- 1Ministry of Education and Shanghai Key Laboratory of Children’s Environmental Health, Xinhua Hospital, Shanghai Jiao Tong University School of Medicine, Shanghai, China
- 2Department of Reproductive Immunology, Shanghai First Maternity and Infant Hospital, Tongji University School of Medicine, Shanghai, China
- 3Department of Obstetrics and Gynecology, Duke University School of Medicine, Durham, NC, United States
The underlying correlative mechanisms between Insulin resistance (IR) and recurrent pregnancy loss (RPL) in patients without polycystic ovarian syndrome (PCOS) remain inconclusive. To investigate the association between triglyceride (TG) levels, lymphocyte subsets, and IR in RPL patients without PCOS and obesity. Eighty-nine subjects with an unexplained RPL, independent of PCOS/obesity were enrolled in this study. A 75-g oral glucose tolerance test was performed on each subject with plasma tested for glucose and insulin. The fasting venous blood of all subjects was collected for routine clinical chemistry analysis. Lymphocyte subsets were analyzed by four-color flow cytometry. As a result, TG levels were significantly elevated in RPL patients with IR compared to those without IR. Pearson linear correlation model and receiver operating characteristic (ROC) curve analyses revealed a significant positive association between TG and HOMA-IR index value. In multiple logistic regression analysis, TG was significantly associated with the risk of hyperinsulinemia and increased CD3+CD4+/CD3+CD8+ ratio which was significantly negatively correlated with disposition index (DI30) and DI120, indicators for insulin sensitivity. In addition, DI30 and DI120 were significantly decreased in the higher CD3+CD4+/CD3+CD8+ group. Our findings showed that the elevated TG and altered immune responses in RPL patients with IR are independent of PCOS and obesity, and could be used as an indicator of IR in RPL patients. These results contribute to the understanding of the pathophysiology of IR in RPL for potential prevention and therapeutic targets.
Introduction
Miscarriage, the most common complication of pregnancy, is the spontaneous loss of a conceptus before 20 weeks’ gestation, and it affects approximately 15% of reproductive-age women recognized clinically (1). There are two types of miscarriage: sporadic and recurrent. Recurrent pregnancy loss (RPL), defined as three or more consecutive pregnancy losses, occurs in approximately 1% of childbearing couples (2). Actually, many clinicians define RPL as two or more losses, due to the recurrence rate is similar to that after three losses, and it affects around 1% to 5% of couples (2–4). Historically, RPL has been attributed to either genetic, structural, infective, endocrine, immune, or unexplained causes (2, 5–8). Endocrine disturbances have been postulated as an important cause of RPL, which widens the scope of investigation.
In recent years, researchers have focused on the connection between polycystic ovarian syndrome (PCOS), insulin resistance (IR), and RPL (9, 10). About 70% of women with PCOS have IR (11), and spontaneous pregnancy loss occurs in 40% women with PCOS (12). Data from clinical trials also showed that IR is common in women with RPL (13–16), and has been associated with an increased rate of pregnancy loss in PCOS patients (17). In fact, there are many RPL subjects with IR who do not have PCOS and/or obesity. It has been reported that non-obese Asians (BMI < 25 kg/m2) can readily develop metabolic abnormalities (18, 19). Tamura et al., also reported that impaired insulin clearance and hyperinsulinemia could occur in apparently healthy subjects (20). Studies on the underlying correlative mechanisms between IR and RPL in patients without PCOS remain inconclusive. Guidelines from the Endocrine Society commonly recommend using metformin for women with IR and glucose intolerance due to its functional role on activating the insulin receptor and increasing glucose uptake. However, a randomized double-blind study also indicated that metformin has no major effects on glucose homeostasis in pregnant women with PCOS (21, 22), which indicates there are other underlying causes of IR in RPL patients beyond abnormal glucose metabolism. Therefore, it is imperative to understand the pathophysiology of IR in RPL for potential prevention and therapeutic targets.
Insulin resistance is characterized by a decreased responsiveness of insulin sensitivity and supraphysiological levels of insulin. It has been observed that a chronic inflammatory state may contribute to the development of IR (23, 24). Dysregulation of fat metabolism, characterized by significantly increased lipid accumulations, and triglycerides (TG) level, are known to induce low grade inflammation (25, 26). It was reported that the level of LDL cholesterol increases, whereas that of HDL cholesterol decreases in PCOS (27). Furthermore, elevated TG/HDL cholesterol ratio was significantly associated with IR and could be used as a predictor of IR in women with PCOS (28). However, the link between TG levels, inflammation, and IR in women without PCOS is unclear and need further study. It’s worth noting that the T cell lymphocytes play a pivotal role in regulating the effector stage of the immune response, and increased lymphocyte proliferation is associated with an inflammatory response (29–31). An increased ratio of CD4+/CD8+ lymphocytes was found in lipopolysaccharide (LPS) challenged pigs (32). IR was reported to be related to the abnormal percentage of lymphocyte subsets in RPL (33, 34). A recent study revealed that a decrease of regulatory B cells (CD19+CD24hiCD38hi) in peripheral blood is associated with IR, and can increase the risk of postprandial hyperinsulinemia (35). Yan et al. also demonstrated that IR was associated with cellular immune abnormalities in RPL patients (34).
Taken together, we hypothesized that cellular immune abnormalities and/or abnormal fat metabolism might contribute to the pathophysiology of IR in RPL subjects without PCOS. To test our hypothesis, we investigated the relationship between fat metabolism and immune cell status in peripheral blood and homeostasis model assessment for insulin resistance (HOMA-IR) parameters in an RPL cohort with/without IR, independent of PCOS/obesity.
Materials and Methods
Participant Enrolment
Women were recruited at the first visit and were eligible for enrollment in this study if: (i) they were age less than 35 years with a body-mass index (BMI) no more than 27.9 kg/m2 (non-obesity reference value standards of BMI of Chinese), and (ii) had regular ovulatory menstrual cycles and were actively trying to conceive with a history of unexplained RPL (two or more consecutive pregnancy losses before 20 weeks of gestation). The diagnosis of pregnancy loss was confirmed by ultrasound. (iii) The current male partner had normal semen testing by computer-assisted analysis. Age criterion was applied because the likelihood of pregnancy loss, due to chromosomal abnormalities is higher in older women. Subjects with a verifiable cause of miscarriage were excluded (Figure 1). The exclusion criteria were: (i) abnormality of the uterus such as uterine septae and bicornuate uterus; (ii) karyotype abnormality in either the parents or the embryo. Karyotyping abnormity was defined as abnormal chromosome number (such as trisomy, polyploidy, and monosomy X) and abnormal chromosome structure (either a balanced reciprocal translocation or a Robertsonian translocation). (iii) luteal phase defect (diagnosed by combining basal body temperature and serum progesterone levels < 10 ng/mL) (36, 37), hyperprolactinemia (non-pregnant serum prolactin levels > 30 ng/mL), or hyperandrogenemia (serum testosterone levels > 0.7 ng/mL); (iv) with PCOS which was diagnosed by the presence of all following criteria: (menstrual irregularities, polycystic ovarian morphology (PCOM), and/or hyperandrogenemia). Moreover, women diagnosed with non-PCOS with hyperandrogenemia, ovulatory dysfunction, or PCOM are excluded for further analyses; (v) presence of autoantibodies including antinuclear antibodies (ANA), anticardiolipin antibodies (ACL), anti-Beta 2 glycoprotein and extractable nuclear antigens antibodies associated with systemic lupus erythematosus (SLE). The study protocol was approved by the Ethics Committees of Shanghai First Maternity and Infant Hospital (KS1812). Eighty-nine participants were recruited at the Department of Reproductive Immunology, Shanghai First Maternity and Infant Hospital between April 2018 and March 2019. Informed consent was obtained from all participants.
Oral Glucose Tolerance Test and Insulin Releasing Test
A 75 g oral glucose tolerance test (OGTT) was performed on each subject with plasma tested for glucose and insulin in a fasting state. Glucose levels were measured by a glucose oxidase-based assay (Roche Diagnostics GmbH, Mannheim, Germany). Insulin levels were measured by enzyme immunoassay (Roche Diagnostics GmbH, Mannheim, Germany). The plasma glucose levels at time points 0, 30, 60, 120, and 180 min were designated as Glu0, Glu30, Glu60, Glu120, and Glu180, and the insulin level at these time points were designated as Ins0, Ins30, Ins60, Ins120, and Ins180, respectively. In addition, the mean glucose and insulin levels during OGTT were designated as Glumean and Insmean, respectively.
Diagnosis
In this study, IR was diagnosed by the following indicators: 1) impaired glucose tolerance (IGT) or diabetic (38); 2) fasting insulin levels (FINS) ≥ 15 mIU/L (39); 3) HOMA-IR = Ins0 (µIU/ml) × Glu0 (mmol/L)/22.5 > 2.5 (40); and 4) normal FINS, but Ins180 level cannot be back to the normal level (41). The area under the curve (AUC) for glucose and insulin (GluAUC and InsAUC) was determined using the insulin secretion curve adjusted for glucose. The islet function and sensitivity index included the following: (i) a homeostasis model assessment of β cell function (HOMA-β) = [20 × Ins0 (µIU/ml)]/[Glu0 (mmol/L) − 3.5]; (ii) the early insulin secretion index (ΔI30/ΔG30) = [(Ins30 − Ins0) (µIU/ml)]/[(Glu30 − Glu0) (mmol/L)]; (iii) AUC for early-phase insulin secretion (InsAUC30/GluAUC30) = [(Ins0 + Ins30) (pmol/L)]/[(Glu0 + Glu30) (mmol/L)]; (iv) AUC for total insulin secretion (InsAUC120/GluAUC120) = [(Ins0+4×Ins30+3×Ins120) (pmol/L)]/[(Glu0+4×Glu30+ 3×Glu120) (mmol/L)]; (v) the Matsuda insulin sensitivity index (ISIM) = 10,000/[(Glu0 (mg/dl) × Ins0 (µIU/ml) × Glumean (mg/dl) × Insmean (uIU/ml)]0.5; (vi) the disposition index (DI) = HOMA−β/HOMA-IR, representing an adjusted insulin sensitivity according to HOMA-IR; vii) the early-phase disposition index (DI30) = InsAUC30/GluAUC30 × ISIM and the total disposition index (DI120) = InsAUC120/GluAUC120 × ISIM.
Blood Chemistry Assay
The morning fasting venous blood of all subjects was collected for routine clinical chemistry analysis. Serum lipid content, including total cholesterol (TCH), triglyceride (TG), and homocysteine (HCY) was measured using an enzymatic colorimetric assay (Beckman Coulter Inc., Brea, USA). Serum levels of insulin, in addition to thyroid function, including thyrotropin (TSH), free triiodothyronin (FT3), and free thyroxine (FT4) was measured by enzyme immunoassay (Roche Diagnostics GmbH, Mannheim, Germany).
Flow Cytometry Analysis for Lymphocyte Subsets
The lymphocyte subsets, including CD3+, CD3+CD4+, CD3+CD8+, CD19+, and CD16+CD56+ (NK) cells were analyzed by four-color flow cytometry. Venous blood was drawn into EDTA anticoagulant tubes and then 200 µL of the fresh whole blood samples were stained with monoclonal antibodies conjugated with fluorochromes fluorescein isothiocyanate [FITC], phycoerythrin [PE], PE cyanine 5 [PE-Cy 5], and allophycocyanin [APC] and incubated for 20 min in the dark at room temperature. Erythrocytes were lysed by FACS lysing solution (BD Biosciences) and then the blood sample was washed with PBS twice prior to flow cytometric analysis. A FACSCalibur flow cytometer with multiset software (BD Biosciences) was used to analyze the levels of subpopulations.
Statistical Analysis
All data are shown as means ± standard deviation (SD), and statistically analyzed using the SPSS 22.0 software package. Comparisons of continuous variables with normal distribution between the groups were performed using Student’s t-test, and Chi-square test was performed for categorical data analysis. Pearson’s correlation test was used to analyze the correlation between lipid profiles, the lymphocyte subsets, and insulin resistance by regarding as continuous variables. Multiple-factor regression analysis was performed for assessing the association between TG levels, hyperinsulinemia, and CD3+CD4+/CD3+CD8+ ratio. P < 0.05 was considered statistically significant.
Results
Characteristics of Participants.
According to the standard of IR, of the 89 RPL women, 56 participants were diagnosed with insulin resistance and defined as the IR group, and the remaining 33 were the non-IR group. The general characteristics of the study participants are presented in Table 1, which include the following epidemiological factors: age, BMI, education level, smoking status, alcohol-drinking status, reproductive tract virus infection, antibodies screen and medical history. The RPL women with IR exhibited significantly higher BMI compared with the non-IR group although all subjects’ BMI were in a healthy weight range. No significant differences were observed regarding other characteristics.
Comparison of β-Cell Function and Insulin Sensitivity Between IR and Non-IR Group
As shown in Table 2, HOMA-β was significantly higher in the IR group than the non-IR group. Patients with IR exhibited higher values of InsAUC30/GluAUC30 and InsAUC120/GluAUC120, which reflected higher early-phase insulin release and total insulin release, respectively, as opposed to the controls. In addition, RPL women with IR showed a significant decrease in Matsuda index, DI, DI30, and DI120 during OGTT, which indicates the reduced insulin sensitivity. Moreover, patients with IR manifested significantly higher glucose levels at 0, 60, 120, 180 min during OGTT in comparison with the controls, while the insulin levels were markedly higher during the entire period of the OGTT compared to the non-IR group (Figure 2).
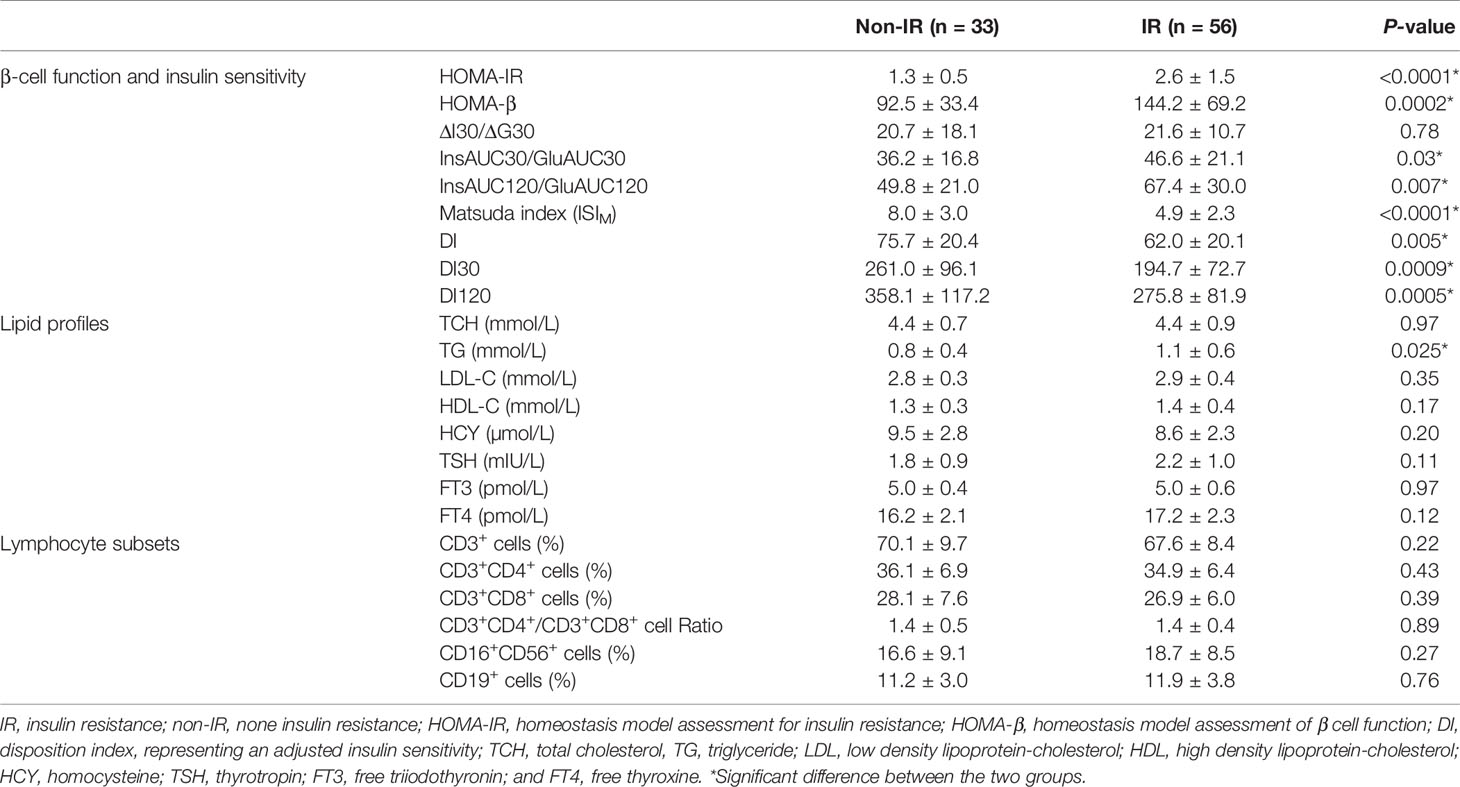
Table 2 Comparison of β-cell function and insulin sensitivity, lipid profiles, and lymphocyte subsets of RPL patient with IR and non-IR.
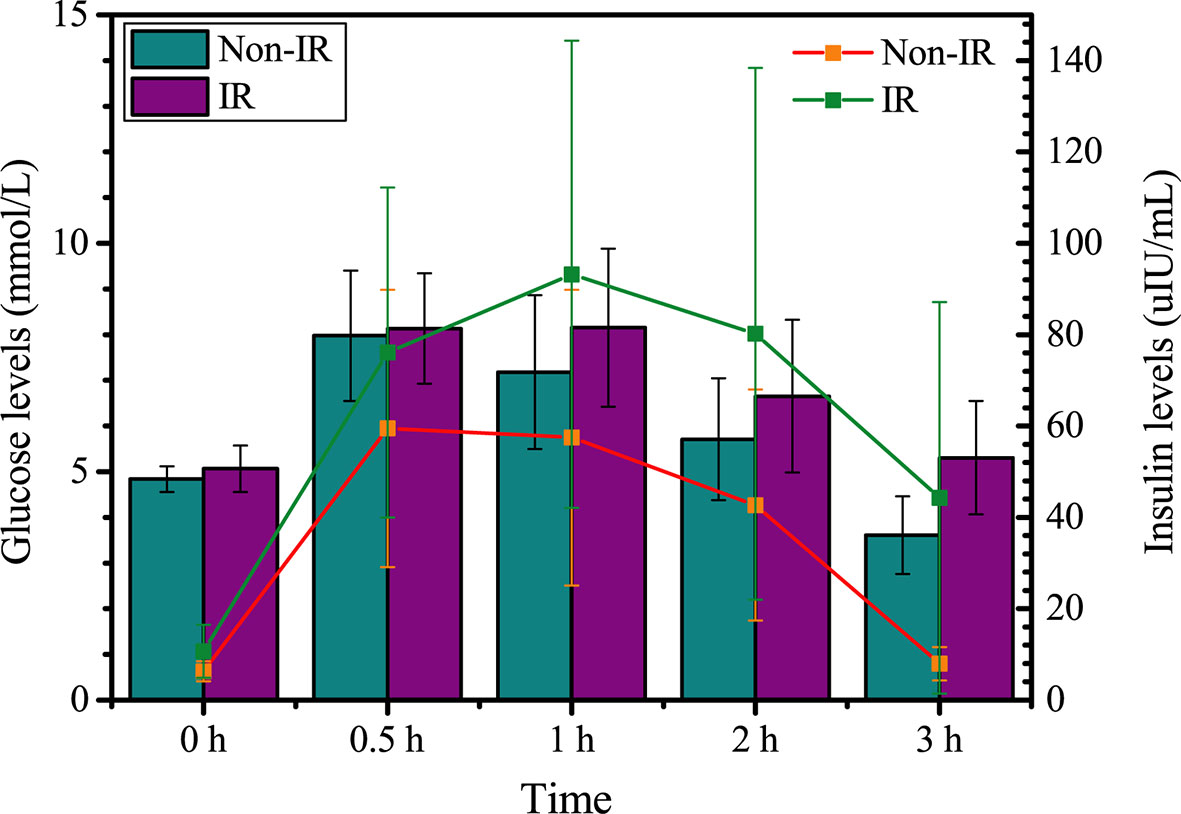
Figure 2 Glucose and insulin levels between insulin resistance group (IR) and non-insulin resistance group (Non-IR).
Association Between TG and Insulin Resistance or Insulin Sensitivity
In the measures of lipid profile, TG levels were significantly elevated in patients with IR (Table 2). Pearson linear correlation model revealed a significant positive association between TG and HOMA-IR, suggesting an increased possibility of insulin resistance as TG levels increased (Figure 3A). A significant negative association was also identified between TG and DI (Figure 3B), DI30 (Figure 3C), DI120 (Figure 3D), indicating that a high TG level was closely associated with a decrease in the insulin sensitivity. Furthermore, a receiver operating characteristic (ROC) curve analysis indicated that TGs were associated with HOMA-IR (Figure 3E), DI (Figure 3F), and DI120 (Figure 3G). Moreover, a significant negative association was identified between TSH and HOMA-IR, however, no significant association was found between TG and TSH (Supplementary Figure 1).
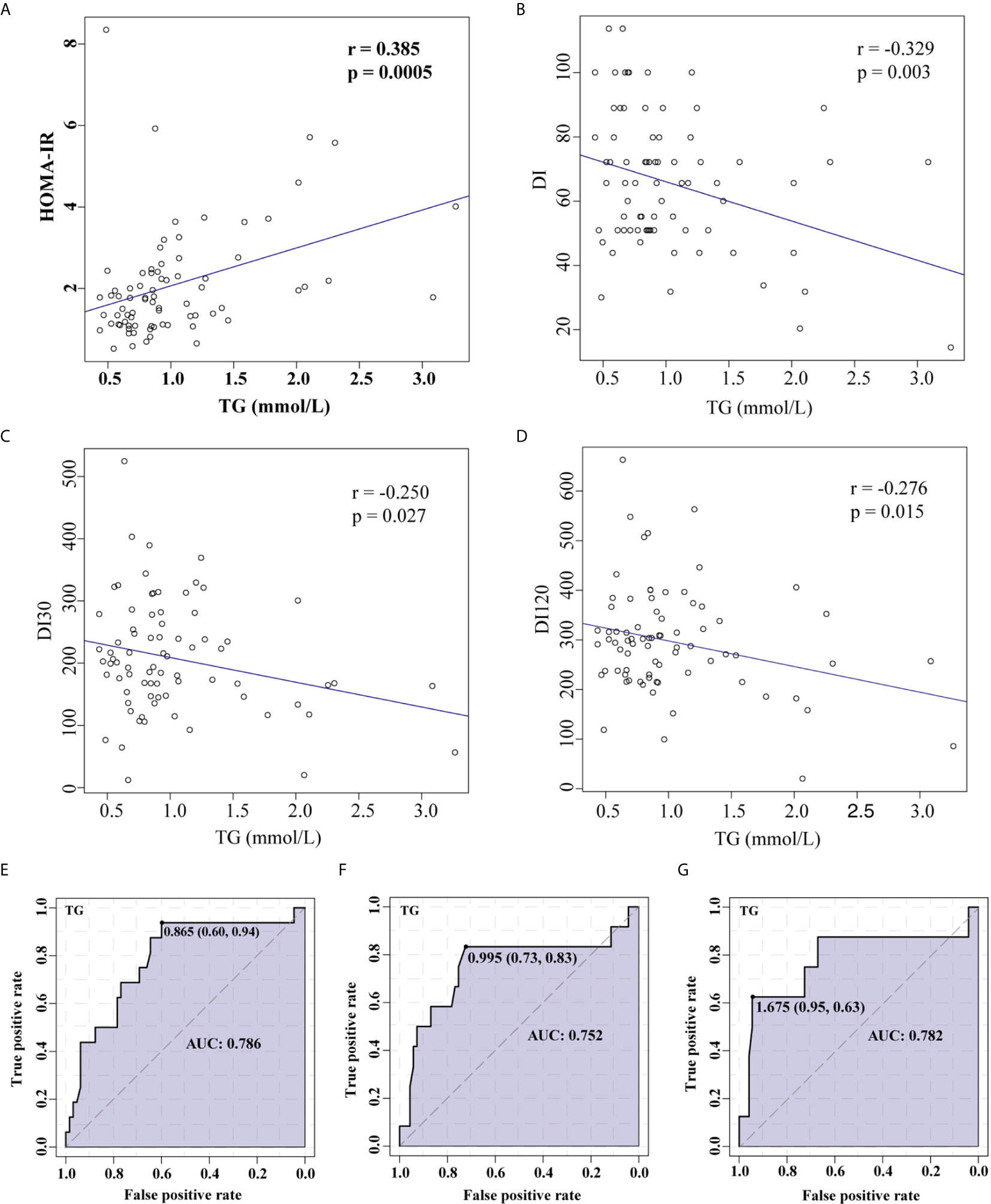
Figure 3 Correlation between TG levels and HOMA-IR or insulin sensitivity demonstrated using Pearson’s linear correlation model and ROC curve analysis. Association between TG and HOMA-IR (A), TG and DI (B), TG and DI30 (C), and TG and DI120 (D). ROC curve analysis for TGs and HOMA-IR (E), DI (F), and DI120 (G).TG, Triglyceride; HOMA-IR, homeostasis model assessment for insulin resistance; DI, disposition index, representing adjusted insulin sensitivity according to HOMA-IR.
An Increase in TG Levels Was Positively Associated With Hyperinsulinemia.
Hyperinsulinemia was defined as a fasting insulin ≥15 mU/L, and/or insulin levels at 2 h ≥80 mU/L during OGTT. In total, 25 enrollments were defined as hyperinsulinemia. Multivariate logistic regression analysis was performed to assess the association between TG and hyperinsulinemia in RPL patients. The results demonstrated that increase in TG was positively associated with hyperinsulinemia (p = 0.02). After adjusting for confounding factors including age, and BMI, there was a 2.77-fold higher risk of developing hyperinsulinemia with increasing TG levels (p = 0.03) (Table 3).
Increased TG Level Was Positively Associated With the Ratio of CD3+CD4+ to CD3+CD8+ Lymphocytes
The ratio of CD3+CD4+ to CD3+CD8+ lymphocytes (CD3+CD4+/CD3+CD8+) was calculated by using the percentages of CD3+CD4+ and CD3+CD8+lymphocyte cells. The ratio of < 1.0 was set as decreased group (N = 22) and those with a ratio ≥ 1.0 were normal group (N = 67) (42). Multivariate logistic regression analysis was performed to assess the association between increased levels of TG and CD3+CD4+/CD3+CD8+ ratio in RPL patients. The results showed that increase in TG was positively associated with an increased CD3+CD4+/CD3+CD8+ ratio (p = 0.02). After adjusting for confounding factors including age, and BMI, there was a 2.85-fold increase in the ratio with an increasing TG levels (p = 0.04) (Table 3).
Increased Ratio of CD3+CD4+ to CD3+CD8+ Lymphocytes Was Negatively Associated With DI30 and DI120
The RPL women with a lower CD3+CD4+/CD3+CD8+ ratio of < 1.0 were set as decreased group and those with a ratio ≥ 1.0 were normal group. As presented in Figure 4A, DI30 and DI120 were significantly decreased in the high CD3+CD4+/CD3+CD8+ group, and the ratio of CD3+CD4+/CD3+CD8+ was significantly negatively correlated with both DI30 (Figure 4B) and DI120 (Figure 4C). Moreover, ROC analysis indicated that CD3+CD4+/CD3+CD8+ were associated with DI30 (Figure 4D), and DI120 (Figure 4E), suggesting that the increased ratio of CD3+CD4+/CD3+CD8+ is accompanied by the reduced insulin sensitivity.
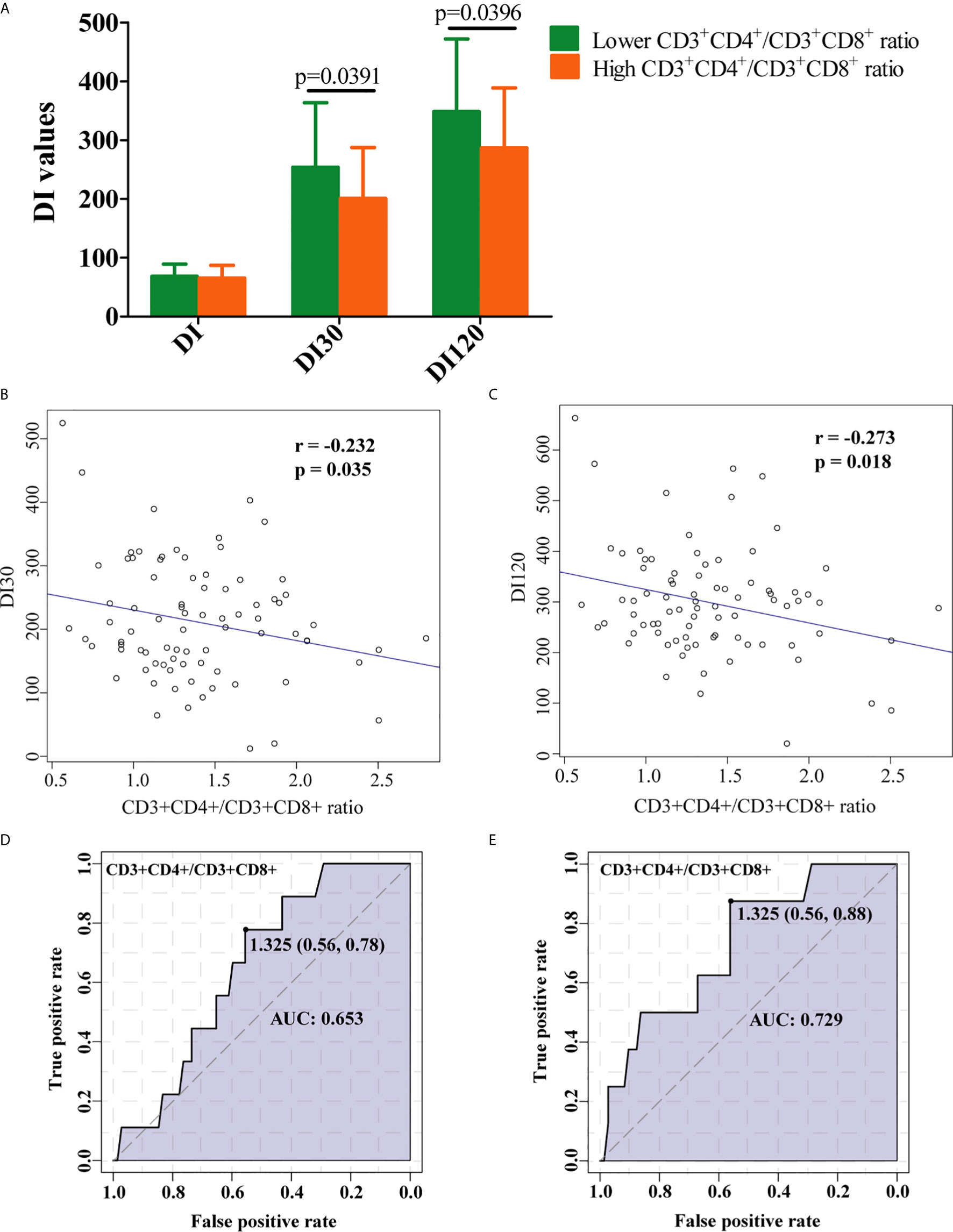
Figure 4 Clinical implication of CD3+CD4+/CD3+CD8+ ratio in RPL patients with IR. (A) RPL women with decreased CD3+CD4+/CD3+CD8+ ratio had significantly higher levels of DI30 and DI120. The increase in the ratio of CD3+CD4+/CD3+CD8+ was marked associated with low (B) DI30 and (C) DI120 levels. ROC curve analysis for CD3+CD4+/CD3+CD8+ ratio and DI30 (D), and DI120 (E). Data is presented as mean ± standard deviation. DI, disposition index, representing adjusted insulin sensitivity according to HOMA-IR.
Discussion
RPL has complex pathogenic mechanisms. Using a unique cohort of RPL patients without PCOS and obesity, we found increased TG levels in subjects with IR compared to those without IR. Moreover, the increased TG was positively associated with the ratio of CD3+CD4+/CD3+CD8+, and was accompanied by reduced insulin sensitivity and hyperinsulinemia. Thus, our study suggests that elevated TG and altered immune response are associated with insulin resistance in this cohort of RPL patients. Strategies to reduce the elevated TG and/or modulate immune system could be considered for treatments of these patients.
Insulin resistance is a condition in which the body fails to properly use insulin to regulate glucose metabolism. The accumulation of excessive insulin in the blood, described as hyperinsulinemia, with normal blood glucose levels indicates the presence of IR (43–45). Patients with PCOS and/or obese appear to have a higher prevalence of IR, and increased rate of pregnancy loss. Recently, it also has been reported that impaired insulin clearance and hyperinsulinemia could occur in non-obese Asians (20). However, the underlying correlative mechanisms between IR and RPL in patients without PCOS/obese remain inconclusive. To investigate the underlying mechanisms, we recruited a unique cohort of RPL patients without PCOS, suspected PCOS, and obese. The current study revealed that the glucose remained normal levels, however, the insulin levels at corresponding time points were significantly higher during OGTT in the IR group. Additionally, increased early-phase insulin release, total insulin release and lower insulin sensitivity indicated the occurrence of IR. Notably, the diagnose of IR in this study was determined based on a Chinese clinical expert consensus (46), which might be better representation for RPL patients in China. These appearance makes it more consistence to investigate the underlying mechanism between IR and RPL patients without PCOS and obese.
There is evidence that the higher fasting levels of TG are associated with IR (47, 48). These findings are consistent with the results of the present study. In this study, we found that higher TG levels were positively correlated with the risk of hyperinsulinemia in RPL women with and without adjustment of age and BMI. However, the underlying connection between higher TG levels and RPL patients with IR remained elusive. It has been demonstrated in mice that lymphocytes participate in the pathogenesis of IR (49). Yan et al. found that a far greater proportion of newly diagnosed RPL patients with IR had an aberrant CD3+CD4+, and CD56+CD16+ cell population compared to RPL patients without IR (34). Our study found that an increase in TG levels positively associated with an increased CD3+CD4+/CD3+CD8+ ratio. Furthermore, an inverse correlation between the CD3+CD4+/CD3+CD8+ ratio and DI values, reflecting insulin sensitivity were found, and increased CD3+CD4+/CD3+CD8+ is an indicator for hyperinsulinemia, revealing the involvement of CD3+CD4+/CD3+CD8+ in RPL patients with IR.
Previous studies indicated that an increased ratio of CD4+/CD8+ was positively correlated with tumor necrosis factor-α (TNF-α) level (50, 51). Notably, TG levels induced a high levels of plasma TNF-α by the increased production of very low density lipoprotein (VLDL) particles (52). And increased TNF-α expression contribute to decreased adiponectin, which plays as an important modulator of insulin sensitivity via the downregulation of the peroxisome proliferator activated receptor-α (PPAR-α) (53, 54). From our observations, we postulate that high TG levels induce IR in RPL patients via the elevated CD3+CD4+/CD3+CD8+ ratio. One possible hypothesis is elevated TG induce aberrant lymphocyte subsets, including increased CD3+CD4+/CD3+CD8+, which serves a proinflammatory role by producing TNF-α, leading to reduced adiponectin production and blocking activation of PPAR-α, thereby resulting in decreased insulin sensitivity. All these findings indicate that higher TG levels are associated with the development of IR.
The strengths of this study include: (i) thoroughly consideration of the exclusion criteria for common causes of pregnancy loss such as uterine abnormalities, karyotype abnormality, luteal phase defect, and abnormal antibodies; (ii) an RPL cohort with and without IR independent of PCOS and abnormal BMI. Several limitations were associated with our study: (i) some information related to IR was not obtained, such as the free fatty acid levels, the detail of regular physical exercise, and family history of diabetes; (ii) this study was a cross-sectional design that made it difficult to explore the causal relationship between TG, CD3+CD4+/CD3+CD8+ ratio and IR. (iii) there is lack of racial diversity in the studied cohort. It was reported that ethnic differences in TG levels should be considered for identifying insulin resistance (55). Tamura et al., reported that impaired insulin clearance and hyperinsulinemia could occur in non-obese Asians and decreased insulin sensitivity in muscle was well correlated with elevated TG levels in non-obese Asians (20). It is possible that TG can be used as an indicator of IR in RPL patients with racial and ethnic disparities. Finally, future studies with diverse populations and large survey samples are warranted.
In conclusion, the results of the present study demonstrated significant increases in the early phase insulin secretion and total insulin secretion during OGTT and an increased TG levels in RPL patients with IR. The increased serum level of TG was positively associated with postprandial hyperinsulinemia. The role of CD3+CD4+/CD3+CD8+ ratio in insulin resistance contributes to the associated interference with islet compensation function. Further investigation is required to determine if CD3+CD4+/CD3+CD8+ ratio is able to act as immune indicators of insulin resistance and islet β-cell function in RPL patients.
Data Availability Statement
The raw data supporting the conclusions of this article will be made available by the authors, without undue reservation.
Ethics Statement
The studies involving human participants were reviewed and approved by the Ethics Committees of Shanghai First Maternity and Infant Hospital. The patients/participants provided their written informed consent to participate in this study.
Author Contributions
LF and YL conceived and designed the experiments. MD, YG, and YL performed sample collection. YL performed experimental processing of the samples and analyzed the data. YL wrote the manuscript, and LF revised the manuscript. SB, LF, and JZ contributed to discussions and reviewed the paper, and gave their approval to the final version of the manuscript. All authors contributed to the article and approved the submitted version.
Funding
This work was supported by the National Natural Science Foundation of China (81903325), and the China Postdoctoral Science Foundation (2018M632138).
Conflict of Interest
The authors declare that the research was conducted in the absence of any commercial or financial relationships that could be construed as a potential conflict of interest.
Acknowledgments
We thank obstetrician Mengyang Du, Guohua Li, and Na Yu (Department of Reproductive Immunology, Shanghai First Maternity and Infant Hospital, Shanghai, China) for helpful sampling assistance.
Supplementary Material
The Supplementary Material for this article can be found online at: https://www.frontiersin.org/articles/10.3389/fendo.2021.621845/full#supplementary-material
Supplementary Figure 1 | Correlation analyses between lipid profiles, the lymphocyte subsets and insulin resistance. HOMA-IR, homeostasis model assessment for insulin resistance; HOMA-β, homeostasis model assessment of β cell function; ISIM, Matsuda insulin sensitivity index; DI, disposition index, representing an adjusted insulin sensitivity; TCH, total cholesterol; TG, triglyceride; TSH, thyrotropin; * indicates p < 0.05, ** indicates p < 0.01, *** indicates p < 0.0001.
References
1. Hertz-Picciotto I, Samuels S. Incidence of early loss of pregnancy. N Engl J Med (1988) 319(22):1483–4. doi: 10.1056/NEJM198812013192214
2. Rai R, Regan L. Recurrent miscarriage. Lancet (2006) 368(9535):601–11. doi: 10.1016/S0140-6736(06)69204-0
3. Branch D, Gibson M, Silver R. Recurrent Miscarriage. N Engl J Med (2010) 363:1740–7. doi: 10.1056/NEJMcp1005330
4. Bender Atik R, Christiansen OB, Elson J, Kolte AM, Lewis S, Middeldorp S, et al. ESHRE guideline: recurrent pregnancy loss. Hum Reprod Open (2018) 2018(2):hoy004. doi: 10.1093/hropen/hoy004
5. Kaur R, Gupta K. Endocrine dysfunction and recurrent spontaneous abortion: An overview. Int J Appl Basic Med Res (2016) 6(2):79–83. doi: 10.4103/2229-516X.179024
6. Medicine PCotASfR. Evaluation and treatment of recurrent pregnancy loss: a committee opinion. Fertil Steril (2012) 98(1556-5653(1556-5653 (Electronic):1103–11. doi: 10.1016/j.fertnstert.2012.06.048
7. Pereza N, Ostojić S, Kapović M, Peterlin B. Systematic review and meta-analysis of genetic association studies in idiopathic recurrent spontaneous abortion. Fertil Steril (2016) 107(1):S0015028216629242. doi: 10.1016/j.fertnstert.2016.10.007
8. Murugappan G, Vyas NM, Lathi RB. Karyotype of first miscarriage is prognostic of subsequent pregnancy outcome. Fertil Steril (2019) 111(4, Supplement):e11–2. doi: 10.1016/j.fertnstert.2019.02.048
9. Rajani S, Mukherjee S, Sharma S, Sarkar HS, Goswami SK, Chakravarty B. Insulin resistance, homocysteine & pregnancy loss in polycystic ovarian syndrome (PCOS): are they related? Fertil Steril (2010) 94(4):S194–4. doi: 10.1016/j.fertnstert.2010.07.754
10. Chen L, Xu WM, Zhang D. Association of abdominal obesity, insulin resistance, and oxidative stress in adipose tissue in women with polycystic ovary syndrome. Fertil Steril (2014) 102(4):1167–74.e1164. doi: 10.1016/j.fertnstert.2014.06.027
11. Goodarzi MO, Korenman SG. The importance of insulin resistance in polycystic ovary syndrome. Fertil Steril (2003) 80(0015-0282(0015-0282 (Print):255–8. doi: 10.1016/S0015-0282(03)00734-9
12. Rai R, Backos M, Rushworth F, Regan L. Polycystic ovaries and recurrent miscarriage–a reappraisal. Hum Reprod (2000) 15(3):612–5. doi: 10.1093/humrep/15.3.612
13. Hong Y, Xie QX, Chen CY, Yang C, Li YZ, Chen DM, et al. Insulin resistance in first-trimester pregnant women with pre-pregnant glucose tolerance and history of recurrent spontaneous abortion. J Biol Regul Homeost Agents (2013) 27(1):225–31. doi: 10.7868/S004446771301005X
14. Banu J, Fatima P, Sultana P, Chowdhury MA, Begum N, Anwary SA, et al. Association of infertile patients having polycystic ovarian syndrome with recurrent miscarriage. Mymen Med J (2014) 23(4):770–3.
15. Ghaffarzad A, Amani R, Darabi M, Cheraghian B. Correlation of Serum Lipoprotein Ratios with Insulin Resistance in Infertile Women with Polycystic Ovarian Syndrome: A Case Control Study. Int J Fertil Steril (2016) 10(1):29–35. doi: 10.22074/ijfs.2016.4765
16. Craig LB, Ke RW, Kutteh WH. Increased prevalence of insulin resistance in women with a history of recurrent pregnancy loss. Fertil Steril (2002) 78(3):487–90. doi: 10.1016/S0015-0282(02)03247-8
17. Ispasoiu CA, Chicea R, Stamatian FV, Ispasoiu F. High fasting insulin levels and insulin resistance may be linked to idiopathic recurrent pregnancy loss: a case-control study. Int J Endocrinol (2013) 2013(2013):576926. doi: 10.1155/2013/576926
18. Chan J, Malik V, Jia W, Kadowaki T, Yajnik C, Yoon K, et al. Diabetes in Asia: epidemiology, risk factors, and pathophysiology. JAMA (2009) 301(20):2129–40. doi: 10.1001/jama.2009.726
19. Pan W, Yeh W, Weng L. Epidemiology of metabolic syndrome in Asia. Asia Pac J Clin Nutr (2008) 17(Suppl 1):37–42.
20. Tamura Y. Ectopic fat, insulin resistance and metabolic disease in non-obese Asians: investigating metabolic gradation. Endocr J (2019) 66(1):1–9. doi: 10.1507/endocrj.EJ18-0435
21. Fougner KJ, Vanky E, Carlsen SM. Metformin has no major effects on glucose homeostasis in pregnant women with PCOS: results of a randomized double-blind study. Scand J Clin Lab Inv (2008) 68(8):771–6. doi: 10.1080/00365510802254620
22. Song J, Ruan X, Gu M, Wang L, Wang H, Mueck AO. Effect of orlistat or metformin in overweight and obese polycystic ovary syndrome patients with insulin resistance. Gynecol Endocrinol (2018) 34(5):413–7. doi: 10.1080/09513590.2017.1407752
23. Xu H, Barnes GT, Yang Q, Tan G, Daseng Y, Chou CJ, et al. Chronic inflammation in fat plays a crucial role in the development of obesity-related insulin resistance. J Clin Invest (2003) 112(12):1821–30. doi: 10.1172/JCI200319451
24. Sáinz N, González-Navarro CJ, Martínez JA, Moreno-Aliaga MJ. Leptin signaling as a therapeutic target of obesity. Expert Opin Ther Tar (2015) 19(7):1–17. doi: 10.1517/14728222.2015.1018824
25. Jin Y, Wu Y, Zeng Z, Jin C, Wu S, Wang Y, et al. From the Cover: Exposure to Oral Antibiotics Induces Gut Microbiota Dysbiosis Associated with Lipid Metabolism Dysfunction and Low-Grade Inflammation in Mice. Toxicol Sci (2016) 154(1):140. doi: 10.1093/toxsci/kfw150
26. Elhady M, Elazab AAAM, Bahagat KA, Abdallah NA, Ibrahim ES. Fatty pancreas in relation to insulin resistance and metabolic syndrome in children with obesity. J Pediatr Endocr Met (2019) 32:19–26. doi: 10.1515/jpem-2018-0315
27. Patel S. Polycystic ovary syndrome (PCOS), an inflammatory, systemic, lifestyle endocrinopathy. J Steroid Biochem (2018) 182:27–36. doi: 10.1016/j.jsbmb.2018.04.008
28. Ketefian A, Amin M, Manning D, Azziz R, Pisarska M, Alexander C. Triglyceride to high-density lipoprotein cholesterol ratio as a predictor of insulin resistance in women with polycystic ovary syndrome (PCOS). Fertil Steril (2011) 96(3):S129. doi: 10.1016/j.fertnstert.2011.07.503
29. Liu YL, Li DF, Gong LM, Yi GF, Gaines AM, Carroll JA. Effects of fish oil supplementation on the performance and the immunological, adrenal, and somatotropic responses of weaned pigs after an lipopolysaccharide challenge. J Anim Sci (2003) 81(11):2758–65. doi: 10.2527/2003.81112758x
30. Romualdo MCDS, Nóbrega FJD. Insulin resistance in obese children and adolescents. J Pediat Brazil (2014) 90(6):600–7. doi: 10.1016/j.jped.2014.03.005
31. Zhu LY, Chen X, Xu ZZ, Xu L, Mao T, Zhang H. Changes and clinical significance of peripheral blood helper T lymphocyte and natural killer (NK) cells in unexplained recurrent spontaneous abortion (URSA) patients after abortion and successful pregnancy. Clin Exp Obstet Gyn (2015) 42(1):62–6.
32. Lai C JY, Li D, Zhao L, Chen X. Effects of dietary conjugated linoleic acid supplementation on performance and immune function of weaned pigs. Arch Anim Nutr (2005) 59(1745-039X(1745-039X (Print):41–51. doi: 10.1080/17450390512331342377
33. Kanninen TT, Bruna Ribeiro DAR, Shirlee J, Ann Marie B, Iara Moreno L, Renzo GCD, et al. Inhibition of autophagy by sera from pregnant women. Reprod Sci (2013) 20(11):1327–31. doi: 10.1177/1933719113485301
34. Yan Y, Bao S, Sheng S, Wang L, Tu W. Insulin resistance in patients with recurrent pregnancy loss is associated with lymphocyte population aberration. Syst Biol Reprod Med (2017) 63(6):397–404. doi: 10.1080/19396368.2017.1378936
35. Yang M, Du C, Wang Y, Liu J. CD19(+)CD24(hi)CD38(hi) regulatory B cells are associated with insulin resistance in type I Hashimoto’s thyroiditis in Chinese females. Exp Ther Med (2017) 14(4):3887–93. doi: 10.3892/etm.2017.4925
36. Jordan J, Craig K, Clifton D, Soules M. Luteal phase defect: the sensitivity and specificity of diagnostic methods in common clinical use. Fertil Steril (1994) 62(1):54–62. doi: 10.1016/S0015-0282(16)56815-0
37. Czyzyk A, Podfigurna A, Genazzani A, Meczekalski B. The role of progesterone therapy in early pregnancy: from physiological role to therapeutic utility. Gynecol Endocrinol (2017) 33(6):421–4. doi: 10.1080/09513590.2017.1291615
38. Kim J, Bacha F, Tfayli H, Michaliszyn S, Yousuf S, Arslanian S. Adipose Tissue Insulin Resistance in Youth on the Spectrum From Normal Weight to Obese and From Normal Glucose Tolerance to Impaired Glucose Tolerance to Type 2 Diabetes. Diabetes Care (2019) 42(2):265–72. doi: 10.2337/dc18-1178
39. Tohidi M, Ghasemi A, Hadaegh F, Derakhshan A, Chary A, Azizi F. Age- and sex-specific reference values for fasting serum insulin levels and insulin resistance/sensitivity indices in healthy Iranian adults: Tehran Lipid and Glucose Study. Clin Biochem (2014) 47(6):432–8. doi: 10.1016/j.clinbiochem.2014.02.007
40. DeUgarte C, Bartolucci A, Azziz R. Prevalence of insulin resistance in the polycystic ovary syndrome using the homeostasis model assessment. Fertil Steril (2005) 83(5):1454–60. doi: 10.1016/j.fertnstert.2004.11.070
41. Jinfang L, Xin L, Su C. Diagnosis and treatment of insulin resistance in patients with polycystic ovary syndrome. Chin J Pract Gynecolo Obstet (2007) 09):663–7. doi: 1005-2216(2007)09-0667-03
42. Chen X, Jl Y, Ye J. Analysis of peripheral blood lymphocyte subsets and prognosis in patients with septic shock. Microbiol Immunol (2011) 55(1348-0421(1348-0421 (Electronic):736–42. doi: 10.1111/j.1348-0421.2011.00373.x
43. Yang X, Quan X, Lan Y, Wei Q, Ye J, Yin X, et al. Serum chemerin level in women with PCOS and its relation with the risk of spontaneous abortion. Gynecol Endocrinol (2018) 34(10):864–7. doi: 10.1080/09513590.2018.1462316
44. Cefalu WT. Insulin resistance: cellular and clinical concepts. Exp Biol Med (2001) 226(1):13–26. doi: 10.1177/153537020122600103
45. Reaven GM. Insulin resistance, cardiovascular disease, and the metabolic syndrome: how well do the emperor’s clothes fit? Diabetes Care (2004) 27(4):1011–2. doi: 10.2337/diacare.27.4.1011
46. Association EamboCmd. Consensus of endocrinologists on diagnosis and treatment of polycystic ovary syndrome. Chin J Endocrinol Metab (2018) 34(1):1–7. doi: 10.3760/cma.j.Łissn.1000-6699.2018.01.001
47. Yeh WC, Tsao YC, Li WC, Tzeng IS, Chen LS, Chen JY. Elevated triglyceride-to-HDL cholesterol ratio is an indicator for insulin resistance in middle-aged and elderly Taiwanese population: a cross-sectional study. Lipids Health Dis (2019) 18(1):176. doi: 10.1186/s12944-019-1123-3
48. Martins LM, Oliveira ARS, Cruz KJC, Torres-Leal FL, Marreiro D. Obesity, inflammation, and insulin resistance. Braz J Pharm Sci (2014) 50(4):677–92. doi: 10.1590/S1984-82502014000400003
49. Solano ME, Sander VA, Ho H, Motta AB, Arck PC. Systemic inflammation, cellular influx and up-regulation of ovarian VCAM-1 expression in a mouse model of polycystic ovary syndrome (PCOS). J Reprod Immunol (2011) 92(1):33–44. doi: 10.1016/j.jri.2011.09.003
50. Moodley Y, Dorasamy T, Venketasamy S, Naicker V, Lalloo U. Correlation of CD4:CD8 ratio and tumour necrosis factor (TNF)alpha levels in induced sputum with bronchoalveolar lavage fluid in pulmonary sarcoidosis. Thorax (2000) 55(8):696–9. doi: 10.1136/thorax.55.8.696
51. Wang K, Wang DC, Feng YQ, Xiang-Feng L. Changes in cytokine levels and CD4+/CD8+ T cells ratio in draining lymph node of burn wound. J Burn Care Res (2007) 28(5):747–53. doi: 10.1097/BCR.0B013E318148C95A
52. Deng Y, Scherer P. Adipokines as novel biomarkers and regulators of the metabolic syndrome. Ann NY Acad Sci (2010) 1212:E1–19. doi: 10.1111/j.1749-6632.2010.05875.x
53. Hajri T, Tao H, Wattacheril J, Marks-Shulman P, Abumrad N. Regulation of adiponectin production by insulin: interactions with tumor necrosis factor-α and interleukin-6. Am J Physiol Endoc M (2011) 300(2):E350–60. doi: 10.1152/ajpendo.00307.2010
54. Yamauchi T, Kamon J, Minokoshi Y, Ito Y, Waki H, Uchida S, et al. Adiponectin stimulates glucose utilization and fatty-acid oxidation by activating AMP-activated protein kinase. Nat Med (2002) 8(11):1288–95. doi: 10.1038/nm788
Keywords: triglyceride, CD3+CD4+/CD3+CD8+ ratio, insulin resistance, recurrent pregnancy loss, insulin sensitivity
Citation: Liu Y, Du M, Gan Y, Bao S, Feng L and Zhang J (2021) Triglyceride Induced Metabolic Inflammation: Potential Connection of Insulin Resistance and Recurrent Pregnancy Loss. Front. Endocrinol. 12:621845. doi: 10.3389/fendo.2021.621845
Received: 27 October 2020; Accepted: 22 March 2021;
Published: 15 April 2021.
Edited by:
Qiongjie Zhou, Fudan University, ChinaReviewed by:
Kok-Min Seow, Shin Kong Wu Ho-Su Memorial Hospital, TaiwanYimin Zhu, Zhejiang University, China
Copyright © 2021 Liu, Du, Gan, Bao, Feng and Zhang. This is an open-access article distributed under the terms of the Creative Commons Attribution License (CC BY). The use, distribution or reproduction in other forums is permitted, provided the original author(s) and the copyright owner(s) are credited and that the original publication in this journal is cited, in accordance with accepted academic practice. No use, distribution or reproduction is permitted which does not comply with these terms.
*Correspondence: Liping Feng, liping.feng@duke.edu; Shihua Bao, baoshihua@tongji.edu.cn; Jun Zhang, junjimzhang@sina.com