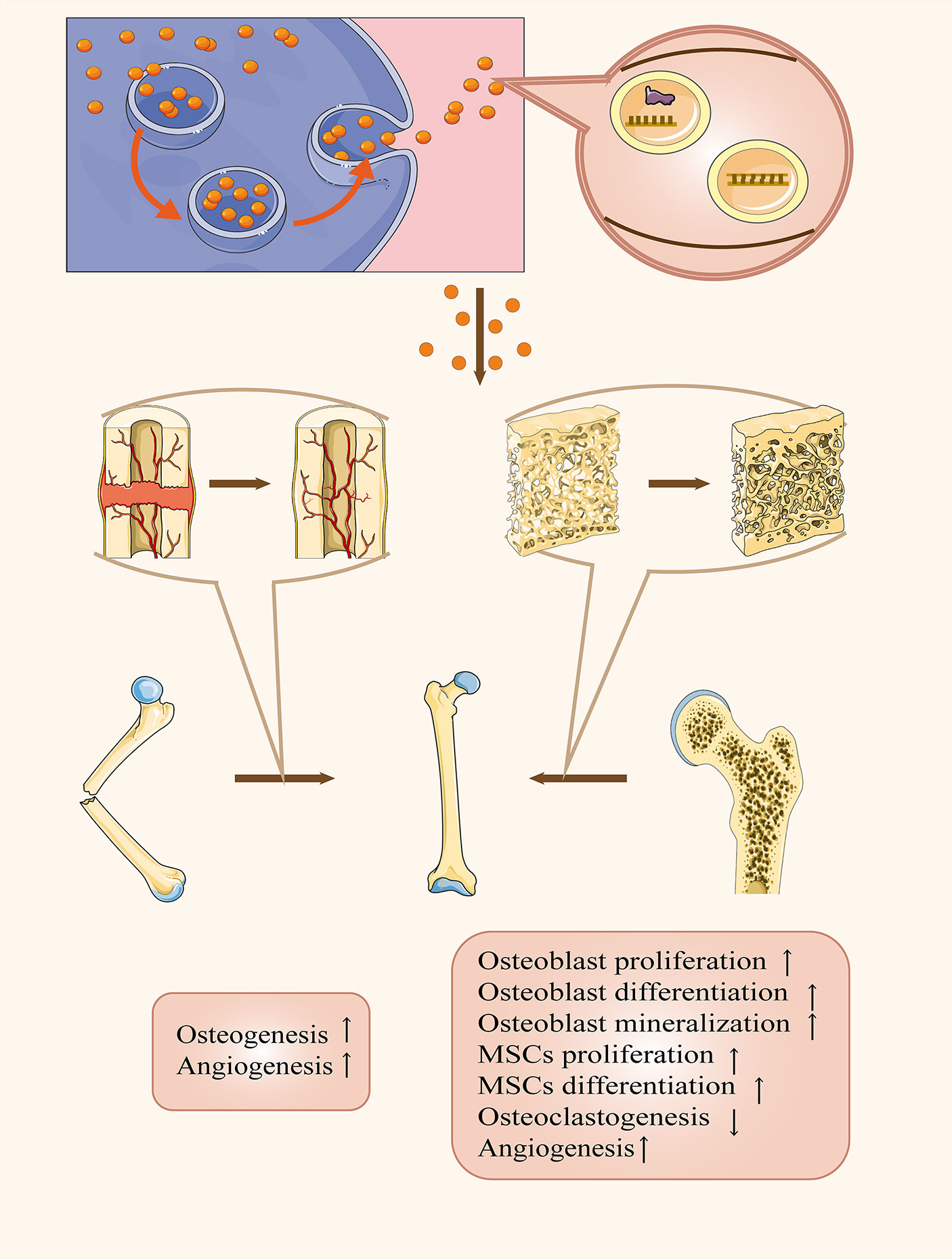
Figure 2 The therapeutic applications of exosomes in OP and related fracture healing. Exosomes can act as therapeutic agents or drug carriers not only to remit OP by facilitating osteoblast proliferation, differentiation, mineralization, and MSCs proliferation, differentiation and angiogenesis as well as suppressing osteoclastogenesis but also accelerating fracture healing via promoting osteogenesis and angiogenesis.
Author Contributions
ZY: Writing - Original Draft and Editing. WZ: Writing - Review and Editing, Visualization. XR: Writing - Original Draft. CT: Writing - Review and Editing, Supervision, Funding acquisition. ZL: Writing - Review and Editing, Supervision, Funding acquisition. All authors contributed to the article and approved the submitted version.
Funding
This work was supported by the National Natural Science Foundation of China (No. 81902745), Hunan Provincial Research and Development Program in Key Areas (2019WK2071, 2020DK2003), and China Postdoctoral Science Foundation (No. 2021M693557).
Conflict of Interest
The authors declare that the research was conducted in the absence of any commercial or financial relationships that could be construed as a potential conflict of interest.
The handling editor declared a shared affiliation with the authors at time of review.
Acknowledgments
Figures of this review were created with the aid of Servier Medical Art (https://smart.servier.com/), reproduced under Creative Commons License attribution 3.0 Unported License.
References
1. Sattui SE, Saag KG. Fracture Mortality: Associations With Epidemiology and Osteoporosis Treatment. Nat Rev Endocrinol (2014) 10(10):592–602. doi: 10.1038/nrendo.2014.125
2. Cummings SR, Eastell R. Risk and Prevention of Fracture in Patients With Major Medical Illnesses: A Mini-Review. J Bone Miner Res (2016) 31(12):2069–72. doi: 10.1002/jbmr.3030
3. Xu F, Yuan LQ. Osteoporosis Overtreatment-A Clinical Problem That Needs to Be Discussed. JAMA Intern Med (2016) 176(7):1033–4. doi: 10.1001/jamainternmed.2016.2731
4. Batty BS, Bionaz M. Graduate Student Literature Review: The Milk Behind the Mustache: A Review of Milk and Bone Biology. J Dairy Sci (2019) 102(8):7608–17. doi: 10.3168/jds.2019-16421
5. Kalluri R, LeBleu VS. The Biology, Function, and Biomedical Applications of Exosomes. Science (2020) 367(6478):eaau6977. doi: 10.1126/science.aau6977
6. Tu C, He J, Chen R, Li Z. The Emerging Role of Exosomal non-Coding RNAs in Musculoskeletal Diseases. Curr Pharm Des (2019) 25(42):4523–35. doi: 10.2174/1381612825666191113104946
7. Jeppesen DK, Fenix AM, Franklin JL, Higginbotham JN, Zhang Q, Zimmerman LJ, et al. Reassessment of Exosome Composition. Cell (2019) 177(2):428–45.e18. doi: 10.1016/j.cell.2019.02.029
8. Tu C, He J, Qi L, Ren X, Zhang C, Duan Z, et al. Emerging Landscape of Circular RNAs as Biomarkers and Pivotal Regulators in Osteosarcoma. J Cell Physiol (2020) 235(12):9037–58. doi: 10.1002/jcp.29754
9. Zhang L, Yu D. Exosomes in Cancer Development, Metastasis, and Immunity. Biochim Biophys Acta Rev Cancer (2019) 1871(2):455–68. doi: 10.1016/j.bbcan.2019.04.004
10. Anel A, Gallego-Lleyda A, de Miguel D, Naval J, Martínez-Lostao L. Role of Exosomes in the Regulation of T-Cell Mediated Immune Responses and in Autoimmune Disease. Cells (2019) 8(2):154. doi: 10.3390/cells8020154
11. Czernek L, Düchler M. Exosomes as Messengers Between Mother and Fetus in Pregnancy. Int J Mol Sci (2020) 21(12):4264. doi: 10.3390/ijms21124264
12. Guo B, Zhuang T, Xu F, Lin X, Li F, Shan SK, et al. New Insights Into Implications of CTRP3 in Obesity, Metabolic Dysfunction, and Cardiovascular Diseases: Potential of Therapeutic Interventions. Front Physiol (2020) 11:570270. doi: 10.3389/fphys.2020.570270
13. Grativvol RS, Cavalcante WCP, Castro LHM, Nitrini R, Simabukuro MM. Updates in the Diagnosis and Treatment of Paraneoplastic Neurologic Syndromes. Curr Oncol Rep (2018) 20(11):92. doi: 10.1007/s11912-018-0721-y
14. Zernecke A, Bidzhekov K, Noels H, Shagdarsuren E, Gan L, Denecke B, et al. Delivery of microRNA-126 by Apoptotic Bodies Induces CXCL12-dependent Vascular Protection. Sci Signal (2009) 2(100):ra81. doi: 10.1126/scisignal.2000610
15. Abd Elmageed ZY, Yang Y, Thomas R, Ranjan M, Mondal D, Moroz K, et al. Neoplastic Reprogramming of Patient-Derived Adipose Stem Cells by Prostate Cancer Cell-Associated Exosomes. Stem Cells (2014) 32(4):983–97. doi: 10.1002/stem.1619
16. Pavlyukov MS, Yu H, Bastola S, Minata M, Shender VO, Lee Y, et al. Apoptotic Cell-Derived Extracellular Vesicles Promote Malignancy of Glioblastoma Via Intercellular Transfer of Splicing Factors. Cancer Cell (2018) 34(1):119–35. doi: 10.1016/j.ccell.2018.05.012
17. Lewis JM, Vyas AD, Qiu Y, Messer KS, White R, Heller MJ. Integrated Analysis of Exosomal Protein Biomarkers on Alternating Current Electrokinetic Chips Enables Rapid Detection of Pancreatic Cancer in Patient Blood. ACS Nano (2018) 12(4):3311–20. doi: 10.1021/acsnano.7b08199
18. Yuan LQ. Novel Strategies for Gene Therapy-Recent Advances in the Use of Exosomes for Disease Treatment. Curr Pharm Des (2019) 25(42):4463. doi: 10.2174/138161282542191230114518
19. Ohno S, Takanashi M, Sudo K, Ueda S, Ishikawa A, Matsuyama N, et al. Systemically Injected Exosomes Targeted to EGFR Deliver Antitumor microRNA to Breast Cancer Cells. Mol Ther (2013) 21(1):185–91. doi: 10.1038/mt.2012.180
20. Katakowski M, Buller B, Zheng X, Lu Y, Rogers T, Osobamiro O, et al. Exosomes From Marrow Stromal Cells Expressing miR-146b Inhibit Glioma Growth. Cancer Lett (2013) 335(1):201–4. doi: 10.1016/j.canlet.2013.02.019
21. Mendt M, Kamerkar S, Sugimoto H, McAndrews KM, Wu CC, Gagea M, et al. Generation and Testing of Clinical-Grade Exosomes for Pancreatic Cancer. JCI Insight (2018) 3(8):e99263. doi: 10.1172/jci.insight.99263
22. Kamerkar S, LeBleu VS, Sugimoto H, Yang S, Ruivo CF, Melo SA, et al. Exosomes Facilitate Therapeutic Targeting of Oncogenic KRAS in Pancreatic Cancer. Nature (2017) 546(7659):498–503. doi: 10.1038/nature22341
23. Shan SK, Lin X, Li F, Xu F, Zhong JY, Guo B, et al. Exosomes and Bone Disease. Curr Pharm Des (2019) 25(42):4536–49. doi: 10.2174/1381612825666191127114054
24. Anderson HC, Garimella R, Tague SE. The Role of Matrix Vesicles in Growth Plate Development and Biomineralization. Front Biosci (2005) 10:822–37. doi: 10.2741/1576
25. Buck DW 2nd, Dumanian GA. Bone Biology and Physiology: Part I. Fundamentals Plast Reconstr Surg (2012) 129(6):1314–20. doi: 10.1097/PRS.0b013e31824eca94
26. Wei J, Li H, Wang S, Li T, Fan J, Liang X, et al. Let-7 Enhances Osteogenesis and Bone Formation While Repressing Adipogenesis of Human Stromal/Mesenchymal Stem Cells by Regulating HMGA2. Stem Cells Dev (2014) 23(13):1452–63. doi: 10.1089/scd.2013.0600
27. Li D, Liu J, Guo B, Liang C, Dang L, Lu C, et al. Osteoclast-Derived Exosomal miR-214-3p Inhibits Osteoblastic Bone Formation. Nat Commun (2016) 7:10872. doi: 10.1038/ncomms10872
28. Loftus A, Cappariello A, George C, Ucci A, Shefferd K, Green A, et al. Extracellular Vesicles From Osteotropic Breast Cancer Cells Affect Bone Resident Cells. J Bone Miner Res (2020) 35(2):396–412. doi: 10.1002/jbmr.3891
29. Ensrud KE. Epidemiology of Fracture Risk With Advancing Age. J Gerontol A Biol Sci Med Sci (2013) 68(10):1236–42. doi: 10.1093/gerona/glt092
30. Schuiling KD, Robinia K, Nye R. Osteoporosis Update. J Midwifery Womens Health (2011) 56(6):615–27. doi: 10.1111/j.1542-2011.2011.00135.x
31. Johnell O, Kanis JA. An Estimate of the Worldwide Prevalence and Disability Associated With Osteoporotic Fractures. Osteoporos Int (2006) 17(12):1726–33. doi: 10.1007/s00198-006-0172-4
32. Armas LA, Recker RR. Pathophysiology of Osteoporosis: New Mechanistic Insights. Endocrinol Metab Clin North Am (2012) 41(3):475–86. doi: 10.1016/j.ecl.2012.04.006
33. Boyce BF, Xing L. Functions of RANKL/RANK/OPG in Bone Modeling and Remodeling. Arch Biochem Biophys (2008) 473(2):139–46. doi: 10.1016/j.abb.2008.03.018
34. Fleming A, Sampey G, Chung MC, Bailey C, van Hoek ML, Kashanchi F, et al. The Carrying Pigeons of the Cell: Exosomes and Their Role in Infectious Diseases Caused by Human Pathogens. Pathog Dis (2014) 71(2):109–20. doi: 10.1111/2049-632x.12135
35. Wang Y, Xu F, Zhong JY, Lin X, Shan SK, Guo B, et al. Exosomes as Mediators of Cell-to-Cell Communication in Thyroid Disease. Int J Endocrinol (2020) 2020:4378345. doi: 10.1155/2020/4378345
36. Xu F, Zhong JY, Lin X, Shan SK, Guo B, Zheng MH, et al. Melatonin Alleviates Vascular Calcification and Ageing Through Exosomal miR-204/miR-211 Cluster in a Paracrine Manner. J Pineal Res (2020) 68(3):e12631. doi: 10.1111/jpi.12631
37. Xie Y, Gao Y, Zhang L, Chen Y, Ge W, Tang P. Involvement of Serum-Derived Exosomes of Elderly Patients With Bone Loss in Failure of Bone Remodeling Via Alteration of Exosomal Bone-Related Proteins. Aging Cell (2018) 17(3):e12758. doi: 10.1111/acel.12758
38. Chen M, Li Y, Lv H, Yin P, Zhang L, Tang P. Quantitative Proteomics and Reverse Engineer Analysis Identified Plasma Exosome Derived Protein Markers Related to Osteoporosis. J Proteomics (2020) 228:103940. doi: 10.1016/j.jprot.2020.103940
39. Zhang Y, Cai F, Liu J, Chang H, Liu L, Yang A, et al. Transfer RNA-derived Fragments as Potential Exosome tRNA-Derived Fragment Biomarkers for Osteoporosis. Int J Rheum Dis (2018) 21(9):1659–69. doi: 10.1111/1756-185x.13346
40. Teng Z, Zhu Y, Zhang X, Teng Y, Lu S. Osteoporosis Is Characterized by Altered Expression of Exosomal Long Non-Coding Rnas. Front Genet (2020) 11:566959. doi: 10.3389/fgene.2020.566959
41. Shao JL, Li H, Zhang XR, Zhang X, Li ZZ, Jiao GL, et al. Identification of Serum Exosomal MicroRNA Expression Profiling in Menopausal Females With Osteoporosis by High-Throughput Sequencing. Curr Med Sci (2020) 40(6):1161–9. doi: 10.1007/s11596-020-2306-x
42. Xie Y, Hu JH, Wu H, Huang ZZ, Yan HW, Shi ZY. Bone Marrow Stem Cells Derived Exosomes Improve Osteoporosis by Promoting Osteoblast Proliferation and Inhibiting Cell Apoptosis. Eur Rev Med Pharmacol Sci (2019) 23(3):1214–20. doi: 10.26355/eurrev_201902_17014
43. Zhao P, Xiao L, Peng J, Qian YQ, Huang CC. Exosomes Derived From Bone Marrow Mesenchymal Stem Cells Improve Osteoporosis Through Promoting Osteoblast Proliferation Via MAPK Pathway. Eur Rev Med Pharmacol Sci (2018) 22(12):3962–70. doi: 10.26355/eurrev_201806_15280
44. Qin Y, Wang L, Gao Z, Chen G, Zhang C. Bone Marrow Stromal/Stem Cell-Derived Extracellular Vesicles Regulate Osteoblast Activity and Differentiation In Vitro and Promote Bone Regeneration In Vivo. Sci Rep (2016) 6:21961. doi: 10.1038/srep21961
45. Qiu M, Zhai S, Fu Q, Liu D. Bone Marrow Mesenchymal Stem Cells-Derived Exosomal Microrna-150-3p Promotes Osteoblast Proliferation and Differentiation in Osteoporosis. Hum Gene Ther [Preprint] (2021). doi: 10.1089/hum.2020.005
46. Bhushan R, Grünhagen J, Becker J, Robinson PN, Ott CE, Knaus P. miR-181a Promotes Osteoblastic Differentiation Through Repression of TGF-β Signaling Molecules. Int J Biochem Cell Biol (2013) 45(3):696–705. doi: 10.1016/j.biocel.2012.12.008
47. Hassan MQ, Maeda Y, Taipaleenmaki H, Zhang W, Jafferji M, Gordon JA, et al. miR-218 Directs a Wnt Signaling Circuit to Promote Differentiation of Osteoblasts and Osteomimicry of Metastatic Cancer Cells. J Biol Chem (2012) 287(50):42084–92. doi: 10.1074/jbc.M112.377515
48. Yang X, Yang J, Lei P, Wen T. Lncrna MALAT1 Shuttled by Bone Marrow-Derived Mesenchymal Stem Cells-Secreted Exosomes Alleviates Osteoporosis Through Mediating microRNA-34c/SATB2 Axis. Aging (Albany NY) (2019) 11(20):8777–91. doi: 10.18632/aging.102264
49. Lu GD, Cheng P, Liu T, Wang Z. Bmsc-Derived Exosomal Mir-29a Promotes Angiogenesis and Osteogenesis. Front Cell Dev Biol (2020) 8:608521. doi: 10.3389/fcell.2020.608521
50. Narayanan R, Huang CC, Ravindran S. Hijacking the Cellular Mail: Exosome Mediated Differentiation of Mesenchymal Stem Cells. Stem Cells Int (2016) 2016:3808674. doi: 10.1155/2016/3808674
51. Qi X, Zhang J, Yuan H, Xu Z, Li Q, Niu X, et al. Exosomes Secreted by Human-Induced Pluripotent Stem Cell-Derived Mesenchymal Stem Cells Repair Critical-Sized Bone Defects Through Enhanced Angiogenesis and Osteogenesis in Osteoporotic Rats. Int J Biol Sci (2016) 12(7):836–49. doi: 10.7150/ijbs.14809
52. Li L, Zhou X, Zhang JT, Liu AF, Zhang C, Han JC, et al. Exosomal miR-186 Derived From BMSCs Promote Osteogenesis Through Hippo Signaling Pathway in Postmenopausal Osteoporosis. J Orthop Surg Res (2021) 16(1):23. doi: 10.1186/s13018-020-02160-0
53. Zuo R, Liu M, Wang Y, Li J, Wang W, Wu J, et al. Bm-MSC-derived Exosomes Alleviate Radiation-Induced Bone Loss by Restoring the Function of Recipient BM-MSCs and Activating Wnt/β-Catenin Signaling. Stem Cell Res Ther (2019) 10(1):30. doi: 10.1186/s13287-018-1121-9
54. Xu R, Shen X, Si Y, Fu Y, Zhu W, Xiao T, et al. MicroRNA-31a-5p From Aging BMSCs Links Bone Formation and Resorption in the Aged Bone Marrow Microenvironment. Aging Cell (2018) 17(4):e12794. doi: 10.1111/acel.12794
55. Cheng P, Chen C, He HB, Hu R, Zhou HD, Xie H, et al. miR-148a Regulates Osteoclastogenesis by Targeting V-maf Musculoaponeurotic Fibrosarcoma Oncogene Homolog B. J Bone Miner Res (2013) 28(5):1180–90. doi: 10.1002/jbmr.1845
56. Jiang LB, Tian L, Zhang CG. Bone Marrow Stem Cells-Derived Exosomes Extracted From Osteoporosis Patients Inhibit Osteogenesis Via Microrna-21/SMAD7. Eur Rev Med Pharmacol Sci (2018) 22(19):6221–9. doi: 10.26355/eurrev_201810_16028
57. Ge M, Ke R, Cai T, Yang J, Mu X. Identification and Proteomic Analysis of Osteoblast-Derived Exosomes. Biochem Biophys Res Commun (2015) 467(1):27–32. doi: 10.1016/j.bbrc.2015.09.135
58. You L, Gu W, Chen L, Pan L, Chen J, Peng Y. MiR-378 Overexpression Attenuates High Glucose-Suppressed Osteogenic Differentiation Through Targeting CASP3 and Activating PI3K/Akt Signaling Pathway. Int J Clin Exp Pathol (2014) 7(10):7249–61.
59. Cui Y, Luan J, Li H, Zhou X, Han J. Exosomes Derived From Mineralizing Osteoblasts Promote ST2 Cell Osteogenic Differentiation by Alteration of microRNA Expression. FEBS Lett (2016) 590(1):185–92. doi: 10.1002/1873-3468.12024
60. Hwang S, Park SK, Lee HY, Kim SW, Lee JS, Choi EK, et al. miR-140-5p Suppresses BMP2-Mediated Osteogenesis in Undifferentiated Human Mesenchymal Stem Cells. FEBS Lett (2014) 588(17):2957–63. doi: 10.1016/j.febslet.2014.05.048
61. Yuan FL, Wu QY, Miao ZN, Xu MH, Xu RS, Jiang DL, et al. Osteoclast-Derived Extracellular Vesicles: Novel Regulators of Osteoclastogenesis and Osteoclast-Osteoblasts Communication in Bone Remodeling. Front Physiol (2018) 9:628. doi: 10.3389/fphys.2018.00628
62. Huynh N, VonMoss L, Smith D, Rahman I, Felemban MF, Zuo J, et al. Characterization of Regulatory Extracellular Vesicles From Osteoclasts. J Dent Res (2016) 95(6):673–9. doi: 10.1177/0022034516633189
63. Sun W, Zhao C, Li Y, Wang L, Nie G, Peng J, et al. Osteoclast-Derived microRNA-Containing Exosomes Selectively Inhibit Osteoblast Activity. Cell Discovery (2016) 2:16015. doi: 10.1038/celldisc.2016.15
64. Zhao C, Sun W, Zhang P, Ling S, Li Y, Zhao D, et al. miR-214 Promotes Osteoclastogenesis by Targeting Pten/PI3k/Akt Pathway. RNA Biol (2015) 12(3):343–53. doi: 10.1080/15476286.2015.1017205
65. Qin Y, Peng Y, Zhao W, Pan J, Ksiezak-Reding H, Cardozo C, et al. Myostatin Inhibits Osteoblastic Differentiation by Suppressing Osteocyte-Derived Exosomal Microrna-218: A Novel Mechanism in Muscle-Bone Communication. J Biol Chem (2017) 292(26):11021–33. doi: 10.1074/jbc.M116.770941
66. Fulzele S, Mendhe B, Khayrullin A, Johnson M, Kaiser H, Liu Y, et al. Muscle-Derived miR-34a Increases With Age in Circulating Extracellular Vesicles and Induces Senescence of Bone Marrow Stem Cells. Aging (Albany NY) (2019) 11(6):1791–803. doi: 10.18632/aging.101874
67. Song H, Li X, Zhao Z, Qian J, Wang Y, Cui J, et al. Reversal of Osteoporotic Activity by Endothelial Cell-Secreted Bone Targeting and Biocompatible Exosomes. Nano Lett (2019) 19(5):3040–8. doi: 10.1021/acs.nanolett.9b00287
68. Yang RZ, Xu WN, Zheng HL, Zheng XF, Li B, Jiang LS, et al. Exosomes Derived From Vascular Endothelial Cells Antagonize Glucocorticoid-Induced Osteoporosis by Inhibiting Ferritinophagy With Resultant Limited Ferroptosis of Osteoblasts. J Cell Physiol [Preprint] (2021). doi: 10.1002/jcp.30331
69. Wang J, Hendrix A, Hernot S, Lemaire M, De Bruyne E, Van Valckenborgh E, et al. Bone Marrow Stromal Cell-Derived Exosomes as Communicators in Drug Resistance in Multiple Myeloma Cells. Blood (2014) 124(4):555–66. doi: 10.1182/blood-2014-03-562439
70. Bae KS, Park JB, Kim HS, Kim DS, Park DJ, Kang SJ. Neuron-Like Differentiation of Bone Marrow-Derived Mesenchymal Stem Cells. Yonsei Med J (2011) 52(3):401–12. doi: 10.3349/ymj.2011.52.3.401
71. Lai RC, Arslan F, Lee MM, Sze NS, Choo A, Chen TS, et al. Exosome Secreted by MSC Reduces Myocardial Ischemia/Reperfusion Injury. Stem Cell Res (2010) 4(3):214–22. doi: 10.1016/j.scr.2009.12.003
72. Yeo RW, Lai RC, Zhang B, Tan SS, Yin Y, Teh BJ, et al. Mesenchymal Stem Cell: An Efficient Mass Producer of Exosomes for Drug Delivery. Adv Drug Delivery Rev (2013) 65(3):336–41. doi: 10.1016/j.addr.2012.07.001
73. Chen TS, Lai RC, Lee MM, Choo AB, Lee CN, Lim SK. Mesenchymal Stem Cell Secretes Microparticles Enriched in Pre-Micrornas. Nucleic Acids Res (2010) 38(1):215–24. doi: 10.1093/nar/gkp857
74. Lai RC, Tan SS, Teh BJ, Sze SK, Arslan F, de Kleijn DP, et al. Proteolytic Potential of the MSC Exosome Proteome: Implications for an Exosome-Mediated Delivery of Therapeutic Proteasome. Int J Proteomics (2012) 2012:971907. doi: 10.1155/2012/971907
75. Zhang Y, Cao X, Li P, Fan Y, Zhang L, Ma X, et al. microRNA-935-Modified Bone Marrow Mesenchymal Stem Cells-Derived Exosomes Enhance Osteoblast Proliferation and Differentiation in Osteoporotic Rats. Life Sci (2021) 1(272):119204. doi: 10.1016/j.lfs.2021.119204
76. Cao G, Meng X, Han X, Li J. Exosomes Derived From Circrna Rtn4-modified Bmscs Attenuate TNF-α-Induced Cytotoxicity and Apoptosis in Murine MC3T3-E1 Cells by Sponging Mir-146a. Biosci Rep (2020) 40(5):BSR20193436. doi: 10.1042/bsr20193436
77. Niedermair T, Lukas C, Li S, Stöckl S, Craiovan B, Brochhausen C, et al. Influence of Extracellular Vesicles Isolated From Osteoblasts of Patients With Cox-Arthrosis and/or Osteoporosis on Metabolism and Osteogenic Differentiation of Bmscs. Front Bioeng Biotechnol (2020) 8:615520. doi: 10.3389/fbioe.2020.615520
78. Zhu Y, Li Z, Zhang Y, Lan F, He J, Wu Y. The Essential Role of Osteoclast-Derived Exosomes in Magnetic Nanoparticle-Infiltrated Hydroxyapatite Scaffold Modulated Osteoblast Proliferation in an Osteoporosis Model. Nanoscale (2020) 12(16):8720–6. doi: 10.1039/d0nr00867b
79. Sato M, Suzuki T, Kawano M, Tamura M. Circulating Osteocyte-Derived Exosomes Contain miRNAs Which Are Enriched in Exosomes From MLO-Y4 Cells. BioMed Rep (2017) 6(2):223–31. doi: 10.3892/br.2016.824
80. Pedersen BK. Muscles and Their Myokines. J Exp Biol (2011) 214(Pt 2):337–46. doi: 10.1242/jeb.048074
81. Murphy C, Withrow J, Hunter M, Liu Y, Tang YL, Fulzele S, et al. Emerging Role of Extracellular Vesicles in Musculoskeletal Diseases. Mol Aspects Med (2018) 60:123–8. doi: 10.1016/j.mam.2017.09.006
82. Michalski MN, McCauley LK. Macrophages and Skeletal Health. Pharmacol Ther (2017) 174:43–54. doi: 10.1016/j.pharmthera.2017.02.017
83. Wei F, Li M, Crawford R, Zhou Y, Xiao Y. Exosome-Integrated Titanium Oxide Nanotubes for Targeted Bone Regeneration. Acta Biomater (2019) 86:480–92. doi: 10.1016/j.actbio.2019.01.006
84. Novel Molecular and Cellular Strategies to Optimize Bone Healing. Biomaterials (2019) 196:1. doi: 10.1016/j.biomaterials.2019.01.040
85. Dimitriou R, Jones E, McGonagle D, Giannoudis PV. Bone Regeneration: Current Concepts and Future Directions. BMC Med (2011) 9:66. doi: 10.1186/1741-7015-9-66
86. Cheng C, Shoback D. Mechanisms Underlying Normal Fracture Healing and Risk Factors for Delayed Healing. Curr Osteoporos Rep (2019) 17(1):36–47. doi: 10.1007/s11914-019-00501-5
87. Bahney CS, Hu DP, Miclau T 3rd, Marcucio RS. The Multifaceted Role of the Vasculature in Endochondral Fracture Repair. Front Endocrinol (Lausanne) (2015) 6:4. doi: 10.3389/fendo.2015.00004
88. Schlickewei CW, Kleinertz H, Thiesen DM, Mader K, Priemel M, Frosch KH, et al. Current and Future Concepts for the Treatment of Impaired Fracture Healing. Int J Mol Sci (2019) 20(22):5805. doi: 10.3390/ijms20225805
89. Einhorn TA, Gerstenfeld LC. Fracture Healing: Mechanisms and Interventions. Nat Rev Rheumatol (2015) 11(1):45–54. doi: 10.1038/nrrheum.2014.164
90. Furuta T, Miyaki S, Ishitobi H, Ogura T, Kato Y, Kamei N, et al. Mesenchymal Stem Cell-Derived Exosomes Promote Fracture Healing in a Mouse Model. Stem Cells Transl Med (2016) 5(12):1620–30. doi: 10.5966/sctm.2015-0285
91. Jiang Y, Zhang J, Li Z, Jia G. Bone Marrow Mesenchymal Stem Cell-Derived Exosomal Mir-25 Regulates the Ubiquitination and Degradation of Runx2 by SMURF1 to Promote Fracture Healing in Mice. Front Med (Lausanne) (2020) 7:577578. doi: 10.3389/fmed.2020.577578
92. Zhang L, Jiao G, Ren S, Zhang X, Li C, Wu W, et al. Exosomes From Bone Marrow Mesenchymal Stem Cells Enhance Fracture Healing Through the Promotion of Osteogenesis and Angiogenesis in a Rat Model of Nonunion. Stem Cell Res Ther (2020) 11(1):38. doi: 10.1186/s13287-020-1562-9
93. Zhang Y, Hao Z, Wang P, Xia Y, Wu J, Xia D, et al. Exosomes From Human Umbilical Cord Mesenchymal Stem Cells Enhance Fracture Healing Through HIF-1α-Mediated Promotion of Angiogenesis in a Rat Model of Stabilized Fracture. Cell Prolif (2019) 52(2):e12570. doi: 10.1111/cpr.12570
94. Zhou J, Liu HX, Li SH, Gong YS, Zhou MW, Zhang JH, et al. Effects of Human Umbilical Cord Mesenchymal Stem Cells-Derived Exosomes on Fracture Healing in Rats Through the Wnt Signaling Pathway. Eur Rev Med Pharmacol Sci (2019) 23(11):4954–60. doi: 10.26355/eurrev_201906_18086
95. Xu T, Luo Y, Wang J, Zhang N, Gu C, Li L, et al. Exosomal miRNA-128-3p From Mesenchymal Stem Cells of Aged Rats Regulates Osteogenesis and Bone Fracture Healing by Targeting Smad5. J Nanobiotechnol (2020) 18(1):47. doi: 10.1186/s12951-020-00601-w
96. Wang Y, Chen W, Zhao L, Li Y, Liu Z, Gao H, et al. Obesity Regulates miR-467/HoxA10 Axis on Osteogenic Differentiation and Fracture Healing by BMSC-derived Exosome LncRNA H19. J Cell Mol Med (2021) 25(3):1712–24. doi: 10.1111/jcmm.16273
97. Liu W, Li L, Rong Y, Qian D, Chen J, Zhou Z, et al. Hypoxic Mesenchymal Stem Cell-Derived Exosomes Promote Bone Fracture Healing by the Transfer of Mir-126. Acta Biomater (2020) 103:196–212. doi: 10.1016/j.actbio.2019.12.020
98. Xiong Y, Chen L, Yan C, Zhou W, Yu T, Sun Y, et al. M2 Macrophagy-derived Exosomal miRNA-5106 Induces Bone Mesenchymal Stem Cells Towards Osteoblastic Fate by Targeting Salt-Inducible Kinase 2 and 3. J Nanobiotechnol (2020) 18(1):66. doi: 10.1186/s12951-020-00622-5
99. Claes L, Recknagel S, Ignatius A. Fracture Healing Under Healthy and Inflammatory Conditions. Nat Rev Rheumatol (2012) 8(3):133–43. doi: 10.1038/nrrheum.2012.1
100. Xu JF, Yang GH, Pan XH, Zhang SJ, Zhao C, Qiu BS, et al. Altered microRNA Expression Profile in Exosomes During Osteogenic Differentiation of Human Bone Marrow-Derived Mesenchymal Stem Cells. PloS One (2014) 9(12):e114627. doi: 10.1371/journal.pone.0114627
101. El-Jawhari JJ, Kleftouris G, El-Sherbiny Y, Saleeb H, West RM, Jones E, et al. Defective Proliferation and Osteogenic Potential With Altered Immunoregulatory Phenotype of Native Bone Marrow-Multipotential Stromal Cells in Atrophic Fracture Non-Union. Sci Rep (2019) 9(1):17340. doi: 10.1038/s41598-019-53927-3
102. Lin X, Xiong D, Peng YQ, Sheng ZF, Wu XY, Wu XP, et al. Epidemiology and Management of Osteoporosis in the People’s Republic of China: Current Perspectives. Clin Interv Aging (2015) 10:1017–33. doi: 10.2147/cia.S54613
103. Ge M, Wu Y, Ke R, Cai T, Yang J, Mu X. Value of Osteoblast-Derived Exosomes in Bone Diseases. J Craniofac Surg (2017) 28(4):866–70. doi: 10.1097/scs.0000000000003463
Keywords: exosomes, osteoporosis, fracture healing, bone physiology, osteoporotic fracture
Citation: Yang Z, Zhang W, Ren X, Tu C and Li Z (2021) Exosomes: A Friend or Foe for Osteoporotic Fracture? Front. Endocrinol. 12:679914. doi: 10.3389/fendo.2021.679914
Received: 12 March 2021; Accepted: 10 May 2021;
Published: 21 June 2021.
Edited by:
Ling-Qing Yuan, Central South University, ChinaReviewed by:
Jun Che, Southern University of Science and Technology, ChinaHuiliang Yang, Brown University, United States
Hongwei Wu, Central South University, China
Copyright © 2021 Yang, Zhang, Ren, Tu and Li. This is an open-access article distributed under the terms of the Creative Commons Attribution License (CC BY). The use, distribution or reproduction in other forums is permitted, provided the original author(s) and the copyright owner(s) are credited and that the original publication in this journal is cited, in accordance with accepted academic practice. No use, distribution or reproduction is permitted which does not comply with these terms.
*Correspondence: Chao Tu, dHVjaGFvQGNzdS5lZHUuY24=; Zhihong Li, bGl6aGlob25nQGNzdS5lZHUuY24=