- 1Department of Biochemistry, Asahikawa Medical University, Hokkaido, Japan
- 2Department of Biological Sciences, Graduate School of Science and Technology, Kumamoto University, Kumamoto, Japan
- 3Freshwater Resources Research Center, Aichi Fisheries Research Institute, Aichi, Japan
- 4Department of Pharmacology, Asahikawa Medical University, Hokkaido, Japan
- 5Noto Marine Laboratory, Institute of Nature and Environmental Technology, Division of Marine Environmental Studies, Kanazawa University, Ishikawa, Japan
- 6Department of Obstetrics-Gynecology, University of Fukui, Fukui, Japan
- 7Department of Nephrology, University of Fukui, Fukui, Japan
- 8Center for Animal Disease Control, University of Miyazaki, Miyazaki, Japan
- 9Molecular Genetics, Institute of Life Sciences, Kurume University, Fukuoka, Japan
- 10ASKA Pharma Medical Co., Ltd., Kanagawa, Japan
- 11Department of Pediatrics, Asahikawa Medical University, Hokkaido, Japan
- 12Department of Pharmacy, University of Rajshahi, Rajshahi, Bangladesh
- 13Department of Reproduction, National Research Institute for Child Health and Development, Tokyo, Japan
Although 11-ketotestosterone (11KT) and testosterone (T) are major androgens in both teleosts and humans, their 5α-reduced derivatives produced by steroid 5α-reductase (SRD5A/srd5a), i.e., 11-ketodihydrotestosterone (11KDHT) and 5α-dihydrotestosterone (DHT), remains poorly characterized, especially in teleosts. In this study, we compared the presence and production of DHT and 11KDHT in Japanese eels and humans. Plasma 11KT concentrations were similar in both male and female eels, whereas T levels were much higher in females. In accordance with the levels of their precursors, 11KDHT levels did not show sexual dimorphism, whereas DHT levels were much higher in females. It is noteworthy that plasma DHT levels in female eels were higher than those in men. In addition, plasma 11KDHT was undetectable in both sexes in humans, despite the presence of 11KT. Three srd5a genes (srd5a1, srd5a2a and srd5a2b) were cloned from eel gonads. All three srd5a genes were expressed in the ovary, whereas only both srd5a2 genes were expressed in the testis. Human SRD5A1 was expressed in testis, ovary and adrenal, whereas SRD5A2 was expressed only in testis. Human SRD5A1, SRD5A2 and both eel srd5a2 isoforms catalyzed the conversion of T and 11KT into DHT and 11KDHT, respectively, whereas only eel srd5a1 converted T into DHT. DHT and 11KDHT activated eel androgen receptor (ar)α-mediated transactivation as similar fashion to T and 11KT. In contrast, human AR and eel arβ were activated by DHT and11KDHT more strongly than T and 11KT. These results indicate that in teleosts, DHT and 11KDHT may be important 5α-reduced androgens produced in the gonads. In contrast, DHT is the only major 5α-reduced androgens in healthy humans.
Introduction
Androgens are sex steroid hormones that play a role in various physiological processes via pathways involving the androgen receptor (AR) (1). Testosterone (T) is the most important androgen in various animal species. It is produced from cholesterol in a series of steps by cytochrome P450 hydroxylases and hydroxysteroid dehydrogenases (HSDs) (Figure 1) (2, 3). Although T is able to strongly activate AR-mediated transactivation, it is converted into a more potent androgen, 5α-dihydrotestosterone (DHT), by steroid 5α-reductases (SRD5A) in steroidogenic tissues and peripheral tissues. Among the five human SRD5A genes, SRD5A1 and SRD5A2 play important roles in DHT production in gonads and peripheral tissues. SRD5A2 is strongly expressed in male tissues, including prostate and epididymis for producing DHT during development (4). Therefore, mutations of the SRD5A2 gene cause 46, XY disorders of sex development, resulting from low DHT production (5–8). SRD5A1 mainly plays roles in the production of neurosteroids involved in anxiety and sexual behavior (4). It also has activity in conversion of T into DHT, although to a lesser extent than that of SRD5A2 (9). In fact, Srd5a1 KO mice showed the abnormalities in these behaviors (10–12), and also partial feminization of the male skeleton (13).
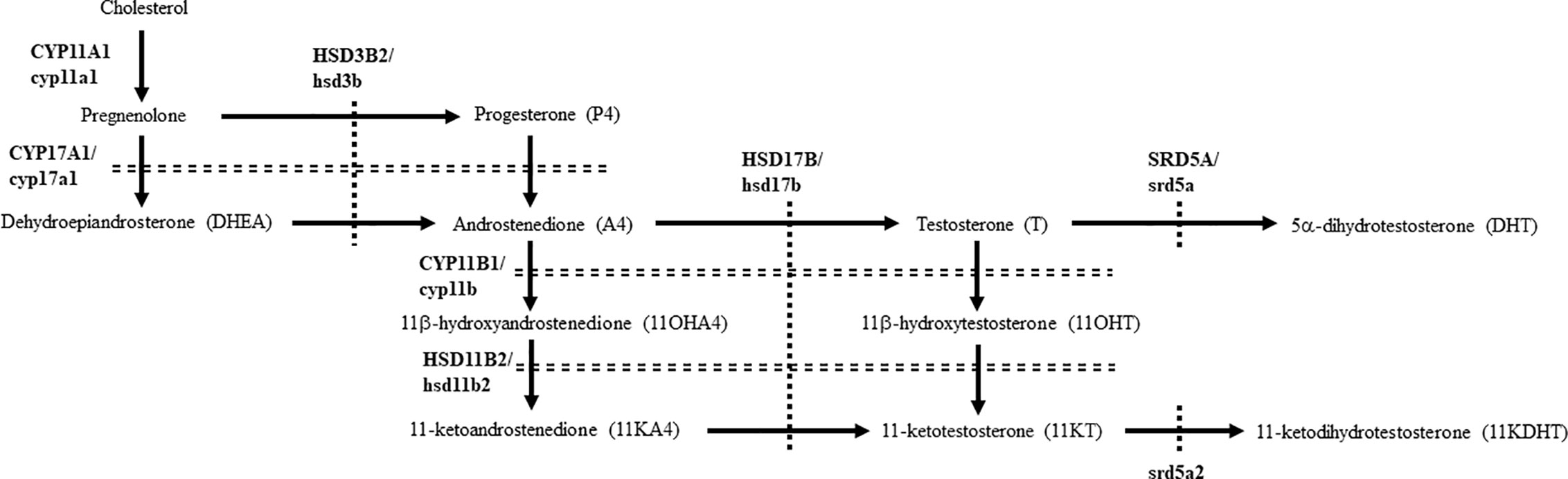
Figure 1 Pathways for producing 11-oxygenated androgens under physiological conditions in humans and teleosts. Human steroidogenic enzymes are indicated by capital letters, whereas teleost counterparts are indicated by small letters.
In teleosts, 11-ketotestosterone (11KT), member of the 11-oxygenated class of androgens, is a major active androgen mainly produced in gonads (14, 15). It is involved in sex differentiation (16), spermatogenesis (17, 18), and oocyte growth (19–21). Although 11KT was regarded as a teleost-specific androgen, it is also a major androgens in some mammals, such as humans, non-human primates, pigs and guinea pigs (22–25). Although 11KT strongly activates both mammalian and teleostan AR/ar (22, 23, 26–29), it is able to be converted into the even more active form, 11-ketodihydrotestosterone (11KDHT), by SRD5A1 and SRD5A2 (30). 11KDHT is produced in prostate cancer cells (30, 31), although it is unclear whether it is also produced in healthy humans. Furthermore, although srd5a genes are present in various teleost species (4, 32, 33), details of DHT and 11KDHT production remained unclear in most species. In this study, we compared the profiles, biosynthesis and functions of DHT and 11KDHT in humans and eels, as representatives of mammals and teleosts that possess abundant classical androgens and 11-oxygenated androgens in both sexes (18, 22–24, 34–36).
Materials and Methods
Human and Eel Blood Samples
The research protocol using human materials was approved at the ethical committee of Asahikawa Medical University and University of Fukui. Blood samples were collected in collection tubes containing heparin from the median cubital vein of 2 healthy women volunteers (obtaining informed consent) at University of Fukui Hospital in 2007. Plasma was separated by centrifugation at 1000g for 5 minutes. Other plasma samples were purchased from AllCells (Alameda, CA, USA) and ProMedDX (Norton, MA, USA). All plasma samples were collected under IRB-approved collection protocols and subject informed consent. The donors were 5 men (aged 32.2 ± 5.5 y) and 5 women (aged 29.2 ± 6.3y).
Animal experiments were performed using protocols approved by the Animal Care and Use Committee of Kumamoto University (Approval Number: A2020-014). All experiments were performed in accordance with the relevant guidelines and regulations. The sexes of juvenile eels were artificially directed by feeding a commercial diet supplemented with or without E2 (37). Male and female eels (>250g body weight) were accumulated for a month to artificial sea water. After accumulation, sexual maturation was induced by the administration of recombinant luteinizing hormone and the crude extracts of commercial salmon pituitary to male and female eels, respectively, as described (38, 39). Blood samples were collected from the animals using a heparinized syringes and needles, and plasma was separated by centrifuging the blood at 1000g for 5 minutes.
Cell Culture, Transfection and Luciferase Assay
HEK293 and CV-1 cells were cultured in DMEM supplemented with 10% fetal bovine serum (FBS) in a humidified atmosphere containing 5% CO2/95% air at 37 °C. Hepa-E1 cells were cultured in E-RDF with 5% FBS at 28 °C. Cells were dispensed into 24-well plates at 5 × 104 cells per well 24 h before transfection. HEK293 and CV-1 cells were transfected using HilyMax (Dojindo Laboratories, Kumamoto, Japan) according to the manufacturer’s instructions. Hepa-E1 cells were transfected using Lipofectamine LTX (Thermo Fisher Scientific, Waltham, MA, USA) according to the manufacturer’s instructions. At 24 h or 2 days post-transfection, the cells were treated with vehicle (EtOH) or androgens. Luciferase activity was determined using a dual luciferase reporter assay system (29, 40). Measurements were made using a MiniLumat LB9506 (Berthold Systems, Aliquippa, PA, USA) in a single tube, with the first assay involving the firefly luciferase, followed by the Renilla luciferase assay. Firefly luciferase activities (relative light units) were normalized by Renilla luciferase activities. Each data point represents the mean of at least three independent experiments.
Reverse Transcriptase-Polymerase Chain Reaction (RT-PCR) and Quantitative PCR (qPCR)
The cDNA from various human tissues were synthesized as described (26, 41). Total RNA from eel tissues was extracted using TRIsure reagent (Bioline, Luckenwalde, Germany). RT-PCR and qPCR were performed as described (40, 42, 43). The cDNA was synthesized from total RNA of each tissue using SuperScript III Reverse Transcriptase (Thermo Fisher Scientific). The reaction products of the RT-PCR assay were subjected to electrophoresis in a 1.25% agar gel, and the resulting bands were visualized by staining with ethidium bromide. In qPCR, each gene was measured via real-time PCR using the LightCycler 480 (Roche Diagnostics, Mannheim, Germany). β-actin (human) and ef1 [eel (44)] were used as the reference genes. Each reaction was conducted in duplicate. As a negative control, template cDNA was replaced by PCR grade water. Relative gene expression levels were determined by using the delta-delta Ct method. The primers used for PCR are described in Supplementary Table 1 and Supplementary Figure 2.
Cloning of Eel Srd5a cDNAs and Phylogenetic Analysis
Putative nucleotide sequences encoding eel srd5a1, srd5a2a and srd5a2b were provided from the database, JPEEL2016 (http://molas.iis.sinica.edu.tw/jpeel2016/). Cloning of ORF sequences were performed by PCR-based methods using ovary and testis cDNAs.
The alignment analysis of SRD5A/srd5a sequences was performed using Clustal W. The neighbor-joining phylogenetic tree was constructed using MEGA version X. Analyzed proteins and their accession numbers are as follows: human SRD5A1 (NP_001038.1), human SRD5A2 (ABQ59050.1), mouse Srd5a2 (NP_444418.1), chicken Srd5a2 (XP_001235447.1), Japanese quail Srd5a1 (XP_015709859.1), Japanese quail Srd5a2 (XP_015713214.1), three-toed box turtle Srd5a1 (XP_024056462.1), three-toed box turtle Srd5a2 (XP_026505351.1), zebrafish srd5a1 (AAI64429.1), zebrafish srd5a2 (XP_005157051.1), Atlantic salmon srda5a1 (XP_014033435.1), Atlantic salmon srd5a2 (NP_001134686.1), rainbow trout srd5a1 (XP_021428075.1). rainbow trout srd5a2 (XP_021413035.1) and elephant shark srd5a1 (NP_001279361.1).
Plasmids
The pQCXIP expressing human SRD5A1, human SRD5A2, eel srd5a1, eel srd5a2a and eel srd5a2b were generated by cloning the open reading frame of each gene into a pQCXIP vector (Invitrogen). The pcDNA3 expressing eel arα and arβ were generated by cloning the open reading frame of each gene into a pcDNA vector (Invitrogen, Carlsbad, CA, USA). A Slp-ARU/Luc reporter, pQCXIP/green fluorescent protein (GFP) and pQCXIP/human AR were prepared as described (29).
Measurements by Liquid Chromatography-Tandem Mass Spectrometry (LC-MS/MS)
Quantification of T, 11-KT, DHT and 11-KDHT in plasma and culture media by LC-MS/MS are based on methods as described [(29), ASKA Pharma Medical Co., Ltd., Kanagawa, Japan]. The lower detection limits of each androgen were 0.25 pg/ml. As internal standards, 11KT-d3, T-13C3, DHT-13C3 and 11KDHT-d3 were added to a medium which was diluted with distilled water. The steroids were extracted with methyl tert-butyl ether (MTBE). After the MTBE layer was evaporated to dryness, the extract was dissolved in 0.5 mL of methanol and diluted with 1 ml of distilled water. The sample was applied to OASIS MAX cartridge which had been successively conditioned with 3 ml of methanol and 3 ml of distilled water. After the cartridge was washed with 1 ml of distilled water, 1 ml of methanol/distilled water/acetic acid (45:55:1,v/v/v), and 1 ml of 1% pyridine solution, the steroids were eluted with 1 ml of methanol/pyridine (100:1,v/v). After evaporation, the residue was reacted with 50 μl of mixed solution (80 mg of 2-methyl-6-nitrobenzoic anhydride, 20 mg of 4-dimethylaminopyridine, 40 mg of picolinic acid and 10 μl of triethylamine in 1 ml of acetonitrile) for 30 min at room temperature. After the reaction, the sample was dissolved in 0.5 ml of ethyl acetate/hexane/acetic acid (15:35:1, v/v/v) and the mixture was applied to HyperSep Silica cartridge (Thermo Fisher Scientific) which had been successively conditioned with 3 mL of acetone and 3 ml of hexane. The cartridge was washed with 1 mL of hexane, and 2 mL of ethyl acetate/hexane (3:7, v/v). T, DHT, 11-KDHT and 11-KT were eluted with 2.5 ml of acetone/hexane (7:3, v/v). After evaporation, the residue was dissolved in 0.1 ml of acetonitrile/distilled water (2:3, v/v) and the solution was subjected to a LC-MS/MS.
Statistical Analysis
Data are presented as the mean ± SEM. Differences between groups (P< 0.05) were assessed by the Student’s t-test, one-way ANOVA followed by Tukey’s multiple comparison tests and two-way ANOVA followed by Tukey’s multiple comparison tests using SigmaPlot 14 (Systat Software Inc., CA, USA) and EZR (Saitama Medical Center, Jichi Medical University, Saitama, Japan) which is a graphical user interface for R (The R Foundation for Statistical Computing, Vienna, Austria) as described (40).
Results
Comparison of DHT and 11-KDHT Levels in Eel and Human Plasma
We measured the plasma concentrations of 11-KT, T, DHT and 11-KDHT both in eels and humans (Figure 2). In eels, 11-KT levels were similar in the two sexes, whereas the T level was much higher level in females (about 175-fold). Consistent with the results of their precursors, 11KDHT levels were similar between the two sexes, whereas DHT was at much higher concentration in females (about 15-fold). Interestingly, the DHT level in female eels was higher than in human males (Figure 2B). As previously reported (45), human plasma T and DHT levels were much higher in males than in females. In humans, plasma 11KT was present in both sexes, whereas 11KDHT was undetectable in both. These observations suggest that although DHT is an androgen common to both eels and humans, 11KDHT is a teleost-specific androgen. Furthermore, the sexual dimorphism of plasma DHT and T levels is reversed between humans and eels.
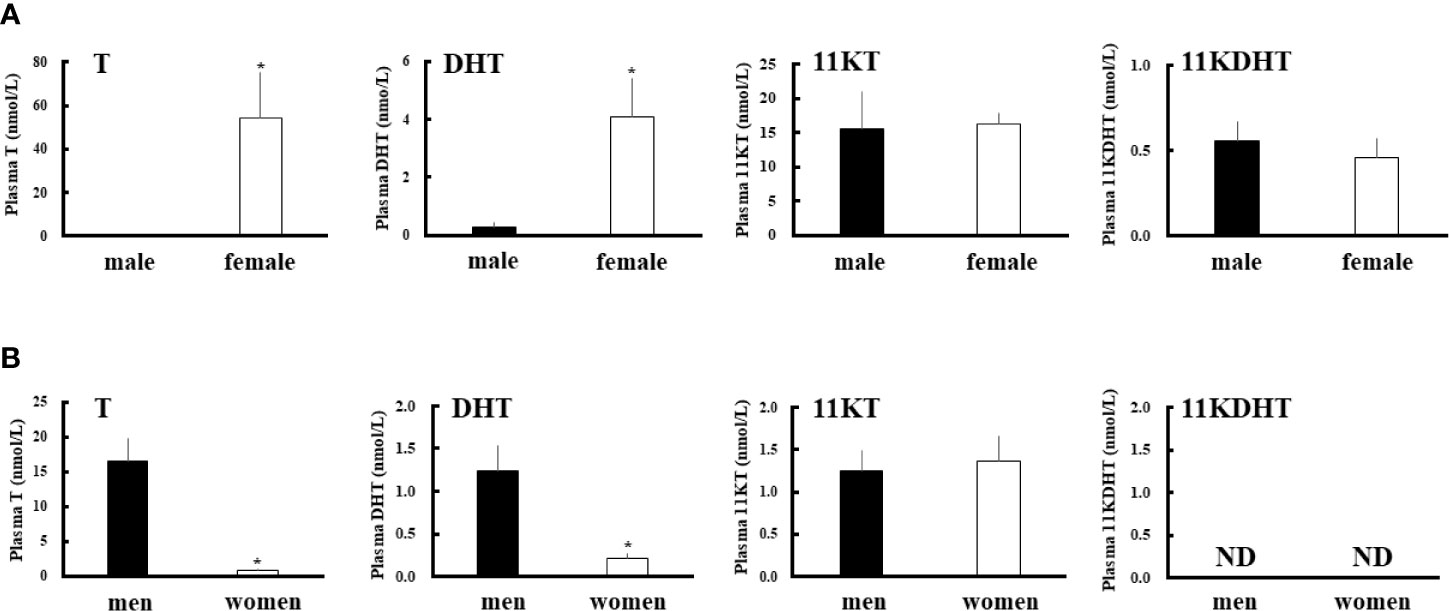
Figure 2 Plasma androgens levels in eels (A) and humans (B). Plasma T, DHT, 11-KT and 11-KDHT levels in each sex were measured by LC-MS/MS. Data represent the mean ± SEM (n =4 for eels and n=5 for humans, for each sex). *P < 0.05 vs male eels or men.
Comparison of Expression and Enzymatic Activities of Eel Srd5a and Human SRD5A Genes
To reveal DHT and 11KDHT biosynthesis pathways from T and 11KT in teleost, respectively, eel srd5a genes were cloned from testis and ovary cDNA templates by RT-PCR. A single isoform srd5a1 and two isoforms of srd5a2 (srd5a2a and srd5a2b) genes were isolated in eel (Supplementary Figure 1, Figure 3A). Eel srd5a1 cDNA (DDBJ accession number: LC602244) comprised an open reading frame (ORF) of 798 bp encoding 265 amino acids (aa) (Supplementary Figure 1A, Figure 3A). It shared approximately 50% and 40% aa identities with human SRD5A1 and SRD5A2/Srd5a2, respectively (Figure 3B). Eel srd5a2a (DDBJ accession number: LC602245) and srd5a2b (DDBJ accession number: LC602246) cDNAs comprised ORFs of 762 and 756 bp encoding 253 and 251 aa, respectively (Supplementary Figures 1B, C, Figure 3A). These isoforms shared comparatively high similarities (66.9%) with each other. On the other hand, srd5a2a and srd5a2b shared 50% and 40% aa identities with human SRD5A2 and SRD5A1/srd5a1, respectively. Alignments of these proteins revealed the conservation of an aa sequence with the C-terminus (Figure 3A). Phylogenetic analysis showed that each of the srd5a genes was included in the corresponding cluster of vertebrate SRD5A/Srd5a/srd5a genes (Figure 3C). The analysis also suggested that among the two srd5a2 isoforms, srd5a2b probably represents an ancestral form of vertebrate SRD5A2/Srd5a2/srd5a2.
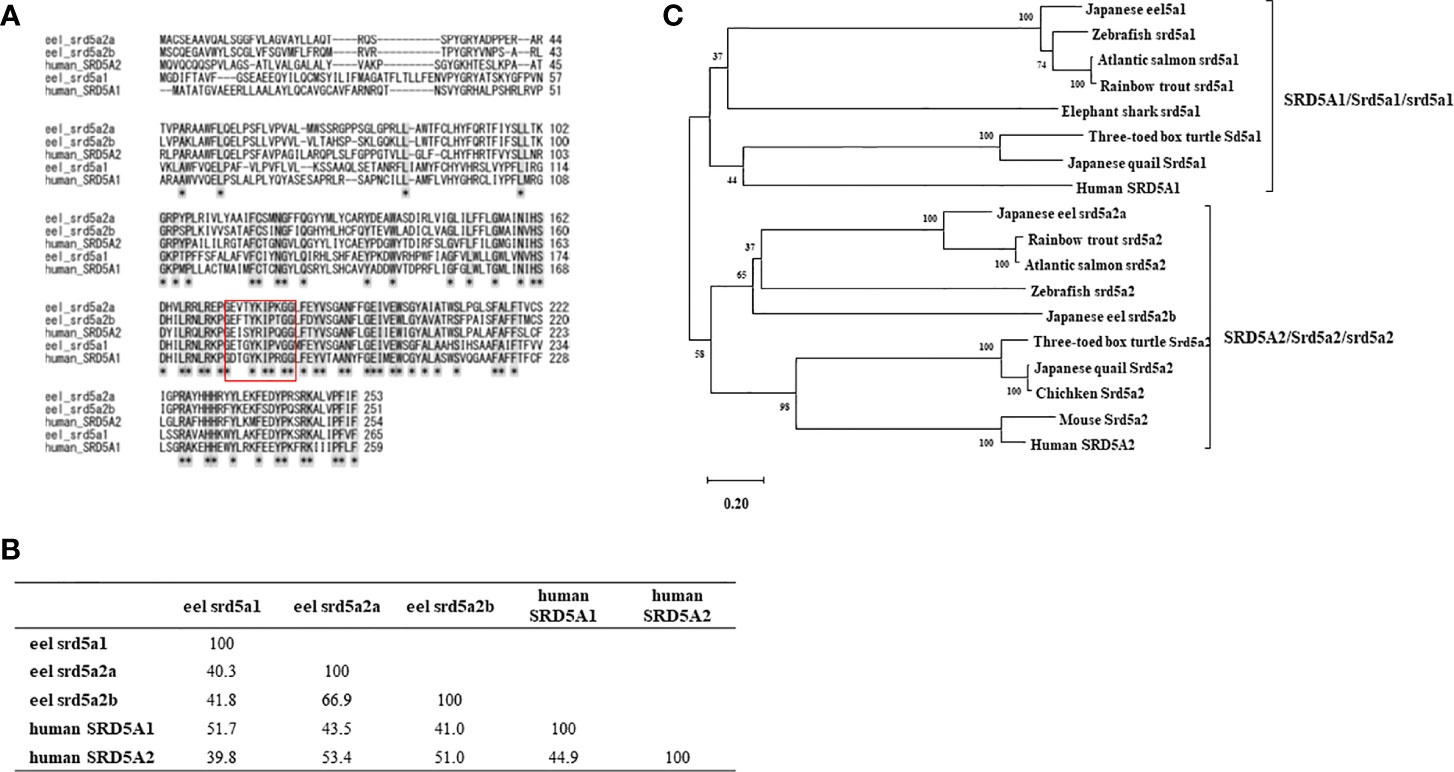
Figure 3 Alignment (A) and identities of amino acid sequence (B), phylogenic analyses (C) for eel srd5a and human SRD5A. (A) Alignment of the deduced SRD5A/srd5a amino acid sequences of eel and human. Conserved NADPH-binding domain (GXXGXXXXXGG) is shown by a box. (B) Comparisons of deduced amino acid identities between eel srd5a1, eel srd5a2a, eel srd5a2b, human SRD5A1 and human SRD5A2. (C) The phylogenetic tree of SRD5A/Srd5a/srd5a proteins. Bootstrap values (100 resamplings) are indicated by numbers.
Tissue expression analyses of mRNA by qPCR revealed that eel srd5a1 was expressed in liver and ovary, but not in testis (Figure 4A). Both srd5a2 isoforms were expressed in liver, testis and ovary, although ovarian srd5a2b expression levels were significantly higher than testicular levels. Human SRD5A1 was expressed in all examined tissues including the primary steroidogenic tissues, testis, ovary and adrenal (Figure 4B). In contrast SRD5A2 was expressed only in liver, prostate and testis (Figure 4B).
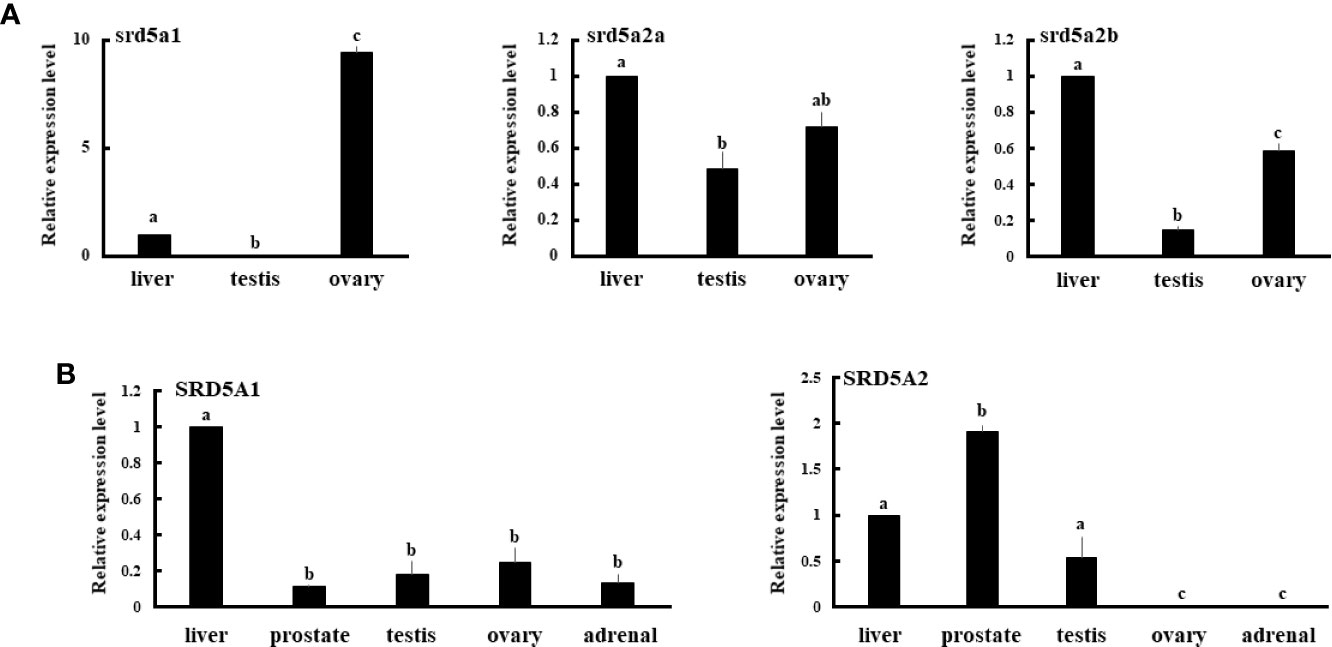
Figure 4 Expression of eel srd5a genes (A) and human SRD5A genes (B) in various tissues. mRNA expression of each gene in each tissue was analyzed by qPCR and normalized to ef-1 (A) or β-actin (B) expression. Data represent the mean ± SEM of at least three independent samples. Values marked by different letters are significantly different (P< 0.05).
To investigate the enzymatic activities of SRD5A/srd5a isoforms for conversion of T and 11KT to DHT and 11KDHT, respectively, expression vectors of GFP and each of SRD5A/srd5a genes were transfected in HEK293 cells. Then, T or 11KT was added to the culture medium at 10-9 M for 3h. All eel srd5a and human SRD5A isoforms catalyzed the conversion of T into DHT (Figure 5A). In the eel, those activities were significantly higher in both srd5a2 isoforms than in srd5a1. Conversion of 11KT into 11KDHT was catalyzed by both eel srd5a2 isoforms and human SRD5A (Figure 5B). Eel srd5a2a and human SRD5A2 had significant higher activities than eel srd5a2b and human SRD5A1, respectively. In contrast, eel srd5a1 showed no activity for conversion 11KT to 11KDHT.
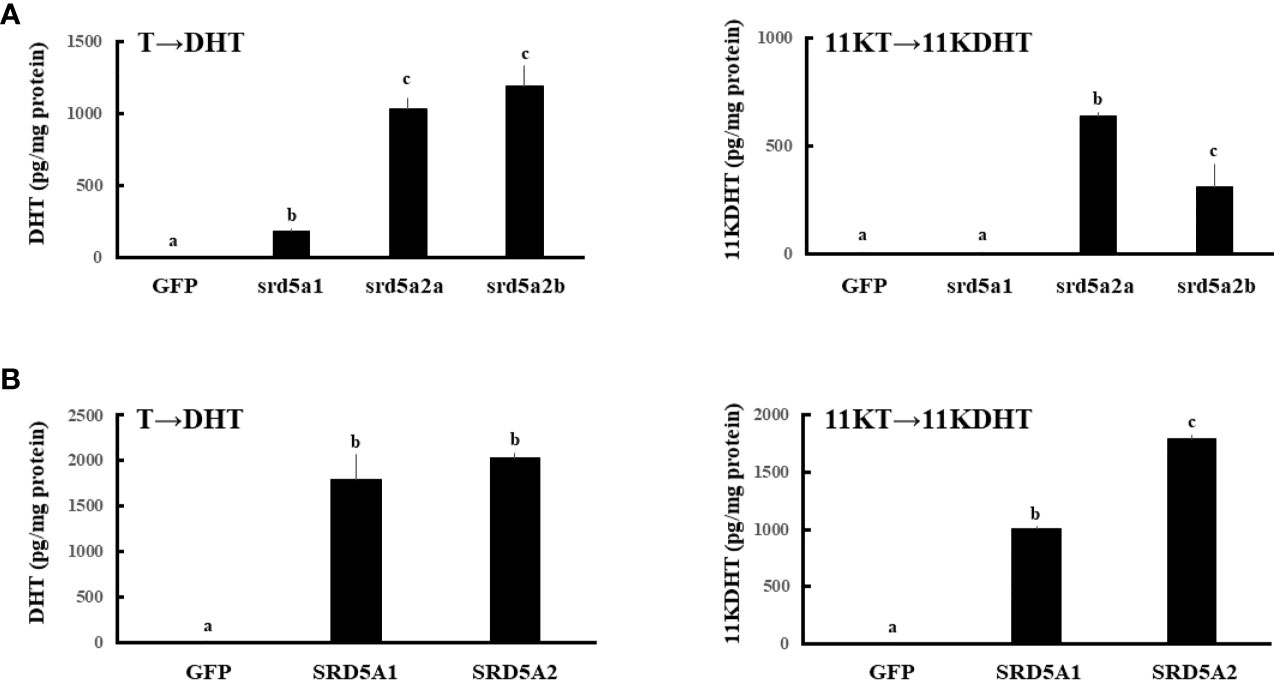
Figure 5 Evaluation of the enzymatic activities of eel srd5a (A) and human SRD5A (B). Expression vectors of each gene were transfected, and 24h after transfection, cells were incubated with T (1 nM) or 11KT (1 nM) for 3h. Concentrations of DHT and 11KDHT in culture media were analyzed by LC-MS/MS. Each column represents the mean ± SEM (n =3 for each group) of three independent experiments. Values marked by different letters are significantly different (P< 0.05).
Effects of DHT and 11-KDHT on AR/ar-Mediated Transactivation
To investigate the effects of 5α-reduced androgens on eel ars (arα and arβ) and mammalian AR, we compared the androgen-dependent transcriptional activities of DHT and 11KDHT with the activities of T and 11KT using the luciferase reporter system in fish and mammalian cell lines (Figure 6). DHT and 11KDHT at >10-8 M activated eel arα-mediated transactivation in a similar manner to that of T and 11KT (Figure 6A). DHT and 11KDHT at >10-8 M also activated eel arβ, although to a greater extent than T and 11KT (Figure 6A). Human AR-mediated transactivation was increased by DHT and 11KDHT above 10-10 M in a concentration-dependent manner (Figure 6B). Both were stronger activators of human AR than T and 11KT, paralleling the response of eel arβ. These results indicate that 5α-reduced androgens strongly activate AR/ars-mediated transactivation in both humans and teleosts.
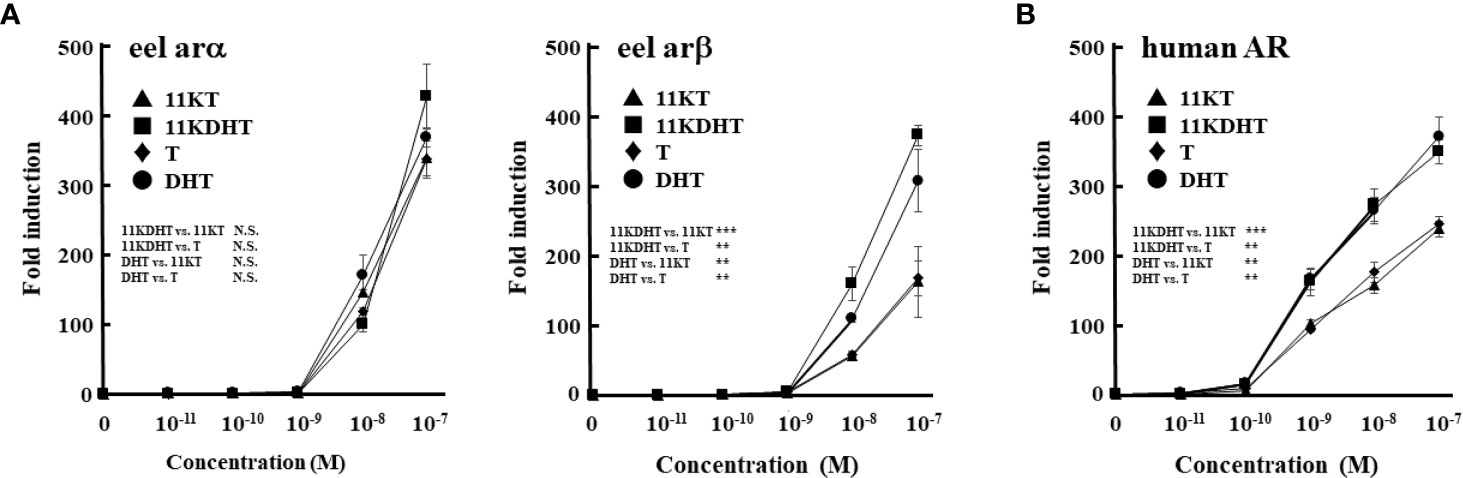
Figure 6 Eel ars (A) and human AR (B)-mediated transactivation by each androgen in Hepa-E1 cells and CV-1 cells, respectively. Hepa-E1 cells and CV-1 cells were transfected with the ARE-Luc vector and each ar/AR-expression vector. At 24 h post-transfection, cells were incubated with or without increasing concentrations of 11KT, 11KDHT, T and DHT for 24 h. Data represent the mean ± sem of at least three independent experiments. Results of two-way ANOVA (the additives and their concentrations as factors) followed by multiple comparison tests are given (N.S. P≧0.05; **P < 0.01; ***P < 0.001).
Discussion
This study demonstrated that DHT and 11KDHT are potential important androgens in eels. In contrast, 11KDHT was undetectable in healthy human plasma, although DHT was present.
DHT plays very important roles in mammalian sex differentiation as the most potent androgen (4). Mutations of the SRD5A2 gene cause 46, XY disorders of sex development, resulting from low DHT production (4, 8). In contrast, little attention has been received in the existence and functions of DHT in fish, because it is thought to be relatively biologically inactive. Therefore, it is unexpectable that plasma DHT levels in eels were higher than those in men. In contrast to humans and other mammals, it was the female-dominated androgen in eels. These differences probably reflect the opposite sexual dimorphism of T levels, as a precursor to DHT. This result is consistent with a previous report that plasma T concentrations of wild females become higher than those of males during silvering, prepubertal stage in eels (46). T is the predominant male androgen in mammals, whereas plasma profiles of T are species-specific among teleosts (35); T is often the predominant androgen both males and females. Therefore, it is possible that the profiles of plasma DHT differ among teleost species. Until now, male-dominant androgens are never reported at least in sexually matured eels. T and DHT are necessary for regulating various male physiological functions in humans during long reproductive age ensuing puberty (1). In contrast, male eels are considered to die after their first spawning following the sexual maturation (47). Therefore, androgens like T and DHT in humans might be unnecessary in maturated male eels. This hypothesis might be supported by the facts that plasma T levels in females are abundant in the salmons [also die after their first spawning (48)]. In contrast to DHT, 11KDHT levels did not show any sexual differences. This might also result from the absence of sexual dimorphism of precursor (11KT) levels. 11-oxygenated androgens levels including 11KT are very low in most female teleosts, except in eels, sturgeon, salmonids and mullet (35). Therefore, 11KDHT could be the dominant male androgen in most teleosts. Regardless of the differences in sexual plasma profiles, DHT and 11KDHT strongly activate various teleost ars [Figure 6 (49, 50)], which suggest that DHT and 11KDHT are important androgens in teleost species. Future study should investigate the plasma profiles of these 5α-reduced androgens in many teleost species. In contrast to eels, 11KDHT is not present in healthy human plasma, even though its precursor 11KT exists. This is likely owing to the deficiency of SRD5A2 expression (main converting enzyme from 11KT to 11KDHT) in the adrenal glands, which are the main source of 11-oxygenated androgens in humans (36). However, 11KDHT is detectable in prostate tissues and plasma samples of prostate cancer patients (31). Thus, the possibility that 11KDHT functions in humans under the pathological conditions should not be ruled out.
In addition to a single SRD5A1 homolog, there are two SRD5A2 homologs in eels, which showed relatively similar activities for production of 5α-reduced androgens. These srd5a2 paralogs perhaps occurred by the teleost-specific genome duplication. Although such srd5a2 paralogs in some species are registered in databases, their prevalence and general importance among Teleostei are unclear. In the future study, it is necessary to investigate the presence of srd5a2 paralogs in teleosts. Consistent with our results, previous studies reported that human SRD5A2 showed higher activities than SRD5A1 in converting T and 11KT into DHT and 11KDHT, respectively (9, 30). Such difference between SRD5A/Srd5a/srd5a isoforms is probably conserved during evolution, despite eel srd5a1 being completely inactive in converting 11KT to 11KDHT. In addition to studying the profiles of 5α-reduced androgens, it would be interesting to evaluate the activities of SRD5A/Srd5a/srd5a homologs in various animal species to reveal the transitions of these androgens during evolution.
The 5α-reduced androgens, DHT and 11KDHT more strongly activated human AR and eel arβ than T and 11KT did, whereas such remarkable differences were not detected for eel arα. In teleosts, the two ar genes have been formed by duplication of an ancestral AR gene during a teleost specific genome duplication event. Based on the phylogenic analyses, Ogino and colleagues proposed that teleost arβ preserves the ancestral AR functions, whereas arα has acquired new properties during more rapid evolution (28). Our results are consistent with this hypothesis; i.e. eel arα has lost its preference for the 5α-reduced androgens. In human, DHT is essential for various physiological phenomena as a stronger activator of AR than T, despite plasma concentrations of DHT are much lower than those of T (4). Therefore, it is possible that in eel, DHT and 11KDHT are especially important in arβ-expressing tissues, including testis and ovary (47, 49, 51). In contrast, previous studies have suggested that 11KT is the predominant androgen activating teleost ars (28, 52, 53). Although DHT activates almost teleost ars, the activation is often reported to be weaker than with 11KT, including the case of eel arβ (49). Such discrepancy reflects the different cell lines used in those studies. Previous studies have used mammalian cell lines. In the preset study, we used Japanese eel hepatocyte-derived Hepa-E1 cells for measuring the eel ar-mediated transactivation. In support of the above hypothesis, activation of eel ars by each of the androgens was very low (less than 10-fold relative to vehicle groups) in CV-1 cells even at 10-7 M (data not shown), whereas it was over several hundred-fold higher in Hepa-E1 cells, at levels similar to that of human AR in CV-1 cells. A similar phenomenon was noted in measurements of flounder estrogen receptor-mediated transactivation by estrogens and estrogenic compounds (54). Because most teleost transcription factors are strongly activated in reporter assays using mammalian cells, sex steroid receptors might be an exception in poorly activated in mammalian cells. Future studies should compare the effects of T, 11KT, DHT and 11KDHT on teleost ar-mediated transactivation using fish cell lines.
In summary, we demonstrated that DHT and 11KDHT are potent endogenous androgens in fish. The properties of these androgens could provide useful insights into elucidating ambiguous ar-mediated phenomena reported in fish. In contrast, 11KDHT is undetectable in healthy humans. Nevertheless, it has been often reported that the profiles of 11-oxygenated androgens are markedly changed under pathological conditions (36, 55–59). It is important to investigate the presence of 11KDHT in these androgen-dependent diseases to seek novel targets for therapy.
Data Availability Statement
‘The raw data supporting the conclusions of this article will be made available by the authors, without undue reservation.
Ethics Statement
The studies involving human participants were reviewed and approved by The ethical committee of Asahikawa Medical University and University of Fukui. The patients/participants provided their written informed consent to participate in this study. The animal study was reviewed and approved by The Animal Care and Use Committee of Kumamoto University.
Author Contributions
TY, YI, TSe, JU, ST, NS, AU and TK obtained funding and designed the study. TY, HI, YI, TSe, JU, MI, MO, DM, TI, TSa, YM and TK performed the experiments and collected the data. TY, HI, YI, MK and TK wrote the manuscript. All authors contributed to the article and approved the submitted version.
Conflict of Interest
Author YM was employed by the company ASKA Pharmaceutical Medical Co., Ltd.
The remaining authors declare that the research was conducted in the absence of any commercial or financial relationships that could be construed as a potential conflict of interest.
Acknowledgments
We also thank Dr. T. Taniguchi and Ms. S. Tsunoda for administrative assistance. This study was performed under the cooperative research program of Institute of Nature and Environmental Technology, Kanazawa University (Accept No.20003) in 2020. This work was supported in part by JSPS Grant Number 19K09794 (Grant-in-Aid for Scientific Research (C)) and 19K07117 (Grant-in-Aid for Scientific Research (C)) granted by Japan Society for the Promotion of Science, the Smoking Research Foundation of Japan (2020G006) and by the Grant of National Center for Child Health and Development (29-1). We thank Harry Taylor, Ph D., from Edanz Group (http://en-author-services.edanz.com/ac) for editing a draft of this manuscript.
Supplementary Material
The Supplementary Material for this article can be found online at: https://www.frontiersin.org/articles/10.3389/fendo.2021.657360/full#supplementary-material
References
1. Mooradian AD, Morley JE, Korenman SG. Biological actions of androgens. Endocr Rev (1987) 8:1–28. doi: 10.1210/edrv-8-1-1
2. Miller WL, Auchus RJ. The molecular biology, biochemistry, and physiology of human steroidogenesis and its disorders. Endocr Rev (2011) 32:81–151. doi: 10.1210/er.2010-0013
3. Miller WL. Steroidogenesis: Unanswered Questions. Trends Endocrinol Metab (2017) 28:771–93. doi: 10.1016/j.tem.2017.09.002
4. Robitaille J, Langlois VS. Consequences of steroid-5α-reductase deficiency and inhibition in vertebrates. Gen Comp Endocrinol (2020) 290:113400. doi: 10.1016/j.ygcen.2020.113400
5. Andersson S, Berman DM, Jenkins EP, Russell DW. Deletion of steroid 5 alpha-reductase 2 gene in male pseudohermaphroditism. Nature (1991) 354:159–61. doi: 10.1038/354159a0
6. Okeigwe I, Kuohung W. 5-Alpha reductase deficiency: a 40-year retrospective review. Curr Opin Endocrinol Diabetes Obes (2014) 21:483–7. doi: 10.1097/med.0000000000000116
7. Mendonca BB, Batista RL, Domenice S, Costa EM, Arnhold IJ, Russell DW, et al. Steroid 5α-reductase 2 deficiency. J Steroid Biochem Mol Biol (2016) 163:206–11. doi: 10.1016/j.jsbmb.2016.05.020
8. Batista RL, Mendonca BB. Integrative and Analytical Review of the 5-Alpha-Reductase Type 2 Deficiency Worldwide. Appl Clin Genet (2020) 13:83–96. doi: 10.2147/tacg.s198178
9. Barnard L, Nikolaou N, Louw C, Schiffer L, Gibson H, Gilligan LC, et al. The A-ring reduction of 11-ketotestosterone is efficiently catalysed by AKR1D1 and SRD5A2 but not SRD5A1. J Steroid Biochem Mol Biol (2020) 202:105724. doi: 10.1016/j.jsbmb.2020.105724
10. Koonce CJ, Frye CA. Female mice with deletion of Type One 5α-reductase have reduced reproductive responding during proestrus and after hormone-priming. Pharmacol Biochem Behav (2014) 122:20–9. doi: 10.1016/j.pbb.2014.03.010
11. Ford MM, Nickel JD, Kaufman MN, Finn DA. Null mutation of 5α-reductase type I gene alters ethanol consumption patterns in a sex-dependent manner. Behav Genet (2015) 45:341–53. doi: 10.1007/s10519-014-9694-2
12. Tanchuck-Nipper MA, Ford MM, Hertzberg A, Beadles-Bohling A, Cozzoli DK, Finn DA. Sex Differences in Ethanol’s Anxiolytic Effect and Chronic Ethanol Withdrawal Severity in Mice with a Null Mutation of the 5α-Reductase Type 1 Gene. Behav Genet (2015) 45:354–67. doi: 10.1007/s10519-014-9691-5
13. Windahl SH, Andersson N, Börjesson AE, Swanson C, Svensson J, Movérare-Skrtic S, et al. Reduced bone mass and muscle strength in male 5α-reductase type 1 inactivated mice. PLoS One (2011) 6:e21402. doi: 10.1371/journal.pone.0021402
14. Tokarz J, Möller G, Hrabě de Angelis M, Adamski J. Steroids in teleost fishes: A functional point of view. Steroids (2015) 103:123–44. doi: 10.1016/j.steroids.2015.06.011
15. Rajakumar A, Senthilkumaran B. Steroidogenesis and its regulation in teleost-a review. Fish Physiol Biochem (2020) 46:803–18. doi: 10.1007/s10695-019-00752-0
16. Golshan M, Alavi SMH. Androgen signaling in male fishes: Examples of anti-androgenic chemicals that cause reproductive disorders. Theriogenology (2019) 139:58–71. doi: 10.1016/j.theriogenology.2019.07.020
17. Miura T, Yamauchi K, Takahashi H, Nagahama Y. Hormonal induction of all stages of spermatogenesis in vitro in the male Japanese eel (Anguilla japonica). Proc Natl Acad Sci U S A (1991) 88:5774–8. doi: 10.1073/pnas.88.13.5774
18. Nagahama Y, Miura T, Kobayashi T. The onset of spermatogenesis in fish. Ciba Found Symp (1994) 182:255–67; discussion 267-70. doi: 10.1002/9780470514573.ch14
19. Kazeto Y, Tosaka R, Matsubara H, Ijiri S, Adachi S. Ovarian steroidogenesis and the role of sex steroid hormones on ovarian growth and maturation of the Japanese eel. J Steroid Biochem Mol Biol (2011) 127:149–54. doi: 10.1016/j.jsbmb.2011.03.013
20. Tuan Nguyen A, Chia JHZ, Kazeto Y, Wylie MJ, Mark Lokman P. Induction of oocyte development in previtellogenic eel, Anguilla australis. Gen Comp Endocrinol (2020) 291:113404. doi: 10.1016/j.ygcen.2020.113404
21. Wang W, Zhu H, Tian Z, Sun A, Dong Y, Dong T, et al. Effects of 11-Ketotestosterone on Development of the Previtellogenic Ovary in the Sterlet, Acipenser ruthenus. Front Endocrinol (Lausanne) (2020) 11:115. doi: 10.3389/fendo.2020.00115
22. Rege J, Nakamura Y, Satoh F, Morimoto R, Kennedy MR, Layman LC, et al. Liquid chromatography-tandem mass spectrometry analysis of human adrenal vein 19-carbon steroids before and after ACTH stimulation. J Clin Endocrinol Metab (2013) 98:1182–8. doi: 10.1210/jc.2012-2912
23. Imamichi Y, Yuhki KI, Orisaka M, Kitano T, Mukai K, Ushikubi F, et al. 11-Ketotestosterone Is a Major Androgen Produced in Human Gonads. J Clin Endocrinol Metab (2016) 101:3582–91. doi: 10.1210/jc.2016-2311
24. Rege J, Garber S, Conley AJ, Elsey RM, Turcu AF, Auchus RJ, et al. Circulating 11-oxygenated androgens across species. J Steroid Biochem Mol Biol (2019) 190:242–9. doi: 10.1016/j.jsbmb.2019.04.005
25. Davio A, Woolcock H, Nanba AT, Rege J, O’Day P, Ren J, et al. Sex Differences in 11-Oxygenated Androgen Patterns Across Adulthood. J Clin Endocrinol Metab (2020) 105:e2921–9. doi: 10.1210/clinem/dgaa343
26. Yazawa T, Mizutani T, Yamada K, Kawata H, Sekiguchi T, Yoshino M, et al. Differentiation of adult stem cells derived from bone marrow stroma into Leydig or adrenocortical cells. Endocrinology (2006) 147:4104–11. doi: 10.1210/en.2006-0162
27. Ogino Y, Katoh H, Kuraku S, Yamada G. Evolutionary history and functional characterization of androgen receptor genes in jawed vertebrates. Endocrinology (2009) 150:5415–27. doi: 10.1210/en.2009-0523
28. Ogino Y, Kuraku S, Ishibashi H, Miyakawa H, Sumiya E, Miyagawa S, et al. Neofunctionalization of Androgen Receptor by Gain-of-Function Mutations in Teleost Fish Lineage. Mol Biol Evol (2016) 33:228–44. doi: 10.1093/molbev/msv218
29. Yazawa T, Imamichi Y, Uwada J, Sekiguchi T, Mikami D, Kitano T, et al. Evaluation of 17β-hydroxysteroid dehydrogenase activity using androgen receptor-mediated transactivation. J Steroid Biochem Mol Biol (2020) 196:105493. doi: 10.1016/j.jsbmb.2019.105493
30. Storbeck KH, Bloem LM, Africander D, Schloms L, Swart P, Swart AC. 11β-Hydroxydihydrotestosterone and 11-ketodihydrotestosterone, novel C19 steroids with androgenic activity: a putative role in castration resistant prostate cancer? Mol Cell Endocrinol (2013) 377:135–46. doi: 10.1016/j.mce.2013.07.006
31. du Toit T, Bloem LM, Quanson JL, Ehlers R, Serafin AM, Swart AC. Profiling adrenal 11β-hydroxyandrostenedione metabolites in prostate cancer cells, tissue and plasma: UPC(2)-MS/MS quantification of 11β-hydroxytestosterone, 11keto-testosterone and 11keto-dihydrotestosterone. J Steroid Biochem Mol Biol (2017) 166:54–67. doi: 10.1016/j.jsbmb.2016.06.009
32. Martyniuk CJ, Bissegger S, Langlois VS. Current perspectives on the androgen 5 alpha-dihydrotestosterone (DHT) and 5 alpha-reductases in teleost fishes and amphibians. Gen Comp Endocrinol (2013) 194:264–74. doi: 10.1016/j.ygcen.2013.09.019
33. García-García M, Sánchez-Hernández M, García-Hernández MP, García-Ayala A, Chaves-Pozo E. Role of 5α-dihydrotestosterone in testicular development of gilthead seabream following finasteride administration. J Steroid Biochem Mol Biol (2017) 174:48–55. doi: 10.1016/j.jsbmb.2017.07.024
34. Miura T, Yamauchi K, Takahashi H, Nagahama Y. Human chorionic gonadotropin induces all stages of spermatogenesis in vitro in the male Japanese eel (Anguilla japonica). Dev Biol (1991) 146:258–62. doi: 10.1016/0012-1606(91)90468-i
35. Lokman PM, Harris B, Kusakabe M, Kime DE, Schulz RW, Adachi S, et al. 11-Oxygenated androgens in female teleosts: prevalence, abundance, and life history implications. Gen Comp Endocrinol (2002) 129:1–12. doi: 10.1016/s0016-6480(02)00562-2
36. Turcu AF, Rege J, Auchus RJ, Rainey WE. 11-Oxygenated androgens in health and disease. Nat Rev Endocrinol (2020) 16:284–96. doi: 10.1038/s41574-020-0336-x
37. Inaba H, Hara S, Horiuchi M, Ijiri S, Kitano T. Gonadal expression profiles of sex-specific genes during early sexual differentiation in Japanese eel, Anguilla japonica. Fisheries Sci (2021) 87:203–9. doi: 10.1007/s12562-020-01491-5
38. Ohta H, Sato H, Imaizumi Y, Kazeto Y. Changes in milt volume and sperm quality with time after an injection of recombinant Japanese eel luteinizing hormone in male Japanese eels. Aquaculture (2017) 479:150–4. doi: 10.1016/j.aquaculture.2017.05.044
39. Horiuchi M, Izumi H, Lokman PM, Ijiri S, Adachi S. Relationship between abundance and lozalization of maternal messenger RNA and eg quality in artficailly matured Japanese eel, Anguilla japonica. Fisheries Sci (2020) 86:43–56. doi: 10.1007/s12562-019-01377-1
40. Yazawa T, Imamichi Y, Yuhki KI, Uwada J, Mikami D, Shimada M, et al. Cyclooxygenase-2 is acutely induced by CCAAT/enhancer-binding protein β to produce prostaglandin E (2) and F (2α) following gonadotropin stimulation in Leydig cells. Mol Reprod Dev (2019) 86:786–97. doi: 10.1002/mrd.23163
41. Yazawa T, Inaoka Y, Okada R, Mizutani T, Yamazaki Y, Usami Y, et al. PPAR-gamma coactivator-1alpha regulates progesterone production in ovarian granulosa cells with SF-1 and LRH-1. Mol Endocrinol (2010) 24:485–96. doi: 10.1210/me.2009-0352
42. Imamichi Y, Sekiguchi T, Kitano T, Kajitani T, Okada R, Inaoka Y, et al. Diethylstilbestrol administration inhibits theca cell androgen and granulosa cell estrogen production in immature rat ovary. Sci Rep (2017) 7:8374. doi: 10.1038/s41598-017-08780-7
43. Yazawa T, Sato T, Nemoto T, Nagata S, Imamichi Y, Kitano T, et al. 11-Ketotestosterone is a Major Androgen Produced in Porcine Adrenal Glands and Testes. J Steroid Biochem Mol Biol (2021). doi: 10.1016/j.jsbmb.2021.105847
44. Jeng SR, Wu GC, Yueh WS, Kuo SF, Dufour S, Chang CF. Gonadal development and expression of sex-specific genes during sex differentiation in the Japanese eel. Gen Comp Endocrinol (2018) 257:74–85. doi: 10.1016/j.ygcen.2017.07.031
45. Shiraishi S, Lee PW, Leung A, Goh VH, Swerdloff RS, Wang C. Simultaneous measurement of serum testosterone and dihydrotestosterone by liquid chromatography-tandem mass spectrometry. Clin Chem (2008) 54:1855–63. doi: 10.1373/clinchem.2008.103846
46. Han YS, Liao IC, Tzeng WN, Huang YS, Yu JY. Serum estradiol-17beta and testosterone levels during silvering in wild Japanese eel Anguilla japonica. Comp Biochem Physiol B Biochem Mol Biol (2003) 136:913–20. doi: 10.1016/j.cbpc.2003.09.002
47. Jeng SR, Pasquier J, Yueh WS, Chen GR, Lee YH, Dufour S, et al. Differential regulation of the expression of cytochrome P450 aromatase, estrogen and androgen receptor subtypes in the brain-pituitary-ovarian axis of the Japanese eel (Anguilla japonica) reveals steroid dependent and independent mechanisms. Gen Comp Endocrinol (2012) 175:163–72. doi: 10.1016/j.ygcen.2011.11.005
48. Onuma T, Higashi Y, Ando H, Ban M, Ueda H, Urano A. Year-to-year differences in plasma levels of steroid hormones in pre-spawning chum salmon. Gen Comp Endocrinol (2003) 133:199–215. doi: 10.1016/s0016-6480(03)00171-0
49. Ikeuchi T, Todo T, Kobayashi T, Nagahama Y. cDNA cloning of a novel androgen receptor subtype. J Biol Chem (1999) 274:25205–9. doi: 10.1074/jbc.274.36.25205
50. Takeo J, Yamashita S. Rainbow trout androgen receptor-alpha fails to distinguish between any of the natural androgens tested in transactivation assay, not just 11-ketotestosterone and testosterone. Gen Comp Endocrinol (2000) 117:200–6. doi: 10.1006/gcen.1999.7398
51. Vílchez MC, Santangeli S, Maradonna F, Gioacchini G, Verdenelli C, Gallego V, et al. Effect of the probiotic Lactobacillus rhamnosus on the expression of genes involved in European eel spermatogenesis. Theriogenology (2015) 84:1321–31. doi: 10.1016/j.theriogenology.2015.07.011
52. Olsson PE, Berg AH, von Hofsten J, Grahn B, Hellqvist A, Larsson A, et al. Molecular cloning and characterization of a nuclear androgen receptor activated by 11-ketotestosterone. Reprod Biol Endocrinol (2005) 3:37. doi: 10.1186/1477-7827-3-37
53. Katsu Y, Hinago M, Sone K, Urushitani H, Guillette LJ Jr., Iguchi T. In vitro assessment of transcriptional activation of the estrogen and androgen receptors of mosquitofish, Gambusia affinis affinis. Mol Cell Endocrinol (2007) 276:10–7. doi: 10.1016/j.mce.2007.06.004
54. Kitano T, Koyanagi T, Adachi N, Sakimura K, Takamune K, Abe S. Assesment of estrogenic chemicals usig an estrogen receptor a-(ERa) and ERb-mediated reporter gene assay in fish. Marine Biol (2006) 149:49–55. doi: 10.1007/s00227-005-0206-z
55. Turcu AF, Nanba AT, Chomic R, Upadhyay SK, Giordano TJ, Shields JJ, et al. Adrenal-derived 11-oxygenated 19-carbon steroids are the dominant androgens in classic 21-hydroxylase deficiency. Eur J Endocrinol (2016) 174:601–9. doi: 10.1530/eje-15-1181
56. Pretorius E, Arlt W, Storbeck KH. A new dawn for androgens: Novel lessons from 11-oxygenated C19 steroids. Mol Cell Endocrinol (2017) 441:76–85. doi: 10.1016/j.mce.2016.08.014
57. Rege J, Turcu AF, Kasa-Vubu JZ, Lerario AM, Auchus GC, Auchus RJ, et al. 11-Ketotestosterone Is the Dominant Circulating Bioactive Androgen During Normal and Premature Adrenarche. J Clin Endocrinol Metab (2018) 103:4589–98. doi: 10.1210/jc.2018-00736
58. Yoshida T, Matsuzaki T, Miyado M, Saito K, Iwasa T, Matsubara Y, et al. 11-oxygenated C19 steroids as circulating androgens in women with polycystic ovary syndrome. Endocr J (2018) 65:979–90. doi: 10.1507/endocrj.EJ18-0212
Keywords: DHT, 11KDHT, androgen receptor, 5α-reductase, testosterone
Citation: Yazawa T, Inaba H, Imamichi Y, Sekiguchi T, Uwada J, Islam MS, Orisaka M, Mikami D, Ida T, Sato T, Miyashiro Y, Takahashi S, Khan MRI, Suzuki N, Umezawa A and Kitano T (2021) Profiles of 5α-Reduced Androgens in Humans and Eels: 5α-Dihydrotestosterone and 11-Ketodihydrotestosterone Are Active Androgens Produced in Eel Gonads. Front. Endocrinol. 12:657360. doi: 10.3389/fendo.2021.657360
Received: 22 January 2021; Accepted: 26 February 2021;
Published: 23 March 2021.
Edited by:
Honoo Satake, Suntory Foundation for Life Sciences, JapanCopyright © 2021 Yazawa, Inaba, Imamichi, Sekiguchi, Uwada, Islam, Orisaka, Mikami, Ida, Sato, Miyashiro, Takahashi, Khan, Suzuki, Umezawa and Kitano. This is an open-access article distributed under the terms of the Creative Commons Attribution License (CC BY). The use, distribution or reproduction in other forums is permitted, provided the original author(s) and the copyright owner(s) are credited and that the original publication in this journal is cited, in accordance with accepted academic practice. No use, distribution or reproduction is permitted which does not comply with these terms.
*Correspondence: Takashi Yazawa, eWF6YXdhQGFzYWhpa2F3YS1tZWQuYWMuanA=