- 1University of Kansas School of Medicine, Kansas City, KS, United States
- 2Children’s Mercy Kansas City, Kansas City, MO, United States
- 3University of Missouri-Kansas City School of Medicine, Kansas City, MO, United States
- 4University of Kansas Medical Center, Kansas City, KS, United States
- 5Center for Children’s Healthy Lifestyles & Nutrition, Kansas City, MO, United States
Obesity is the single greatest risk factor for nonalcoholic fatty liver disease (NAFLD). Without intervention, most pediatric patients with NAFLD continue to gain excessive weight, making early, effective weight loss intervention key for disease treatment and prevention of NAFLD progression. Unfortunately, outside of a closely monitored research setting, which is not representative of the real world, lifestyle modification success for weight loss in children is low. Bariatric surgery, though effective, is invasive and can worsen NAFLD postoperatively. Thus, there is an evolving and underutilized role for pharmacotherapy in children, both for weight reduction and NAFLD management. In this perspective article, we provide an overview of the efficacy of weight reduction on pediatric NAFLD treatment, discuss the pros and cons of currently approved pharmacotherapy options, as well as drugs commonly used off-label for weight reduction in children and adolescents. We also highlight gaps in, and opportunities for, streamlining obesity trials to include NAFLD assessment as a valuable, secondary, therapeutic outcome measure, which may aid drug repurposing. Finally, we describe the already available, and emerging, minimally-invasive biomarkers of NAFLD that could offer a safe and convenient alternative to liver biopsy in pediatric obesity and NAFLD trials.
Introduction
With 340 million children worldwide affected by obesity (1), the rate of nonalcoholic fatty liver disease (NAFLD) is expected to rise because obesity remains the single most significant risk factor for NAFLD (2). The pathophysiology of NAFLD in obesity is complex and likely involves the interplay and dysregulation of multiple mechanisms beyond the scope of this publication (e.g., insulin resistance, cytokine signaling, gut microbiota) (3); however, continued abnormal weight gain is a common culprit in fueling the disease. NAFLD affects up to 70% of children and up to 90% of adults with obesity (2), and has become a leading indication for liver transplant in the United States (4). Without intervention, more than 75% of children with obesity will continue to gain excessive weight and become adults with obesity (5). Therefore, in addition to effective childhood weight management strategies, readily available non-invasive diagnostic and monitoring modalities are needed for NAFLD management, in order to prevent obesity-associated NAFLD progression from simple hepatic steatosis to steatohepatitis, fibrosis, cirrhosis, hepatocellular carcinoma, and end-stage liver failure in adulthood.
Weight reduction through lifestyle modification remains the first-line treatment approach for NAFLD (6). The present work aims to review the efficacy of weight reduction on pediatric NAFLD and to illustrate opportunities for improving NAFLD diagnosis, monitoring, and treatment outcomes through pharmacologic interventions.
Lifestyle Modifications for Weight Reduction
Lifestyle modification for weight reduction and lasting improvement in health outcomes requires change in multiple areas, including diet and exercise (7). Weight loss of as little as one kilogram has been shown to improve clinical serum biomarkers of NAFLD in children (8), and biopsy-proven NAFLD if weight is subsequently maintained for 24 months (9). Consequently, many dietary interventions have been explored in pediatrics, including portion controlled, reduced glycemic load, low carbohydrate, meal replacement, and time restricted eating, as well as very low calorie diets and protein sparing modified fasting for more severe obesity (10–14). Similar to findings in adults (15), the most effective diet for pediatric weight management is the one that can be sustained successfully over time, with generally less adherence observed for low carbohydrate diets and better adherence to portion control and reduced glycemic load (10). These dietary changes are the recommended first step for NAFLD management, in combination with regular moderate to high intensity exercise (2, 6). Although there is currently insufficient evidence to recommend one type of exercise program over another for NAFLD (16), there is some evidence to suggest that a diet low in free sugar (17) and the Mediterranean diet specifically may have added benefit in treating NAFLD, independent of weight loss (18). Although adherence to these two diets was exceptionally high in some pediatric studies (8–10), this is atypical of most other research studies (19), and likely attributable to intense follow-up (8–10) and in-home interventions (10) that are not available, nor practical in the clinical setting. Dietary recommendations for weight loss and NAFLD are summarized in Figure 1, and are generally recommended in conjunction with exercise (>150 minutes/week moderate intensity, or >75 minutes/week high intensity) (16), or moderate to vigorous physical activity daily for at least 20 minutes (with a goal of 60 minutes) (7).
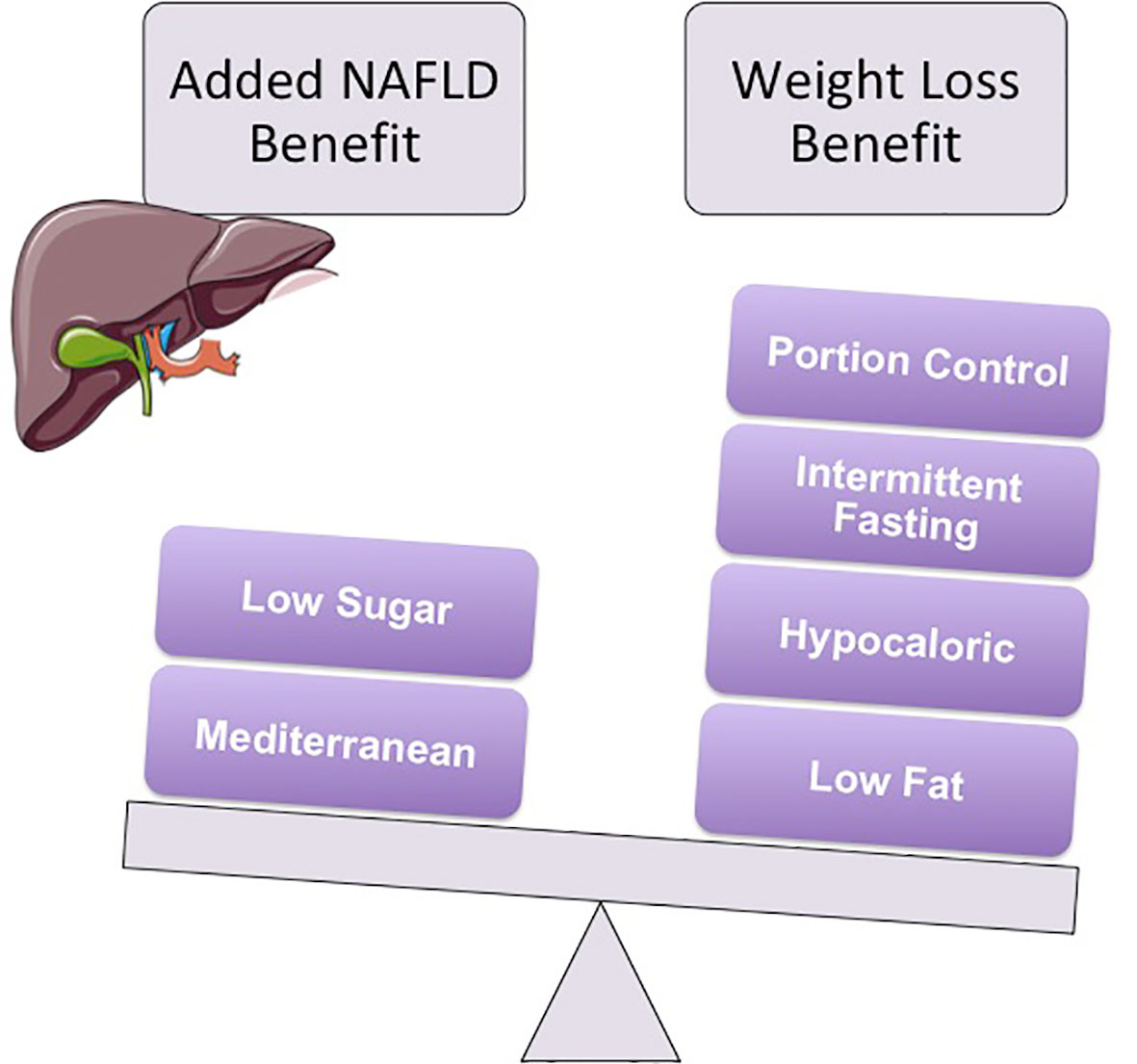
Figure 1 Multiple diets have shown efficacy for weight reduction in children. Ultimately, the best diet for weight management is the one that can be sustained over time. However, both the Mediterranean (i.e., low in red meat and high in fruits, vegetables, grains, nuts, grilled chicken and fish) and the low free sugar diet have shown added benefit in pediatric NAFLD, independent of weight reduction. Generally these diets are recommended in conjunction with regular exercise (e.g., at least 150 minutes/week of moderate intensity or >75 minutes/week of high intensity exercise), or moderate to vigorous physical activity daily for at least 20 minutes (with a target goal of 60 minutes daily).
In clinical practice, these lifestyle modifications show heterogeneity in patient adherence and treatment response (20). In pediatrics, obesity is defined by body mass index (BMI) at or above the 95th percentile for age and sex, and treatment response assessed using the change in the percent of the 95th percentile (%BMIp95) (21, 22). In a large national prospective registry of children participating in non-surgical, multicomponent, intensive behavioral clinical treatment for obesity (n=6,454), the mean change in %BMIp95 after lifestyle modification was -1.88% at 4 to 6 months, -2.5% at 7 to 9 months, and -2.86% at >12 months – showing a modest decline in weight loss over time. Only those patients who achieved -5.2% change in %BMIp95 showed improvement in alanine aminotransferase (ALT), a clinical biomarker of liver injury (23). Whereas, those patients who experienced a change from normal to abnormal ALT had an increase in %BMIp95 of 0.48% (23), highlighting heterogeneity in treatment success.
Upon closer examination of the experience of a single program in this registry, only one third of the 769 participants achieved a >5% decrease in %BMIp95–the amount of BMI change associated with improvement in ALT and liver health (20). Increased number of clinical follow-up visits and days of physical activity were associated with greater odds of successful weight loss, defined as achieving >5% decrease in %BMIp95 (23). To illustrate the close clinical contact necessary for weight loss program success, guidance for moderately intense lifestyle modification therapy from the United States Preventative Services Task Force (USPSTF) recommends 26-75 patient contact hours over a 6-12 month period (24). However, in our experience, children with NAFLD are typically seen in clinic for approximately one hour every 2-3 months, falling short of the USPSTF-recommended contact hour target for weight management.
Surgery for Weight Reduction
Bariatric surgery (e.g., laparoscopic sleeve gastrectomy) is an effective intervention for weight reduction (25), with post-surgical weight loss of approximately 20% total body weight in both adults (26) and children (27). Although less common in pediatrics, bariatric surgery is becoming an increasingly recognized option for children greater than 10 to 12 years of age with BMI >120% of the 95th percentile (or BMI of 35 kg/m2) and comorbid NAFLD (26, 28). Recent investigations in adolescents suggest that bariatric surgery results in a four-fold reduction in hepatic fat fraction, with resolution of hepatic steatosis at 6 months post-surgery on imaging (29, 30), and 100% resolution of steatosis and 90% resolution of fibrosis on liver biopsy at 12 months post-surgery (27).
Despite its promise in treating pediatric obesity and NAFLD, bariatric surgery also appears to worsen NAFLD in a subset of patients. Although no pediatric data are available, in a large meta-analysis of 32 adult studies on bariatric surgery, 19 reported worsening or new NAFLD features, including steatosis, inflammation, and fibrosis in approximately 12% of patients postoperatively (25). Alarmingly, no apparent risk factors could be identified for this serious postoperative complication (25). Thus, the risks of bariatric surgery should be balanced against the potential for advantageous surgical outcomes for both obesity and NAFLD, and pharmacologic agents discussed as treatment adjuncts and/or alternative options.
Pharmacotherapy for Weight Reduction
Currently, two pharmaceutical agents have approval from the United States Food and Drug Administration (FDA) for weight loss indications in children >12 years (orlistat and liraglutide), and one additional agent for adolescents >16 years (phentermine) (31, 32). Of these agents, orlistat’s use is somewhat limited by its gastrointestinal side-effect profile (e.g., diarrhea, bloating) (33); although, it was found to be moderately effective in decreasing BMI from 0.55 up to 4.2 kg/m2 (34–37). Phentermine is a stimulant and a Class IV controlled substance, necessitating increased consideration when prescribing in children. Additional screening and monitoring is necessary in children with congenital heart disease, cardiovascular disease, and thyroid abnormalities similar to protocols used when prescribing stimulants for other indications common in pediatrics (32). Phentermine also does not appear to be as effective in achieving weight loss in adolescents as in adults (31). As such, ligratulide–a GLP1 agonist newly approved by the FDA in December 2020 for the treatment of pediatric obesity–may soon emerge as the frontrunner. After somewhat disappointing BMI reduction results in an earlier trial in children with type II diabetes (38), results from a more recent, randomized, controlled trial demonstrate a decrease in BMI by 1.58 kg/m2 compared to placebo, when liraglutide was administered to children with obesity along with lifestyle modifications (39). Although therapeutic end-points for NAFLD were not included in this trial, greater than 5% reduction in BMI, previously associated with improvement in NAFLD (20), was achieved in almost half (43%) of liraglutide-treated participants, versus 19% of participants receiving lifestyle modification therapy alone (39). Additional GLP1 agonists are under investigation and it is our opinion that NAFLD assessment ought to be considered as a secondary treatment outcome in these trials, particularly as minimally-invasive biomarkers of NAFLD become more readily available and mitigate the need for liver biopsy.
The overall efficacy of GLP1 agonists appears comparable to other medications already labeled for pediatric indications and being used off-label for weight loss in children. For example, metformin, a first-line treatment for pediatric type II diabetes mellitus, has been associated with a modest BMI reduction in children (40). Recent studies looking at metformin demonstrated a decrease of -1.07 and -1.3 kg/m2 over 6 months (41), with a wide range of success (i.e., +0.38 to -2.52 kg/m2 BMI change) noted in some studies (40). Although the observed variability in treatment response may, in part, be related to variability in adherence to concomitant lifestyle modifications, we believe it is also related to variability in systemic drug exposure (i.e., pharmacokinetics) achieved from a given metformin dose administered to an individual child (42). Additional research is ongoing into potential sources of inter-individual variability (e.g., genetics) in metformin pharmacokinetics and pharmacodynamics (i.e., drug efficacy). Meanwhile, the GLP1 agonist exenatide appears to show efficacy comparable to metformin, with placebo-subtracted reduction of 1.3 kg/m2 (approximately -2.7% BMI) over 3 months, and -4% BMI over 6 months, in pediatrics (41). A small clinical study of topiramate, an anti-epilepsy drug already approved for pediatric use, also shows a 6-month decrease in BMI similar to exenatide (-4.6%), in 48 pediatric patients with severe obesity (43). Thus, medications currently indicated for pediatric weight reduction, as well as those approved for pediatric indications and administered off-label for the purpose of weight reduction, may have a role in weight management for pediatric patients. However, currently available data suggest that the range of weight loss achieved with pharmacotherapy may be only marginally greater than lifestyle modifications alone.
In randomized controlled trials, the mean weight change for lifestyle modification therapy was -1.8% BMI, compared to -2.8% BMI when therapy was combined with liraglutide or topiramate (44). Similarly, the mean pooled reduction in BMI in response to metformin therapy in children with obesity was -0.86 kg/m2, and fell within the BMI reduction ranges from lifestyle modifications alone (-0.02 to -1.10 kg/m2) (45). Importantly, on average, none of these weight management approaches achieved a reliable >5% reduction in BMI, deemed necessary for NAFLD improvement (20). Thus, though weight-reduction is an important first-line treatment for children with NAFLD, and may be achieved through lifestyle modifications, bariatric surgery or pharmacotherapy, each approach is associated with substantial variability in treatment response. This leaves a gap in universally effective treatments for pediatric NAFLD, and a potential role for precision therapeutics and pharmacotherapy.
Pharmacotherapy for NAFLD
Currently, there are no FDA-approved medications for pediatric NAFLD. However, the landscape of potential therapeutic agents for NAFLD is evolving rapidly and showing promise, as highlighted in recently published reviews (26, 46) beyond the scope of this publication. In addition to novel therapeutic agents (e.g., obeticholic acid, fibroblast growth factor 19 & 21 analogs, thyroid hormone receptor-β agonists), there is also interest in repurposing existing drugs–already approved for pediatric obesity or obesity comorbidities–for the treatment of pediatric NAFLD (47).
One example is the lipid-lowering drug class statins. In addition to improving cardiovascular disease outcomes, statins have demonstrated significant improvement in hepatic function for patients with obesity and NAFLD (48, 49), likely through secondary anti-inflammatory effects. In adults with NAFLD, statin therapy is well-tolerated and has a similar, low frequency of hepatotoxicity comparable to placebo (48, 50), making it a potential candidate for NAFLD management. However, efficacy data remain equivocal (50) and genotype-stratified investigations may be necessary to identify therapeutic target ranges appropriate for NAFLD treatment indications.
It would be remiss not to mention oxidative stress as a potential therapeutic target in NAFLD management. Anti-oxidants (e.g. vitamin E, omega-3 fatty acids) have been used both for the treatment of pediatric weight reduction and NAFLD, with mixed results (8, 51–53). In one longitudinal, randomized, placebo-controlled trial, vitamin E did not demonstrate advantage over weight reduction through lifestyle modifications for NAFLD or obesity (8). In another longitudinal, placebo-controlled trial of vitamin E vs. metformin, neither agent resulted in sustained ALT reduction (51). However, resolution of steatohepatitis was significantly higher with vitamin E compared to placebo, even though BMI reduction was equivocal (51). Conversely, in a trial of omega-3 fatty acids, children treated with omega-3s were more likely to have BMI reduction compared to placebo, but reduction in ALT was equivocal (52, 53). Collectively, these studies indicate equipoise in anti-oxidant efficacy for NAFLD and highlight the need for further study.
Many other agents, already being used on and off-label for weight reduction, have been proposed to have therapeutic benefit for NAFLD (54), though few have been evaluated in children. These include orlistat; GLP1 agonists, such as liraglutide, semaglutide, and exenatide; and SGLT2 inhibitors. While some pediatric studies of orlistat have shown select improvement in serum liver injury markers (55), the majority of studies have not examined the effect of orlistat on liver adiposity or histopathology. Available data to date demonstrate no clear improvement in liver pathology that is independent of orlistat-induced weight loss (54), making orlistat a questionable drug candidate for primary NAFLD indications in children (56). To our knowledge, liraglutide and other GLP1 agonists have not been evaluated as pharmacotherapy for pediatric NAFLD; however, multiple studies in adults demonstrate promising improvement in hepatic steatosis and liver injury markers associated with GLP1 agonist treatment (54, 57). It is unclear whether this effect on NAFLD is independent of weight-loss, a caveat that extends to SGLT2 inhibitors, which also demonstrate some benefit for NAFLD treatment in adults (58). Parallel studies in pediatrics are indicated, particularly now that liraglutide is approved for weight loss indications in children, and more agents may be on the horizon soon.
Metformin, a drug already FDA-approved for type II diabetes in children and used off-label for pediatric weight loss (40, 41), has also shown some promise for repurposing in pediatric NAFLD. In a study of lifestyle modifications combined with either metformin or placebo in children with obesity and insulin resistance +/- NAFLD, the metformin group demonstrated a significant decrease in NAFLD scores and NAFLD prevalence, while the placebo group experienced an increase in both (59). Other studies in pediatrics using metformin at lower doses demonstrated isolated improvement in hepatic ballooning (51) or lobular inflammation (9) on histopathology, but failed to show an overall improvement in steatosis or NAFLD scores, suggesting that the effects of metformin on NAFLD may be dose-dependent. Improvement in serum ALT has been equivocal between studies, with 2 out of 4 pediatric studies demonstrating improvement in ALT (59, 60). However, this is likely because ALT, along with other currently available clinical markers of liver injury, lacks specificity for NAFLD (25).
Minimally-Invasive Biomarkers of NAFLD
The diagnostic gold standard for NAFLD in children is liver biopsy. However, liver biopsy is invasive and not practical for recurrent monitoring of NAFLD. Instead, serum ALT is the most commonly used biomarker of NAFLD, but it is non-specific and can be normal in >80% of patients with NAFLD (25). Therefore, minimally-invasive and highly-specific biomarkers are urgently needed to diagnose and monitor NAFLD.
In the past decade, several new, potential biomarkers of NAFLD have been identified by serum proteomics/metabolomics analyses (61, 62). Based on their origin, these markers can be grouped into hepatokines, adipokines, and other cytokines. Although detailed review of these novel biomarker candidates is beyond the scope of this publication, the most promising biomarkers are summarized in Table 1. In addition, serum bile acids (BAs) are highlighted as another emerging class of biomarkers.
BAs are synthesized in the liver, excreted into the bile, metabolized by the intestinal microbiome, and undergo several enterohepatic recirculations. Therefore, changes in the BA metabolome could be pathognomonic for various stages of NAFLD. The ratio of the primary BAs, cholic acid and chenodeoxycholic acid, is higher in patients with simple steatosis, as well as more advanced NAFLD (90). Compared to healthy controls, total fasting and postprandial primary BAs, and the ratios of taurine- and glycine-conjugated BAs, are consistently higher in the sera of adults with nonalcoholic steatohepatitis (NASH) (91). However, the role of secondary BAs as potential biomarkers remains debated, as the two studies above showed different results. Puri et al. observed lower total secondary BAs in adults with NASH compared to controls (90), whereas Ferslew et al. observed higher secondary BAs (91). In pediatrics, BAs study results are sparse and inconsistent, but could offer a valuable, minimally-invasive modality for NAFLD monitoring during treatment trials. Significantly lower total serum BA concentrations were found in one pediatric study of hepatic steatosis (92), but not in others (93, 94). Notably, two of these studies demonstrated decreased concentrations of glycine- and taurine-conjugated deoxycholic acid in children with NAFLD steatosis and/or NASH, compared to healthy controls (92, 93). The third study also found serum concentrations of glycine conjugated deoxycholic acid to be lower in children with NAFLD, but not in children with NASH (94). Together, these observations suggest that bile acid homeostasis in pediatric NAFLD may be distinct from the adult disease phenotype. Therefore, BAs results should not be extrapolated from adults to children, and further investigation in pediatric patients is warranted.
While serum biomarkers of NAFLD remain under investigation, several liver imaging biomarkers have become available. Magnetic resonance proton density fat fraction (MR-PDFF) is a non-invasive technique that quantifies the percentage of fat within a given volume of tissue. It correlates closely with steatosis grade on liver biopsy in children (95) and accurately captures longitudinal changes in pediatric NAFLD over time (96). However, it is only available in specialized tertiary care centers and is expensive. Ultrasound techniques for fat quantification are not yet widely available, but could offer a cheaper, more accessible alternative to magnetic resonance fat quantification, and facilitate more consistent and better quality disease monitoring for pediatric obesity and NAFLD trials.
For those imaging biomarkers that are widely available, one consistent shortcoming is that they perform best for tracking more severe stages of NAFLD/NASH, typical of adult disease. Grayscale ultrasound has been used for many years as a qualitative estimate of hepatic fat content. However, this technique is unreliable and non-specific for hepatic fat quantification, due to overlap in appearance between fatty and fibrotic liver (97, 98). Similarly, although both ultrasound and magnetic resonance elastography can quantify liver stiffness as a surrogate for fibrosis, NAFLD presents a challenge because measurement values are affected by both fat and fibrosis (99). Therefore, an ideal pediatric imaging surveillance program would incorporate quantitative imaging measurements that could discriminate fat and fibrosis.
Discussion
Obesity is the single greatest risk factor for NAFLD in adults and children (2). Although weight loss of as little as 1 kilogram can improve NAFLD in children (8), consistent implementation and maintenance of intensive lifestyle modifications necessary to induce and sustain such weight loss are low (19, 20). Bariatric surgery is becoming increasingly recognized as an option for weight reduction in children (26), but it is invasive, and there is an unpredictable subset of patients who experience worsening liver fibrosis and NAFLD progression after surgery (25). This leaves room for pharmacologic interventions to aid with weight loss in pediatric obesity to treat and prevent NAFLD.
Without intervention, most pediatric patients with NAFLD continue to gain excessive weight (5) and become adults with NAFLD. Thus, inclusion of children and adolescents in pharmacology trials of novel weight-loss and NAFLD treatments is paramount. Repurposing drugs already labeled for pediatric use in comorbidities associated with obesity may be a complementary strategy to expand pediatric NAFLD treatment options beyond lifestyle modifications or bariatric surgery. Importantly, incorporation of NAFLD treatment end-points into obesity trials up-front would streamline efforts to assess a given drug’s efficacy for pediatric NAFLD, as well as obesity, and potentially help discriminate drug effect from weight-loss effect on NAFLD.
Regardless of the treatment approach selected, to streamline and facilitate treatment trials in pediatrics, minimally-invasive, highly-specific biomarkers of NAFLD are urgently needed to augment advanced imaging techniques that offer an alternative to liver biopsy, but are expensive and inaccessible in most clinical settings. A concerted effort must be made to include children in NAFLD biomarkers and therapeutics trials in order to intervene early and effectively in disease progression that is fueled by continued excessive weight gain from childhood into adulthood. Best strategies for including children in NAFLD trials are under development, as referenced in a draft guidance for industry from the FDA (100), and additional tips for optimal patient selection and age-appropriate therapeutic end-points can be found in a recent review by Alkhouri et al. (101).
Data Availability Statement
The original contributions presented in the study are included in the article/supplementary material. Further inquiries can be directed to the corresponding author.
Author Contributions
VS and CF conceived the idea for the manuscript. VS, CH-C, SC, IC, JW, BS, and JF provided expert opinion. All authors contributed to the article and approved the submitted version.
Funding
This work was supported in part by grant 5K23DK115827-02 (V.S.), a CTSA grant from NCATS awarded to the University of Kansas for Frontiers: University of Kansas Clinical and Translational Science Institute (Ul1TR003266), with VS as a recipient, and a CTSA grant from NCATS awarded to the University of Kansas for Frontiers: University of Kansas Clinical and Translational Science Institute (KL2TR002367), with JW as recipient. The contents are solely the responsibility of the authors and do not necessarily represent the official views of the NIH or NCATS.
Conflict of Interest
The authors declare that the research was conducted in the absence of any commercial or financial relationships that could be construed as a potential conflict of interest.
References
1. World Health Organization. (2018). Available at: https://www.who.int/end-childhood-obesity/publications/taking-action-childhood-obesity-report/en/ (Accessed January 11, 2021).
2. Temple J, Cordero P, Li J, Nguyen V, Oben JA. A Guide to Non-Alcoholic Fatty Liver Disease in Childhood and Adolescence. Int J Mol Sci (2016) 17(6):947. doi: 10.3390/ijms17060947
3. Fang YL, Chen H, Wang CL, Liang L. Pathogenesis of non-alcoholic fatty liver disease in children and adolescence: From “two hit theory” to “multiple hit model”. World J Gastroenterol (2018) 24(27):2974–83. doi: 10.3748/wjg.v24.i27.2974
4. Pais R, Barritt A, Calmus Y, Scatton O, Runge T, Lebray P, et al. NAFLD and liver transplantation: Current burden and expected challenges. J Hepatol (2016) 65(6):1245–57. doi: 10.1016/j.jhep.2016.07.033
5. Lifshitz F. Obesity in Children. J Clin Res Pediatr Endocrinol (2008) 1(2):53–60. doi: 10.4008/jcrpe.v1i2.35
6. Shah J, Okubote T, Alkhouri N. Overview of Updated Practice Guidelines for Pediatric Nonalcoholic Fatty Liver Disease. Gastroenterol Hepatol (2018) 14(7):407–14.
7. Styne DM, Arslanian SA, Connor EL, Farooqi IS, Murad MH, Silverstein JH, et al. Pediatric Obesity-Assessment, Treatment, and Prevention: An Endocrine Society Clinical Practice Guideline. J Clin Endocrinol Metab (2017) 102(3):709–57. doi: 10.1210/jc.2016-2573
8. Nobili V, Manco M, Devito R, Ciampalini P, Piemonte F, Marcellini M. Effect of Vitamin E on Aminotransferase Levels and Insulin Resistance in Children with Nonalcoholic Fatty Liver Disease. Aliment Pharmacol Ther (2006) 24:1553–61. doi: 10.1111/j.1365-2036.2006.03161.x
9. Nobili V, Manco M, Devito R, Di Ciommo V, Comparcola D, Sartorelli M, et al. Lifestyle Intervention and Antioxidant Therapy in Children with Nonalcoholic Fatty Liver Disease: A Randomized, Controlled Trial. Hepatology (2008) 4(1):119–28. doi: 10.1002/hep.22336
10. Kirk S, Brehm B, Saelens BE, Woo JG, Kissel E, D’Alessio D, et al. Role of carbohydrate modification in weight management among obese children: a randomized clinical trial. J Pediatr (2012) 161(2):320–7. doi: 10.1016/j.jpeds.2012.01.041
11. Berkowitz RI, Wadden TA, Gehrman CA, Bishop-Gilyard CT, Moore RH, Womble LG, et al. Meal replacements in the treatment of adolescent obesity: a randomized controlled trial. Obesity (Silver Spring) (2011) 19(6):1193–9. doi: 10.1038/oby.2010.288
12. Fox CD, Kaizer AM, Rudser KD, Nathan BM, Gross AC, Sunni M, et al. Meal-Replacements followed by Topiramate for the Treatment of Adolescent Severe Obesity: A Pilot Randomized Controlled Trial. Obesity (Silver Spring) (2016) 24(12):2553–61. doi: 10.1002/oby.21633
13. Jebeile H, Gow ML, Lister NB, Haghighi MM, Ayer J, Cowell CT, et al. Intermittent Energy Restriction Is a Feasible, Effective, and Acceptable Intervention to Treat Adolescents with Obesity. J Nutr (2019) 149(7):1189–97. doi: 10.1093/jn/nxz049
14. Andela S, Burrows TL, Baur LA, Coyle DH, Collins CE, Gow ML. Efficacy of very low-energy diet programs for weight loss: A systematic review with meta-analysis of intervention studies in children and adolescents with obesity. Obes Rev (2019) 20(6):871–82. doi: 10.1111/obr.12830
15. Ge L, Sadeghirad B, Ball GDC, da Costa BR, Hitchcock CL, Svendrovski A, et al. Comparison of dietary macronutrient patterns of 14 popular named dietary programs for weight and cardiovascular risk factor reduction in adults: systematic review and network meta-analysis of randomized trials. BMJ (2020) 369:m696. doi: 10.1136/bmj.m696
16. Schwimmer JB, Ugalde-Nicalo P, Welsh J, Angeles J, Cordero M, Harlow K, et al. Effect of a Low Free Sugar Diet vs Usual Diet on Nonalcoholic Fatty Liver Disease in Adolescent Boys. JAMA (2019) 321(3):256. doi: 10.1001/jama.2018.20579
17. Thyfault JP, Rector RS. Exercise Combats Hepatic Steatosis: Potential Mechanisms and Clinical Implications. Diabetes (2020) 69:517–24. doi: 10.2337/dbi18-0043
18. Ryan MC, Itsiopoulos C, Thodis T, Ward G, Trost N, Jofferberth S, et al. The Mediterranean diet improves hepatic steatosis and insulin sensitivity in individuals with non-alcoholic fatty liver disease. J Hepatol (2013) 59(1):138–43. doi: 10.1016/j.jhep.2013.02.012
19. Cakir M, Akbulut UE, Okten A. Association between Adherence to the Mediterranean Diet and Presence of Nonalcoholic Fatty Liver Disease in Children. Child Obes (2016) 12(4):278–85. doi: 10.1089/chi.2015.0197
20. Gorecki MC, Feinglass JM, Binns HJ. Characteristics Associated with Successful Weight Management in Youth with Obesity. J Pediatr (2019) 212:35–43. doi: 10.1016/j.jpeds.2019.05.039
21. Freedman DS, Berenson GS. Tracking of BMI z Scores for Severe Obesity. Pediatrics (2017) 140(3):e20171072. doi: 10.1542/peds.2017-107
22. Freedman DS, Butte NF, Taveras EM, Lundeen EA, Blanck HM, Goodman AB, et al. BMI z-Scores are a poor indicator of adiposity among 2- to 19-year-olds with very high BMIs, NHANES 1999-2000 to 2013-2014. Obesity (2017) 25(4):739–46. doi: 10.1002/oby.21782
23. Kumar S, King EC, Christison AL, Kelly AS, Ariza AJ, Borzutzky C, et al. Health Outcomes of Youth in Clinical Pediatric Weight Management Programs in POWER. J Pediatr (2019) 208:57–65.e4. doi: 10.1016/j.jpeds.2018.12.049
24. Whitlock EP, O’Connor EA, Williams SB, Beil TL, Lutz KW. Effectiveness of weight management interventions in children: a targeted systematic review for the USPSTF. Pediatrics (2010) 125(2):e396–418. doi: 10.1542/peds.2009-1955
25. Lee Y, Doumouras AG, Yu J, Brar K, Banfield L, Gmora S, et al. Complete Resolution of Nonalcoholic Fatty Liver Disease After Bariatric Surgery: A Systematic Review and Meta-analysis. Clin Gastroenterol Hepatol (2019) 17(6):1040–60. doi: 10.1016/j.cgh.2018.10.017
26. Nobili V, Alisi A, Valenti L, Miele L, Feldstein AE, Alkhouri A. NAFLD in children: new genes, new diagnostic modalities and new drugs. Nat Rev Gastroenterol Hepatol (2019) 16:517–30. doi: 10.1038/s41575-019-0169-z
27. Manco M, Mosca A, De Peppo F, Caccamo R, Cutrere R, Giordano U, et al. The Benefit of Sleeve Gastrectomy in Obese Adolescents on Nonalcoholic Steatohepatitis and Hepatic Fibrosis. J Pediatr (2017) 180:31–7e2. doi: 10.1016/j.jpeds.2016.08.101
28. Pratt JSA, Browne A, Browne NT, Bruzoni M, Cohen M, Desai A. ASMBS pediatric metabolic and bariatric surgery guidelines, 2018. Surg Obes Relat Dis (2018) 14:882–901. doi: 10.1016/j.soard.2018.03.019
29. Luo RB, Suzuki T, Hooker JC, Covarrubias Y, Schlein A, Liu S, et al. How bariatric surgery affects liver volume and fat density in NAFLD patients. Surg Endosc (2018) 32:1675–82. doi: 10.1007/s00464-017-5846-9
30. Hui SCN, Wong SKH, Ai Q, Yeung DKW, Ng EKW, Chu WCW. Observed changes in brown, white, hepatic and pancreatic fat after bariatric surgery: evaluation with MRI. Eur Radiol (2019) 29(2):849–56. doi: 10.1007/s00330-018-5611-z
31. Ryder JR, Kaizer A, Rudser KD, Gross A, Kelly AS, Fox CK. Effect of phentermine on weight reduction in a pediatric weight management clinic. Int J Obes (Lond) (2017) 41(1):90–3. doi: 10.1038/ijo.2016.185
32. Srivastava G, Fox CK, Kelly AS, Jastreboff AM, Browne AF, Browne NT, et al. Clinical Considerations Regarding the Use of Obesity Pharmacotherapy in Adolescents with Obesity. Obesity (Silver Spring) (2019) 27(2):190– 204. doi: 10.1002/oby.22385
33. Ozkan B, Bereket A, Turan S, Keskin S. Addition of orlistat to conventional treatment in adolescents with severe obesity. Eur J Pediatr (2004) 163(12):738–41. doi: 10.1007/s00431-004-1534-6
34. O’Connor EA, Evans CV, Burda BU, Walsh ES, Eder M, Lozano P. Screening for Obesity and Intervention for Weight Management in Children and Adolescents: Evidence Report and Systematic Review for the US Preventive Services Task Force. JAMA (2017) 317(23):2427–44. doi: 10.1001/jama.2017.0332
35. McDuffie JR, Calis KA, Uwaifo GI, Sebring NC, Fallon EM, Hubbard VS, et al. Three-month tolerability of orlistat in adolescents with obesity-related comorbid conditions. Obes Res (2002) 10(7):642–50. doi: 10.1038/oby.2002.87
36. Chanoine JP, Hampl S, Jensen C, Boldrin M, Hauptman J. Effect of orlistat on weight and body composition in obese adolescents: a randomized controlled trial. JAMA (2005) 293(23):2873–83. doi: 10.1001/jama.293.23.2873
37. Maahs D, de Serna DG, Kolotkin RL, Ralston S, Sandate J, Qualls C, et al. Randomized, double-blind, placebo-controlled trial of orlistat for weight loss in adolescents. Endocr Pract (2006) 12(1):18–28. doi: 10.4158/EP.12.1.18
38. Danne T, Biester T, Kapitzke K, Jacobsen SH, Jacobsen LV, Carlsson Petri KC, et al. Liraglutide in an Adolescent Population with Obesity: A Randomized, Double-Blind, Placebo-Controlled 5-Week Trial to Assess Safety, Tolerability, and Pharmacokinetics of Liraglutide in Adolescents Aged 12-17 Years. J Pediatr (2017) 181:146–53.e143. doi: 10.1016/j.jpeds.2016.10.076
39. Kelly AS, Auerbach P, Barrientos-Perez M, Gies I, Hale PM, Marcus C, et al. A Randomized, Controlled Trial of Liraglutide for Adolescents with Obesity. N Engl J Med (2020) 382(22):2117–28. doi: 10.1056/NEJMoa1916038
40. McDonagh MS, Selph S, Ozpinar A, Foley C. Systematic review of the benefits and risks of metformin in treating obesity in children aged 18 years and younger. JAMA Pediatr (2014) 168(2):178–84. doi: 10.1001/jamapediatrics.2013.4200
41. Kelly AS, Fox CK, Rudser KD, Gross AC, Ryder JR. Pediatric obesity pharmacotherapy: current state of the field, review of the literature and clinical trial considerations. Int J Obes (Lond) (2016) 40(7):1043–50. doi: 10.1038/ijo.2016.69
42. Hosey-Cojocari C, Halpin K, Yan Y, Leeder J. Towards an individualized dosing strategy to reduce pharmacokinetic (PK) variability and improve response to metformin in children with insulin resistance or type 2 diabetes. Clin Pharmacol Ther (2020) 107(Suppl 1):S110, Abstract PIII–117.
43. Fox CK, Marlatt KL, Rudser KD, Kelly AS. Topiramate for weight reduction in adolescents with severe obesity. Clin Pediatr (Phila) (2015) 54(1):19–24. doi: 10.1177/0009922814542481
44. Ryder JR, Kaizer AM, Jenkins TM, Kelly AS, Inge TH, Shaibi GQ. Heterogeneity in Response to Treatment of Adolescents with Severe Obesity: The Need for Precision Obesity Medicine. Obesity (Silver Spring) (2019) 27(2):288–94. doi: 10.1002/oby.22369
45. O’Connor EA, Evans CV, Burda BU, Walsh ES, Eder M, Lozano P. Screening for Obesity and Intervention for Weight Management in Children and Adolescents Evidence Report and Systematic Review for the US Preventive Services Task Force. JAMA (2017) 317(23):2427–44. doi: 10.1001/jama.2017.0332
46. Attia SL, Softic S, Mouzaki M. Evolving Role for Pharmacotherapy in NAFLD/NASH. Clin Transl Sci (2021) 14(1):11–19. doi: 10.1111/cts.12839
47. Friesen CS, Chan SS, Wagner JB, Hosey-Cojocari C, Csanaky IL, Shakhnovich V. Critical Need For Pharmacologic Treatment Options In NAFLD: A Pediatric Perspective. Clin Transl Sci (2020) 00:1–3. doi: 10.1111/cts.12952
48. Athyros VG, Tziomalos K, Gossios TD, Griva T, Anagnosstis P, Kargiotis K, et al. Safety and efficacy of long-term statin treatment for cardiovascular events in patients with coronary heart disease and abnormal liver tests in the Greek Atorvastatin and Coronary Heart Disease Evaluation (GREACE) Study: a post-hoc analysis. Lancet (2010) 376(9756):1916–22. doi: 10.1016/S0140-6736(10)61272-X
49. Tikkanen MJ, Fayyad R, Faergeman O, Olsson AG, Wun C, Laskey R, et al. Effect of intensive lipid lowering with atorvastatin on cardiovascular outcomes in coronary heart disease patients with mild-to-moderate baseline elevations in alanine aminotransferase levels. Int J Cardiol (2013) 168(4):3846–52. doi: 10.1016/j.ijcard.2013.06.024
50. Pastori D, Polimeni L, Baratta F, Pani A, Del Ben M, Angelico F. The efficacy and safety of statins for the treatment of nonalcoholic fatty liver disease. Dig Liver Dis (2015) 47(1):4–11. doi: 10.1016/j.dld.2014.07.170
51. Lavine JE, Schwimmer J, Van Natta ML. Effect of Vitamin E or Metformin for Treatment of Nonalcoholic Fatty Liver Disease in Children and Adolescents: The TONIC Randomized Controlled Trial. JAMA (2011) 305:1659–68. doi: 10.1001/jama.2011.520
52. Janczyk W, Lebensztein D, Wierzbicka-Rucinka A, Mazur A, Neuhoff-Murawska J, Matusik P, et al. Omega-3 Fatty acids therapy in children with nonalcoholic Fatty liver disease: a randomized controlled trial. J Pediatr (2015) 166(6):1358–63.e1-3. doi: 10.1016/j.jpeds.2015.01.056
53. Boyraz M, Pirgon Ö, Dündar B, Çekmez F, Hatipoğlu N. Long-Term Treatment with n-3 Polyunsaturated Fatty Acids as a Monotherapy in Children with Nonalcoholic Fatty Liver Disease. J Clin Res Pediatr Endocrinol (2015) 7(2):121–7. doi: 10.4274/jcrpe.1749
54. Pan CS, Stanley TL. Effect of Weight Loss Medications on Hepatic Steatosis and Steatohepatitis: A Systematic Review. Front Endocrinol (2020) 11:70. doi: 10.3389/fendo.2020.00070
55. Norgren S, Danielsson P, Jurold R, Lotborn M, Marcus C. Orlistat treatment in obese prepubertal children: a pilot study. Acta Paediatr (2003) 92(6):666–70. doi: 10.1111/j.1651-2227.2003.tb00596.x
56. Mitchel EB, Lavine JE. Review article: the management of paediatric nonalcoholic fatty liver disease. Aliment Pharmacol Ther (2014) 40(10):1155–70. doi: 10.1111/apt.12972
57. Mantovani A, Petracca G, Beatrice G, Csermely A, Lonardo A, Targher G. Glucagon-Like Peptide-1 Receptor Agonists for Treatment of Nonalcoholic Fatty Liver Disease and Nonalcoholic Steatohepatitis: An Updated Meta-Analysis of Randomized Controlled Trials. Metabolites (2021) 11(2):73. doi: 10.3390/metabo11020073
58. Scheen AJ. Beneficial effects of SGLT2 inhibitors on fatty liver in type 2 diabetes: A common comorbidity associated with severe complications. Diabetes Metab (2019) 45(3):213–23. doi: 10.1016/j.diabet.2019.01.008
59. Nadeau KJ, Ehlers LB, Zeitler PS, Love-Osborne K. Treatment of Non-Alcoholic Fatty Liver Disease with Metformin versus Lifestyle Intervention in Insulin-Resistant Adolescents. Pediatr Diabetes (2009) 10(1):5–13. doi: 10.1111/j.1399-5448.2008.00450.x
60. Schwimmer JB, Middleton MS, Deutsch R, Lavine JE. A phase 2 clinical trial of metformin as a treatment for non-diabetic paediatric non-alcoholic steatohepatitis. Aliment Pharmacol Ther (2005) 21(7):871–9. doi: 10.1111/j.1365-2036.2005.02420.x
61. Flisiak-Jackiewicz M, Lebensztejn DM. Update on pathogenesis, diagnostics and therapy of nonalcoholic fatty liver disease in children. Clin Exp Hepatol (2019) 5:11–21. doi: 10.5114/ceh.2019.83152
62. Miller MH, Walsh SV, Atrih A, Huang JT, Ferguson MA, Dillon JF. Serum proteome of nonalcoholic fatty liver disease: A multimodal approach to discovery of biomarkers of nonalcoholic steatohepatitis. J Gastroenterol Hepatol (2014) 29:1839–47. doi: 10.1111/jgh.12614
63. Heydari M, Cornide-Petronio ME, Jimenez-Castro MB, Peralta C. Data on adiponectin from 2010 to 2020: Therapeutic target and prognostic factor for liver diseases? Int J Mol Sci (2020) 21(15):5242. doi: 10.3390/ijms21155242
64. Klusek-Oksiuta M, Bialokoz-Kalinowska I, Tarasow E, Wojtkowska M, Werpachowska I, Lebensztejn DM. Chemerin as a novel non-invasive serum marker of intrahepatic lipid content in obese children. Ital J Pediatr (2014) 40:84. doi: 10.1186/s13052-014-0084-4
65. Mohamed AA, Sabry S, Abdallah AM, Elazeem NAA, Refaey D, Algebaly HAF, et al. Circulating adipokines in children with nonalcoholic fatty liver disease: Possible noninvasive diagnostic markers. Ann Gastroenterol (2017) 30:457–63. doi: 10.20524/aog.2017.0148
66. Polyzos SA, Aronis KN, Kountouras J, Raptis DD, Vasiloglou MF, Mantzoros CS. Circulating leptin in non-alcoholic fatty liver disease: A systematic review and meta-analysis. Diabetologia (2016) 59:30–43. doi: 10.1007/s00125-015-3769-3
67. Senates E, Colak Y, Yesil A, Coskunpinar E, Sahin O, Kahraman OT, et al. Circulating resistin is elevated in patients with non-alcoholic fatty liver disease and is associated with steatosis, portal inflammation, insulin resistance and nonalcoholic steatohepatitis scores. Minerva Med (2012) 103:369–76.
68. Johannsen K, Flechtner-Mors M, Kratzer W, Koenig W, Boehm BO, Schmidberger J, et al. Association between visfatin and hepatic steatosis in the general population during long-term follow-up. Horm Metab Res (2019) 51:602–7. doi: 10.1055/a-0897-8565
69. Elkabany ZA, Hamza RT, Ismail EAR, Elsharkawy A, Yosry A, Musa S, et al. Serum visfatin level as a noninvasive marker for nonalcoholic fatty liver disease in children and adolescents with obesity: Relation to transient elastography with controlled attenuation parameter. Eur J Gastroenterol Hepatol (2020) 32:1008–16. doi: 10.1097/MEG.0000000000001608
70. Angin Y, Arslan N, Kuralay F. Leptin-to-adiponectin ratio in obese adolescents with nonalcoholic fatty liver disease. Turk J Pediatr (2014) 56:259–66.
71. Polyzos SA, Kountouras J, Anastasilakis AD, Geladari EV, Mantzoros CS. Irisin in patients with nonalcoholic fatty liver disease. Metabolism (2014) 63:207–17. doi: 10.1016/j.metabol.2013.09.013
72. Hu J, Ke Y, Wu F, Liu S, Ji C, Zhu X, et al. Circulating irisin levels in patients with nonalcoholic fatty liver disease: A systematic review and meta-analysis. Gastroenterol Res Pract (2020) 2020:8818191. doi: 10.1155/2020/8818191
73. Reinehr T, Roth CL. Fetuin-a and its relation to metabolic syndrome and fatty liver disease in obese children before and after weight loss. J Clin Endocrinol Metab (2008) 93:4479–85. doi: 10.1210/jc.2008-1505
74. Haukeland JW, Dahl TB, Yndestad A, Gladhaug IP, Loberg EM, Haaland T, et al. Fetuin a in nonalcoholic fatty liver disease: In vivo and in vitro studies. Eur J Endocrinol (2012) 166:503–10. doi: 10.1530/EJE-11-0864
75. Li H, Fang Q, Gao F, Fan J, Zhou J, Wang X, et al. Fibroblast growth factor 21 levels are increased in nonalcoholic fatty liver disease patients and are correlated with hepatic triglyceride. J Hepatol (2010) 53:934–40. doi: 10.1016/j.jhep.2010.05.018
76. Reinehr T, Woelfle J, Wunsch R, Roth CL. Fibroblast growth factor 21 (fgf-21) and its relation to obesity, metabolic syndrome, and nonalcoholic fatty liver in children: A longitudinal analysis. J Clin Endocrinol Metab (2012) 97:2143–50. doi: 10.1210/jc.2012-1221
77. Flisiak-Jackiewicz M, Bobrus-Chociej A, Wasilewska N, Tarasow E, Wojtkowska M, Lebensztejn DM. Can hepatokines be regarded as novel non-invasive serum biomarkers of intrahepatic lipid content in obese children? Adv Med Sci (2019) 64:280–84. doi: 10.1016/j.advms.2019.02.005
78. Choi HY, Hwang SY, Lee CH, Hong HC, Yang SJ, Yoo HJ, et al. Increased selenoprotein p levels in subjects with visceral obesity and nonalcoholic fatty liver disease. Diabetes Metab J (2013) 37:63–71. doi: 10.4093/dmj.2013.37.1.63
79. Polyzos SA, Kountouras J, Tsatsoulis A, Zafeiriadou E, Katsiki E, Patsiaoura K, et al. Sex steroids and sex hormone-binding globulin in postmenopausal women with nonalcoholic fatty liver disease. Hormones (Athens) (2013) 12:405–16. doi: 10.1007/BF03401306
80. Lan F, Misu H, Chikamoto K, Takayama H, Kikuchi A, Mohri K, et al. Lect2 functions as a hepatokine that links obesity to skeletal muscle insulin resistance. Diabetes (2014) 63:1649–64. doi: 10.2337/db13-0728
81. Yoo HJ, Hwang SY, Choi JH, Lee HJ, Chung HS, Seo JA, et al. Association of leukocyte cell-derived chemotaxin 2 (lect2) with nafld, metabolic syndrome, and atherosclerosis. PloS One (2017) 12:e0174717. doi: 10.1371/journal.pone.0174717
82. Walenbergh SM, Houben T, Hendrikx T, Jeurissen ML, van Gorp PJ, Vreugdenhil AC, et al. Plasma cathepsin d levels: A novel tool to predict pediatric hepatic inflammation. Am J Gastroenterol (2015) 110:462–70. doi: 10.1038/ajg.2015.29
83. Sayin O, Tokgoz Y, Arslan N. Investigation of adropin and leptin levels in pediatric obesity-related nonalcoholic fatty liver disease. J Pediatr Endocrinol Metab (2014) 27:479–84. doi: 10.1515/jpem-2013-0296
84. Romanowska A, Lebensztejn DM, Skiba E, Tarasow E, Kaczmarski M. Retinol binding protein-4 as a serum biomarker of intrahepatic lipid content in obese children–preliminary report. Acta Biochim Pol (2011) 58:35–8. doi: 10.18388/abp.2011_2282
85. Manco M, Marcellini M, Giannone G, Nobili V. Correlation of serum tnf-alpha levels and histologic liver injury scores in pediatric nonalcoholic fatty liver disease. Am J Clin Pathol (2007) 127:954–60. doi: 10.1309/6VJ4DWGYDU0XYJ8Q
86. Jarrar MH, Baranova A, Collantes R, Ranard B, Stepanova M, Bennett C, et al. Adipokines and cytokines in non-alcoholic fatty liver disease. Aliment Pharmacol Ther (2008) 27:412–21. doi: 10.1111/j.1365-2036.2007.03586.x
87. Pacifico L, Bonci E, Marandola L, Romaggioli S, Bascetta S, Chiesa C. Increased circulating zonulin in children with biopsy-proven nonalcoholic fatty liver disease. World J Gastroenterol (2014) 20:17107–14. doi: 10.3748/wjg.v20.i45.17107
88. Hendy OM, Elsabaawy MM, Aref MM, Khalaf FM, Oda AMA, El Shazly HM. Evaluation of circulating zonulin as a potential marker in the pathogenesis of nonalcoholic fatty liver disease. APMIS (2017) 125:607–13. doi: 10.1111/apm.12696
89. Flisiak-Jackiewicz M, Bobrus-Chociej A, Tarasow E, Wojtkowska M, Bialokoz-Kalinowska I, Lebensztejn DM. Predictive role of interleukin-18 in liver steatosis in obese children. Can J Gastroenterol Hepatol (2018) 2018:3870454. doi: 10.1155/2018/3870454
90. Puri P, Daita K, Joyce A, Mirshahi F, Santhekadur PK, Cazanave S, et al. The presence and severity of nonalcoholic steatohepatitis is associated with specific changes in circulating bile acids. Hepatology (2018) 67:534–48. doi: 10.1002/hep.29359
91. Ferslew BC, Xie G, Johnston CK, Su M, Stewart PW, Jia W, et al. Altered bile acid metabolome in patients with nonalcoholic steatohepatitis. Dig Dis Sci (2015) 60:3318– 3328. doi: 10.1007/s10620-015-3776-8
92. Jahnel J, Zohrer E, Alisi A, Ferrari F, Ceccarelli S, De Vito R, et al. Serum bile acid levels in children with nonalcoholic fatty liver disease. J Pediatr Gastroenterol Nutr (2015) 61:85–90. doi: 10.1097/MPG.0000000000000774
93. Lu LP, Wan YP, Xun PC, Zhou KJ, Chen C, Cheng SY, et al. Serum bile acid level and fatty acid composition in chinese children with nonalcoholic fatty liver disease. J Dig Dis (2017) 18:461–71. doi: 10.1111/1751-2980.12494
94. Nobili V, Alisi A, Mosca A, Della Corte C, Veraldi S, De Vito R, et al. Hepatic farnesoid x receptor protein level and circulating fibroblast growth factor 19 concentration in children with nafld. Liver Int (2018) 38:342–9. doi: 10.1111/liv.13531
95. Schwimmer JB, Middleton MS, Begling C, Newton KP, Awai HI, Paiz MN, et al. Magnetic resonance imaging and liver histology as biomarkers of hepatic steatosis in children with nonalcoholic fatty liver disease. Hepatology (2015) 61(6):1887–95. doi: 10.1002/hep.27666
96. Middleton MS, Van Natta ML, Heba ER, Alazraki A, Trout AT, Masand P, et al. Diagnostic Accuracy of Magnetic Resonance Imaging. Hepatic Proton Density Fat Fraction in Pediatric Nonalcoholic Fatty Liver Disease. Hepatology (2018) 67(3):858–72. doi: 10.1002/hep.29596
97. Expert Panel on Gastrointestinal Imaging, Horowitz JM, Arif-Tiwari H, Asranis SK, Hindman NM, Kaur H, et al. ACR Appropriateness Criteria® Chronic Liver Disease. J Am Coll Radiol (2017) 14(5S):S103–17. doi: 10.1016/j.jacr.2017.02.011
98. Tchelepi H, Ralls PW, Radin R, Grant E. Sonography of diffuse liver disease. J Ultrasound Med (2002) 21(9):1023–32. doi: 10.7863/jum.2002.21.9.1023
99. Kim DW, Park C, Yoon HM, Jung AY, Lee JS, Jung SC, et al. Technical performance of shear wave elastography for measuring liver stiffness in pediatric and adolescent patients: a systematic review and meta-analysis. Eur Radiol (2019) 29(5):2560–72. doi: 10.1007/s00330-018-5900-6
100. United States Food and Drug Administration. (2018). Available at: https://www.fda.gov/media/119044/download (Accessed December 1, 2020).
Keywords: NAFLD, pediatric, weight losing effect, pharmacotherapy, pediatric trials, pediatric NAFLD, weight loss efficacy
Citation: Friesen CS, Hosey-Cojocari C, Chan SS, Csanaky IL, Wagner JB, Sweeney BR, Friesen A, Fraser JD and Shakhnovich V (2021) Efficacy of Weight Reduction on Pediatric Nonalcoholic Fatty Liver Disease: Opportunities to Improve Treatment Outcomes Through Pharmacotherapy. Front. Endocrinol. 12:663351. doi: 10.3389/fendo.2021.663351
Received: 02 February 2021; Accepted: 25 March 2021;
Published: 13 April 2021.
Edited by:
Luca Busetto, Università degli Studi di Padova, ItalyReviewed by:
Mikiko Watanabe, Sapienza University of Rome, ItalyVibha Singhal, Massachusetts General Hospital and Harvard Medical School, United States
Copyright © 2021 Friesen, Hosey-Cojocari, Chan, Csanaky, Wagner, Sweeney, Friesen, Fraser and Shakhnovich. This is an open-access article distributed under the terms of the Creative Commons Attribution License (CC BY). The use, distribution or reproduction in other forums is permitted, provided the original author(s) and the copyright owner(s) are credited and that the original publication in this journal is cited, in accordance with accepted academic practice. No use, distribution or reproduction is permitted which does not comply with these terms.
*Correspondence: Valentina Shakhnovich, vshakhnovich@cmh.edu