- 1Department for Obesity, Reproductive and Metabolic Disorders, Clinic for Endocrinology, Diabetes and Metabolic Diseases, University Clinical Centre of Serbia, Faculty of Medicine, University of Belgrade, Belgrade, Serbia
- 2Department of Pulmonology, Clinical Hospital Centre Zemun, Faculty of Medicine, University of Belgrade, Belgrade, Serbia
- 3Institute of Medical Statistics and Informatics, Faculty of Medicine, University of Belgrade, Belgrade, Serbia
- 4Department of Endocrinology, Clinical Center of Montenegro, Podgorica, Montenegro
Background: Disrupted sleep affects cardio-metabolic and reproductive health. Obstructive sleep apnea syndrome represents a major complication of obesity and has been associated with gonadal axis activity changes and lower serum testosterone concentration in men. However, there is no consistent opinion on the effect of obstructive sleep apnea on testosterone levels in men.
Objective: The aim of this study was to determine the influence of obstructive sleep apnea on total and free testosterone levels in severely obese men.
Materials and methods: The study included 104 severely obese (Body Mass Index (BMI) ≥ 35 kg/m2) men, aged 20 to 60, who underwent anthropometric, blood pressure, fasting plasma glucose, lipid profile, and sex hormone measurements. All participants were subjected to polysomnography. According to apnea-hypopnea index (AHI) patients were divided into 3 groups: <15 (n = 20), 15 - 29.9 (n = 17) and ≥ 30 (n = 67).
Results: There was a significant difference between AHI groups in age (29.1 ± 7.2, 43.2 ± 13.2, 45.2 ± 10.2 years; p < 0.001), BMI (42.8 ± 5.9, 43.2 ± 5.9, 47.1 ± 7.8 kg/m2; p = 0.023), the prevalence of metabolic syndrome (MetS) (55%, 82.4%, 83.6%, p = 0.017), continuous metabolic syndrome score (siMS) (4.01 ± 1.21, 3.42 ± 0.80, 3.94 ± 1.81, 4.20 ± 1.07; p = 0.038), total testosterone (TT) (16.6 ± 6.1, 15.2 ± 5.3, 11.3 ± 4.44 nmol/l; p < 0.001) and free testosterone (FT) levels (440.4 ± 160.8, 389.6 ± 162.5, 294.5 ± 107.0 pmol/l; p < 0.001). TT level was in a significant negative correlation with AHI, oxygen desaturation index (ODI), BMI, MetS and siMS. Also, FT was in a significant negative correlation with AHI, ODI, BMI, age, MetS and siMS. The multiple regression analysis revealed that both AHI and ODI were in significant correlation with TT and FT after adjustment for age, BMI, siMS score and MetS components.
Conclusion: Obstructive sleep apnea is associated with low TT and FT levels in severely obese men.
Introduction
Obesity is a complex metabolic disorder with a markedly increased prevalence in both the developed and underdeveloped countries. Over the past four decades, the percentage of obese people has doubled among females and quadrupled among males (1, 2).
Excess body weight is a crucial risk factor for mortality and morbidity, especially in obese men with body mass index (BMI) over 35 kg/m2 (1, 3). The risk of developing male infertility increases with obesity severity. Alterations in sex steroid hormones contribute to infertility in obese men (4). Obesity in men is associated with low testosterone and low measured or calculated free and bioavailable testosterone (1). Men with a BMI of 35–40 kg/m2 can have up to 50% less free and total testosterone when compared to age-matched peers with a normal BMI (5). Low testosterone levels in obese men are considered a consequence of reduced sex hormone binding globulin (SHBG) synthesis, increased androgens aromatization to estradiol, and central gonadal axis suppression. Complex metabolic disorders, increased pro-inflammatory adipocytokines, impaired insulin signaling in the central nervous system, dysregulated leptin signaling, and increased estrogen may lead to hypothalamic suppression via effects on kisspeptin neurons in obese men (6, 7).
Furthermore, metabolic syndrome, as an adverse health consequence of obesity, is associated with lower testosterone levels independent of age and BMI (8, 9). Disrupted sleep affects cardio-metabolic and reproductive health. The most important clinical cause of disrupted sleep is obstructive sleep apnea syndrome (OSAS) (10). OSAS is emerging as a new area of interest for andrological issues (4). It is characterized by repetitive episodes of upper airway obstruction that occur during sleep and is associated with a complete (apnea) or incomplete (hypopnea) cessation of airflow. This is commonly accompanied by loud snoring and reduction in blood oxygen saturation, followed by arousal, fragmented sleep, and daytime sleepiness (11, 12). The most important epidemiological risk factor for sleep apnea is obesity, preceding age, and male gender (13). The prevalence of OSAS in obese individuals is over 30%, and 50-98% in morbidly obese patients (14). There is growing evidence to support an independent association of OSAS with cardiovascular, neuropsychiatric, pulmonary, and renal disorders as well as with metabolic and endocrine co-morbidities. There is convincing evidence that OSAS is also an independent risk factor for metabolic syndrome (15). It is clinically noticeable that obese men with sleep apnea have lower than expected concentrations of total testosterone (TT) and free testosterone (FT) (3, 9, 10). However, the independent effect of OSAS on blood testosterone concentrations has been shown in some (9, 16–20) but not in all cross-sectional studies (21, 22).
Considering the inconclusive data, the purpose of this study was to determine the influence of obstructive sleep apnea on TT and FT levels in severely obese men.
Materials and Methods
Subjects
This was an observational, cross-sectional study. We have evaluated 165 severely obese men (BMI ≥ 35kg/m2), aged 20 to 60, admitted to the Department for Obesity, Metabolic and Reproductive Disorders at the Clinic for Endocrinology, Diabetes and Metabolic Diseases University, Clinical Centre of Serbia between 2006 and 2016.
Detailed personal history, the biochemical and endocrinological evaluation was conducted in order to detect and exclude patients with: hypercortisolism (0) and/or hypothyroidism (5), history of alcohol consumption (≥ 2 units per day) or substance abuse (2), hormonal therapy (3), hypogonadism due to the pituitary (3) or testicular diseases (3), craniofacial abnormalities (2), liver or kidney diseases (2), neuromuscular diseases (4), chronic obstructive pulmonary disease (4), asthma (3), manifest cardiologic diseases (13), malignancies (2), psychiatric diseases (3) and missing data (12). In total, sixty-one patients were excluded from the study as provided in the STROBE flowchart (Figure 1).
This study was designed in agreement with the Declaration of Helsinki and was approved by the local Ethical Committee. The patients gave their informed consent.
Study Protocol
All study patients underwent anthropometric and blood pressure measurements, biochemical and hormonal analysis, as well as the overnight polysomnography study.
Anthropometry and Measurement of Blood Pressure
Weight was measured in the morning, without shoes, in light clothing, using a medical scale with an accuracy of 0.1 kg. Height was measured without shoes, using a stadiometer with an accuracy of 0.1 cm. Waist, hip, and neck circumferences expressed in centimeters were measured using a non-elastic band in the standing position. Waist circumference was measured at the end of the expiration at the midline between the lowest point of the costal arch and the highest point of the iliac crest. Hip circumference was measured at the level of the large trochanter of the femur (23). Neck circumference was measured at the level of the cricothyroid membrane (24). The systolic (SBP) and diastolic (DBP) blood pressure was measured using a standard sphygmomanometer in the sitting position: three values were obtained with a 2-minute time-lapse in between, and the average was recorded (23).
Blood Samples
Fasting blood samples were taken to measure glucose, triglyceride (Tg), high-density lipoprotein (HDL), albumin, luteinizing hormone (LH), follicle-stimulating hormones (FSH), estradiol, testosterone, and SHBG in the morning following polysomnography.
Sleep Study
The apnea-hypopnea index (AHI) and the oxygen desaturation index (ODI) were derived from nocturnal sleep studies using a seven-channel portable sleep recorder (Stardust II, Respironics, Inc., USA). The system detected apneas and hypopneas by measuring: pressure-based airflow with snoring detection, pulse rate, arterial oxyhemoglobin saturation, chest or abdominal effort, and body position changes. The sleep studies were scored at the hospital by a certified sleep specialist. Apneas were defined as a total cessation of airflow for at least 10s if a respiratory effort was present. Hypopneas were defined as a decrease in nasal pressure signal of ≥ 30% of baseline, which was associated with a ≥ 3% desaturation. The apnea-hypopnea index (AHI) was calculated as the total number of obstructive apneas and hypopneas per hour of sleep. OSAS was classified based on the AHI as follows: mild (≥ 5 and < 15 events/h), moderate (≥ 15 and < 30 events/h), or severe (≥ 30 events/h). The oxygen desaturation index (ODI) was defined as the number of oxygen desaturations ≥3% per hour of sleep (25).
Biochemical and Hormonal Assays
The fasting plasma glucose (FPG) levels were measured by the glucose-oxidase method (Beckman). Fasting serum lipid levels (HDL and Tg) were analyzed enzymatically using a commercial kit (Bushranger Mannheim GmbH Diagnostica). The serum LH (The ImmuChem hLH IRMA kit, ICN Biomedicals, Inc., CA, USA, CV 2.4%), FSH (The ImmuChem FSH- CT IRMA kit, ICN Biomedicals, Inc., CV 2.6%), estradiol (ESTR- US- CT Cisbio, Bioassays, CV 2.8%), testosterone (TESTO-CT2, Cisbio International, CV 3.1%), and SHBG (SHBG-RIACT, Cisbio International, France, CV 3.6%) were measured by radioimmunoassay.
Calculations
Metabolic syndrome (MetS) was defined by three of five criteria: FPG ≥ 5.6mmol/l or antidiabetic therapy, increased waist circumference equal to or greater than 94 cm, TG ≥ 1.7mmol/l, HDL < 1.0mmol/l or antilipidemic therapy, and blood pressure ≥ 130/85 mmHg or therapy (26).
To evaluate the metabolic syndrome, we used siMS score - continuous metabolic syndrome score for quantification of patients’ metabolic status. siMS score (siMS) was calculated using the following formula: siMS score = 2*Waist/Height + FPG/5.6 + Tg/1.7 + SBP/130—HDL/1.02 (27).
FT was calculated based on TT, SHBG, and albumin with the formula as reported by Vermeulen et al. (28). TT < 11 nmol/l and FT < 220 pmol/l were deemed low (29).
Statistical Analysis
Results are presented as count (%), mean ± standard deviation, or median (25th-75th percentile) depending on the data type and distribution. Groups were compared with parametric (ANOVA) and nonparametric (Kruskal-Wallis test, Mantel-Haenszel chi-square test for trend) tests. To test the correlation between the variables, Pearson and Spearman’s correlation were used. Multiple linear regression analysis was performed to evaluate the relationship between the dependent variable and independent variables. All p values below 0.05 were considered significant. All data were analyzed using SPSS 20.0 (IBM Corp. Released 2011. IBM SPSS Statistics for Windows, Version 20.0. Armonk, NY: IBM Corp.).
Results
The general characteristics of the study subjects are summarized in Table 1. The mean age was 41.8 ± 11.9 years, the mean BMI was 45.7 ± 7.4 kg/m2, and the mean weight was 144.9 ± 24.2 kg. 77.9% of patients had MetS, 48.1% had low concentrations of the TT, and 29.8% had low concentrations of FT. The prevalence of sleep apnea was 96.2%, out of which 15.4% had mild, 16.3% moderate, and 64.5% had severe obstructive sleep apnea.
Patients were divided into groups according to AHI levels in order to present values of clinical parameters in an easy-to-understand fashion. Taking into account that the group with no OSAS was too small (only 4 patients), we classified patients into 3 AHI groups: AHI < 15 (20 patients), AHI 15 - 29.9 (17 patients), and AHI ≥ 30 (67 patients) as presented in Table 1. Our results showed a clear significant difference between AHI groups in TT and FT levels (p<0.001). There was also a significant difference between AHI groups in terms of age, BMI, waist and neck circumference, blood pressure levels, hypertension prevalence, triglyceride, high-density lipoprotein and FPG levels, metabolic syndrome prevalence, and siMS score (Table 1). There was no significant difference in TT (12.8 ± 5.3 vs. 13.1 ± 5.5 nmol/l; p = 0.734) or FT level (346.1 ± 124.9 vs. 331.6 ± 154.1 pmol/l; p = 0.605), AHI (46.4 ± 29.7 vs. 44.7 ± 27.4; p = 0.762) and ODI (44.5 ± 31.8 vs. 42.7 ± 29.9; p = 0.767) between smokers and non-smokers.
There was a significant negative correlation between TT and AHI (r = -0.409, p < 0.001) and ODI (r = -0.458, p < 0.001) levels. There was also a significant negative correlation between FT and AHI (r = -0.389, p < 0.001) and ODI (r = -0.438, p <0.001) levels (Figure 2). Both TT and FT levels were in a significant negative correlation with BMI (r = -0.269, p = 0.006 and r = -0.311, p = 0.001, respectively), weight (r = -0.203, p = 0.039 and r = -0.227, p = 0.02, respectively), hip circumference (r = -0. 234, p = 0.017 and r = -0.243, p = 0.013, respectively), some metabolic parameters such as waist circumference (r = -0.374, p < 0.001 and r = -0.398, p < 0.001, respectively) DBP (r = -0.264, p = 0.007 and r = -0.294, p = 0.002, respectively), FPG (r = -0.274, p = 0.005 and r = -0.296, p = 0.002, respectively), and also with MetS prevalence r = -0.193, p = 0.049 and r = -0.195, p = 0.048, respectively) and siMS score levels (r = -0.321, p = 0.001 and r = -0.283, p = 0.004, respectively) (Table 2). FT levels were in a significant negative correlation with age (r = -0.346, p<0.001) (Table 2).
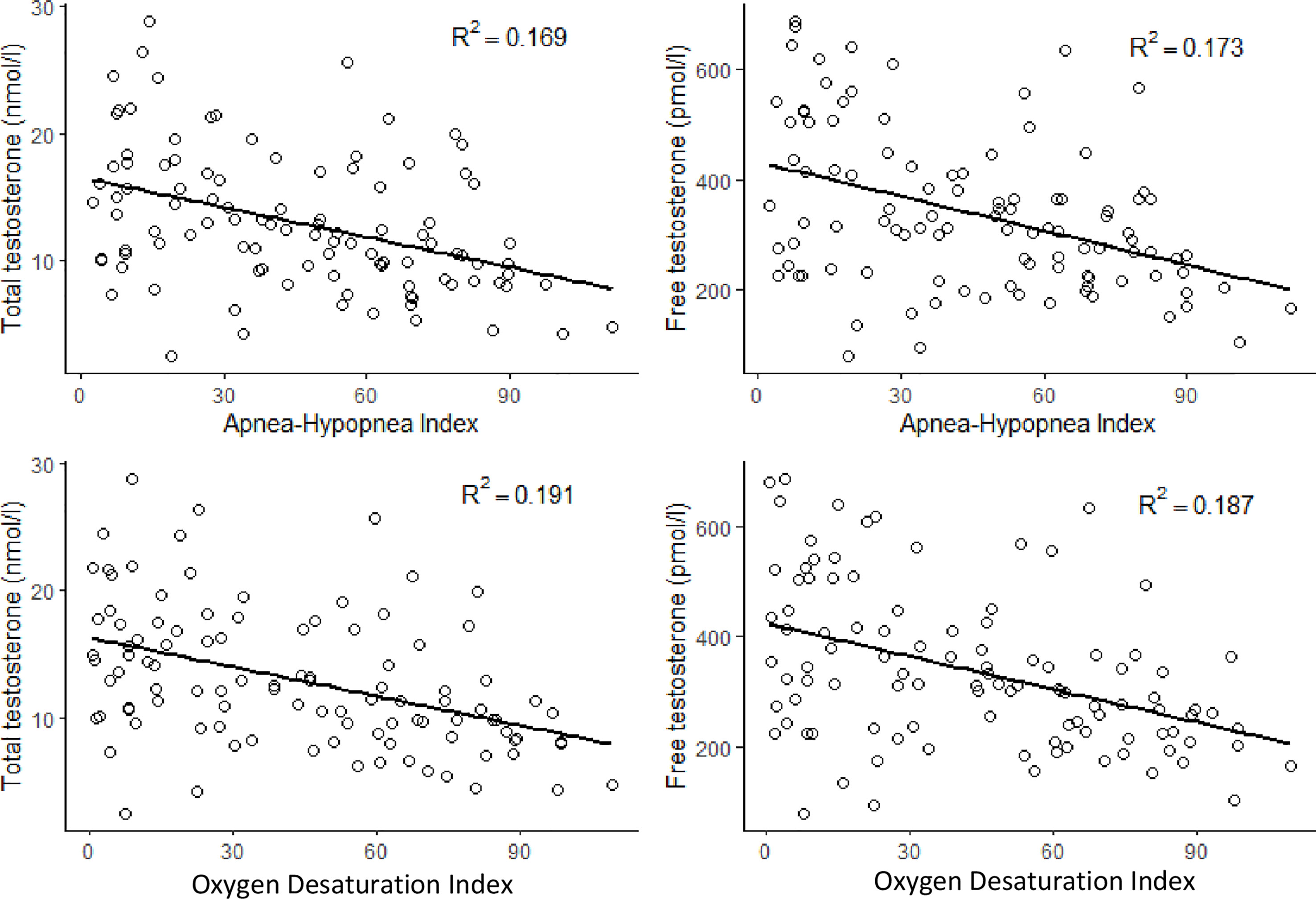
Figure 2 Correlations between total testosterone and free testosterone with apnea-hypopnea index and oxygen desaturation index.
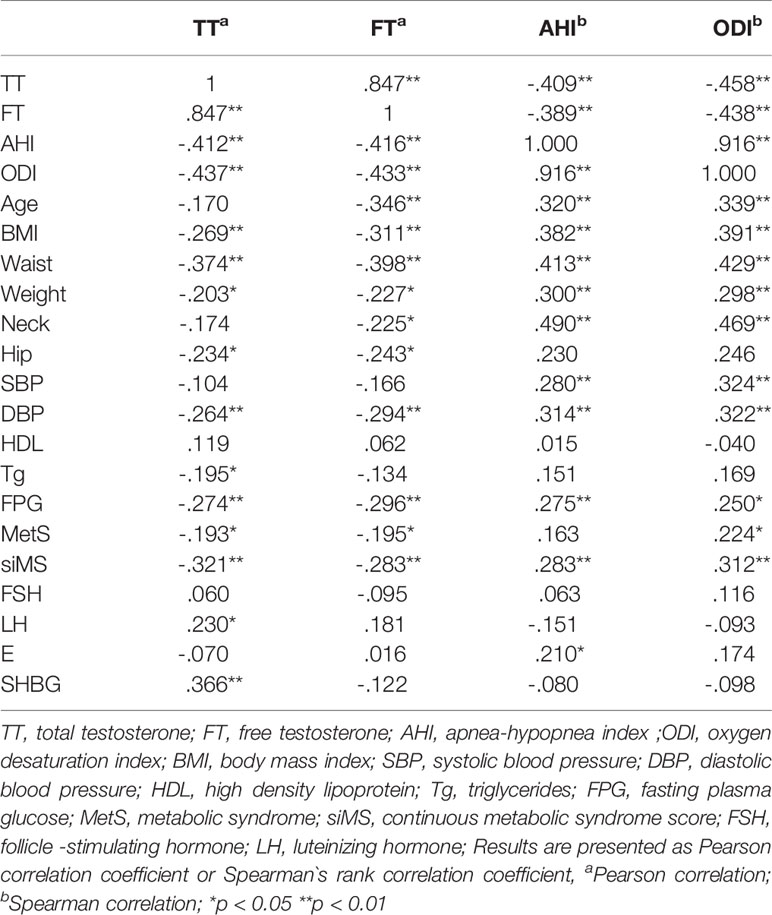
Table 2 Correlation matrix between testosterone, free testosterone, AHI, ODI and general characteristics of patients.
Furthermore, there was a significant positive correlation between AHI and ODI levels with age (r = 0.320, p = 0.001 and r = 0.339, p < 0.001, respectively), BMI (r = 0.382, p < 0.001 and r = 0.391, p < 0.001, respectively), weight (r = 0.300, p = 0.002 and r = 0.298, p = 0.002, respectively) waist (r = 0.413, p < 0.001 and r = 0.429, p < 0.001, respectively), hip (r = 0.230, p = 0.019 and r = 0.246, p = 0.012) and neck circumference (r = 0.490, p < 0.001 and r = 0.469, p < 0.001, respectively), SBP (r = 0.280, p = 0.004 and r = 0.324, p = 0.001, respectively), DBP (r = 0.314, p = 0.001 and r = 0.322, p = 0.001, respectively), FPG (r = 0.275, p = 0.005 and r = 0.250, p = 0.001, respectively), and siMS score levels (r = 0.283, p = 0.004 and r = 0.312, p< 0.001, respectively) (Table 2).
In multiple regression analysis, after adjustment for age, BMI, siMS score and MetS components, both AHI and ODI were in significant correlation with TT and FT (p < 0.05) (Table 3). The results of multiple linear regression analysis did not change in subgroup of patients with BMI ≥ 40kg/m2 (80 patients in total) (Table 4). The calculated variance inflation factors showed no multicollinearity in regression models.
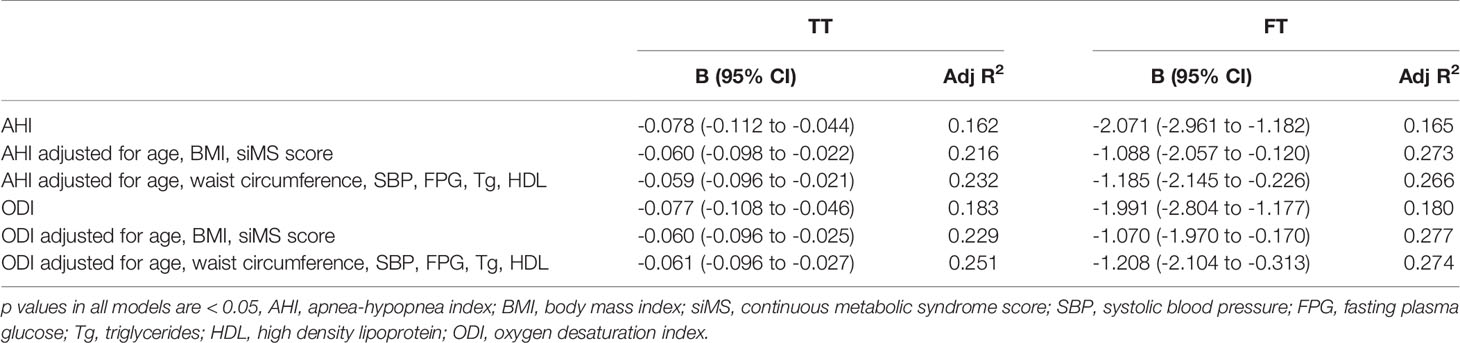
Table 3 Multiple linear regression model for prediction of total testosterone (TT) and free testosterone (FT).

Table 4 Multiple linear regression model for prediction of total testosterone (TT) and free testosterone (FT) for patients with BMI ≥ 40 kg/m2.
In order to assess if TT and FT can be used as discriminative variables for the assessment of sleep apnea severity (using 15 and 30 level cut-off for AHI) we have performed receiver-operating characteristic (ROC) curves analysis. AUC for AHI ≥ 15 for TT was AUCTT = 0.714 (95% CI 0.587 - 0.841; p = 0.003) with cut off = 14.5 (Sn = 0.726; Sp = 0.650), and for FT AUCFT = 0.719 (95% CI 0.588 - 0.851; p = 0.002) with cut off = 412 (Sn = 0.821; Sp = 0.600) (Figure 3A).
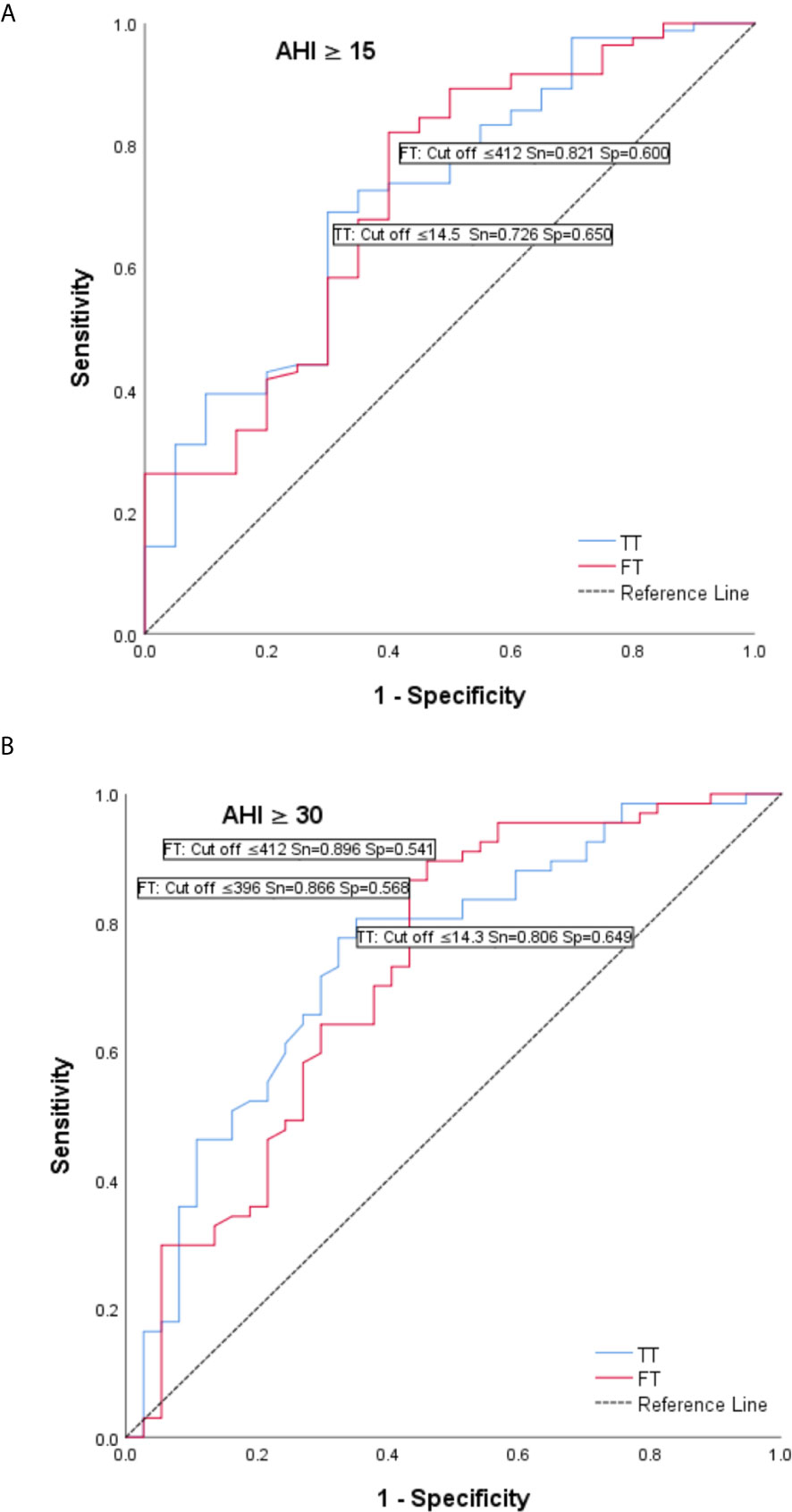
Figure 3 Receiver operating characteristic (ROC) curves – total testosterone (T) and tree testosterone (FT) as discriminative variables for the assessment of sleep apnea severity. (A) AUC for AHI ≥ 15 for TT was AUCT = 0.714 (95% CI 0.587 - 0.841; p = 0.003) with cut off = 14.5 (Sn = 0.726; Sp = 0.650), and for FT AUCFT = 0.719 (95% CI 0.588 - 0.851; p = 0.002) with cut off = 412 (Sn = 0.821; Sp = 0.600). (B) AUC for AHI ≥ 30 for TT was AUCT = 0.748 (95% CI 0.648 - 0.849; p < 0.001) with cut off = 14.3 (Sn = 0.806; Sp = 0.649) and for FT AUCFT = 0.728 (95% CI 0.620 - 0.836; p < 0.001) with cut off = 396 (Sn = 0.866; Sp = 0.568).
AUC for AHI ≥ 30 for TT was AUCTT = 0.748 (95% CI 0.648 - 0.849; p < 0.001) with cut off = 14.3 (Sn = 0.806; Sp = 0.649) and for FT AUCFT = 0.728 (95% CI 0.620 - 0.836; p < 0.001) with cut off = 396 (Sn = 0.866; Sp = 0.568) (Figure 3B).
Discussion
Obesity is considered to be the main cause of hypogonadism in men. Hypogonadism is a clinical entity characterized by low serum TT concentration and/or FT concentration and associated symptoms and signs of testosterone deficiency (30). Obesity-related hypogonadism is functional, and it can be reverted by substantial weight loss achieved with non-surgical or surgical interventions (31). Hypoandrogenemia is a term referring to the finding of subnormal testosterone concentrations in men without taking into consideration clinical symptoms or signs of decreased serum testosterone levels. The prevalence of hypoandrogenemia is 4% to 5% in the general male population and as much as 20% to 40% in obese men (30). In our study, 48.1% of severely obese men had TT level < 348.3 ng/dl, and 29.8% had FT level < 70.0 pg/ml. Our results are consistent with the previous data that the presence of low TT and FT in men is closely related to the increased BMI with the highest hypoandrogenemia in more severe obesity (30).
Age is the most prominent predictor of most diseases in humans. Chronological aging per se and age-related changes in overall health and lifestyle are associated with natural declines in serum testosterone (32), presented in our study as a negative relationship between FT and age. Abdominal adiposity is one of five clinical risk factors used as diagnostic criteria for metabolic syndrome. Although obesity and metabolic syndrome frequently coexist, this is not always the case.
A significant proportion of obese individuals do not have metabolic syndrome, and, conversely, metabolic syndrome may be present in non-obese individuals (33). In our study, 77.9% of severely obese men had metabolic syndrome. The metabolic syndrome in obese men is associated with a further decline in testosterone level, with a negative inverse relationship between TT and/or FT levels and metabolic syndrome (8, 34, 35), also observed in our study.
The most striking result to emerge from our data is that OSAS, measured by both AHI and ODI, is an independent determinant of serum testosterone concentration in severely obese men after adjustment for BMI, age, or siMS score. Moreover, the ROC analysis showed that both TT and FT levels could be used as discriminative variables for the assessment of sleep apnea severity.
Patients with OSAS have reduced quality and quantity of sleep due to sleep fragmentation, intermittent nocturnal hypoxia, reduced deep and rapid eye movement (REM) sleep, reduced sleep duration, and sleep efficiency, all of which lead to pituitary-gonadal dysfunction and low testosterone levels in male patients (5, 36).
The association of respiratory hypoxia and low serum testosterone in men was observed as early as 40 years ago when it was shown that in patients with chronic obstructive pulmonary disease, erectile dysfunction and low serum testosterone concentrations correlated with the degree of arterial hypoxia (37, 38). A couple of years later, the same results were obtained in patients with pulmonary fibrosis (39), pulmonary heart (40), and Pickwick syndrome (41). Kouchiyama et al., in the study conducted on 24 patients, showed that greater nocturnal oxygen desaturation in men led to the disruption of the circadian rhythm of testosterone secretion (42).
In the animal models, studies focusing on the effects of continuous or intermittent hypoxia on sex hormones were inconclusive because - depending on the study - they showed an increase (43), decrease (44, 45), and unchanged testosterone concentrations (46).
Partial or complete upper airway obstruction in OSAS patients is the cause of not only nocturnal oxygen desaturation but also sleep fragmentation. A significant number of studies have been carried out by Luboshitzky and colleagues to investigate whether reproductive hormones are correlated with sleep patterns in men with OSAS. They showed that the patients with fragmented sleep had a blunted nocturnal rise of testosterone only if they did not show REM sleep (47). The same team suggested that OSAS in male patients is associated with reduced androgen secretion resulting from altered pituitary-gonadal function (48). This is caused by obesity and aging, with hypoxia and sleep fragmentation being additional contributing factors in decreasing pulsatile testosterone secretion in these patients (49).
Clinical evidence is contradictory, with some (9, 16–20), but not all (21, 22), studies reporting that OSAS is a factor favoring hypoandrogenemia independent of obesity. This could be due to high heterogeneity of study design, patients’ characteristics such as age and BMI, exclusion or inclusion criteria, limited or absent covariates in the data analyses, and the time point of data collection (9, 16–22).
Hammoud et al. published a study similar to ours. This study included 89 severely obese men with a BMI ≥ 35 kg/m2 to examine the effect of sleep apnea on the reproductive hormones and sexual function in obese men. They showed that increased severity of sleep apnea is associated with lower TT and FT levels independent of age and BMI, which is consistent with the results of our study (18). Their study, unlike our study, did not take into account the metabolic syndrome as a contributing factor to hypoandrogenemia in obese men.
Concerning this latter aspect, there is only one study published by Gambineri et al. that analyzed the severity of OSAS, testosterone levels, and some of the parameters of MetS (waist circumference, FPG, HDL, Tg) in severely obese men. They suggested that OSAS may contribute to causing metabolic abnormalities in men and that this relationship may be in part related to the reduced testosterone concentrations (9). Taking this into consideration, we included metabolic syndrome as a potential confounding factor for hypoandrogenemia in our obese men.
Gambineri and colleagues documented that in men with obesity and OSAS, the severity of hypoxia measured by ODI may be an additional factor in reducing testosterone levels, regardless of BMI and abdominal fatness (9). Our study shows that AHI and ODI, as pivotal markers of OSAS severity are in significant correlation with TT and FT independent of age, BMI, siMS score and MetS components in severely obese men.
The effects of OSAS treatment on testosterone levels are debatable. Continuous positive airway pressure (CPAP) is the most effective non-surgical treatment for OSAS. Furthermore, the efficacy of CPAP on hypoandrogenemia in OSAS male subjects are still controversial. Some studies have demonstrated that CPAP elevates testosterone levels (49, 50). However, the majority of other studies, including two meta-analyses, have reached a different conclusion (51, 52). As pointed out by one of the latest systematic reviews, a small number of included studies in their meta-analysis reported an adequate CPAP use (4 h per night on at least 70% of nights), and thus the results might reflect, at least in part, suboptimal CPAP therapy. Furthermore, Santamaria et al. thoroughly conducted a prospective study of uvulopalatopharyngoplasty therapy effects on testosterone levels in male subjects with moderate and severe OSAS. Interestingly, they showed improvement in testosterone levels three months after the surgery, with correlated improvement in sleep-disordered breathing without significant changes in BMI (53). On the other hand, CPAP as the mainstay of treatment for OSAS will not cure obesity, as a cornerstone of OSAS and metabolic syndrome, as well as hypoandrogenemia in obese men. In any case, significant weight loss clearly improved OSAS, metabolic syndrome, and hypogonadism associated with obesity (1–5, 54).
Some limitations of this investigation should be acknowledged. The design of our study did not provide for monitoring the symptoms of sexual dysfunction and hypogonadism in the subjects. Thus, we can discuss sex hormones only from the perspective of hypoadrogenism and not in terms of hypogonadism. Another limitation of our study is that instead of using ‘full’ polysomnography, we used a seven-channel portable sleep recorder. Given the fact that there was no electroencephalography monitoring, we do not have data about the relationships between sleep stages and sex hormone levels. Also, we could not assess if the physical activity or regular alcohol consumption influenced the results of our study, as we did not have this data available.
Conclusion
Our data show that obstructive sleep apnea syndrome is in significant correlation with TT and FT levels in severely obese men. Further research is needed to elucidate the complex link between sleep apnea and testosterone levels in obese men for the purpose of appropriate management of these patients.
Data Availability Statement
The raw data supporting the conclusions of this article will be made available by the authors, without undue reservation.
Ethics Statement
The studies involving human participants were reviewed and approved by The Faculty of Medicine, University of Belgrade, Ethics Committee. The patients/participants provided their written informed consent to participate in this study.
Author Contributions
MT-G, MV, and SV: conceived and designed the study, collected and contributed to the data, and analyzed and interpreted data. MT-G: wrote the manuscript. MV and SV: revised the article. MI, LM, ZA, MS, and AĐ: collected and contributed to the data and revised the article. AK: analyzed and interpreted data, and revised the article. IS: analyzed and interpreted data, made tables, and revised the article. All authors contributed to the article and approved the submitted version.
Conflict of Interest
The authors declare that the research was conducted in the absence of any commercial or financial relationships that could be construed as a potential conflict of interest.
Publisher’s Note
All claims expressed in this article are solely those of the authors and do not necessarily represent those of their affiliated organizations, or those of the publisher, the editors and the reviewers. Any product that may be evaluated in this article, or claim that may be made by its manufacturer, is not guaranteed or endorsed by the publisher.
Abbreviations
BMI, body mass index; SHBG, sex hormone-binding globulin; OSAS, obstructive sleep apnea syndrome; Tg, triglyceride; HDL, high-density lipoprotein; LH, luteinizing hormone; FSH, follicle-stimulating hormone; AHI, apnea-hypopnea index; ODI, oxygen desaturation index; MetS, metabolic syndrome; siMS, continuous metabolic syndrome score.
References
1. Corona G, Rastrelli G, Monami M, Saad F, Luconi M, Lucchese M, et al. Body Weight Loss Reverts Obesity-Associated Hypogonadotropic Hypogonadism: A Systematic Review and Meta-Analysis. Eur J Endocrinol (2013) 168:829–43. doi: 10.1530/EJE-12-0955
2. Chourdakis M. Obesity: Assessment and Prevention. Clin Nutr ESPEN (2020) 39:1–14. doi: 10.1016/j.clnesp.2020.07.012
3. Mah PM, Wittert GA. Obesity and Testicular Function. Mol Cell Endocrinol (2010) 316:180–6. doi: 10.1016/j.mce.2009.06.007
4. Hammoud AO, Carrell DT, Gibson M, Peterson CM, Meikle AW. Updates on the Relation of Weight Excess and Reproductive Function in Men: Sleep Apnea as a New Area of Interest. Asian J Androl (2012) 14:77–81. doi: 10.1038/aja.2011.64
5. Sultan S, Patel AG, El-Hassani S, Whitelaw B, Leca BM, Vincent RP, et al. Male Obesity Associated Gonadal Dysfunction and the Role of Bariatric Surgery. Front Endocrinol (Lausanne) (2020) 11:408. doi: 10.3389/fendo.2020.00408
6. Corona G, Vignozzi L, Sforza A, Mannucci E, Maggi M. Obesity and Late-Onset Hypogonadism. Mol Cell Endocrinol (2015) 418:120–33. doi: 10.1016/j.mce.2015.06.031
7. Grossmann M, Ng Tang Fui M, Cheung AS. Late-Onset Hypogonadism: Metabolic Impact. Andrology (2019) 8:1519–29. doi: 10.1111/andr.12705
8. Corona G, Monami M, Rastrelli G, Aversa A, Tishova Y, Saad F, et al. Testosterone and Metabolic Syndrome: A Meta-Analysis Study. J Sex Med (2011) 8:272–83. doi: 10.1111/j.1743-6109.2010.01991.x
9. Gambineri A, Pelusi C, Pasquali R. Testosterone Levels in Obese Male Patients With Obstructive Sleep Apnea Syndrome: Relation to Oxygen Desaturation, Body Weight, Fat Distribution and the Metabolic Parameters. J Endocrinol Invest (2003) 26:493–8. doi: 10.1007/BF03345209
10. Liu PY. A Clinical Perspective of Sleep and Andrological Health: Assessment, Treatment Considerations, and Future Research. J Clin Endocrinol Metab (2019) 104:4398–417. doi: 10.1210/jc.2019-00683
11. Flemons WW, Buysse D, Redline S, Oack A, Strohl K, Wheatley J, et al. Sleep-Related Breathing Disorders in Adults: Recommendations for Syndrome Definition and Measurement Techniques in Clinical Research. Sleep (1999) 22:667–89. doi: 10.1093/sleep/22.5.667
12. Gottlieb DJ, Punjabi NM. Diagnosis and Management of Obstructive Sleep Apnea: A Review. JAMA - J Am Med Assoc (2020) 323:1380–400. doi: 10.1001/jama.2020.3514
13. Lanfranco F, Motta G, Minetto MA, Baldi M, Balbo M, Ghigo E, et al. Neuroendocrine Alterations in Obese Patients With Sleep Apnea Syndrome. Int J Endocrinol (2010) 2010:474518. doi: 10.1155/2010/474518
14. Pillar G, Shehadeh N. Abdominal Fat and Sleep Apnea: The Chicken or the Egg? Diabetes Care (2008) 31:S303–9. doi: 10.2337/dc08-s272
15. McNicholas WT. Obstructive Sleep Apnoea and Comorbidity–an Overview of the Association and Impact of Continuous Positive Airway Pressure Therapy. Expert Rev Respir Med (2019) 13:251–61. doi: 10.1080/17476348.2019.1575204
16. Canguven O, Salepci B, Albayrak S, Selimoglu A, Balaban M, Bulbul M. Is There a Correlation Between Testosterone Levels and the Severity of the Disease in Male Patients With Obstructive Sleep Apnea? Arch Ital di Urol e Androl (2010) 82:143–7.
17. Kirbas G, Abakay A, Topcu F, Kaplan A, Ünlü M, Peker Y. Obstructive Sleep Apnoea, Cigarette Smoking and Serum Testosterone Levels in a Male Sleep Clinic Cohort. J Int Med Res (2007) 35:38–45. doi: 10.1177/147323000703500103
18. Hammoud AO, Walker JM, Gibson M, Cloward TV, Hunt SC, Kolotkin RL, et al. Sleep Apnea, Reproductive Hormones and Quality of Sexual Life in Severely Obese Men. Obesity (2011) 19:1118–23. doi: 10.1038/oby.2010.344
19. Meston N, Davies RJO, Mullins R, Jenkinson C, Wass JAH, Stradling JR. Endocrine Effects of Nasal Continuous Positive Airway Pressure in Male Patients With Obstructive Sleep Apnoea. J Intern Med (2003) 254:447–54. doi: 10.1046/j.1365-2796.2003.01212.x
20. Grunstein RR, Handelsman DJ, Lawrence SJ, Blackwell C, Caterson ID, Sullivan CE. Neuroendocrine Dysfunction in Sleep Apnea: Reversal by Continuous Positive Airways Pressure Therapy. J Clin Endocrinol Metab (1989) 68:352–8. doi: 10.1210/jcem-68-2-352
21. Barrett-Connor E, Dam TT, Stone K, Harrison SL, Redline S, Orwoll E. The Association of Testosterone Levels With Overall Sleep Quality, Sleep Architecture, and Sleep-Disordered Breathing. J Clin Endocrinol Metab (2008) 93:2602–9. doi: 10.1210/jc.2007-2622
22. Clarke BM, Vincent AD, Martin S, Adams R, Appleton S, Vakulin A, et al. Obstructive Sleep Apnea is Not an Independent Determinant of Testosterone in Men. Eur J Endocrinol (2020) 183:31–9. doi: 10.1530/EJE-19-0978
23. Tančić-Gajić M, Vujović S, Vukčević M, Ivović M, Drezgić M, Marina LV, et al. Effects of Alternate Fasting or Very Low Calorie Diet and Low Calorie Diet on Metabolic Syndrome in Severely Obese Patients. Hippokratia (2012) 16:335–41.
24. Lam B, Ip MSM, Tench E, Ryan CF. Craniofacial Profile in Asian and White Subjects With Obstructive Sleep Apnoea. Thorax (2005) 60:504–10. doi: 10.1136/thx.2004.031591
25. Berry RB, Budhiraja R, Gottlieb DJ, Gozal D, Iber C, Kapur VK, et al. Rules for Scoring Respiratory Events in Sleep: Update of the 2007 AASM Manual for the Scoring of Sleep and Associated Events. J Clin Sleep Med (2012) 8:597–619. doi: 10.5664/jcsm.2172
26. Alberti KGMM, Eckel RH, Grundy SM, Zimmet PZ, Cleeman JI, Donato KA, et al. Harmonizing the Metabolic Syndrome: A Joint Interim Statement of the International Diabetes Federation Task Force on Epidemiology and Prevention; National Heart, Lung, and Blood Institute; American Heart Association; World Heart Federation; International. Circulation (2009) 120:1640–5. doi: 10.1161/CIRCULATIONAHA.109.192644
27. Soldatovic I, Vukovic R, Culafic D, Gajic M, Dimitrijevic-Sreckovic V. SiMS Score: Simple Method for Quantifying Metabolic Syndrome. PloS One (2016) 11:e0146143. doi: 10.1371/journal.pone.0146143
28. Vermeulen A, Verdonck L, Kaufman JM. A Critical Evaluation of Simple Methods for the Estimation of Free Testosterone in Serum. J Clin Endocrinol Metab (1999) 84:3666–72. doi: 10.1210/jcem.84.10.6079
29. Bhasin S, Brito JP, Cunningham GR, Hayes FJ, Hodis HN, Matsumoto AM, et al. Testosterone Therapy in Men With Hypogonadism: An Endocrine Society. J Clin Endocrinol Metab (2018) 103:1715–44. doi: 10.1210/jc.2018-00229
30. Molina-Vega M, Asenjo-Plaza M, García-Ruiz MC, Varea-Marineto E, Casal-Nievas N, Álvarez-Millán JJ, et al. Cross-Sectional, Primary Care–Based Study of the Prevalence of Hypoandrogenemia in Nondiabetic Young Men With Obesity. Obesity (2019) 27:1584–90. doi: 10.1002/oby.22579
31. Crisóstomo L, Pereira SC, Monteiro MP, Raposo JF, Oliveira PF, Alves MG. Lifestyle, Metabolic Disorders and Male Hypogonadism – A One-Way Ticket? Mol Cell Endocrinol (2020) 516:110945. doi: 10.1016/j.mce.2020.110945
32. Travison TG, Araujo AB, Kupelian V, O’Donnell AB, McKinlay JB. The Relative Contributions of Aging, Health, and Lifestyle Factors to Serum Testosterone Decline in Men. J Clin Endocrinol Metab (2007) 92:549–55. doi: 10.1210/jc.2006-1859
33. Kip KE, Marroquin OC, Kelley DE, Johnson BD, Kelsey SF, Shaw LJ, et al. Clinical Importance of Obesity Versus the Metabolic Syndrome in Cardiovascular Risk in Women: A Report From the Women’s Ischemia Syndrome Evaluation (WISE) Study. Circulation (2004) 109:706–13. doi: 10.1161/01.CIR.0000115514.44135.A8
34. Gautier A, Bonnet F, Dubois S, Massart C, Grosheny C, Bachelot A, et al. Associations Between Visceral Adipose Tissue, Inflammation and Sex Steroid Concentrations in Men. Clin Endocrinol (Oxf) (2013) 78:373–8. doi: 10.1111/j.1365-2265.2012.04401.x
35. Brand JS, van der Tweel I, Grobbee DE, Emmelot-Vonk MH, van der Schouw YT. Testosterone, Sex Hormone-Binding Globulin and the Metabolic Syndrome: A Systematic Review and Meta-Analysis of Observational Studies. Int J Epidemiol (2011) 40:189–207. doi: 10.1093/ije/dyq158
36. Fernandez CJ, Chacko EC, Pappachan JM. Male Obesity-Related Secondary Hypogonadism – Pathophysiology, Clinical Implications and Management. Eur Endocrinol (2019) 15:83–90. doi: 10.17925/EE.2019.15.2.83
37. Semple D’A.P, Beastall GH, Watson WS, Hume R. Serum Testosterone Depression Associated With Hypoxia in Respiratory Failure. Clin Sci (1980) 58:105–6. doi: 10.1042/cs0580105
38. Semple PD, Beastall GH, Hume R. Male Sexual Dysfunction, Low Serum Testosterone and Respiratory Hypoxia. Br J Sex Med (1980) 7:48–53.
39. Semple PD, Beastall GH, Brown TM, Stirling KW, Mills RJ, Watson WS. Sex Hormone Suppression and Sexual Impotence in Hypoxic Pulmonary Fibrosis. Thorax (1984) 39:46–51. doi: 10.1136/thx.39.1.46
40. d’A Semple P, Watson WS, Beastall GH, Hume R. Endocrine and Metabolic Studies in Unstable Cor Pulmonale. Thorax (1983) 38:45–9. doi: 10.1136/thx.38.1.45
41. d’A Semple P, Graham A, Malcolm Y. Hypoxia, Depression of Testosterone, and Impotence in Pickwickian Syndrome Reversed by Weight Reduction. Br Med J (1984) 289:801–2. doi: 10.1136/bmj.289.6448.801
42. Kouchiyama S, Honda Y, Kuriyama T. Influence of Nocturnal Oxygen Desaturation on Circadian Rhythm of Testosterone Secretion. Respiration (1990) 57:359–63. doi: 10.1159/000195872
43. Cho YM, Chou JC, Fang CM, Hu S, Wang KL, Wang SW, et al. Chronic Intermittent Hypoxia Stimulates Testosterone Production in Rat Leydig Cells. Life Sci (2019) 233:116694. doi: 10.1016/j.lfs.2019.116694
44. Wilson EN, Anderson M, Snyder B, Duong P, Trieu J, Schreihofer DA, et al. Chronic Intermittent Hypoxia Induces Hormonal and Male Sexual Behavioral Changes: Hypoxia as an Advancer of Aging. Physiol Behav (2018) 189:64–73. doi: 10.1016/j.physbeh.2018.03.007
45. Liu GL, Yu F, Dai DZ, Zhang GL, Zhang C, Dai Y. Endoplasmic Reticulum Stress Mediating Downregulated StAR and 3-Beta-HSD and Low Plasma Testosterone Caused by Hypoxia Is Attenuated by CPU86017-RS and Nifedipine. J BioMed Sci (2012) 19:4. doi: 10.1186/1423-0127-19-4
46. Soukhova-O’Hare GK, Shah ZA, Lei Z, Nozdrachev AD, Rao CV, Gozal D. Erectile Dysfunction in a Murine Model of Sleep Apnea. Am J Respir Crit Care Med (2008) 178:644–50. doi: 10.1164/rccm.200801-190OC
47. Luboshitzky R, Zabari Z, Shen-Orr Z, Herer P, Lavie P. Disruption of the Nocturnal Testosterone Rhythm by Sleep Fragmentation in Normal Men. J Clin Endocrinol Metab (2001) 86:1134–9. doi: 10.1210/jcem.86.3.7296
48. Luboshitzky R, Aviv A, Hefetz A, Herer P, Shen-Orr Z, Lavie L, et al. Decreased Pituitary-Gonadal Secretion in Men With Obstructive Sleep Apnea. J Clin Endocrinol Metab (2002) 87:3394–8. doi: 10.1210/jcem.87.7.8663
49. Luboshitzky R, Lavie L, Shen-Orr Z, Lavie P. Pituitary-Gonadal Function in Men With Obstructive Sleep Apnea. The Effect of Continuous Positive Airways Pressure Treatment. Neuroendocrinol Lett (2003) 24:463–7.
50. Li Z, Tang T, Wu W, Gu L, Du J, Zhao T, et al. Efficacy of Nasal Continuous Positive Airway Pressure on Patients With OSA With Erectile Dysfunction and Low Sex Hormone Levels. Respir Med (2016) 119:130–4. doi: 10.1016/j.rmed.2016.09.001
51. Zhang XB, Lin QC, Zeng HQ, Jiang XT, Chen B, Chen X. Erectile Dysfunction and Sexual Hormone Levels in Men With Obstructive Sleep Apnea: Efficacy of Continuous Positive Airway Pressure. Arch Sex Behav (2016) 45:235–40. doi: 10.1007/s10508-015-0593-2
52. Cignarelli A, Castellana M, Castellana G, Perrini S, Brescia F, Natalicchio A, et al. Effects of CPAP on Testosterone Levels in Patients With Obstructive Sleep Apnea: A Meta-Analysis Study. Front Endocrinol (Lausanne) (2019) 10:551. doi: 10.3389/fendo.2019.00551
53. SANTAMARIA JD, PRIOR JC, FLEETHAM JA. Reversible Reproductive Dysfunction in Men With Obstructive Sleep Apnoea. Clin Endocrinol (Oxf) (1988) 28:461–70. doi: 10.1111/j.1365-2265.1988.tb03680.x
Keywords: obesity, metabolic syndrome, sleep apnea, testosterone, male
Citation: Tančić-Gajić M, Vukčević M, Ivović M, Marina LV, Arizanović Z, Soldatović I, Stojanović M, Đogo A, Kendereški A and Vujović S (2021) Obstructive Sleep Apnea Is Associated With Low Testosterone Levels in Severely Obese Men. Front. Endocrinol. 12:622496. doi: 10.3389/fendo.2021.622496
Received: 28 October 2020; Accepted: 16 June 2021;
Published: 26 July 2021.
Edited by:
Elisabetta Baldi, University of Florence, ItalyReviewed by:
Angelo Cignarelli, University of Bari Aldo Moro, ItalyFrancesco Lotti, University of Florence, Italy
Copyright © 2021 Tančić-Gajić, Vukčević, Ivović, Marina, Arizanović, Soldatović, Stojanović, Đogo, Kendereški and Vujović. This is an open-access article distributed under the terms of the Creative Commons Attribution License (CC BY). The use, distribution or reproduction in other forums is permitted, provided the original author(s) and the copyright owner(s) are credited and that the original publication in this journal is cited, in accordance with accepted academic practice. No use, distribution or reproduction is permitted which does not comply with these terms.
*Correspondence: Ljiljana V. Marina, bGppa2thQHlhaG9vLmNvbQ==; bGppbGphLm1hcmluYUBnbWFpbC5jb20=