- 1Department of Biology, University of Winnipeg, Winnipeg, MB, Canada
- 2Department of Immunology, University of Manitoba, Winnipeg, MB, Canada
Introduction: Insulin-like peptide 5 (INSL5) is a peptide hormone with proposed actions in glucose homeostasis and appetite regulation via its cognate receptor, relaxin family peptide receptor 4 (RXFP4). Here, we look for evidence for their involvement in the immune system using a mouse model.
Methods: In silico analyses: we queried public databases for evidence of expression of INSL5-RXFP4 in immune system tissues/cells (NCBI’s SRA and GeoProfiles) and disorders (EMBO-EBI) and performed phylogenetic footprinting to look for evidence that they are regulated by immune-associated transcription factors (TFs). Experimental analyses: We characterized the expression and correlation of INSL5/RXFP4 and other immune system markers in central and peripheral immune organs from C57/bl6 mice in seven cohorts. We tested whether fluctuations in circulating INSL5 induce an immune response, by injecting mice with 30 μg/kg of INSL5 peptide in the peritoneum, and examining levels of immune markers and metabolic peptides in plasma. Lastly, we quantified the expression of Rxfp4 in T-cells, dendritic cells and cell lines derived from human and mouse and tested the hypothesis that co-incubation of ANA-1 cells in INSL5 and LPS alters cytokine expression.
Results: We find Insl5 expression only in thymus (in addition to colon) where its expression was highly correlated with Il-7, a marker of thymocyte development. This result is consistent with our in silico findings that Insl5 is highly expressed in thymic DP, DN thymocytes and cortical TEC’s, and with evidence that it is regulated by thymocyte-associated TF’s. We find Rxfp4 expression in all immune organs, and moderately high levels in DCs, particularly splenic DCs, and evidence that it is regulated by immune-associated TF’s, such as STAT’s and GATA. Systemic effects: We observed significantly elevated concentrations of blood GLP-1, GIP, GCG and PYY following intraperitoneal injection of INSL5, and significantly altered expression of cytokines IL-5, IL-7, M-CSF, IL-15, IL-27 and MIP-2. Immune cell effects: Incubation of ANA-1 cells with INSL5 impeded cell growth and led to a transient elevation of IL-15 and sustained reduction in IL-1β, IL-6 and TNFα.
Conclusion: We propose that INSL5-RXFP4 play a novel role in both central and peripheral immune cell signaling.
Introduction
Insulin like peptide 5 (INSL5) is a novel peptide hormone belonging to the relaxin (RLN)/INS/IGF superfamily that plays roles in neuroendocrine processes via its cognate receptor, relaxin family peptide receptor 4 (RXFP4) (1–3). Primary tissues of expression for Insl5 are the L-cells of the distal colon, hypothalamus, kidney, thymus and reproductive tissues; while Rxfp4 is expressed in the colon and subtending vagus nerve efferents, as well as in the cerebellum, reproductive tissues and kidney: (4, 5) GTEX consortium). Collectively these tissues of expression correlate well with current hypotheses that INSL5 a) is a gut hormone that modulates glucose metabolism either directly or indirectly via gluconeogenesis (6–8); b) is an orexigenic hormone that influences satiety, through both gut-nervous system and hypothalamus-cerebellum cross-talk with RXFP4 (9); c) plays roles in female and male fertility (6, 10, 11), and d) together with RXFP4, are markers of colorectal and breast cancer progression (12–15).
Since the discovery of INSL5 in 1999, there have been a few indications that it may also play a role in the immune system. For example, Insl5 and/or Rxfp4 transcripts have been found in immune system tissues such as thymus, leukocytes, bone marrow and lymph nodes (5), and a study in lean and obese men and women, found a negative correlation of INSL5 with the pro-inflammatory biomarker C-reactive protein (CRP) (16). Here, we explore, for the first time, the hypothesis that INSL5 and RXFP4 play roles in the immune system using both in silico and experimental methods. To this end, we mined public databases for evidence of the expression of Insl5/Rxfp4 in immune tissues/cells and performed phylogenetic footprinting to look for evidence that they are regulated by immune-associated transcription factors (TFs). Next, we used experimental methods to test the hypothesis that Insl5/Rxfp4 are expressed in both central and peripheral immune tissues. To this end, we quantified expression of Insl5 and Rxfp4 using qPCR in central and peripheral immune tissues (thymus, blood, bone marrow, colon and spleen) in C57/Bl6 mice stratified by age (from 3 weeks to >12 months), and tested the hypothesis that they exhibit age-dependent expression in thymus by examining both their change in expression over age cohorts and the correlation of their expression with other genes known to be involved in thymus development or involution.
Finding evidence of broad expression of Rxfp4 in immune tissues and subsets, we moved to an in vivo model and tested whether intraperitoneal (i.p.) injection of INSL5 in C57/Bl6 mice resulted in changes in the levels of circulating immune system mediators, and/or metabolic peptides, given the known insulinotropic effects of INSL5. Finally, we looked at the cellular level, and hypothesized that INSL5 may convey signals through RXFP4 expression on major immune cell subsets. Accordingly, we assessed the expression of Rxfp4 in CD4+ T-cells and bone-marrow derived and splenic DCs (BMDC, sDCs), as well as in five cell lines derived from human and mouse tissues and then performed an experiment in which we pre-incubated mouse ANA-1 bone-marrow derived macrophages (BMDM) in INSL5 for up to 18 h, and then stimulated cells with LPS and screened for expression of focal cytokines.
Our results indicate a broader expression of Insl5/Rxfp4 in immune system tissues and cells than previously described. We find evidence that Insl5 plays a role in the development of thymocytes based on in silico datamining of RNA-seq datasets and phylogenetic footprinting analyses and from experimental data that show a correlation of Insl5 and Il-7 in age-stratified cohorts of C57/Bl6 mice. In the peripheral immune system, we show that Rxfp4 is expressed in diverse immune cell subsets of the innate immune system, particularly splenic dendritic cells, and find that INSL5 treatment alters levels of pro-and anti-inflammatory cytokines both in vitro and in vivo; most notably a decrease in pro-inflammatory cytokines IL-6 and IL-1β. Thus, we conclude that INSL5-RXFP4 play a novel role in the immune system.
Methods
In Silico Analyses
NCBI-SRA: We used the human and mouse full length coding sequences (Human Insl5 ENST00000304526, Human Rxfp4 ENST00000368318; Mouse Insl5, ENSMUST00000106869 Mouse Rxfp4 ENST00000368318), to query immune-relevant RNA seq datasets in the sequence read archive (SRA) for relevant tissues and cellular subsets (www.ncbi.nlm.nih/SRA). Only experiments for which fragments from the full gene length were identified in at least 5 experiments were counted as positive hits. Phylogenetic footprinting: We employed phylogenetic footprinting to identify potential transcription factor binding sites (TFBS’s) in the regulation of Insl5 and Rxfp4 by scanning promoter/intronic/UTR regions of each gene with positional weight matrices (PWMs) of known TF’s (17, 18). Putative regulatory regions were identified by aligning 1,000 bp upstream, the intron and 3’ UTR for Insl5 or 3,000 bp upstream for Rxfp4 for both human and mouse using mVISTA’s Shuffle-LAGAN glocal alignment algorithm (19, 20) and viewed using a 100 bp sliding window and a 70% conservation threshold on a percent identity plot (PIP) (17, 21). Conserved DNA sequences were extracted and submitted to PROMO [http://alggen.lsi.upc.es/cgi-bin/promo_v3/promo/promoinit.cgi?dirDB=TF_8.3], to identify potential TFBS’s based on the TRANSFAC database (22–24), and candidate TF’s were grouped into nine biological categories (25) ding - those with immune-related functions were selected for further analyses. Search for pan-vertebrate conservation of immune-related TFBS’s and experimental support of TF’s from the ENCODE project. The union of all immune-associated TF’s predicted to be in regulatory regions for Insl5 or Rxfp4 were used as queries for the pan-vertebrate TFBS finder tool Contra V3 using a core and similarity stringency of 0.95 and 0.85 (26). The data were then visualized in the UCSC genome browser (http://genome.ucsc.edu) with the phastConsElements100 vertebrate alignment table and the ENCODE regulation tracks for H3K4Me1 marks (open chromatin), DNase I hypersensitivity clusters and confirmed Chip-Seq TFBS’s of one of the 181 TF’s in one or more cell lines interrogated by the ENCODE consortium (27).
Experimental Work
Animals: The effect of aging on Insl5/Rxfp4 gene and protein expression was determined by qPCR since no validated antibody is available for purchase. For this purpose, 22 C57BL/6 strain (C57BL/6NCrl, Charles River Laboratories, Wilmington, MA) mice were used, 19 belonged to cohorts between three and 16 weeks old, and three were retired breeders aged between 52–78 weeks. Mice were held at the University of Winnipeg animal care facility under standard protocol of housing, feeding and euthanasia. Following euthanasia, mice were dissected and samples of thymus, blood (drawn from the left ventricle), bone marrow (flushed out from tibia with 1X sterile PBS, and then centrifuged at 3,000 g for 10 min), liver, spleen, colon, and testes were bi-sectioned and flash frozen in liquid nitrogen. For the cell line experiments on mouse bone marrow derived (BMDCs) and splenic dendritic cells (sDCs), 8–10-week old C57BL6 male mice were used and housed in University of Manitoba animal care facility. All experiments were carried out in accordance with UACC guidelines and regulations, as described in the animal care protocols for the mice at University of Winnipeg (protocol no. 00284/04606) and the University of Manitoba (protocol no. 14-014) following approval by the Animal Care committees at both institutions.
Insl5/Rxfp4 Expression in Mouse Tissues
The expression of Insl5 and Rxfp4 across age cohort and in different tissues was assessed using qPCR. Total RNA was extracted from colon, thymus, and spleen using the RNeasy Plus Universal Mini Kit (QIAgen) following the manufacturer’s protocol, from blood and bone marrow using a phenol-chloroform protocol described in (28), and from cell culture using Trizol as per the manufacturers protocol (ThermoFisher). cDNA was synthesized using 2 μg of total RNA for tissues or 1 μg of RNA for cell culture samples, using Superscript III (ThermoFisher) and random primers (Bio-Rad) in a final reaction volume of 20 ul, and diluted to 10 ng RNA/μl and stored at -20°C until use. Quantitative PCR was performed using FAM-labelled probes (Bio-Rad) for our focal genes Insl5, Rxfp4, and for Ghr and Foxn1 (Ensembl IDs: ENSMUSG00000066090, ENSMUSG00000049741, ENSMUSG00000055737, ENSMUSG00000002057 respectively), which are associated with maintenance of thymic architecture (29–31). Additionally, we screened for expression of cytokine lL-7, which is involved in the development/selection of thymocytes between double-negative (DN) and double positive (DP) stages in the thymic cortex and differentiation of T-cells in the thymic medula using primers sequences obtained from Ortman et al. (32). Two reference genes were employed, Ubc-2 and 18S rRNA using published primers, which have been shown to exhibit stable expression across immune system tissues and cell types (33, 34) (listed in Table S1). The efficiency and specificity of the qPCR primers and probes were validated using gel electrophoresis, qPCR melt curve visualization and DNA sequencing of PCR products [see (28)]. For qPCR, 10 μl reaction volumes were prepared using SsoAdvanced Universal SYBR Green Master Mix (Bio-Rad) for primers, and SsoAdvanced Universal Probes Supermix (Bio-Rad) for FAM-labelled probes; reactions were run in a CFX Connect thermocycler (Bio-Rad) using an annealing/extension temperature of 60°C and a standard cycling protocol. Expression of genes across time points and tissues was analysed using the comparative threshold cycle (Ct), ΔΔCt method (35) by first calibrating the expression of genes relative to the geometric mean of the Ct value of the two reference genes in each tissue (i.e. ΔCttissue1GOI = Ct(tissue1)(geometric mean reference genes) – Ct(tissue1)(target gene)) and then relative to a calibrator tissue (for single-gene analyses of Rxfp4 across immune tissues) or time-point for the time-based analyses (3 weeks). Additionally, correlations between transcript abundance of Insl5 and Rxfp4 with each other and Il-7, Ghr and Foxn1 were performed by converting the raw qPCR values to molecule counts using the R-package MCMC.qPCR (36).
Systemic Effects of INSL5
Given the presence of Insl5 and, more so, Rxfp4 in central and peripheral immune tissues, we next sought to assess whether mice exhibited a peripheral immune response to intraperitoneal injection of Insl5 peptide in two pilot experiments (n = 8 and n = 14, respectively). Three days prior to the start of the experiment, mice were subjected to 70% calorie restriction (9); at which point ad libitum chow access was replaced with 3.4 g of chow delivered each morning throughout the experiment for a maximum of five days. On the fourth day, experimental mice were i.p. injected with INSL5 peptide (Phoenix Pharmaceuticals, 035-40A) at a concentration of 30 μg/kg of mouse weight, diluted in sterile 1x PBS (BioShop) for a total volume per injection of 100 μl (8, 9). Control mice were injected i.p. with 100 μl of 1 x PBS as time-matched vehicle control. Following terminal anesthesia, 200 μl was sampled from left ventricle of the heart at 6, 24, and 48h post injection and suspended in 5% v/v 0.5 M EDTA (disodium dehydrate, ph 7.4, Amersham) for anti-coagulation and kept on ice until centrifugation. Blood was spun for 10 min at 1,000 g to separate plasma. Serum was prepared according to Eve Technologies (Calgary, Canada, see Table S4). A discovery cytokine panel of 32 cytokines and chemokines of broad relevance, and a TH-17 panel including 11 cytokines were analyzed (n = 14, Supplementary File 3). For the metabolic assay, 11 peptides were analysed (n = 8, Supplementary File 3) including amylin (active), C-Peptide 2, GIP (total), GLP-1 (active), Ghrelin (active), Glucagon, Insulin, Leptin, PP, PYY, and Resistin. The effect of INSL5 injection on plasma cytokine and metabolic markers, was assessed using general linear models (GLM); variables were box-cox transformed, and treatment included as a fixed effect using the control group as reference (1,0 coding). Type III sums of squares were used to test for global effects of the treatment using F-statistics and post-hoc Tukey tests used to identify which treatment group (level) exhibited significantly different expression from the control. Cytokine levels in splenic T-cells: Single cell suspension of spleens from i.p. injected mice were stained ex vivo for expression of CD3, CD4, CD8, IL-17, IL-10, TNFα, and IFN-γ. Fluorochrome-conjugated anti-mouse antibodies (mAbs) were obtained from eBioscience (San Diego, CA). For intracellular cytokine staining, after surface staining, cells were fixed in 2% paraformaldehyde (Sigma-Aldrich), permeabilized with 0.1% saponin (Sigma-Aldrich) in staining buffer, and stained with specific fluorochrome-conjugated mAbs against IL-17, IL-10, TNFα, and IFN-γ. Cells were acquired using BD FACS Canto II (BD Bioscience, Mississauga, ON, Canada) and analyzed using FlowJo software (Tree Star, Ashland, OR). Additional graphs and statistical analyses were done using GraphPad Prism software version 8.0 (GraphPad, La Jolla, CA).
INSL5 Expression in and Effect on Immune System Cell Subsets
We next examined whether Rxfp4 is expressed in immune cell subsets, including bone-marrow derived and splenic DCs, as well as five macrophage and cancer cell lines from mouse or human, respectively. BMDCs: Bone marrow cell culture was established for production of precursor DCs (immature) by plating bone marrow single cell suspension on five individual petri dishes at 2 million cells per plate for four time points at 1, 3, and 7 days post-differentiation with complete RPMI (DMEM supplemented with 10% heat-inactivated fetal bovine serum, 2 mmol L-glutamine, 100 U/ml Penicillin, and 100 μg/ml streptomycin) and GM-CFS (20 ng/ml; PeproTech, Rocky Hill, NJ, USA). Additional complete RPMI and GM-CFS (20 ng/ml) was added on day 3. Additional cells from day 7 were counted and divided into three treatments of 1 million cells with five replicates: unstimulated immature DC’s, or stimulated with LPS (100 ng) for 6 or 24 h. RNA was collected from all samples and screened for the presence of Rxfp4 and cd14, a marker of DC differentiation, for all cell fractions. Dendritic cells: DCs were isolated from three spleens following the protocol outlined by EasySep™ Mouse CD11b Positive Selection Kit II (STEMCELL) and the purity of the DC fraction assessed by flow cytometry by gating on CD11c. Cell lines. We screened five cell lines for expression of Insl5 and Rxfp4 including two macrophage cell lines from mice (ANA-1 and BALB/c), and three cancer cell lines from human (Jurkat, SupT, MCF-7). Cells were grown to a confluence of 2.0x106 cell, RNA extracted using Trizol (ThermoFisher) using the manufacturer’s direction, and qPCR performed with pre-designed SYBR primers (purchased from Sigma-Aldrich) for the focal genes (Insl5 and Rxfp4) and two house-keeping genes per species (ubc-2 and S18 in mouse and GAPDH and RPLPO in human) using SsoAdvanced Universal SYBR Green Master Mix (Bio-Rad) as above. The relative expression of Insl5 and Rxfp4 was assessed relative to the house-keeping genes as: ΔCttissue1GOI = Ct(tissue1) (geometric mean reference genes) – Ct(tissue1)(target gene).
Effect of Insl5 Incubation on Cytokine Expression in ANA-1 Mouse Macrophages
Next, we examined the effect of INSL5 on growth rate of the ANA-1 cells and their expression of cytokines by treating cells with 100 nM INSL5 and 1 ug/ml of LPS simultaneously (co-treatment), or pre-treating cells with INSL5 for 3, 6, 12, or 18 h prior to stimulation with LPS, and included a treatment with LPS alone as control. Each of the six treatments was established in triplicate, and the experiment run for two durations: stimulating cells in INSL5/LPS or LPS alone for 12 or 36 h. At the end of the experiment, the number of viable cells at 12 or 24 h was re-counted on a cell counter, RNA extracted and qPCR performed as above to assess changes in the expression of six cytokines: IL-1β, IL6, IL10, TNFα, and Il-5, IL-15, the first six being important immune modulators and the latter two exhibited significant changes in the injection experiment. The relative expression of cytokines was assessed using a Bayesian analyses of qRT-PCR data as implemented in the R package mcmc.qpcr (36). This approach is robust to handling genes with low expression (typical of many cytokines). It employs a generalized linear mixed model based on a prior Poisson-lognormal distribution of values, and then calculates a Bayesian z-score (the mean of the posterior divided by its standard deviation) to perform a standard z-test and derive two-tailed p-values. Differences in viable cell counts among treatments were calculated using the glm function in R and the log2 fold-difference of cytokines between each treatment group and the control was assessed by correcting the above Bayesian two-tailed p-values for difference among treatments with a Bonferonni global correction for multiple testing (α = 0.05/75 test, p < 0.00067) from the summary$genewise output in MCMC.qpcr (36). All analyses were conducted in R v4.0.2.
Results
Insl5 and Rxfp4 Signatures Are Present in Immune System Organ-Derived RNA-Seq Data
Insl5 expression was restricted to the thymus and specific lymphocyte subsets (Table 1 and Table S2). In the thymus, Insl5 was highly expressed in thymocytes overall, especially in DP and DN thymocytes but also in thymic epithelial cells (TECs) – cortical TEC’s (cTECs) and to a lesser extent in medullary TEC’s (mTECs), and thymic natural Th17 cells (Table 1). Peripherally, Insl5 was expressed at low levels in naïve and Th1 CD4+ T-cells, CD8+ T-cells and intestinal intraepithelial lymphocytes (IELs). Compared to Insl5, Rxfp4 was more broadly expressed across all queried immune tissues and cell types (Table 1, Table S2). Substantial (> 10 reads per sample) Rxfp4 expression was detected in blood, spleen, lymph node, and bone marrow, while lower levels were also found in thymus. Of the queried cell types, hematopoietic stem cells, splenic CD8+ memory T-cells and monocytes had the highest levels of Rxfp4 expression, while lower Rxfp4 expression was detected across most other cell types.
Phylogenetic Foot-Printing of Insl5 and Rxfp4 Regulatory Regions Identifies Immune-Related Transcription Factors
Based on the in silico analyses of CNE’s between human and mouse, three conserved regions for both Insl5 and Rxfp4 were identified (Supplementary File 2, Figures 2A, B); the subtending sequences were extracted and submitted to promo for TF identification. Fifty-one TFs were identified as potentially regulating the expression of Rxfp4 and/or Insl5 (Supplementary File 2); the majority of them belonged to the zinc-finger, helix-turn-helix and winged helix-turn-helix DNA-binding families (Supplementary File 2). Genomic rearrangement of the regions flanking Insl5 in the murid lineage rendered it difficult to identify CNE’s for Insl5, and most identified CNE’s for Insl5 were intronic (Supplementary File 2, Figure 2A). Twenty TF’s were identified as having potential immune/haematopoietic functions and submitted to ContraV3 for further pan-vertebrate analyses using the gene regions in human as reference (Supplementary File 2). For Insl5, there was strong ab initio support that the glucocorticoid receptor (GR), STAT4, STAT5a/STAT5b, T cell Factor 1 (TCF-1), c-ETS-1, FOXP3, and STAT1 (Figure 1A, Supplementary File 2), are potential TF’s, and some support for binding to RUNX3, NF-AT. The genomic region housing Insl5 is not characterized for H3K4Me1 marks (open chromatin), DNase I hypersensitivity clusters, or Chip-Seq experiments by the ENCODE project. For Rxfp4, there was strong support for TFBS motifs that could bind: GR, STAT1, STAT4, STAT5a/5b, TCF-1, c-ETS1, GATA1-3 (Figure 1B, Supplementary File 2 for full details of all sites and support). Of these TF’s, GR, TCF-1, STAT4 and STAT5b are not included in the ENCODE 181 TF Chip-Seq data, but there is Chip-Seq evidence that GATA2 and GATA3 are TF’s for Rxfp4 in HUVEC (GATA2) and T-47D (GATA3) cell lines—the former an umbilical cord epithelial cell line and the latter an epithelial cancer cell line. Thus, it appears that GR, STAT4, STAT5a/5b and TCF-1 may regulate both Insl5 and Rxfp4, while FOX-P3 may additionally regulate Insl5 and GATA1, 2, and 3 Rxfp4 (Figure 1C), the latter of which is supported by experimental evidence from the ENCODE project.
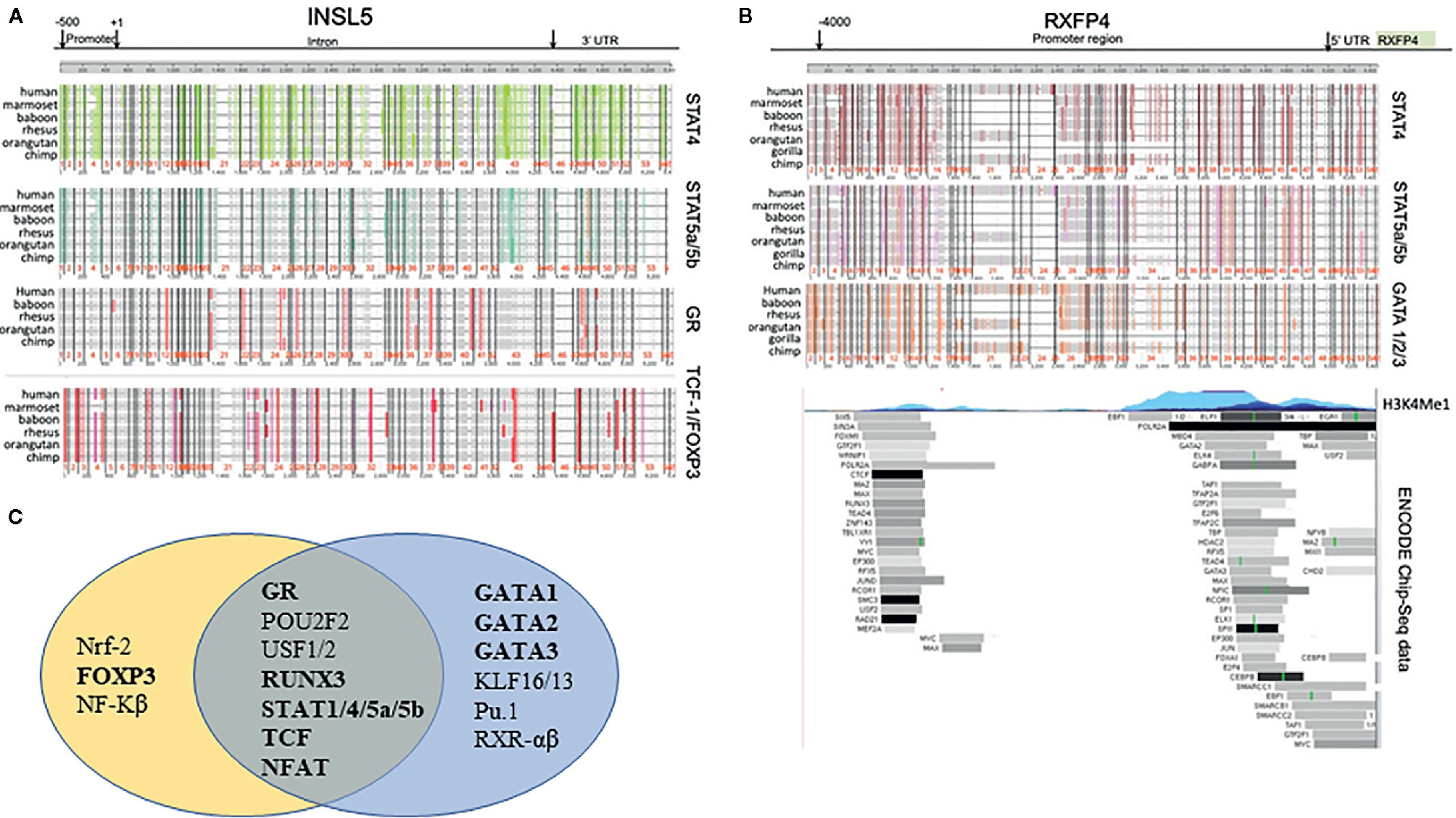
Figure 1 Phylogenetic footprinting analysis of the regulatory regions neighbouring Insl5 and Rxfp4, as well as experimental support for regulatory regions and TF’s associated with the Rxfp4 gene (ENCODE project). (A) Evidence for conserved TFBS motifs in regulatory regions of Insl5 across vertebrates relative to the gene in humans. Upper panels – conserved motifs for STAT4, STAT5a/STAT5b, GR and TCF-1/FOXP3 in the core promoter (500bp) intron, and 3’UTR of Insl5 (full details and other TF’s presented in supplementary data). (B) Evidence for conserved TFBS motifs in regulatory regions of Rxfp4. Upper panel, Conserved TFBS in 4000 bp region upstream and in the 5’UTR relative to the gene in humans for STAT4, STAT5a/STAT5b and GATA 1/2/3. Middle panel H3K4Me1 sites showing evidence of open chromatin. Lower panel – Experimental evidence for binding of TF’s examined by ENCODE (181 in total) in multiple cell lines and tissues (data last accessed January 2020). (C) Venn Diagram showing potential transcription factors involved in Immune/Haematopoietic processes for Insl5 and Rxfp4.
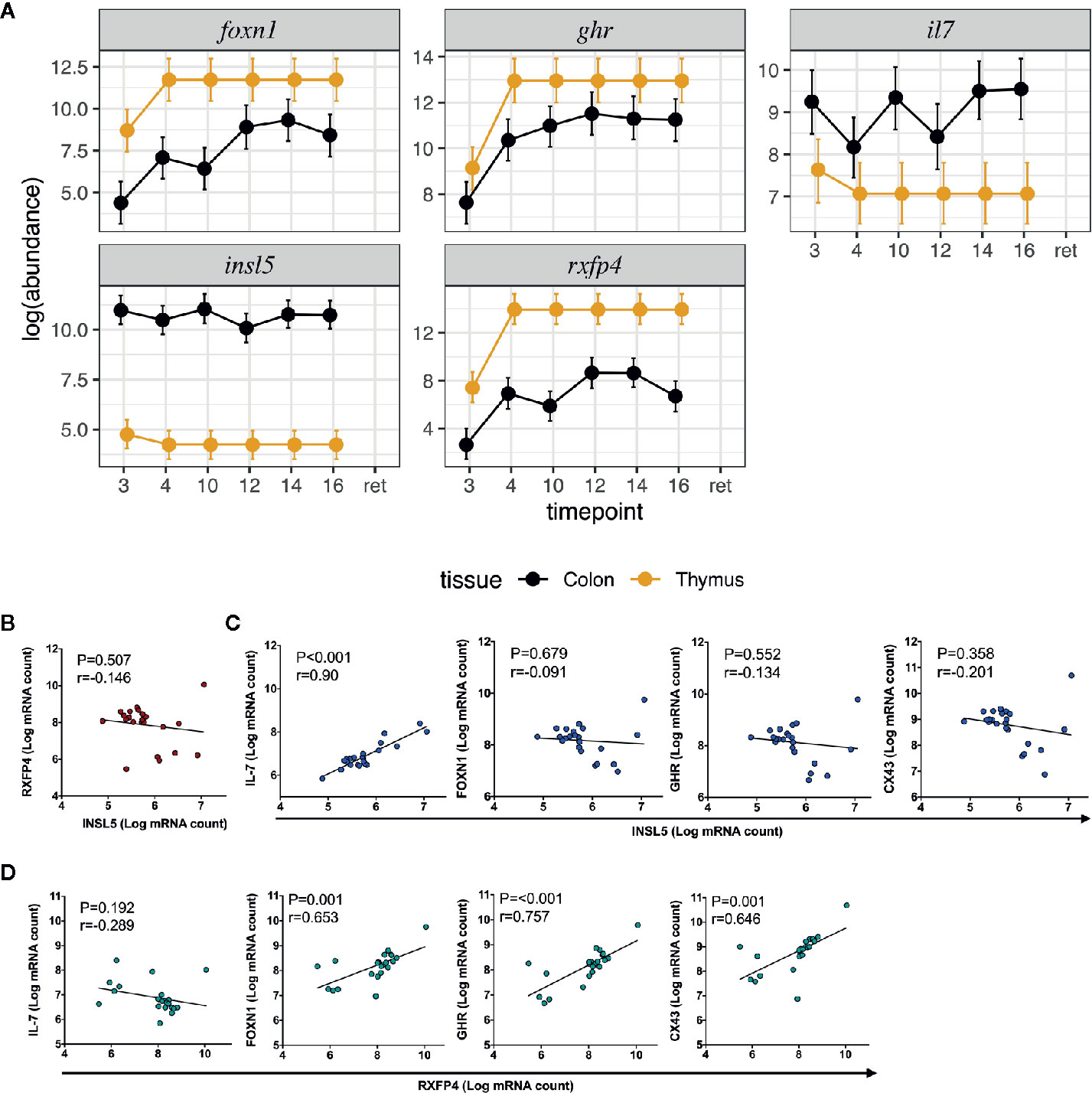
Figure 2 Expression and correlation profiles of Insl5, Rxfp4, FoxN1, Ghr, and Il-7 in mouse immune tissues; (A) Temporal and spatial expression of Insl5, Rxfp4, Ghr, and Il-7 in colon and thymus of mice aged 3–16 weeks and retired breeders, (B) Correlation of Insl5 and Rxfp4 expression in thymus, (C) Correlation of Insl5 vs Il-7, FoxN1, and Ghr in thymus, (D) Correlation of Rxfp4 vs Il-7, FoxN1, and Ghr in thymus. Correlation analyses were done using R-package MCMC.qPCR by converting the raw qPCR values to molecule counts.
Insl5 and Rxfp4 are Variably Expressed in Mouse Immune Tissues
We next sought to validate the presence of Insl5 and Rxfp4 in blood, thymus, bone marrow, colon, and spleen in C57/Bl6 mice stratified by age (from 3 weeks to >12 months). Consistent with the RNA-seq profiles, Insl5 mRNA was detected only in thymus and colon (Figure 2A, Figure S2). However, total thymic expression of Insl5 was relatively uniform and lower than that of thymic markers Il-7 and FoxN1, and lower than Rxfp4 (Figure 2A). In thymus, the expression of Rxfp4 was similar to that of thymic markers FoxN1 and Ghr, lowest at 3 weeks, but then increasing markedly and remaining at similar levels in older animals (Figure 2A). Rxfp4 mRNA was detected in all immune system tissues, exhibiting the highest expression in thymus and spleen, followed by bone marrow and then blood (Figure 3, Figure S1). There was no significant change in expression within a tissue over time, except in blood, for which expression of Rxfp4 was significantly lower in 3-week old mice relative to all other age cohorts (Figure 3). We examined the correlation in expression of Insl5 and Rxfp4 with each other and three markers of thymocyte development. While no correlation was observed between Insl5 and Rxfp4 (Figure 2B), Insl5 expression was highly correlated with that of Il-7 (r = 0.9, p < 0.001) (Figure 2C), and expression of Rxfp4 correlated with that of FoxN1 (r-0.65, p < 0.001) and Ghr (r = 0.75, p < 0.001) (Figure 2D).
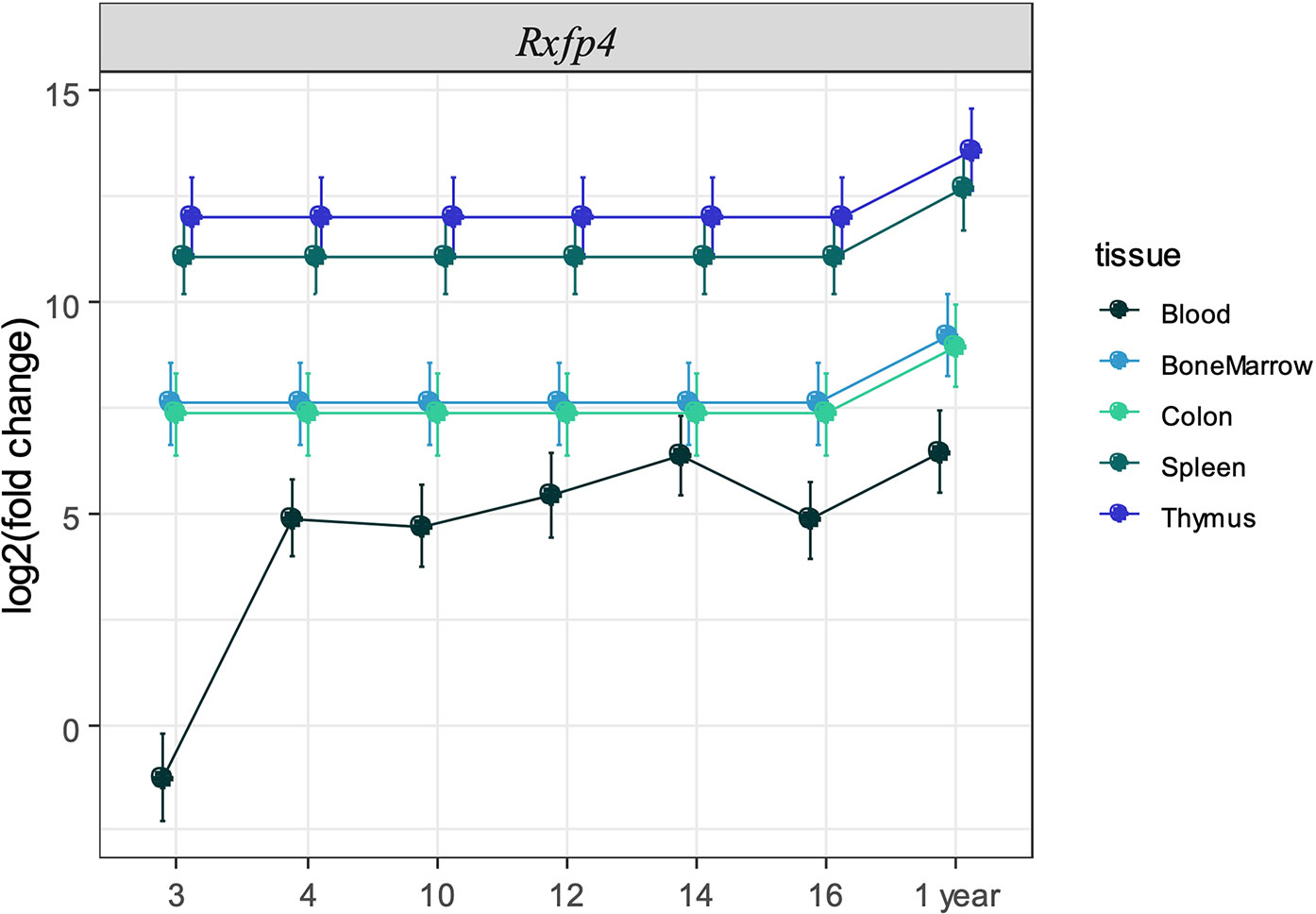
Figure 3 Expression of Rxfp4 across immune-related tissues and cells. Log2 fold change in expression of Rxfp4 relative to the housekeeping genes Ubc-2, 18S rRNA, and relative to the expression in blood in three-week old mice for each tissue.
INSL5 Treatment Changes Levels of Immune and Metabolic Markers in Mouse
We reasoned that if INSL5-RXFP4 axis is indeed implicated in immune system signaling as suggested by in silico and expression analyses, then INSL5 fluctuation may have detectable effects on systemic markers of the immune response. To test this, we performed INSL5 injections and assessed the changes in levels of circulating immune mediators and metabolic peptides. In support of the insulinotropic features of INSL5, we observed significantly elevated concentrations of blood GLP-1 (6 h, 24 h, p.i.) and C-peptide (24 h, p.i.) (Figure 4C) relative to vehicle, as well as Insulin (24 h, p.i.) (Table S6). Significant elevations were seen also for glucagon (24 h, p.i., Table S6), which inhibits insulin secretion, as well as for PP (6 h p.i), PYY (24 h p.i.) and Amylin (24 h, p.i.) (Table S6). Of the cytokines surveyed, 27 produced sufficient levels for analyses: of these, significant alterations were seen for MIP-2 and G-CSF (MIP-2 increased and G-CSF decreased at 24 and 48 h p.i.), while treatment level effects were seen for IL-7 and IL-5 (both decreased at 6 h p.i.), eotaxin, (decreased at 24 h p.i.) and M-CSF, IL-15 and IL-27 (all at 48 h p.i.) (Figures 4A, B, Table S5). Thus, systemic injection of INSL5 confirmed the possible insulinotropic effects of INSL5, and resulted in changes in 7 cytokines, predominantly those involved with mediating homeostatic responses, particularly those affecting macrophage proliferation.
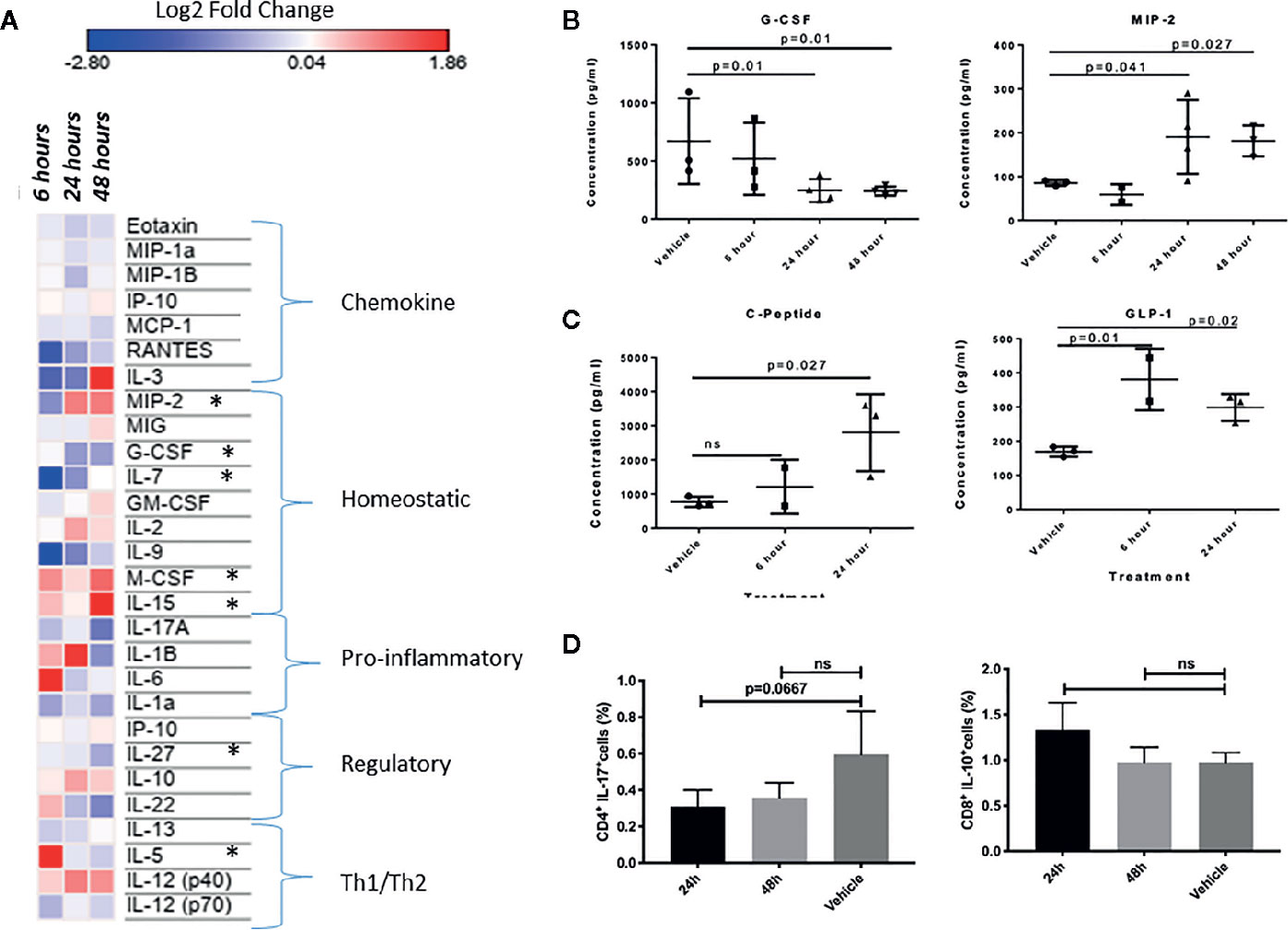
Figure 4 (A) Heat-map visualization of changes to 27 chemokines and cytokines following in vivo injection of INSL5. Contrast analyses comparing control (PBS injection) to three post-injection intervals (6, 24, and 48 h) found significant differences in seven cytokines over time (MIP-2, G-CSF, IL-7, M-CSF, IL-15, IL-27, IL-5). (B) Difference in plasma concentration of G-CSF and MIP-2 in control (PBS injected) from treated (INSL5 injected) mice 6, 24, and 48 h intraperitoneal injection. (C) Mice injected with INSL5 showed significantly different levels of GIP, and insulinotropic hormone GLP-1. (D) Decreased IL-17 and increased IL-10 levels 24 h post-INSL5 i.p. injection in mouse splenic CD4+ and CD8+ T-cells, respectively. Percent positive cell populations detected using BD FACSCanto-II. Statistical analyses using one-way ANOVA, multiple comparisons.
In parallel to plasma analyses, the levels of IL-17, IL-10, TNFα, and IFN-γ were assessed in splenic CD4+ and CD8+ T-cells using FLOW cytometry 24 and 48 h following INSL5 injection. CD4+ T-cells had a higher proportion of IL-17+ populations than CD8+ cells (Supplementary File 4), and IL-17 was decreased in CD4+ T-cells at 24 h (Figure 4D), replicating the alterations measured in plasma (Figure 4A). Anti-inflammatory IL-10 exhibited an increased trend in CD8+ T-cells, though not statistically significant (Figure 4D). Pro-inflammatory TNFα and IFN-γ exhibited an overall decreasing trend in both subsets of T-cells (Supplementary File 4), though significant effects in comparison to control were not observed likely due to the limited time release of TNFα and IFN-γ.
INSL5 Affects Cell Proliferation and Immune Markers in Immune Cell Subsets
Given the broad expression of Rxfp4 across immune tissues and cell subsets, we hypothesized that INSL5 may convey signals through major immune cell subsets, such as T-cells and dendritic cells. In agreement with the in silico data, dendritic cells and mouse cell lines derived from DCs (e.g. ANA-1 and Balb/c macrophages cell lines) expressed detectable levels of Rxfp4 (Figure 5A); and ANA-1 cells also expressed Insl5 (Figure 5B). Rxfp4 expression increased over time in BMDC’s, and was higher in whole spleen (9-fold) than the non-DC’s portion of spleen (Figure 5A). No Rxfp4 expression was detected in splenic T-cells (not shown). Rxfp4 was expressed in all three human cancer cell lines, Jurkat, MCF-7 and SupT, while Insl5 was also expressed in the latter two, with both genes showing the highest expression in the breast cancer MCF-7 cells (Figure 5B). We then used mouse macrophage ANA-1 cells to examine the effect of INSL5 on cytokine expression following inflammatory activation of cells using LPS. Overall, INSL5 appeared to suppress cell growth; the number of viable cells was significantly higher in the LPS control than those co-treated with INSL5/LPS following 12 h of stimulation (Figure 5C).
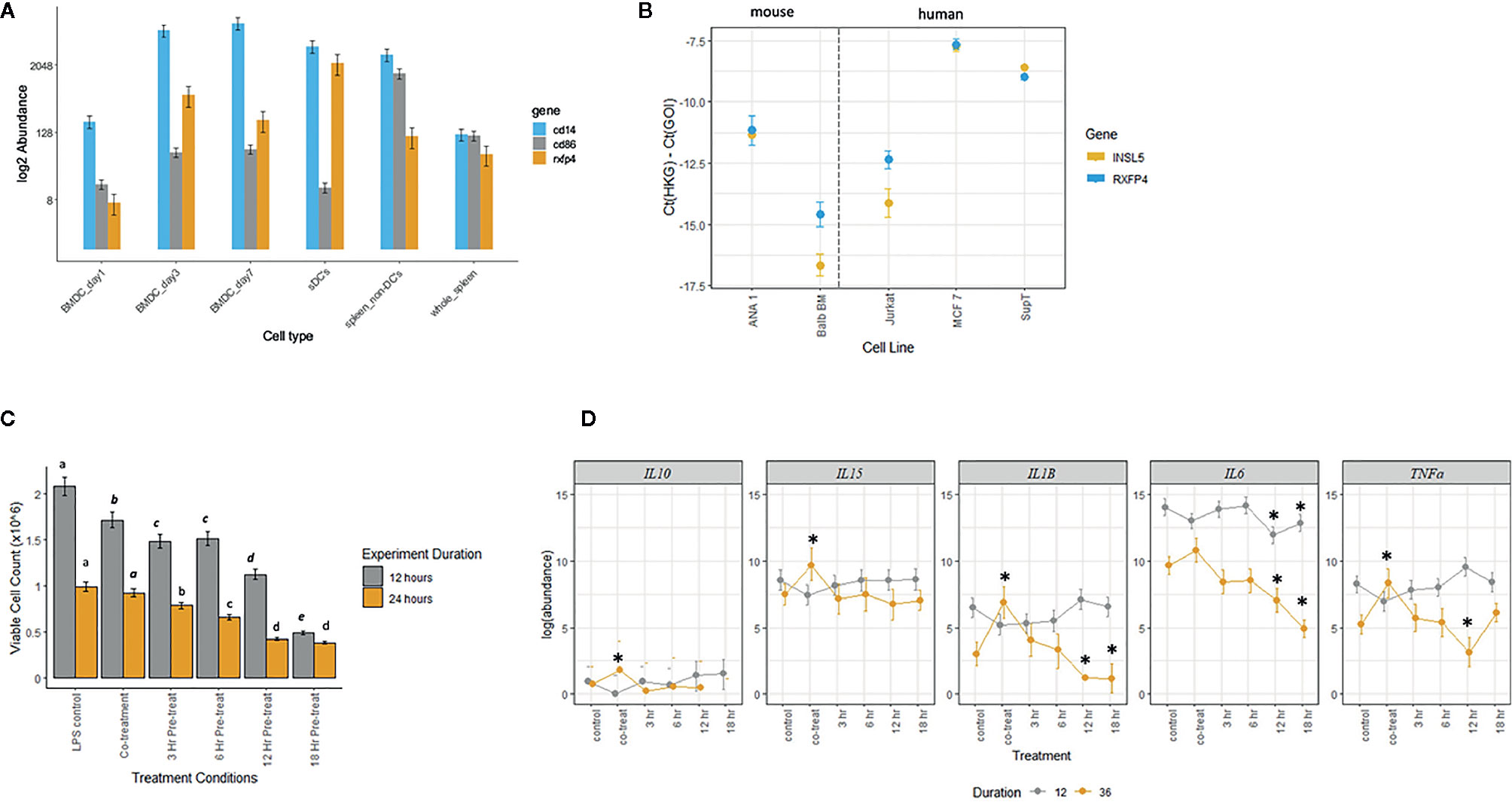
Figure 5 (A) Estimated abundance of Rxfp4, cd86 and cd14 in bone-marrow derived dendritic cells (BMDC’s) one, three, and seven days post-differentiation in GM-CSF, whole spleen, splenic dendritic cell (sDC’s), and non-dendritic cell (non-DC) fraction of spleen. (B) Expression of Insl5 (yellow) and Rxfp4 (blue) in two mouse macrophage lines, ANA-1 and Balb-BM, relative to the geometric mean of two housekeeping genes, ubc-2 and S18 (left panel) and in three human cancer cell lines, Jurkat, MCF-7 and SupT, relative to gapdh and rplpo. (C). Number of viable ANA-1 cells after 12 or 24 h stimulation with LPS in six treatment lines: LPS control, co-treatment with INSL5 and LPS, and pre-incubation in INSL5 for 3, 6, 12, or 18 h prior to LPS stimulation. Differences in the number of cells per treatment was assessed using ANOVA and treatments with significantly different means within an experiment (12 or 24 h) are indicated in letters. (D) Bayesian generalized linear mixed model analyses of the change in abundance of cytokines in six treatments following 12 or 36 h or LPS stimulation in ANA-1 cells. The log2 fold-difference between each treatment against LPS control was calculated using a standard z-test and then two-tailed p-values calculated. Only those comparisons that were significant after correcting for multiple tests (p,0.00067) were deemed significant. See methods for details, and Table S7 for fold-change and p-values.
Secondly, for all cells pre-treated with INSL5, the number of viable cells after both 12 and 24 h was significantly lower than the control or co-treatment lines (Figure 5C). For cytokine expression, incubation of ANA-1 cells in INSL5 resulted in no significant differences in expression of IL-10 or IL-5 (not shown as levels were zero for many time points). Levels of IL-15 were significantly higher in the co-treatment and 3 h INSL5 pre-treatment compared to control for cells stimulated for 36 but not 12 h, which is consistent with the in vivo injection experiment. Levels of IL-1β did not change significantly among treatments following 12 h of stimulation, however co-treatment of cells with INSL and LPS resulted in higher expression of IL-1β after 36 h, and cells pre-treated with INSL5 for 12 or 18 h exhibited significantly lower levels of pro-inflammatory cytokine IL-1β (Figure 5D). Similarly, levels of the pro-inflammatory cytokine IL-6 were significantly lower following INSL5-LPS co-treatment than in the control, and lower in cells pre-treated with INSL5 for 12 or 18 h prior to LPS stimulation for both time courses (Figure 5D). Finally, levels of TNFα were not different among treatments following 12 h of stimulation, but they were higher in cells co-treated with INSL5/LPS after 36 h of stimulation with LPS, and lower than control cells in cells pre-treated with INSL5 for 12 h (Figure 5D) (see Table S7 for all results). Collectively, this suggest that INSL5 has an overall inhibitory effect on ANA-1 macrophage proliferation and an anti-inflammatory effect on cytokine release.
Discussion
Using a combination of in silico and experimental approaches, we find evidence that INSL5 can alter cytokine profiles in the peripheral immune system potentially via its cognate receptor, RXFP4, which is expressed in both central and peripheral immune tissues, and on some immune system subsets, particularly those involved with the innate immune system. We detected expression of Insl5 in thymus and colon; and find that in thymus, Insl5 was most highly expressed in the youngest cohort of mice, where it was strongly correlated with expression of Il-7, a marker of early thymocyte development. Through data mining, we show that Insl5 is specifically expressed in some immune cell subsets, particularly in the thymus (DP and DN thymocytes, cTEC’s), intestinal epithelial lymphocytes (IEL’s) and a few CD4+ T-cell subsets. Through phylogenetic footprinting we identified strong evidence for regulation by immune-related TF’s including: STAT’s (STAT 1, STAT 4, STAT 5a/5b), which work via JAK/STAT signaling pathways to regulate the expression of genes in cell survival, proliferation, immune response, and hematopoiesis; FOXP3, a master regulator of the development of T-cells, as well as TCF-1 and NF-AT, which enhance thymus-specific gene expression (Supplementary File 2).
For RXFP4, using qPCR, we found higher expression of Rxfp4 than Insl5 across all immune tissues (except colon), including the thymus. Our experimental work in thymus was based on examination of whole thymic tissue using verified Taq-man probes, but our finding of higher expression of Rxfp4 than Insl5 in thymus runs contrary to our bioinformatics data mining which identified high expression of Insl5 in many thymus-specific RNA-sequencing datasets at NCBI (Table S2). One possible explanation for this result is that Rxfp4 is expressed at lower levels in many thymic cell subsets, while Insl5 may be expressed at higher levels in a few cell subsets (e.g. DP thymocytes), a hypothesis that is consistent with our database mining. We further find that the expression of thymic Rxfp4 was correlated with that of FoxN1 and Ghr across all age cohorts, and that Rxfp4 expression was not lower in the oldest cohort of mice. FoxN1 is a transcription factor that regulates hundreds of genes in the thymus during T-cell development, particularly contributing to the function of thymic epithelial cells (TEC’s) (31, 37), while GHR (via GH secretagogues) is also associated with maintenance of TEC’s and thymus cytoarchitecture (29, 30). Collectively, these results indicate that further investigation into the potential role of INSL5 in thymocyte development, perhaps during DN-DP selection or through the development FOXP3+ T-regulatory cells is warranted.
The interplay between endocrine hormones and the immune system is complex. However, many appetite regulating hormones can also regulate levels of cytokines in vitro and systemically, markedly GLP-1 (38–40). INSL5 and GLP-1 are co-secreted from L-cells together with PYY (41); notably all three of these gut hormones were found to be upregulated following i.p. injection of INSL5, consistent with recent transcriptomic data showing that they are co-regulated (41). GLP-1 is emerging as a player not only in metabolic glucose control, but also in orchestrating immune responses in the gut (40). Since INSL5 levels were shown to be highly elevated in the distal guts of germ-free mice (42), we reasoned that their response to changing microbial conditions in the gut (7) could mediate an anti-inflammatory response to facilitate microbial colonization.
Thus, we performed i.p. injections in a mouse model using concentrations of INSL5 peptide found to have significant effects on the feeding response by (9) and looked for changes in plasma cytokines which are indicative of a systemic immune response. We aimed to validate the concentration and mode of INSL5 injection by confirming the insulinotropic effect following i.p. injection of INSL5 and measured significant increases in GLP-1 (6 and 24 hr, p.i.), Insulin and C-peptide (24 hr, p.i.) after injection as well as a transient increase in PP followed by PYY, when compared to sham injection. Although this suggests an overall insulinotropic effect of INSL5 as found in other research (6, 8), levels of glucagon, which impedes insulin secretion, were also higher, underscoring the need for more research on the effect of INSL5 in glucose metabolism as suggested by other authors (3, 7).
We then sought to assay levels of a discovery panel of cytokines representing cytokines with diverse roles in immune cell signaling 6, 24, and 48 h post injection. Overall, 7 cytokines exhibited changes in expression level at one or more time points following injection, three of them were chemokines or homeostatic cytokines that specifically modulate macrophage proliferation (MIP-2, G-CSF and M-CSF) (43, 44) or other homeostatic processes (IL-7 and IL-15) (45–47) while the other two fell into regulatory (IL-27) or Th1/Th2 responses (IL-5) (48) In a parallel experiment, we measured a decrease in inducer of inflammation IL-17 in the CD4+ splenic T-cells isolated from INSL5 injected mice (49), as well as a marginal increase in the anti-inflammatory IL-10 in both CD4+ and CD8+ T-cells, more notably in the latter (50).
Next, we wanted to further probe whether specific immune cell subsets expressed Rxfp4, and then responded to INSL5 following LPS stimulation. We show here, for the first time, that naïve BMDCs differentiated in the presence of GM-CSF, do not express Rxfp4 on the first day of differentiation, but do on days 3 and 7 of differentiation. Further, expression of Rxfp4 was six-fold higher in the sDCs compared to the non-DCs fraction. Turning to mouse and human cell lines, we found that Rxfp4 was expressed in both mouse macrophage cell lines (ANA-1 and BALB/c), as well as three human cancer cell lines (Jurkat, MCF-7, and SupT). To test the hypothesis that an Rxfp4-expressing macrophage cell line may mediate immune-responses to INSL5, we employed the mouse BMDM cell line, ANA-1, and conducted an experiment to test whether cells pre-incubated with INSL5 had altered levels of cytokines relative to cells not pre-incubated with INSL5 (co-treatment) or to the control (LPS alone). All treatments were stimulated in LPS for two-time courses (12 or 36 h) and both viable cell counts and levels of six cytokines assayed by qPCR. We chose cytokines that were observed to show systemic effects in the injection experiment (IL-5 and IL-15), or are known to be important immune modulators (IL-10, IL-1B, IL-6 and TNFα). Several of the cytokines showed elevated levels of expression in the co-treatment compared to control (IL-10, IL-15, IL-1β, and TNFα) after 36 h of LPS stimulation, and both IL-1β and IL6 showed a significant reduction in expression when cells were pre-incubated in INSL5 for 12 or 18 h following both 12 and 36 h of stimulation (IL-6) or following only 36 h of stimulation (IL-1β).
These results were also consistent with the injection experiment for IL-15 and IL-6 (IL-1β levels were not high enough for detection in the in vivo experiment) and suggest that INSL5 could alter the inflammatory response under some conditions. Pro-inflammatory IL-6 is secreted in response to microbial proteins and is elevated in gut inflammatory diseases (51). Tessaro et al. (52) found that stimulation of BMDM’s with insulin and LPS led to a significant increase in IL-6 and TNFα, via upregulation of the PI3K and ERK 1/2 pathways, in diabetic but not non-diabetic mice, but found a downregulation of IL-6 and TNFα following insulin + LPS in tissue resident macrophages (alveolar and peritoneal macrophages) (52). Here, we find evidence of a down-regulation of IL-6 and IL-1β and to a lesser extent in TNFα in the BMDM’s from a non-diabetic C57/Bl6+ mice. The findings that incubation in INSL5 depressed proliferation of ANA-1 cells, and reduced pro-inflammatory cytokine release, suggest that the effect of INSL5 on tissue-resident and non-resident macrophages and dendritic cells warrants further exploration.
Our data mining uncovered broad similarities in the expression of Insl5/Rxfp4 in human and mouse when data on both species was available, but there are known differences between the species in both the tissues and cell subsets that express them, even in colon (53). Of relevance here, most of our experimental and data mining results for the expression of Insl5/Rxfp4 in thymus are derived from mouse, because no human data was available. In the gut, however, there is evidence that Rxfp4 is expressed in immune-relevant cell types in both human and mouse, including goblet cells (53), the vagus nerve in mouse (9), as well as splenic DC’s (here). Thus, it is possible that mouse is a reasonable model to study the possible immune function of these genes, despite the fact that the peptide sequence conservation between the species is modest (75% for INSL5 and 74% for RXFP4), and there are genomic re-arrangements in the regions housing the genes, a situation potentially associated with the pseudogenization of the genes in some murids.
If INSL5 has immunomodulatory effects in the gut-immune axis, it would be expected to play a role in metabolic syndromes, such as inflammatory bowel disease and Crohn’s. Retrieval of the RNA-seq data at the EMBL-EBI expression atlas (www.ebi.ac.uk/gxa/home), last accessed May 2019) uncovered 26 experiments for Insl5 and 25 for Rxfp4 in which significant differences were observed between treatment arms: five of these studies found decreased expression of Insl5 or Rxfp4 in association with metabolic disorders such as Crohn’s disease, while 22 (11-Insl5, 11-Rxfp4) found significant changes in gene expression associated with various cancer including colon and breast (summarized in Table S3). Further, a clinical study in humans undergoing bariatric surgery found that plasma levels of INSL5 were inversely correlated with C-reactive protein (CRP), a marker of inflammation secreted by the liver into plasma in response to acute stress (16), and positively correlated with BMI and insulin resistance (11, 16). Taken together with the results presented here, we propose that INSL5 and RXFP4 play roles in immune system signaling, both in the central and peripheral immune systems. Our findings indicate potential roles in thymocyte selection in the central immune system as well as on macrophage proliferation in the peripheral immune system. Further investigation is required to tease apart the specific roles of INSL5-RXFP4 and aid our understanding of the peptide’s role in the immune-metabolic axis and conditions of health and disease.
Data Availability Statement
Publicly available datasets were analyzed in this study. Existing datasets are available in a publicly accessible repository (SRA and EMBO/EBI). The relevant accession numbers can be found in our Supplementary Files 1, 2. For cytokine and metabolic assays, and log 2 fold changes, p-values used for the qPCR data, the raw values can be found in Supplementary File 3.
Ethics Statement
The animal study was reviewed and approved by Animal Care Committees of University of Winnipeg and University of Manitoba.
Author Contributions
SG and BV conceptualized the experimental work in collaboration with JU, SY, and AS. Experiments were performed by BV, CO, JD, and DR. SG, BV, CO, and SY analyzed the data. SG and BV wrote the manuscript with editorial contributions from co-authors. All authors contributed to the article and approved the submitted version.
Funding
This research was funded by a Discovery Grant DG-06203 from the Natural Sciences and Engineering Research Council of Canada to SG, an NSERC-USRA to JD, a University of Winnipeg Graduate Student Scholarship to BV, and a University of Winnipeg Major Grant, awarded to SG.
Conflict of Interest
The authors declare that the research was conducted in the absence of any commercial or financial relationships that could be construed as a potential conflict of interest.
Acknowledgments
The authors would like to thank the animal care staff at University of Winnipeg, Robyn Cole, and Dan Wasyliw, for assistance.
Supplementary Material
The Supplementary Material for this article can be found online at: https://www.frontiersin.org/articles/10.3389/fendo.2020.610672/full#supplementary-material
References
1. Belgi A, Hossain MA, Shabanpoor F, Chan L, Zhang S, Bathgate RA, et al. Structure and function relationship of murine insulin-like peptide 5 (INSL5): free C-terminus is essential for RXFP4 receptor binding and activation. Biochemistry (2011) 50(39):8352–61. doi: 10.1021/bi201093m
2. Wang XY, Guo YQ, Shao XX, Liu YL, Xu ZG, Guo ZY. Identification of important residues of insulin-like peptide 5 and its receptor RXFP4 for ligand-receptor interactions. Arch Biochem Biophys (2014) 558:127–32. doi: 10.1016/j.abb.2014.07.010
3. Ang SY, Hutchinson DS, Patil N, Evans BA, Bathgate RA, Halls ML, et al. Signal transduction pathways activated by insulin-like peptide 5 at the relaxin family peptide RXFP4 receptor. Br J Pharmacol (2016) 174(10):1077–89. doi: 10.1111/bph.13522
4. Liu C, Chen J, Sutton S, Roland B, Kuei C, Farmer N, et al. Identification of Relaxin-3/INSL7 as a Ligand for GPCR142. J Biol Chem (2003) 278(50):50765–70. doi: 10.1074/jbc.M308996200
5. Liu C, Kuei C, Sutton S, Chen J, Bonaventure P, Wu J, et al. INSL5 is a high affinity specific agonist for GPCR142 (GPR100). J Biol Chem (2005) 280(1):292–300. doi: 10.1074/jbc.M409916200
6. Burnicka-Turek O, Mohamed BA, Shirneshan K, Thanasupawat J, Hombach-Klonisch S, Klonisch T, et al. INSL5-deficient mice display an alteration in glucose homeostasis and an impaired fertility. Endocrinology (2012) 153(10):4655–65. doi: 10.1210/en.2012-1161
7. Lee YS, De Vadder F, Tremaroli V, Wichmann A, Mithieux G, Bäckhed F. Insulin-like peptide 5 is a microbially regulated peptide that promotes hepatic glucose production. Mol Metab (2016) 5(4):263–70. doi: 10.1016/j.molmet.2016.01.007
8. Luo X, Li T, Zhu Y, Dai Y, Zhao J, Guo ZY, et al. The insulinotrophic effect of insulin-like peptide 5 in vitro and in vivo. Biochem J (2015) 466(3):467–73. doi: 10.1042/BJ20141113
9. Grosse J, Heffron H, Burling K, Hossain MA, Habib AM, Rogers GJ, et al. Insulin-like peptide 5 is an orexigenic gastrointestinal hormone. Proc Natl Acad Sci USA (2014) 111(30):11133–8. doi: 10.1073/pnas.1411413111
10. Yeganeh IS, Taromchi AH, Fathabadi FF, Nejatbakhsh R, Novin MG, Shokri S. Expression and localization of relaxin family peptide receptor 4 in human spermatozoa and impact of insulin-like peptide 5 on sperm functions. Reprod Biol (2017) 17(4):327–32. doi: 10.1016/j.repbio.2017.09.004
11. Bicer M, Alan M, Alarslan P, Guler A, Kocabas GU, Imamoglu C, et al. Circulating insulin-like peptide 5 levels and its association with metabolic and hormonal parameters in women with polycystic ovary syndrome. J Endocrinol Invest (2019) 42(3):303–12. doi: 10.1007/s40618-018-0917-x
12. Lee H, Lee S, Jeong D, Kim SJ. Ginsenoside Rh2 epigenetically regulates cell-mediated immune pathway to inhibit proliferation of MCF-7 breast cancer cells. J Ginseng Res (2018) 42(4):455–62. doi: 10.1016/j.jgr.2017.05.003
13. Sun G, Li Y, Peng Y, Lu D, Zhang F, Cui X, et al. Identification of differentially expressed genes and biological characteristics of colorectal cancer by integrated bioinformatics analysis. J Cell Physiol (2019) 234(9):15215–24. doi: 10.1002/jcp.28163
14. Thanasupawat T, Hammje K, Adham I, Ghia JE, Del Bigio MR, Krcek J, et al. INSL5 is a novel marker for human enteroendocrine cells of the large intestine and neuroendocrine tumours. Oncol Rep (2013) 29(1):149–54. doi: 10.3892/or.2012.2119
15. Mashima H, Ohno H, Yamada Y, Sakai T, Ohnishi H. INSL5 may be a unique marker of colorectal endocrine cells and neuroendocrine tumors. Biochem Biophys Res Commun (2013) 432(4):586–92. doi: 10.1016/j.bbrc.2013.02.042
16. Wagner IV, Flehmig G, Scheuermann K, Löffler D, Körner A, Kiess W, et al. Insulin-Like Peptide 5 Interacts with Sex Hormones and Metabolic Parameters in a Gender and Adiposity Dependent Manner in Humans. Horm Metab Res (2016) 48(9):589–94. doi: 10.1055/s-0042-109869
17. Hardison RC. Conserved noncoding sequences are reliable guides to regulatory elements. Trends Genet (2000) 16(9):369–72. doi: 10.1016/S0168-9525(00)02081-3
18. Tautz D. Evolution of transcriptional regulation. Curr Opin Genet Dev (2000) 10(5):575–9. doi: 10.1016/S0959-437X(00)00130-1
19. Brudno M, Poliakov A, Minovitsky S, Ratnere I, Dubchak I. Multiple whole genome alignments and novel biomedical applications at the VISTA portal. Nucleic Acids Res (2007) 35(Web Server issue):W669–74. doi: 10.1093/nar/gkm279
20. Mayor C, Brudno M, Schwartz JR, Poliakov A, Rubin EM, Frazer KA, et al. VISTA : visualizing global DNA sequence alignments of arbitrary length. Bioinformatics (2000) 16(11):1046–7. doi: 10.1093/bioinformatics/16.11.1046
21. Dermitzakis ET, Reymond A, Antonarakis SE. Conserved non-genic sequences - an unexpected feature of mammalian genomes. Nat Rev Genet (2005) 6(2):151–7. doi: 10.1038/nrg1527
22. Farre D, Roset R, Huerta M, Adsuara JE, Roselló L, Albà MM, et al. Identification of patterns in biological sequences at the ALGGEN server: PROMO and MALGEN. Nucleic Acids Res (2003) 31(13):3651–3. doi: 10.1093/nar/gkg605
23. Heinemeyer T, Wingender E, Reuter I, Hermjakob H, Kel AE, Kel OV, et al. Databases on transcriptional regulation: TRANSFAC, TRRD and COMPEL. Nucleic Acids Res (1998) 26(1):362–7. doi: 10.1093/nar/26.1.362
24. Messeguer X, Escudero R, Farré D, Nuñez O, Marti’nez J, Albà MM. PROMO: detection of known transcription regulatory elements using species-tailored searches. Bioinformatics (2002) 18(2):333–4. doi: 10.1093/bioinformatics/18.2.333
25. Vaquerizas JM, Kummerfeld SK, Teichmann SA, Luscombe NM. A census of human transcription factors: function, expression and evolution. Nat Rev Genet (2009) 10(4):252–63. doi: 10.1038/nrg2538
26. Kreft Ł, Soete A, Hulpiau P, Botzki A, Saeys Y, De Bleser P. ConTra v3: a tool to identify transcription factor binding sites across species, update 2017. Nucleic Acids Res (2017) 45(W1):W490–4. doi: 10.1093/nar/gkx376
27. Consortium EP. An integrated encyclopedia of DNA elements in the human genome. Nature (2012) 489(7414):57–74. doi: 10.1038/nature11247
28. Vahkal B. Exploring the role of insulin like peptide 5 (INSL5) and its cognate receptor in the immune system. In: Biology. Winnipeg: University of Winnipeg (2017). p. 159.
29. Morrhaye G, Kermani H, Legros JJ, Baron F, Beguin Y, Moutschen M, et al. Impact of growth hormone (GH) deficiency and GH replacement upon thymus function in adult patients. PloS One (2009) 4(5):e5668. doi: 10.1371/journal.pone.0005668
30. Taub DD, Murphy WJ, Longo DL. Rejuvenation of the aging thymus: growth hormone-mediated and ghrelin-mediated signaling pathways. Curr Opin Pharmacol (2010) 10(4):408–24. doi: 10.1016/j.coph.2010.04.015
31. Zuklys S, Handel A, Zhanybekova S, Govani F, Keller M, Maio S, et al. Foxn1 regulates key target genes essential for T cell development in postnatal thymic epithelial cells. Nat Immunol (2016) 17(10):1206–15. doi: 10.1038/ni.3537
32. Ortman CL, Dittmar KA, Witte PL, Le PT. Molecular characterization of the mouse involuted thymus: aberrations in expression of transcription regulators in thymocyte and epithelial compartments. Int Immunol (2002) 14(7):813–22.
33. Albershardt TC, Iritani BM, Ruddell A. Evaluation of reference genes for quantitative PCR analysis of mouse lymphocytes. J Immunol Methods (2012) 384(1-2):196–9. doi: 10.1016/j.jim.2012.07.020
34. Kang DW, Choi CY, Cho YH, Tian H, Di Paolo G, Choi KY, et al. Targeting phospholipase D1 attenuates intestinal tumorigenesis by controlling beta-catenin signaling in cancer-initiating cells. J Exp Med (2015) 212(8):1219–37. doi: 10.1084/jem.20141254
35. Schmittgen TD, Livak KJ. Analyzing real-time PCR data by the comparative C(T) method. Nat Protoc (2008) 3(6):1101–8. doi: 10.1038/nprot.2008.73
36. Matz MV, Wright RM, Scott JG. No control genes required: Bayesian analysis of qRT-PCR data. PloS One (2013) 8(8):e71448. doi: 10.1371/journal.pone.0071448
37. Zook EC, Krishack PA, Zhang S, Zeleznik-Le NJ, Firulli AB, Witte PL, et al. Overexpression of Foxn1 attenuates age-associated thymic involution and prevents the expansion of peripheral CD4 memory T cells. Blood (2011) 118(22):5723–31. doi: 10.1182/blood-2011-03-342097
38. Lee YS, Jun HS. Anti-Inflammatory Effects of GLP-1-Based Therapies beyond Glucose Control. Mediators Inflammation (2016) 2016:3094642. doi: 10.1155/2016/3094642
39. Rosario W, D’Alessio D. An Innate Disposition for a Healthier Gut: GLP-1R Signaling in Intestinal Epithelial Lymphocytes. Diabetes (2015) 64(7):2329–31. doi: 10.2337/db15-0436
40. Zietek T, Rath E. Inflammation Meets Metabolic Disease: Gut Feeling Mediated by GLP-1. Front Immunol (2016) 7:154. doi: 10.3389/fimmu.2016.00154
41. Billing LJ, Smith CA, Larraufie P, Goldspink DA, Galvin S, Kay RG, et al. Co-storage and release of insulin-like peptide-5, glucagon-like peptide-1 and peptideYY from murine and human colonic enteroendocrine cells. Mol Metab (2018) 16:65–75. doi: 10.1016/j.molmet.2018.07.011
42. Larsson E, Tremaroli V, Lee YS, Koren O, Nookaew I, Fricker A, et al. Analysis of gut microbial regulation of host gene expression along the length of the gut and regulation of gut microbial ecology through MyD88. Gut (2012) 61(8):1124–31. doi: 10.1136/gutjnl-2011-301104
43. Wolpe SD, Sherry B, Juers D, Davatelis G, Yurt RW, Cerami A. Identification and characterization of macrophage inflammatory protein 2. Proc Natl Acad Sci USA (1989) 86(2):612–6. doi: 10.1073/pnas.86.2.612
44. Lacey DC, Achuthan A, Fleetwood AJ, Dinh H, Roiniotis J, Scholz GM, et al. Defining GM-CSF- and macrophage-CSF-dependent macrophage responses by in vitro models. J Immunol (2012) 188(11):5752–65. doi: 10.4049/jimmunol.1103426
45. Benahmed M, Meresse B, Arnulf B, Barbe U, Mention JJ, Verkarre V, et al. Inhibition of TGF-beta signaling by IL-15: a new role for IL-15 in the loss of immune homeostasis in celiac disease. Gastroenterology (2007) 132(3):994–1008. doi: 10.1053/j.gastro.2006.12.025
46. Jacobs SR, Michalek RD, Rathmell JC. IL-7 is essential for homeostatic control of T cell metabolism in vivo. J Immunol (2010) 184(7):3461–9. doi: 10.4049/jimmunol.0902593
47. Zhu J, Paul WE. Peripheral CD4+ T-cell differentiation regulated by networks of cytokines and transcription factors. Immunol Rev (2010) 238(1):247–62. doi: 10.1111/j.1600-065X.2010.00951.x
48. Romagnani S. The Th1/Th2 paradigm. Immunol Today (1997) 18(6):263–6. doi: 10.1016/S0167-5699(97)80019-9
49. Gaffen SL, Jain R, Garg AV, Cua DJ. The IL-23-IL-17 immune axis: from mechanisms to therapeutic testing. Nat Rev Immunol (2014) 14(9):585–600. doi: 10.1038/nri3707
50. Couper KN, Blount DG, Riley EM. IL-10: the master regulator of immunity to infection. J Immunol (2008) 180(9):5771–7. doi: 10.4049/jimmunol.180.9.5771
51. Cauvi DM, Cauvi G, Toomey CB, Jacquinet E, Pollard KM. From the Cover: Interplay Between IFN-gamma and IL-6 Impacts the Inflammatory Response and Expression of Interferon-Regulated Genes in Environmental-Induced Autoimmunity. Toxicol Sci (2017) 158(1):227–39. doi: 10.1093/toxsci/kfx083
52. Tessaro FH, Ayala TS, Nolasco EL, Bella LM, Martins JO. Insulin Influences LPS-Induced TNF-alpha and IL-6 Release Through Distinct Pathways in Mouse Macrophages from Different Compartments. Cell Physiol Biochem (2017) 42(5):2093–104. doi: 10.1159/000479904
Keywords: insulin-like peptide 5, relaxin family peptide receptor 4, immune markers, immune system, metabolic syndrome, gut peptide hormone
Citation: Vahkal B, Yegorov S, Onyilagha C, Donner J, Reddick D, Shrivastav A, Uzonna J and Good SV (2021) Immune System Effects of Insulin-Like Peptide 5 in a Mouse Model. Front. Endocrinol. 11:610672. doi: 10.3389/fendo.2020.610672
Received: 26 September 2020; Accepted: 16 November 2020;
Published: 14 January 2021.
Edited by:
Jiri Jiracek, Academy of Sciences of the Czech Republic (ASCR), CzechiaCopyright © 2021 Vahkal, Yegorov, Onyilagha, Donner, Reddick, Shrivastav, Uzonna and Good. This is an open-access article distributed under the terms of the Creative Commons Attribution License (CC BY). The use, distribution or reproduction in other forums is permitted, provided the original author(s) and the copyright owner(s) are credited and that the original publication in this journal is cited, in accordance with accepted academic practice. No use, distribution or reproduction is permitted which does not comply with these terms.
*Correspondence: Brett Vahkal, YnZhaGthbEB1b3R0YXdhLmNh; Sara V. Good, cy5nb29kQHV3aW5uaXBlZy5jYQ==
†Present address: Brett Vahkal, Department of Biochemistry, Microbiology and Immunology, University of Ottawa, Ottawa, ON, Canada