- 1Instituto de Inmunología, Genética y Metabolismo (INIGEM), Facultad de Farmacia y Bioquímica, Universidad de Buenos Aires (UBA), Consejo Nacional de Investigaciones Científicas y Técnicas (CONICET), Buenos Aires, Argentina
- 2Instituto de Investigaciones Biomédicas en Retrovirus y Sida (INBIRS), Facultad de Medicina, Universidad de Buenos Aires (UBA), Consejo Nacional de Investigaciones Científicas y Técnicas (CONICET), Buenos Aires, Argentina
Brucellosis is a prevalent global zoonotic infection but has far more impact in developing countries. The adipocytes are the most abundant cell type of adipose tissue and their secreted factors play an important role in several aspects of the innate and adaptive immune response. Here, we demonstrated the ability of Brucella abortus to infect and replicate in both adipocytes and its precursor cells (pre-adipocytes) derived from 3T3-L1 cell line. Additionally, infection of pre-adipocytes also inhibited adipogenesis in a mechanism independent of bacterial viability and dependent on lipidated outer membrane protein (L-Omp19). B. abortus infection was able to modulate the secretion of IL-6 and the matrix metalloproteases (MMPs) -2 and-9 in pre-adipocytes and adipocytes, and also modulated de transcription of adiponectin, leptin, and resistin in differentiated adipocytes. B. abortus-infected macrophages also modulate adipocyte differentiation involving a TNF-α dependent mechanism, thus suggesting a plausible interplay between B. abortus, adipocytes, and macrophages. In conclusion, B. abortus is able to alter adipogenesis process in adipocytes and its precursors directly after their infection, or merely their exposure to the B. abortus lipoproteins, and indirectly through soluble factors released by B. abortus-infected macrophages.
Introduction
Brucellosis is a prevalent global zoonotic infection but has far more impact in developing countries (1). Clinical manifestations of brucellosis are frequently associated with inflammatory responses in the organs affected (2). Adipose tissue represents one of the largest organs and constitutes up to 25% of the mass of the body in normal weight individuals (3). It is distributed throughout the body and is made up of different cell types that are involved in storing energy, regulating metabolism in addition to fulfilling neuroendocrine and immunological functions (3). Adipocytes secrete a multiplicy of adipokines, including adiponectin, leptin, and various cytokines such as IL-1β and IL-6. The resident immune cells—which include lymphocytes and macrophages—also secrete multiple inflammatory mediators, again including classical cytokines and chemokines (4, 5).
Adipocytes are the most abundant cell type of adipose tissue and their secreted factors play an important role in several aspects of the innate and adaptive immune response among other functions (6). Therefore, these cells could participate in the modulation of the immune response during Brucella infection. On the other hand, adipocytes are specialized for fat storage regulating the balance of energy and homeostasis (7). The pathogens could take advantage of this characteristic, turning adipose tissue into a survival niche. In fact, Mycobacterium tuberculosis, Trypanosoma cruzi, influenza virus A, Chlamydia pneumoniae, HIV -among other pathogens- are housed in adipose tissue (8–11). The cell and tissue in which Brucella persists during chronic infection remain largely unknown. Most studies describe the location of the bacteria based on the site of isolation or the histopathology of the disease, but the place where the bacterium resides has not been elucidated (12, 13).
Adipocytes differentiate from mesenchymal stem cells. During the differentiation process, the transcriptional factor CCAAT/enhancer-binding protein (C/EBP)β is transiently induced leading to activation of two master adipogenic transcription factors, peroxisome proliferator-activated receptor (PPAR)γ, and C/EBPα. These mediators stimulate each other to activate the transcription of genes implicated in lipid metabolism (14). Adipocyte differentiation is regulated by hormones, cytokines, growth factors, and also by matrix metalloproteinase (MMPs) (15, 16). A recent study performed in canine fetuses and neonates naturally infected with B. canis revealed its intracellular localization in adipocytes. B. canis was localized near to the lipid droplet and in the same place of the endoplasmic reticulum. The adipocytes from neonates and fetuses are immature and present features of pre-adipocytes. During their differentiation process, the pre-adipocytes express unfolded proteins as occurs during the intracellular replication of Brucella spp. (17, 18). This finding suggests that pre-adipocytes could be a replicative niche for Brucella spp. during the differentiation process. In the present study, we investigate whether B. abortus can infect and survive in differentiated adipocyte and its precursors, as well as the incumbency on both adipogenesis and immune response modulation, on the inflammatory response during brucellosis.
Materials and Methods
Bacterial Culture
Brucella abortus S2308, DsRed-expressing B. abortus 2308 (provided by Diego Comerci, UNSAM University, Argentina), were grown for 18 h in 10 ml tryptic soy agar supplemented with yeast extract (Merck) with constant agitation (150 rpm) at 37°C. Bacteria were collected and inoculums prepared as previously described (19). To obtain heat-killed B. abortus (HKBA), bacteria were washed with sterile physiological solution and heat-killed at 70°C for 20 min. Absence of bacterial viability was verified by the lack of its in vitro growth on trypticase soy agar (TSA). Live B. abortus manipulations were performed in biosafety level 3 facilities.
Cell Culture
3T3-L1 fibroblasts were obtained from the American Type Culture Collection (ATCC, Manassas, VA) and were culture in DMEM (Gibco) containing 10% of heat-inactivated fetal bovine serum (FBS) (Gibco), 2 mM of L-glutamine (Gibco), 1 mM of sodium pyruvate (Gibco), and penicillin-streptomycin. The murine macrophage cell line J774 was grown in DMEM with 10% FBS and supplemented as previously described.
Adipocyte Differentiation
3T3-L1 cells were seeded at 5 x 104 cells/well in 24-well plates and allowed to reach confluence. After 2 days (day 0), the medium was changed to differentiation medium (DMEM, 0.5 mM 3-isobutyl-1-methylxanthine (IBMX), 1 µM dexamethasone (DM), and 1 µg/ml human insulin), all from SIGMA. At day 2, the medium was replaced by a maintenance medium (10% FBS and 1 µg/ml insulin). Full differentiation was reached at day 10–15.
Adipocyte differentiation was evaluated by oil red O staining (Sigma). Cultures in 24-well plates were fixed for 1 h with 10% formalin and then washed with 60% isopropanol, stained for 30 min by complete immersion in a working solution of 6% oil red O, and wash repeatedly with water. Ten microscopic fields per well in three wells per condition were quantified for each experiment. The percentage of adipocytes was calculated for the nontreated/noninfected controls.
Cellular Infection
3T3-L1 preadipocytes and adipocytes were separately seeded in two different densities: 2x104 cells per well in 24 well plate, and 1x105 cell per well in 6 well plate. Besides, J774.A1 macrophages were seeded at a density of 3x105 cells per well in 24 well plates. These three cell types were infected with B. abortus S2308 or DsRed-expressing B. abortus S2308 at different multiplicities of infection (MOI) 100 to 1,000 or at MOI 100 for J774.A1 cell line. After the bacterial suspension was dispensed, the plates were centrifuged for 10 min at 2,000 rpm and then incubated for 2 h at 37°C under a 5% CO2 atmosphere. Cells were extensively washed with DMEM to remove extracellular bacteria and incubated in medium supplemented with 100 μg/ml gentamicin and 50 μg/ml streptomycin to kill extracellular bacteria. Adipocytes and preadipocytes were harvested at different times to determine cytokine production, MMP secretion, lipid droplets staining and adiponectin, leptin, PPAR-γ, C/EBP-α, C/EBP-β gene transcription. Supernatants from J774.A1 macrophages were harvested at 24 h post-infection and sterilized by filtration through a 0.22 nitrocellulose filter. To evaluate the intracellular replication of B. abortus, the infected cells were washed and lysed at different time post-infection with 0.2% (vol/vol) of triton X-100. The number of viable intracellular bacteria was determined by the count of CFU/ml from serial dilutions to the tenth in TSA plates.
Brucella-Derived Lipoproteins and LPS
B. abortus L-Omp19 and U-Omp19 were obtained as previously described (20). Both recombinant proteins contained 0.25 endotoxin U/µg protein, as assessed by Limulus amoebocyte lysates (Associates of Cape Cod, East Falmouth, MA, USA). Protein concentration was determined by the BCA method (Pierce, Rockford, IL, USA). Ignacio Moriyon from the University of Navarra, Pamplona, Spain kindly provided B. abortus S2308 LPS and E. coli O111K58H2 LPS. Pam3Cys was acquired from Boehringer Mannheim (Indianapolis, IN, USA).
Confocal Microscopy
3T3-L1 differentiated cells seeded onto glass coverslips were infected with DsRed expressing-B. abortus S2308 at MOI 100 as was previously described. At different time points, cells were fixed with paraformaldehyde, permeabilized with 0.3% Triton X-100, and then lipid droplets were stained with 1 µg/ml of Bodipy 493/503 (Invitrogen), and nucleus were stained with TO-PRO®-3 (Invitrogen). The coverslips were mounted in PBS-glycerin (9:1 vol/vol) and analyzed in a FV-1000 confocal microscope with an oil-immersion Plan Apochromatic 60X NA1.42 objective (Olympus). Ten microscopic fields per well in 3 wells per condition were quantified for each experiment. The percentage of adipocytes was calculated to the noninfected controls. To determine B. abortus replication we visualized DsRed-expressing B. abortus positive areas by confocal microscopy and quantified using Image J software (National Institutes of Health).
The amount and individual diameter size of the lipid droplets in the image were measured using Image J software and data were loaded into GraphPad Prism 5.0 (GraphPad Software, La Jolla, CA, USA) and evaluated for average lipid droplet size and size-frequency distribution for individual adipocytes. The adipocytes containing lipid droplets with a mean diameter >1 µM were classified as cells with big lipid droplets size.
Measurement of Cytokine Concentrations
Secretion of IL-1β, IL-6, and TNF-α were quantified by ELISA in culture supernatants following the manufacturer’s instructions (BD Pharmingen, San Diego, CA).
No cross-reactivity with other mouse cytokines was identified. For IL1β ELISA were tested IL-1β IL-2, IL-3, IL-4, IL-5, IL-6, IL-7, IL-9, IL-10, IL-12 (p70), IL-15, IFN-γ, MCP-1, TCA3, and TNF-α; for IL-6 ELISA were tested IL-1β, IL-2, IL-3, IL-4, IL-5, IL-7, IL-9, IL-10, IL-12 (p70), IL-15, IFN-γ, GM-CSF, MCP-1, TCA3, and TNF-α; for IL1 β ELISA were tested IL-2, IL-3, IL-4, IL-5, IL-6, IL-7, IL-9, IL-10, IL-12 (p70), IL-15, IFN-γ, MCP-1, TCA3, and TNF-α. Sensitivity for IL-1 β, IL-6, and TNF-α the limit of detection is 15.6 pg/ml.
Zymography
Gelatinase activity was assayed by the method of Hibbs et al. with modifications, as described (21, 22). Briefly, a total of 20 μl of cell culture supernatants from infected cells or untreated controls were mixed with 5 μl of 5X loading buffer [0.25 M Tris (pH 6.8), 50% glycerol, 5% SDS, and bromophenol blue crystals] and loaded onto 10% SDS-PAGE gels containing 1 mg/ml gelatin (Sigma-Aldrich, Buenos Aires, Argentina). Following electrophoresis, gels were washed with a solution containing 50 mM Tris-HCl (pH 7.5) and 2.5% Triton X-100 (buffer A) for 30 min and with buffer A added with 5 mM CaCl2 and 1 μM ZnCl2 for 30 min and were later incubated with buffer A with additional 10 mM CaCl2 and 200 mM NaCl for 48 h at 37°C. Gelatin activity was visualized by the staining of the gels with 0.5% Coomassie blue. Unstained bands indicated the presence of gelatinase activity.
Gelatinase Activity Under Native Conditions
Gelatinase activity in unprocessed culture supernatants (native conditions) was measured by using a gelatinase/collagenase fluorometric assay kit (EnzChek; Invitrogen, Carlsbad, CA) according to the manufacturer’s instructions. The EnzChek kit contains DQ gelatin, a fluorescein-conjugated gelatin so heavily labeled with fluorescein that fluorescence is quenched. When this substrate is digested by gelatinases or collagenases it yields highly fluorescent peptides, and fluorescence increase is proportional to proteolytic activity. Collagenase purified from Clostridium histolyticum provided in the assay kit serves as a control enzyme. Plates were read in a fluorescence plate reader (Victor3; Perkin-Elmer, Waltham, MA).
mRNA Extraction and Quantitative Real-Time PCR
Total RNA was extracted from cells using the kit Quick-RNA MiniPrep Kit (Zymo Research) according to the manufacturer’s instructions. cDNA was synthesized from 1 μg total RNA with the enzyme reverse transcriptase Improm-II (Promega). Real-time PCR was done with a SYBR green as a DNA binding fluorescent dye using a StepOne Real-Time PCR System (Applied Biosystems). The pairs of primers used were the following: adiponectin sense:5´-GACGACACCAAAAGGGCTCA-3´, antisense: 5´-GAGTGCCATCTCTGCCATCA-3´, leptin sense: 5´-TCCCTGCCTCAGACCAGTG-3´, antisense: 5´-TAGAGTGAGGCTTCCAGGACG-3´, PPAR-γ sense: 5´-CTGATGGCATTGTGAGACAT-3´, antisense: 5´-ATGTCTCACAATGCCATCAG-3´, C/EBP-α sense: 5´-TGTGCGAGCACGAGACGTC-3´, antisense: 5´-AACTCGTCGTTGAAGGCGG-3´, C/EBP-β sense: 5´-GCTGAGCGACGAGTACAAGA-3´, antisense: 5´-CAGCTCCAGCACCTTGTG-3´, β-actin sense: 5´-AACAGTCCGCCTAGAAGCAC-3´, antisense: 5´-CGTTGACATCCGTAAAGACC-3´.
The amplification cycle for adiponectin, leptin, PPAR-γ, C/EBP-α, and C/EBP-β was the following 10 min 95 °C, 40 cycles for 15 s at 95°C, 60°C for 30 s, and 72°C for 60 s. All primer sets yielded a single product of the correct size. The fold change (relative expression) in gene expression was calculated using the relative quantitation method (2−ΔΔCt). Relative expression levels were normalized against β-actin. Intra experiment CT values differences between samples were less than 0.5.
Statistical Analysis
Each experiment was performed at least three times with different culture preparations. Data were represented as mean ± SD measured in triplicate from at least three individual experiments. Statistical analysis was performed with one-way ANOVA. Multiple comparisons between all pairs of groups were made with Tukey’s posttest, and those against two groups were made with Student᾽s t test, Mann-Whitney test. To determine normality, the Shapiro-Wilk normality test was used. Graphical and statistical analyses were performed with GraphPad Prism 5.0 software. P<0.05 was the minimum level for accepting a statistically significant difference between groups.
Results
B. abortus Infects and Replicates in Both Pre-Adipocytes and Adipocytes, and Inhibits Adipogenesis
There are no former reports about the interaction between B. abortus and adipogenic cells. Thus, we first determined the differential capacity of B. abortus to infect pre-adipocytes and adipocytes. As shown in Figures 1A. B. abortus was internalized by both, pre-adipocytes and adipocytes in vitro. However, the multiplication efficiency was dissimilar between them. In pre-adipocytes the number of intracellular bacteria was increased by more than one log at 24 h post-infection and continued growing thereafter. By contrast, adipocytes were less permissive for B. abortus growth, and the number of bacteria in adipocytes was significantly lower than that observed for pre-adipocytes (p < 0.01). The number of intracellular bacteria in adipocytes increased by one log at 48 h post-infection and then decline.
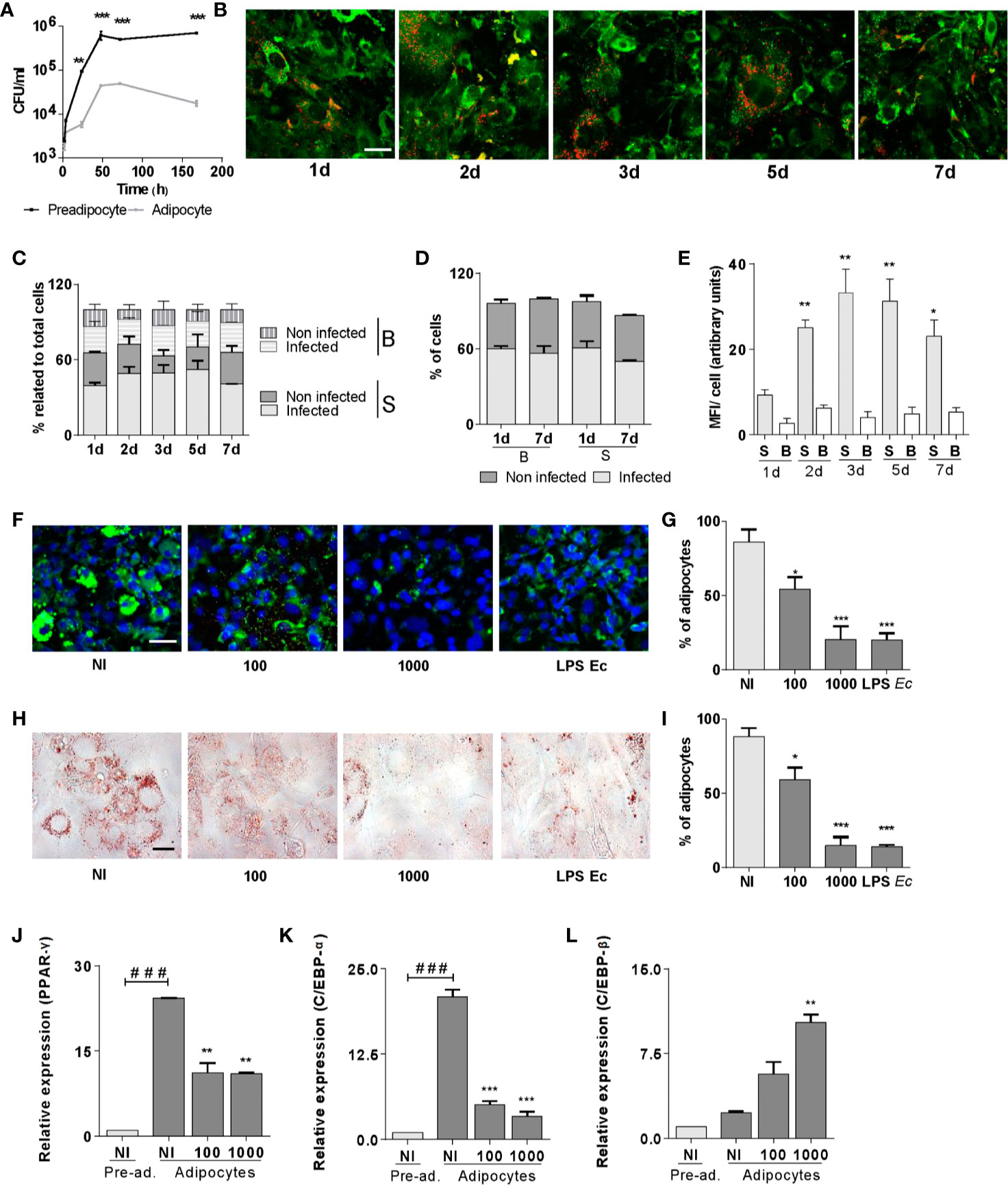
Figure 1 B. abortus replicates in pre-adipocytes and adipocytes and inhibits adipogenesis. Infection with B. abortus was performed at a multiplicity of infection (MOI)= 100, and CFU was determined at different times of infection in pre-adipocytes and adipocytes. (A). Intracellular DsRed-expressing B. abortus in adipocytes assessed by confocal microscopy, lipid droplets were stained with Bodipy 493/503 (B). Quantification of the experiment performed in B (C–E). Percentage of infected and non-infected adipocytes with small (S) and big (B) lipids droplets respect to total cells at each time (C). Percentage of infected and non-infected cells at 1 and 7 d post infection present in adipocytes with S or B droplets cells respect to total S o B cells at each time. Quantification of bacterial replication is measured as means of fluorescence intensity (MFI) (E). Effect of B. abortus infection at MOI 100 and 1,000 on adipocyte differentiation determined by lipid droplets staining with Bodipy 493/503 (F), quantified by cell counts (G) and Oil Red O staining (H), quantified by cell counts (I). Effect of B. abortus infection on PPAR-γ (J), C/EBP-α (K), and C/EBP-β (L) transcription determined by RT-qPCR in adipocytes after 24 h post-infection. LPS from E. coli (LPS Ec) was used as a positive control. Scale bar: 30 µm. Data are given as the mean ± SD measured in triplicate from at least three individual experiments. Ten microscopic fields per condition were quantified for each experiment. Data shown are from a representative experiment of three performed. *P < 0.05; **P < 0.01; ***P < 0.001vs non infected cells (NI). ###P< 0.001 vs non infected pre-adipocytes (NI/Pre-ad.).
At seven days of adipogenic differentiation, the culture is heterogeneous with cells that present small and big lipid droplets (more than 1 µm in diameter). At this time, cells were infected with DsRed-expressing B. abortus and lipid droplets were stained with Bodipy 493/503, and cells were evaluated at different times post-infection. Our results indicate that B. abortus invades adipocytes in a manner that was independent of the lipid droplets size (Figures 1B–D). However, B. abortus preferentially replicates in adipocytes with small lipid droplets (Figure 1E).
To assess whether the infection affects adipocyte differentiation, pre-adipocytes were infected with B. abortus at MOI 100 and 1,000, in the presence of differentiation medium for 2 days, and then incubated with maintenance medium. E. coli LPS was used as a control. At 15 days post-infection cells were fixed. The presence of differentiated adipocytes was revealed by lipid droplets staining with Bodipy 493/503 and Oil Red O (Figures 1F–I). B. abortus infection inhibited adipocyte differentiation as was revealed by a reduction of lipid droplets formation.
Given the ability of B. abortus infection to inhibit adipocyte differentiation, subsequent experiments were carried out to determine whether such infection could also modulate the transcription of the essential pro-adipogenic factors C/EBP-α, C/EBP-β, and PPAR-γ (14). For this purpose, pre-adipocytes were infected, incubated with a differentiation/maintenance medium, and mRNA levels of mentioned pro-adipogenic factors were measured at 15 days. B. abortus infection promoted a decrease in the transcription of C/EBP-α and PPAR-γ genes despite an increase in the transcription of C/EBP-β gen (Figures 1J–L). Altogether, these results indicate that B. abortus replicates preferentially in pre-adipocytes slowing down their normal adipogenesis.
B. abortus Infection Modulates Proinflammatory Cytokines and Adipokines Secretion in Pre-Adipocytes and Adipocytes
The adipose tissue physiology is committed to the expression of cytokines and adipokines. They not only control fat metabolism but also immune homeostasis (6). Infection with B. abortus at MOI 100 and 1,000 induced IL-6 (Figures 2A, B) but not IL-1β nor TNF-α (not shown) secretion by both pre-adipocytes and adipocytes. In adipocytes, the mRNA transcription level of leptin remained unaltered after the B. abortus infection, but the levels of mRNA of adiponectin and resistin are significantly (p < 0.01) inhibited respect to uninfected control cells (Figures 2C–E). This is in concordance with the inhibitory effect of B. abortus infection on adipogenesis, since transcription of adipokine genes are controlled by the transcription factor PPAR-γ (23). These results indicate that B. abortus infection modulates the expression of cytokines and adipokines in pre-adipocytes and adipocytes.
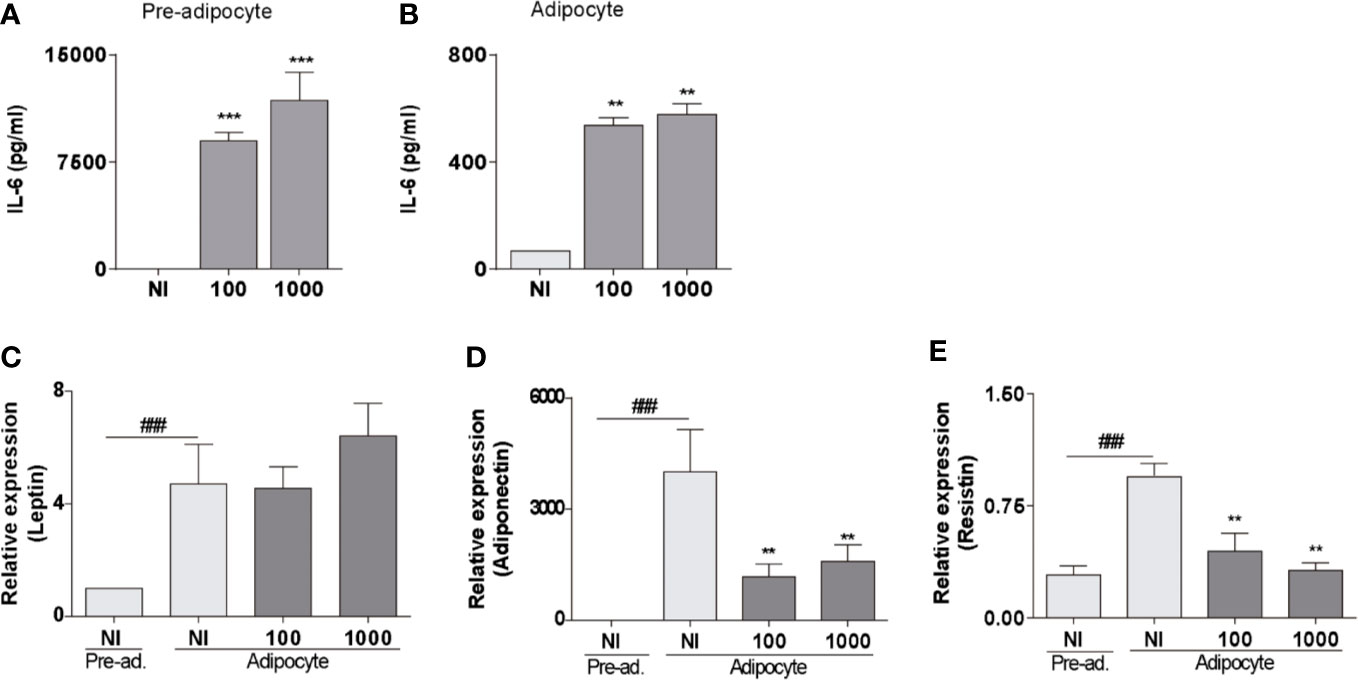
Figure 2 B. abortus infection modulates proinflammatory cytokines and adipokines secretion in pre-adipocytes and adipocytes. ELISA determination of IL-6 in pre-adipocytes (A) and adipocytes (B) measured in culture supernatants at 24 h post-infection. Determination of leptin (C), adiponectin (D), and resistin (E) by RT-qPCR in B. abortus -infected adipocyte cells at MOI 100 and 1,000 at 24 h post-infection. Data are given as the mean ± SD measured in triplicate from five individual experiments. Data shown are from a representative experiment of five performed **P < 0.01; ***P < 0.001vs non infected cells (NI). ###P< 0.001 vs non infected pre-adipocytes (NI/Pre-ad.)
B. abortus Infection Induces MMPs Secretion in Pre-Adipocytes but Was Unable to Modulate MMPs in Adipocytes
Cytokines and adipokines have been shown to stimulate MMPs secretion from different cell types (24–30). Additionally, pre-adipocytes and adipocytes can produce MMP-2 and MMP-9 (15). To analyze whether B. abortus is capable of modulating their MMP-2 and MMP-9 activity, separated pre-adipocyte and adipocyte cells were infected with B. abortus at MOI 100 and 1,000, and after 24 h MMPs activity was evaluated, in culture supernatants, by gelatin zymography. Supernatants, from B. abortus-infected pre-adipocyte displayed a significant increase in MMP-2 and MMP-9 gelatinase activity than that of uninfected cells (Figures 3A–C). By contrast, in adipocytes, no further increase of the MMP-2 and MMP-9 activity was detected for any MOI of infection (Figures 3D–F). The activity of MMPs in vivo is counterbalanced by the tissue inhibitor’s action including TIMPs (31). Therefore, the net gelatinase or collagenase activity in a complex sample, such as culture supernatants, depends on the balance between MMP and TIMP activities. This net activity is not revealed by zymography, since MMP-TIMP complexes may dissociate during gel electrophoresis. To assess whether the environment surrounding Brucella-infected pre-adipocytes and adipocytes has an increased net gelatinase activity, culture supernatants from these cells were incubated with a non-fluorescent gelatin-fluorescein conjugate, and the fluorescence unmasked as a consequence of gelatin degradation was measured in a fluorometer. Our results indicated that enzymatic activity is increased significantly in B. abortus-infected supernatants from pre-adipocytes supernatants but it remained unaltered in supernatants from B. abortus-infected adipocytes (Figures 3G, H). Therefore, B. abortus infection increases the MMP-2 and MMP-9 activity in pre-adipocytes, but no in adipocytes.
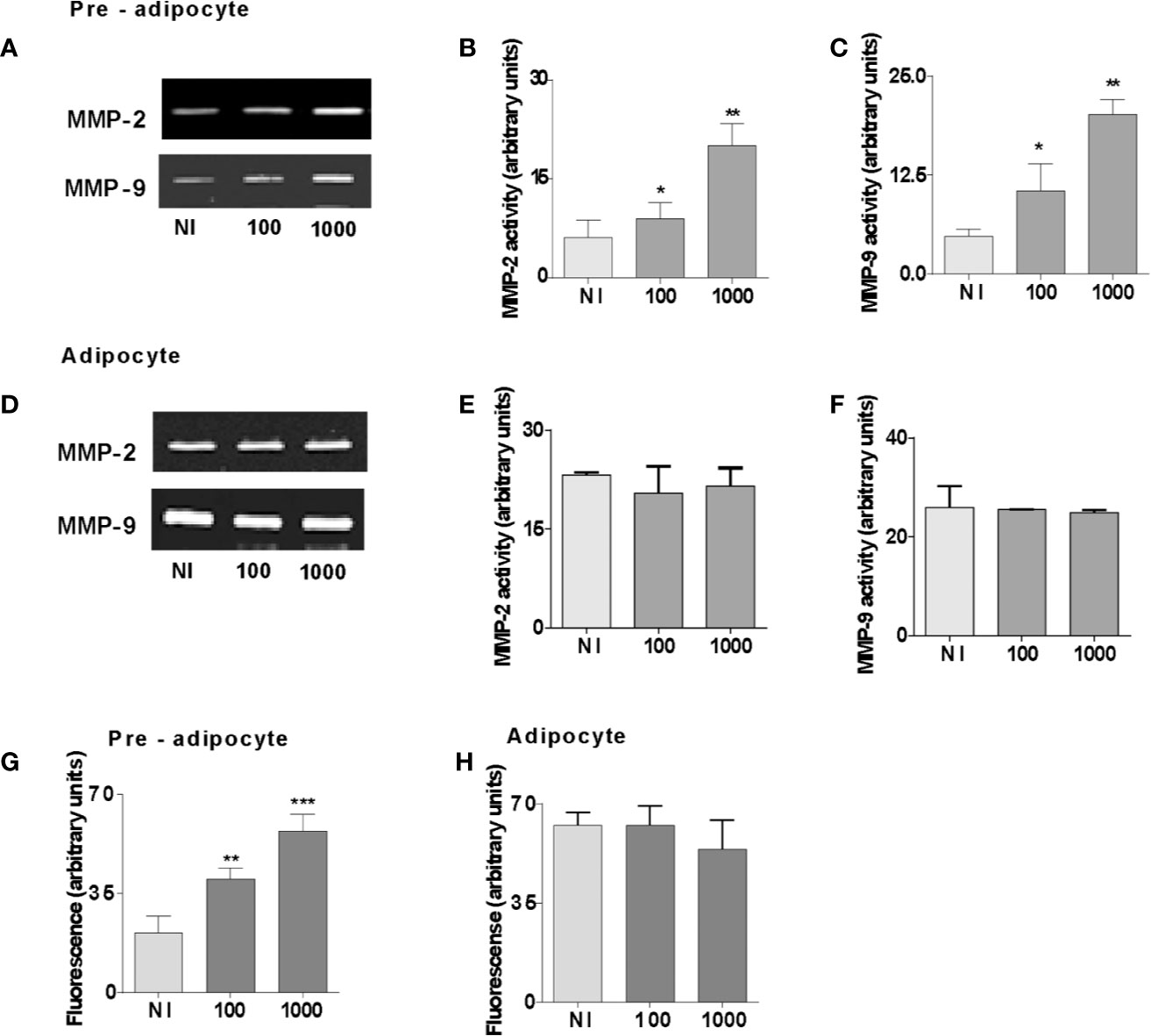
Figure 3 B. abortus infection induces MMPs secretion in pre-adipocytes but was unable to modulate MMPs in adipocytes. MMP-2 and MMP-9 activity were measured by gelatin zymography in culture supernatants from B. abortus-infected preadipocytes (A) and adipocytes (D) at 24 h post-infection. (B, C, E, and F). Densitometric analysis of results from three independent experiments performed in A and D. For MMP activities, the culture supernatants from B. abortus infected preadipocytes (G) and adipocytes (H) with a fluorescein-conjugated gelatin substrate that produces highly fluorescent peptides when gelatin is digested. Data are expressed in fluorescence units informed by the fluorometer. Data are given as the mean ± SD measured in triplicate from four individual experiments. Data shown are from a representative experiment of five performed *P < 0.05; **P < 0.01; ***P < 0.001 vs non infected cells (NI).
B. abortus Lipoproteins Inhibit Adipocyte Differentiation
Previously, we have reported that Brucella lipoproteins are crucial for many responses induced by B. abortus infection (19–21, 32–36). Additionally, adipogenesis inhibition may be a process involving TLR-ligand interactions (37, 38). At first, we evaluated whether viable B. abortus was necessary to inhibit adipocyte differentiation. Oil red staining of adipocytes differentiated in the presence of HKBA revealed a markedly reduced adipocyte differentiation compared with the unstimulated cells. We further assessed the B. abortus LPS or its lipoproteins involvement on adipocyte differentiation inhibition. To this end, pre-adipocytes were differentiated in the presence of B. abortus LPS, or B. abortus lipidated outer membrane protein 19 (L-Omp19), used as a model of Brucella lipoprotein, and compared against unlipidated (U)-Omp19 (20). LPS from E. coli and Pamp3Cys were used as positive controls. As shown in Figure 4, L-Omp19, but not B. abortus LPS, mimicked the inhibition of lipid droplets accumulation induced by B. abortus infection. Nonetheless, the U-Omp19 was not capable to inhibit lipid droplets accumulation (Figure 4). Together these results indicate that the lipoproteins (L-Omp19) from B. abortus but not its LPS participates in the inhibition of adipogenesis among infected pre-adipocytes.
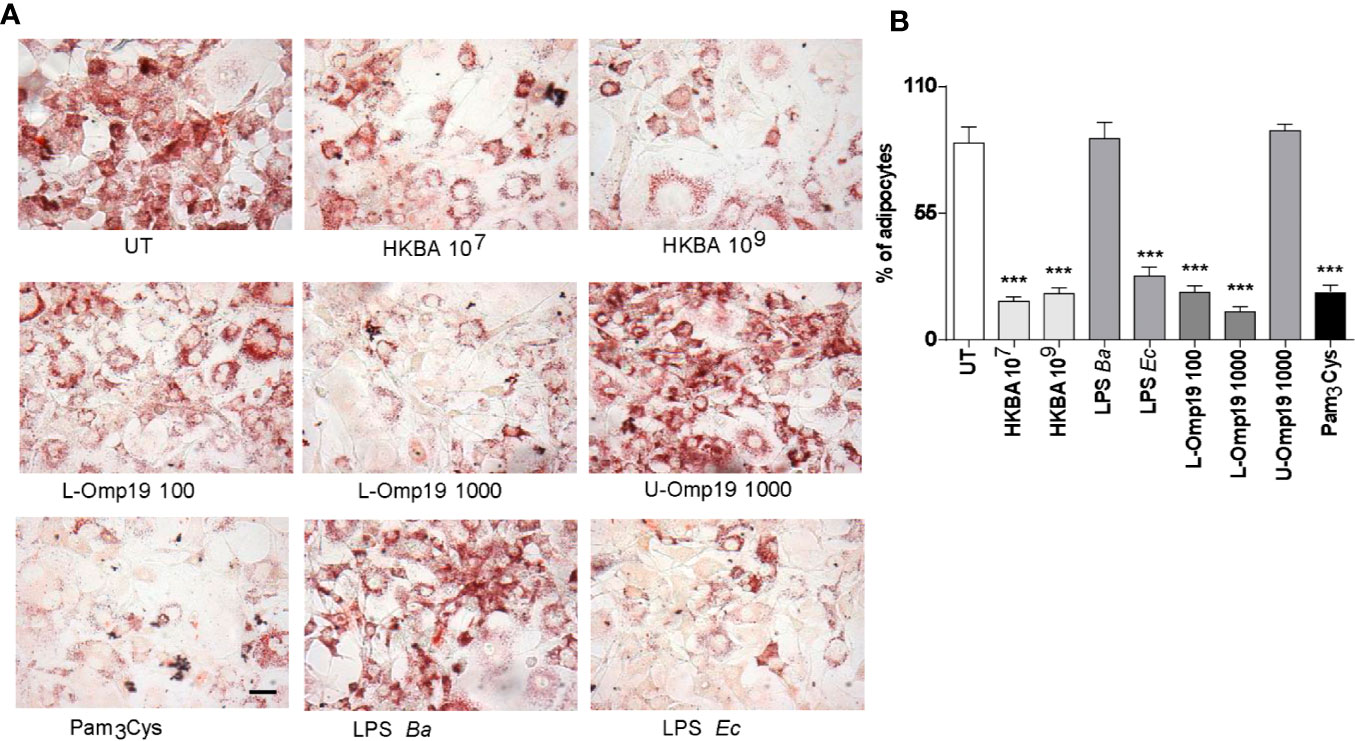
Figure 4 B. abortus lipoproteins inhibits adipocyte differentiation. Pre-adipocytes were differentiated in the presence of HKBA (1x106 and 1x109 bacteria/ml) and E. coli LPS (LPS Ec) (10 ng/ml), B. abortus LPS (LPS Ba) (1,000 ng/ml), L-Omp19 (10, 100, or 1,000 ng/ml), U-Omp19 (1,000 ng/ml), or Pam3Cys (50 ng/ml) or untreated (UT). The presence of adipocytes was revealed by staining of lipid droplets with Oil Red O (A). Quantification of Oil Red O was determined by cell counts (B). Ten microscopic fields per well in three wells per condition were quantified for each experiment. The percentage of adipocytes was calculated to the untreated cells (UT). Scale bar: 30 µm. Data are given as the mean ± SD measured in triplicate from five individual experiments. Data shown are from a representative experiment of five performed ***P < 0.001 vs untreated cells (UT).
B. abortus-Infected Macrophages Modulate Adipocyte Differentiation
Macrophages constitute the main host cell for B. abortus replication (39). We hypothesized that B. abortus-infected macrophages release soluble mediators that may affect adipocyte differentiation. For this goal, the adipocyte differentiation process was studied in the presence of conditioned media from B. abortus-infected macrophages, or uninfected macrophages as control. As shown in Figure 5, the adipocyte differentiation was significantly reduced by conditioned media from B. abortus-infected macrophages but it was not modified by conditioned media from uninfected macrophages (Figure 5).
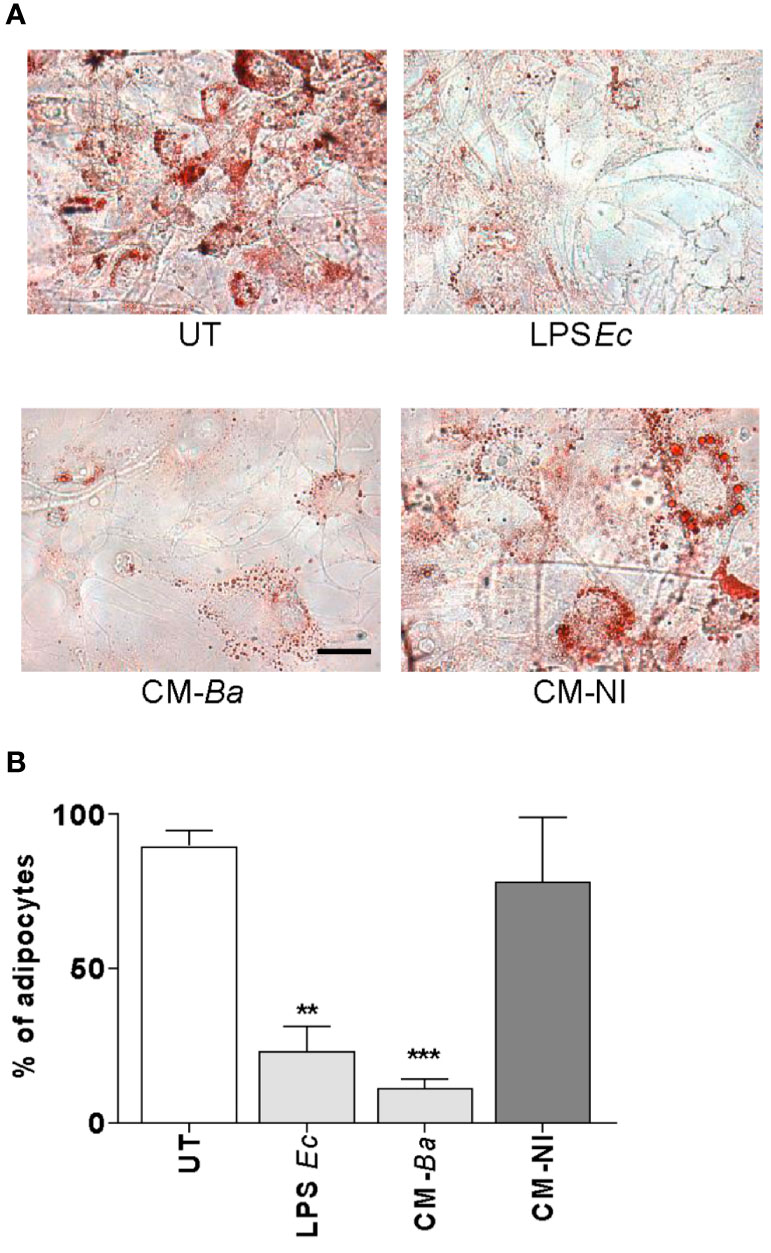
Figure 5 B. abortus-infected macrophages modulate adipocyte differentiation. Pre-adipocytes were differentiated in the presence of culture supernatants from B. abortus infected macrophages at MOI 100 (CM-Ba) or culture supernatants from non-infected macrophages as control (CM-NI). The presence of adipocytes was revealed by staining of lipid droplets with Oil Red O (A). Quantification of Oil Red O was determined by cell counts (B). LPS from E. coli (LPS Ec) was used as a positive control. Ten microscopic fields per well in three wells per condition were quantified for each experiment. The percentage of adipocytes was calculated with respect to untreated cells (UT). Scale bar: 30 µm. Data are given as the mean ± SD measured in triplicate from five individual experiments. Data shown are from a representative experiment of five performed. **P < 0.01; ***P < 0.001 vs untreated cells (UT).
To determine whether conditioned media from B. abortus-infected macrophages affects at early and/or late stage of the adipocyte differentiation process, we added the conditioned media from B. abortus-infected macrophages during the incubation with adipocyte differentiation medium or, during the cultivation with maintenance medium. As a control, conditioned media from uninfected macrophages was included. The adipocyte differentiation was inhibited when conditioned media from B. abortus-infected macrophages was added during the culture of cells with adipocyte differentiation media indicating that suppresses the pre-adipocyte differentiation at an early stage while the addition of conditioned medium during the cultivation with maintenance medium did not affect adipocyte differentiation (Figure 6). Together, these results indicate that soluble factors released from B. abortus- infected macrophages inhibit adipogenesis at an early state.
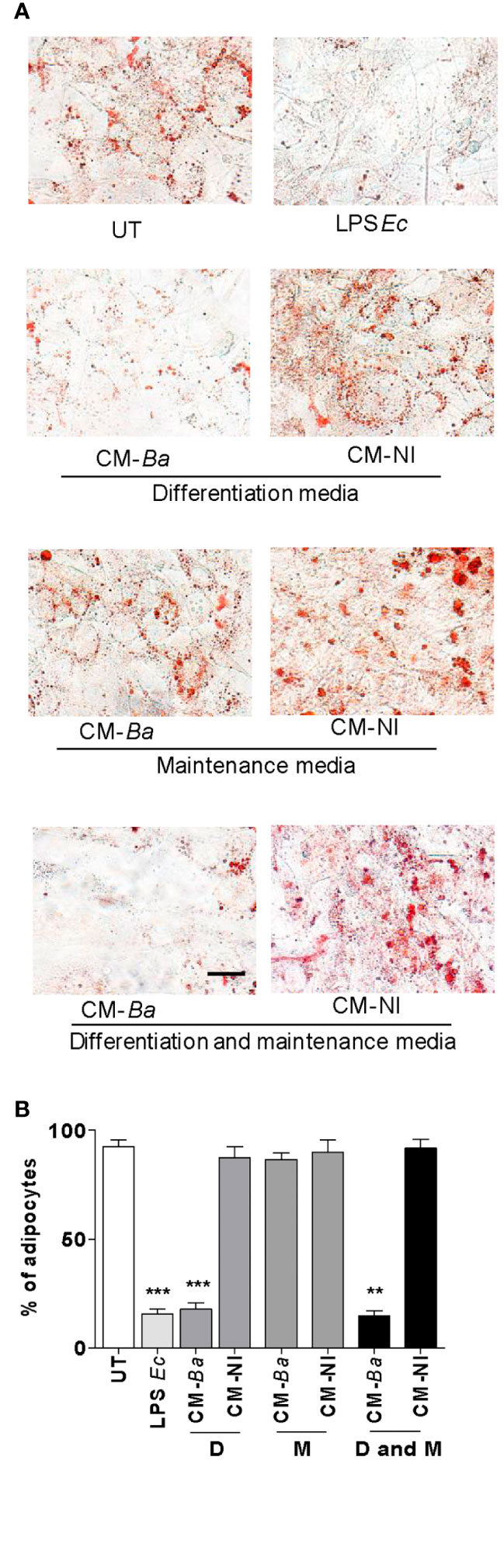
Figure 6 B. abortus-infected macrophages modulate early adipocyte differentiation. Pre-adipocytes were differentiated in the presence of culture supernatants from B. abortus—infected macrophages at MOI 100 (CM-Ba) or culture supernatants from non-infected macrophages as control (CM-NI). Supernatants were added during the early differentiation process (differentiation media, D), later differentiation process (maintenance media, M) or during all differentiation process (differentiation and maintenance media, D and M). The presence of adipocytes was revealed by staining of lipid droplets with Oil Red O (A). Quantification of Oil Red O was determined by cell counts (B). LPS from E. coli (LPS Ec) was used as a positive control. Ten microscopic fields per well in three wells per condition were quantified for each experiment. The percentage of adipocytes was calculated to the untreated cells (UT). Scale bar: 30 µm. Data are given as the mean ± SD from three individual experiments. **P < 0.01; ***P < 0.001 vs untreated cells (UT).
B. abortus-Infected Macrophages Inhibit Adipocyte Differentiation via a Mechanism Dependent on TNF-α
TNF-α is a proinflammatory cytokine able to downregulate the adipocyte differentiation (40). Previously, we have reported that B. abortus-infected macrophages secrete TNF-α (21, 32). To test whether TNF-α released from B. abortus-infected macrophages inhibits adipogenesis, we performed experiments with culture supernatants preincubated with a TNF-α neutralizing antibodies. As shown in Figure 7, the inhibitory effect of B. abortus-infected macrophages on adipocyte differentiation was inhibited by the treatment with TNF-α neutralizing antibody. Also, the isotype control does not affect. Thus, we concluded that TNF-α plays a key role in the inhibition of adipogenesis induced by macrophages-infected with B. abortus.
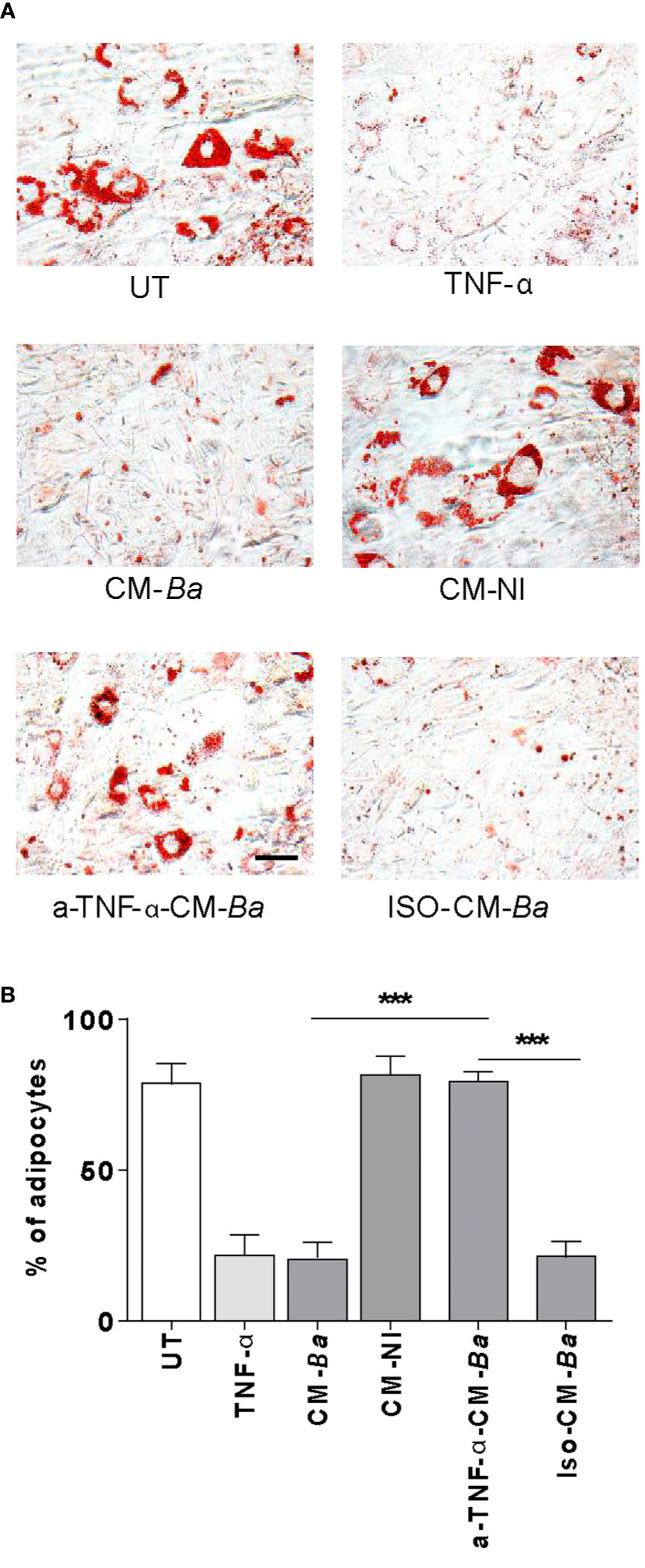
Figure 7 B. abortus-infected macrophages inhibit adipocyte differentiation via a mechanism dependent on TNF-α. Pre-adipocytes were differentiated in the presence of culture supernatants from B. abortus-infected macrophages at MOI 100 (CM-Ba) or culture supernatants from non-infected macrophages as control (CM-NI). Supernatants were incubated with an anti-TNF-α neutralizing antibody (a-TNF-α-CM-Ba) or isotype control (ISO-CM-Ba). TNF-α (1 ng/ml) was used as a positive control. The presence of adipocytes was revealed by staining of lipid droplets with Oil Red O (A). Quantification of Oil Red O was determined by cell counts (B). Ten microscopic fields per well in three wells per condition were quantified for each experiment. The percentage of adipocytes was calculated to the untreated cells (UT). Scale bar: 30 µm. Data are given as the mean ± SD from three individual experiments. ***P < 0.001.
Discussion
The role of adipose tissue in the pathogenesis of infectious diseases has increased attention in recent years (41–45). Adipose tissue constitutes a nutritionally rich organ for the survival of pathogens such as Mycobacterium tuberculosis, Trypanosoma cruzi, HIV, among others (9, 46, 47). Cell types composing adipose tissue include, fibroblasts, smooth muscle, endothelial, and immune cells. In the setting of infectious or non-infectious diseases like diabetes or obesity, adipose-resident immune cells are derived to a proinflammatory phenotype due to a proinflammatory microenvironment. However, adipocytes are the most abundant cell type (48).
Cell types composing adipose tissue include, fibroblasts, smooth muscle, endothelial and immune cells. In the setting of infectious or non-infectious diseases like diabetes or obesity, adipose-resident immune cells are derived to a proinflammatory phenotype due to a proinflammatory microenvironment.
In brucellosis, the tissue and cell type in which the bacteria persist during chronic infection remains unknown. Previous findings have reported the capacity of B. abortus for intracellular replication in several cell types (17, 21, 49). In particular, the Brucella capability to localize intracellularly in adipocytes and its precursors was recently revealed for B. canis (13, 17). Here, we show that B. abortus infects and replicates in both adipocyte and even with higher efficiency, in pre-adipocyte.
An impairment in adipogenesis process may involve an adipokine misbalance induced by B. abortus infection. Adiponectin and resistin have been associated with adipogenesis (50, 51). Leptin acts largely on the hypothalamus, informing the nutritional status of the adipocytes and controlling adipokines and the endocrine role of adipose tissues and food intake, thus regulating energy balance (23). Additionally, leptin could significantly reduce lipid accumulation during the adipocyte differentiation process, indicating an inhibitory effect on adipogenesis. The loss of expression of adipokines and the acquisition of an inflammatory phenotype may also involve the secretion of IL-1β, TNF-α, and IL-6 (52). Here, we have demonstrated an increased secretion of adipokines such as IL-6, but not IL-1β and TNF-α, among infected pre-adipocytes and adipocytes after B. abortus infection, being higher among the former. Likewise, pre-adipocytes depict a higher number of intracellular bacteria than adipocyte cells. Considering that IL-6 could be able to inhibit the anti-inflammatory adipokines adiponectin and resistin in an autocrine and paracrine manner (53), we have measured their transcriptional level after B. abortus infection. For both, the mRNA level appeared significantly diminished after infection. However, although IL-6 also induces leptin production (54, 55), leptin levels were not significantly altered after infection with Brucella abortus. Several transcription regulators, such as PPAR-γ, C/EBP-α, and C/EBP-β are well-known to mediate adipogenesis. The main role of PPAR-γ in adipogenesis during infection has been revealed using in vivo models of infectious diseases (41, 44). Moreover, the expression level of the critical adipogenic transcription factor PPAR-γ was downregulated after B. abortus infection, thus affecting its role in the maintenance of mature adipocyte phenotype (56, 57). C/EBPα is expressed in the late phase of adipocyte differentiation. In contrast, C/EBPβ is expressed very early during adipogenesis and is also required to sustain the expression of PPAR-γ and C/EBPα. After the treatment of preadipocytes with inducers of differentiation, a rapid and transient increase in transcription and expression of C/EBPβ occurs and then decrees during the differentiation process (14). As we expected, B. abortus infection also inhibited C/EBPα transcription (58). On the contrary, B. abortus infection increases the transcription of C/EBPβ. These contradictory results could be explained, at least in part, by the fact that the transcriptional activity of C/EBPβ is regulated by several posttranscriptional modifications (59), including acetylation (60, 61), phosphorylation, and polyubiquitination (62). Additionally, it could be also speculated that overexpression of C/EBPβ may be a compensatory mechanism in response to C/EBPα reduction as was described for brown adipocyte differentiation (63). Further studies are needed to define the mechanism involved during B. abortus infection and the significance of this regulation.
We have observed that B. abortus infection of pre-adipocytes may also alter the extracellular matrix by inducing MMP-2 and MMP-9 secretion. Despite this, finding appears to be opposed to previous studies that reported that these MMPs are involved in extracellular matrix remodeling during adipocyte differentiation (15, 64, 65), two plausible explanations should be considered. First, it is known that other MMPs such as MMP-3 could have the opposite effects and down modulate the adipogenic differentiation (66, 67). Second, the MMPs action is controlled by regulators such as tissue inhibitor of metalloproteinases (TIMPs) as well as members of protease family of ADAMs (68). In vivo, TIMPs counterbalanced the activity of MMPs, and these complexes are dissociated during gelating zymography procedure. However, in general, in inflammatory conditions, TIMPs do not increase in the same degree as MMPs do, thus increasing the MMP/TIMP ratio (4, 69). Accordingly, supernatants from B. abortus-infected pre-adipocytes produced gelatin cleavage when evaluated under native conditions in the fluid phase, with MMP-TIMP complex are not dissociated as occurs during gel electrophoresis. This indicates that other mechanisms are involved during adipogenesis inhibition by B. abortus infection.
Adipogenesis inhibition may be a process involving TLR-ligand interactions (37, 38). Here, we have demonstrated that B. abortus-mediated inhibition of the adipocyte differentiation was independent of the bacterial viability, thus insinuating a potential role elicited by a structural bacterial component. As in our previous studies, the bacteria lipoprotein L-Omp19 plays again a critical role in this sense but not the LPS (20). Such lipid moiety but not its non-lipidated counterpart U-Omp19, was able to mimic adipogenesis inhibition induced by B. abortus infection. The effect was elicited by the lipid moiety, which is likely shared by all bacterial lipoproteins. The genome of B. abortus codifies no less than 80 putative lipoproteins (70). This indicates that lipoproteins present in Brucella could be sufficient to modulate adipocyte physiology.
In adipose tissue, immune cells including macrophages and T cells are the main responsible for inflammatory cytokine production (71, 72). In particular, macrophages participate in adipose tissue dysfunction and reduced adipogenesis (73, 74). Brucella infection is accompanied by the infiltration of inflammatory cells, and the macrophages represent the main replication niche for these bacteria (75). Even though the role of macrophage polarization in the pathogenesis of Brucella species is poorly described until now (76, 77). Here, we have demonstrated that proinflammatory cytokines -such as TNF-α- secreted by macrophages in response to B. abortus infection exert an inhibitory effect on adipogenic differentiation (52). However, we cannot rule out that other cytokines are also present in the conditioned media and contribute to these observations.
Overall, these results suggest a possible scenario in which B. abortus infection via its lipoproteins modulates adipogenesis process and soluble mediators secreted by B. abortus—infected macrophages may contribute to this phenomenon, as a mechanism in which TNF-α is involved.
In conclusion, our results suggest that adipogenesis process could be altered directly after exposure—even without effective multiplication—of the adipocytes and their precursors to Brucella, or their lipoproteins. Furthermore, this same process could be indirectly altered, thanks to soluble mediators such as TNF-α released by macrophages infected by the same bacteria.
These early studies using murine cell lines provide clues regarding potential mechanisms involved during the interaction of B. abortus with adipocytes. Further studies using primary human adipocytes, human adipose tissue explants, and the in vivo murine model will be needed to confirm whether the responses described here have a role in the chronic inflammation and chronicity of the infection.
Data Availability Statement
The raw data supporting the conclusions of this article will be made available by the authors, without undue reservation.
Author Contributions
AP performed the experiments. All authors analyzed the data. MD and JQ wrote the article. MD and JQ designed the experiments, revised the article, and obtained research funding. GG performed a critical read of the manuscript. All authors contributed to the article and approved the submitted version.
Funding
This work was supported by grants from Agencia Nacional of Promoción Científica y Tecnológica (ANPCYT, Argentina), PICT 2014-1111, PICT 2015-0316, PICT 2017-2859 to MD and PICT-2015-1921 to JQ. Funding agencies had no role in study design, data collection and analysis, decision to publish, or preparation of the manuscript.
Conflict of Interest
The authors declare that the research was conducted in the absence of any commercial or financial relationships that could be construed as a potential conflict of interest.
Acknowledgments
We thank Horacio Salomón and the staff of the Instituto de Investigaciones Biomédicas en Retrovirus y Sida (INBIRS), for their assistance with biosafety level 3 laboratory uses.
References
1. Franc KA, Krecek RC, Hasler BN, Arenas-Gamboa AM. Brucellosis remains a neglected disease in the developing world: a call for interdisciplinary action. BMC Public Health (2018) 18(1):125. doi: 10.1186/s12889-017-5016-y
2. Pappas G, Akritidis N, Bosilkovski M, Tsianos E. Brucellosis. N Engl J Med (2005) 352(22):2325–36. doi: 10.1056/NEJMra050570
3. Wu BN, O’Sullivan AJ. Sex differences in energy metabolism need to be considered with lifestyle modifications in humans. J Nutr Metab (2011) 2011:391809. doi: 10.1155/2011/391809
4. Kershaw EE, Flier JS. Adipose tissue as an endocrine organ. J Clin Endocrinol Metab (2004) 89(6):2548–56. doi: 10.1210/jc.2004-0395
5. Ouchi N, Parker JL, Lugus JJ, Walsh K. Adipokines in inflammation and metabolic disease. Nat Rev Immunol (2011) 11(2):85–97. doi: 10.1038/nri2921
6. Ferrante AW Jr. The immune cells in adipose tissue. Diabetes Obes Metab (2013) 15 (Suppl 3):34–8. doi: 10.1111/dom.12154
7. Birsoy K, Festuccia WT, Laplante M. A comparative perspective on lipid storage in animals. J Cell Sci (2013) 126(Pt 7):1541–52. doi: 10.1242/jcs.104992
8. Bouwman JJ, Visseren FL, Bouter KP, Diepersloot RJ. Infection-induced inflammatory response of adipocytes in vitro. Int J Obes (Lond) (2008) 32(6):892–901. doi: 10.1038/ijo.2008.36
9. Damouche A, Lazure T, Avettand-Fenoel V, Huot N, Dejucq-Rainsford N, Satie AP, et al. Adipose Tissue Is a Neglected Viral Reservoir and an Inflammatory Site during Chronic HIV and SIV Infection. PLoS Pathog (2015) 11(9):e1005153. doi: 10.1371/journal.ppat.1005153
10. Maurin T, Saillan-Barreau C, Cousin B, Casteilla L, Doglio A, Penicaud L. Tumor necrosis factor-alpha stimulates HIV-1 production in primary culture of human adipocytes. Exp Cell Res (2005) 304(2):544–51. doi: 10.1016/j.yexcr.2004.12.003
11. Kim JS, Ryu MJ, Byun EH, Kim WS, Whang J, Min KN, et al. Differential immune response of adipocytes to virulent and attenuated Mycobacterium tuberculosis. Microbes Infect (2011) 13(14-15):1242–51. doi: 10.1016/j.micinf.2011.07.002
12. Poester FP, Samartino LE, Santos RL. Pathogenesis and pathobiology of brucellosis in livestock. Rev Sci Tech (2013) 32(1):105–15. doi: 10.20506/rst.32.1.2193
13. de Souza TD, de Carvalho TF, Mol J, Lopes JVM, Silva MF, da Paixao TA, et al. Tissue distribution and cell tropism of Brucella canis in naturally infected canine foetuses and neonates. Sci Rep (2018) 8(1):7203. doi: 10.1038/s41598-018-25651-x
14. Darlington GJ, Ross SE, MacDougald OA. The role of C/EBP genes in adipocyte differentiation. J Biol Chem (1998) 273(46):30057–60. doi: 10.1074/jbc.273.46.30057
15. Bouloumie A, Sengenes C, Portolan G, Galitzky J, Lafontan M. Adipocyte produces matrix metalloproteinases 2 and 9: involvement in adipose differentiation. Diabetes (2001) 50(9):2080–6. doi: 10.2337/diabetes.50.9.2080
16. Kuri-Harcuch W, Velez-delValle C, Vazquez-Sandoval A, Hernandez-Mosqueira C, Fernandez-Sanchez V. A cellular perspective of adipogenesis transcriptional regulation. J Cell Physiol (2019) 234(2):1111–29. doi: 10.1002/jcp.27060
17. Smith JA, Khan M, Magnani DD, Harms JS, Durward M, Radhakrishnan GK, et al. Brucella induces an unfolded protein response via TcpB that supports intracellular replication in macrophages. PLoS Pathog (2013) 9(12):e1003785. doi: 10.1371/journal.ppat.1003785
18. Sha H, He Y, Chen H, Wang C, Zenno A, Shi H, et al. The IRE1alpha-XBP1 pathway of the unfolded protein response is required for adipogenesis. Cell Metab (2009) 9(6):556–64. doi: 10.1016/j.cmet.2009.04.009
19. Scian R, Barrionuevo P, Fossati CA, Giambartolomei GH, Delpino MV. Brucella abortus invasion of osteoblasts inhibits bone formation. Infect Immun (2012) 80(7):2333–45. doi: 10.1128/IAI.00208-12
20. Giambartolomei GH, Zwerdling A, Cassataro J, Bruno L, Fossati CA, Philipp MT. Lipoproteins, not lipopolysaccharide, are the key mediators of the proinflammatory response elicited by heat-killed Brucella abortus. J Immunol (2004) 173(7):4635–42. doi: 10.4049/jimmunol.173.7.4635
21. Scian R, Barrionuevo P, Giambartolomei GH, Fossati CA, Baldi PC, Delpino MV. Granulocyte-macrophage colony-stimulating factor- and tumor necrosis factor alpha-mediated matrix metalloproteinase production by human osteoblasts and monocytes after infection with Brucella abortus. Infect Immun (2011) 79(1):192–202. doi: 10.1128/IAI.00934-10
22. Hibbs MS, Hasty KA, Seyer JM, Kang AH, Mainardi CL. Biochemical and immunological characterization of the secreted forms of human neutrophil gelatinase. J Biol Chem (1985) 260(4):2493–500.
23. Giralt M, Cereijo R, Villarroya F. Adipokines and the Endocrine Role of Adipose Tissues. Handb Exp Pharmacol (2016) 233:265–82. doi: 10.1007/164_2015_6
24. Schram K, Sweeney G. Implications of myocardial matrix remodeling by adipokines in obesity-related heart failure. Trends Cardiovasc Med (2008) 18(6):199–205. doi: 10.1016/j.tcm.2008.10.001
25. Park HY, Kwon HM, Lim HJ, Hong BK, Lee JY, Park BE, et al. Potential role of leptin in angiogenesis: leptin induces endothelial cell proliferation and expression of matrix metalloproteinases in vivo and in vitro. Exp Mol Med (2001) 33(2):95–102. doi: 10.1038/emm.2001.17
26. Moon HS, Lee HG, Seo JH, Chung CS, Guo DD, Kim TG, et al. Leptin-induced matrix metalloproteinase-2 secretion is suppressed by trans-10,cis-12 conjugated linoleic acid. Biochem Biophys Res Commun (2007) 356(4):955–60. doi: 10.1016/j.bbrc.2007.03.068
27. Oku T, Shimada K, Kenmotsu H, Ando Y, Kurisaka C, Sano R, et al. Stimulation of Peritoneal Mesothelial Cells to Secrete Matrix Metalloproteinase-9 (MMP-9) by TNF-alpha: A Role in the Invasion of Gastric Carcinoma Cells. Int J Mol Sci (2018) 19(12):1–14. doi: 10.3390/ijms19123961
28. Harris JE, Fernandez-Vilaseca M, Elkington PT, Horncastle DE, Graeber MB, Friedland JS. IFNgamma synergizes with IL-1beta to up-regulate MMP-9 secretion in a cellular model of central nervous system tuberculosis. FASEB J (2007) 21(2):356–65. doi: 10.1096/fj.06-6925com
29. Kohno Y, Tanimoto A, Cirathaworn C, Shimajiri S, Tawara A, Sasaguri Y. GM-CSF activates RhoA, integrin and MMP expression in human monocytic cells. Pathol Int (2004) 54(9):693–702. doi: 10.1111/j.1440-1827.2004.01682.x
30. Krubasik D, Eisenach PA, Kunz-Schughart LA, Murphy G, English WR. Granulocyte-macrophage colony stimulating factor induces endothelial capillary formation through induction of membrane-type 1 matrix metalloproteinase expression in vitro. Int J Cancer (2008) 122(6):1261–72. doi: 10.1002/ijc.23234
31. Brinckerhoff CE, Matrisian LM. Matrix metalloproteinases: a tail of a frog that became a prince. Nat Rev Mol Cell Biol (2002) 3(3):207–14. doi: 10.1038/nrm763
32. Delpino MV, Barrionuevo P, Macedo GC, Oliveira SC, Genaro SD, Scian R, et al. Macrophage-elicited osteoclastogenesis in response to Brucella abortus infection requires TLR2/MyD88-dependent TNF-alpha production. J Leukoc Biol (2012) 91(2):285–98. doi: 10.1189/jlb.04111185
33. Garcia Samartino C, Delpino MV, Pott Godoy C, Di Genaro MS, Pasquevich KA, Zwerdling A, et al. Brucella abortus induces the secretion of proinflammatory mediators from glial cells leading to astrocyte apoptosis. Am J Pathol (2010) 176(3):1323–38. doi: 10.2353/ajpath.2010.090503
34. Scian R, Barrionuevo P, Giambartolomei GH, De Simone EA, Vanzulli SI, Fossati CA, et al. Potential role of fibroblast-like synoviocytes in joint damage induced by Brucella abortus infection through production and induction of matrix metalloproteinases. Infect Immun (2011) 79(9):3619–32. doi: 10.1128/IAI.05408-11
35. Zwerdling A, Delpino MV, Barrionuevo P, Cassataro J, Pasquevich KA, Garcia Samartino C, et al. Brucella lipoproteins mimic dendritic cell maturation induced by Brucella abortus. Microbes Infect (2008) 10(12-13):1346–54. doi: 10.1016/j.micinf.2008.07.035
36. Zwerdling A, Delpino MV, Pasquevich KA, Barrionuevo P, Cassataro J, Garcia Samartino C, et al. Brucella abortus activates human neutrophils. Microbes Infect (2009) 11(6-7):689–97. doi: 10.1016/j.micinf.2009.04.010
37. Aamir K, Khan HU, Sethi G, Hossain MA, Arya A. Wnt signaling mediates TLR pathway and promote unrestrained adipogenesis and metaflammation: Therapeutic targets for obesity and type 2 diabetes. Pharmacol Res (2020) 152:104602. doi: 10.1016/j.phrs.2019.104602
38. Ajuwon KM, Banz W, Winters TA. Stimulation with Peptidoglycan induces interleukin 6 and TLR2 expression and a concomitant downregulation of expression of adiponectin receptors 1 and 2 in 3T3-L1 adipocytes. J Inflamm (Lond) (2009) 6:8. doi: 10.1186/1476-9255-6-8
40. Cawthorn WP, Heyd F, Hegyi K, Sethi JK. Tumour necrosis factor-alpha inhibits adipogenesis via a beta-catenin/TCF4(TCF7L2)-dependent pathway. Cell Death Differ (2007) 14(7):1361–73. doi: 10.1038/sj.cdd.4402127
41. Ayyappan JP, Ganapathi U, Lizardo K, Vinnard C, Subbian S, Perlin DS, et al. Adipose Tissue Regulates Pulmonary Pathology during TB Infection. mBio (2019) 10(2):1–16. doi: 10.1128/mBio.02771-18
42. Nagajyothi JF, Weiss LM. Advances in understanding the role of adipose tissue and mitochondrial oxidative stress in Trypanosoma cruzi infection. F1000Res (2019) 8:1–7. doi: 10.12688/f1000research.19190.1
43. Lizardo K, Ayyappan JP, Cui MH, Balasubramanya R, Jelicks LA, Nagajyothi JF. High fat diet aggravates cardiomyopathy in murine chronic Chagas disease. Microbes Infect (2019) 21(1):63–71. doi: 10.1016/j.micinf.2018.07.001
44. Gonzalez FB, Villar SR, Toneatto J, Pacini MF, Marquez J, D’Attilio L, et al. Immune response triggered by Trypanosoma cruzi infection strikes adipose tissue homeostasis altering lipid storage, enzyme profile and adipokine expression. Med Microbiol Immunol (2019) 208(5):651–66. doi: 10.1007/s00430-018-0572-z
45. Wanjalla CN, McDonnell WJ, Koethe JR. Adipose Tissue T Cells in HIV/SIV Infection. Front Immunol (2018) 9:2730. doi: 10.3389/fimmu.2018.02730
46. Neyrolles O, Hernandez-Pando R, Pietri-Rouxel F, Fornes P, Tailleux L, Barrios Payan JA, et al. Is adipose tissue a place for Mycobacterium tuberculosis persistence? PLoS One (2006) 1:e43. doi: 10.1371/journal.pone.0000043
47. Combs TP, Nagajyothi, Mukherjee S, de Almeida CJ, Jelicks LA, Schubert W, et al. The adipocyte as an important target cell for Trypanosoma cruzi infection. J Biol Chem (2005) 280(25):24085–94. doi: 10.1074/jbc.M412802200
48. Esteve Rafols M. Adipose tissue: cell heterogeneity and functional diversity. Endocrinol Nutr (2014) 61(2):100–12. doi: 10.1016/j.endonu.2013.03.011
49. Arriola Benitez PC, Scian R, Comerci DJ, Serantes DR, Vanzulli S, Fossati CA, et al. Brucella abortus induces collagen deposition and MMP-9 down-modulation in hepatic stellate cells via TGF-beta1 production. Am J Pathol (2013) 183(6):1918–27. doi: 10.1016/j.ajpath.2013.08.006
50. Ikeda Y, Tsuchiya H, Hama S, Kajimoto K, Kogure K. Resistin affects lipid metabolism during adipocyte maturation of 3T3-L1 cells. FEBS J (2013) 280(22):5884–95. doi: 10.1111/febs.12514
51. Fu Y, Luo N, Klein RL, Garvey WT. Adiponectin promotes adipocyte differentiation, insulin sensitivity, and lipid accumulation. J Lipid Res (2005) 46(7):1369–79. doi: 10.1194/jlr.M400373-JLR200
52. Jiang N, Li Y, Shu T, Wang J. Cytokines and inflammation in adipogenesis: an updated review. Front Med (2019) 13(3):314–29. doi: 10.1007/s11684-018-0625-0
53. Fasshauer M, Kralisch S, Klier M, Lossner U, Bluher M, Klein J, et al. Adiponectin gene expression and secretion is inhibited by interleukin-6 in 3T3-L1 adipocytes. Biochem Biophys Res Commun (2003) 301(4):1045–50. doi: 10.1016/s0006-291x(03)00090-1
54. Wueest S, Konrad D. The role of adipocyte-specific IL-6-type cytokine signaling in FFA and leptin release. Adipocyte (2018) 7(3):226–8. doi: 10.1080/21623945.2018.1493901
55. Trujillo ME, Sullivan S, Harten I, Schneider SH, Greenberg AS, Fried SK. Interleukin-6 regulates human adipose tissue lipid metabolism and leptin production in vitro. J Clin Endocrinol Metab (2004) 89(11):5577–82. doi: 10.1210/jc.2004-0603
56. Nielsen R, Pedersen TA, Hagenbeek D, Moulos P, Siersbaek R, Megens E, et al. Genome-wide profiling of PPARgamma:RXR and RNA polymerase II occupancy reveals temporal activation of distinct metabolic pathways and changes in RXR dimer composition during adipogenesis. Genes Dev (2008) 22(21):2953–67. doi: 10.1101/gad.501108
57. Lefterova MI, Zhang Y, Steger DJ, Schupp M, Schug J, Cristancho A, et al. PPARgamma and C/EBP factors orchestrate adipocyte biology via adjacent binding on a genome-wide scale. Genes Dev (2008) 22(21):2941–52. doi: 10.1101/gad.1709008
58. Lechner S, Mitterberger MC, Mattesich M, Zwerschke W. Role of C/EBPbeta-LAP and C/EBPbeta-LIP in early adipogenic differentiation of human white adipose-derived progenitors and at later stages in immature adipocytes. Differentiation (2013) 85(1-2):20–31. doi: 10.1016/j.diff.2012.11.001
59. Nerlov C. C/EBPs: recipients of extracellular signals through proteome modulation. Curr Opin Cell Biol (2008) 20(2):180–5. doi: 10.1016/j.ceb.2008.02.002
60. Cesena TI, Cardinaux JR, Kwok R, Schwartz J. CCAAT/enhancer-binding protein (C/EBP) beta is acetylated at multiple lysines: acetylation of C/EBPbeta at lysine 39 modulates its ability to activate transcription. J Biol Chem (2007) 282(2):956–67. doi: 10.1074/jbc.M511451200
61. Wiper-Bergeron N, Salem HA, Tomlinson JJ, Wu D, Hache RJ. Glucocorticoid-stimulated preadipocyte differentiation is mediated through acetylation of C/EBPbeta by GCN5. Proc Natl Acad Sci U S A (2007) 104(8):2703–8. doi: 10.1073/pnas.0607378104
62. Hattori T, Ohoka N, Inoue Y, Hayashi H, Onozaki K. C/EBP family transcription factors are degraded by the proteasome but stabilized by forming dimer. Oncogene (2003) 22(9):1273–80. doi: 10.1038/sj.onc.1206204
63. Linhart HG, Ishimura-Oka K, DeMayo F, Kibe T, Repka D, Poindexter B, et al. C/EBPalpha is required for differentiation of white, but not brown, adipose tissue. Proc Natl Acad Sci U S A (2001) 98(22):12532–7. doi: 10.1073/pnas.211416898
64. Chavey C, Mari B, Monthouel MN, Bonnafous S, Anglard P, Van Obberghen E, et al. Matrix metalloproteinases are differentially expressed in adipose tissue during obesity and modulate adipocyte differentiation. J Biol Chem (2003) 278(14):11888–96. doi: 10.1074/jbc.M209196200
65. Lilla J, Stickens D, Werb Z. Metalloproteases and adipogenesis: a weighty subject. Am J Pathol (2002) 160(5):1551–4. doi: 10.1016/S0002-9440(10)61100-5
66. Woessner JF Jr. MMPs and TIMPs. An historical perspective. Methods Mol Biol (2001) 151:1–23. doi: 10.1385/MB:22:1:033
67. Alexander CM, Selvarajan S, Mudgett J, Werb Z. Stromelysin-1 regulates adipogenesis during mammary gland involution. J Cell Biol (2001) 152(4):693–703. doi: 10.1083/jcb.152.4.693
68. Brew K, Nagase H. The tissue inhibitors of metalloproteinases (TIMPs): an ancient family with structural and functional diversity. Biochim Biophys Acta (2010) 1803(1):55–71. doi: 10.1016/j.bbamcr.2010.01.003
69. Malemud CJ, Islam N, Haqqi TM. Pathophysiological mechanisms in osteoarthritis lead to novel therapeutic strategies. Cells Tissues Organs (2003) 174(1-2):34–48. doi: 10.1159/000070573
70. Chain PS, Comerci DJ, Tolmasky ME, Larimer FW, Malfatti SA, Vergez LM, et al. Whole-genome analyses of speciation events in pathogenic Brucellae. Infect Immun (2005) 73(12):8353–61. doi: 10.1128/IAI.73.12.8353-8361.2005
71. Weisberg SP, McCann D, Desai M, Rosenbaum M, Leibel RL, Ferrante AW Jr. Obesity is associated with macrophage accumulation in adipose tissue. J Clin Invest (2003) 112(12):1796–808. doi: 10.1172/JCI19246
72. Wu H, Ghosh S, Perrard XD, Feng L, Garcia GE, Perrard JL, et al. T-cell accumulation and regulated on activation, normal T cell expressed and secreted upregulation in adipose tissue in obesity. Circulation (2007) 115(8):1029–38. doi: 10.1161/CIRCULATIONAHA.106.638379
73. Constant VA, Gagnon A, Landry A, Sorisky A. Macrophage-conditioned medium inhibits the differentiation of 3T3-L1 and human abdominal preadipocytes. Diabetologia (2006) 49(6):1402–11. doi: 10.1007/s00125-006-0253-0
74. Lacasa D, Taleb S, Keophiphath M, Miranville A, Clement K. Macrophage-secreted factors impair human adipogenesis: involvement of proinflammatory state in preadipocytes. Endocrinology (2007) 148(2):868–77. doi: 10.1210/en.2006-0687
75. Atluri VL, Xavier MN, de Jong MF, den Hartigh AB, Tsolis RM. Interactions of the human pathogenic Brucella species with their hosts. Annu Rev Microbiol (2011) 65:523–41. doi: 10.1146/annurev-micro-090110-102905
76. Wang Y, Li Y, Li H, Song H, Zhai N, Lou L, et al. Brucella Dysregulates Monocytes and Inhibits Macrophage Polarization through LC3-Dependent Autophagy. Front Immunol (2017) 8:691. doi: 10.3389/fimmu.2017.00691
Keywords: Brucella, TNF-α, adipogenesis, macrophage, inflammation
Citation: Pesce Viglietti AI, Giambartolomei GH, Quarleri J and Delpino MV (2020) Brucella abortus Infection Modulates 3T3-L1 Adipocyte Inflammatory Response and Inhibits Adipogenesis. Front. Endocrinol. 11:585923. doi: 10.3389/fendo.2020.585923
Received: 22 July 2020; Accepted: 08 September 2020;
Published: 18 September 2020.
Edited by:
Vinicius Frias Carvalho, Oswaldo Cruz Foundation (Fiocruz), BrazilReviewed by:
Rubén Cereijo, University of Barcelona, SpainTobias Fromme, Technical University of Munich, Germany
P Trayhurn, University of Liverpool, United Kingdom
Copyright © 2020 Pesce Viglietti, Giambartolomei, Quarleri and Delpino. This is an open-access article distributed under the terms of the Creative Commons Attribution License (CC BY). The use, distribution or reproduction in other forums is permitted, provided the original author(s) and the copyright owner(s) are credited and that the original publication in this journal is cited, in accordance with accepted academic practice. No use, distribution or reproduction is permitted which does not comply with these terms.
*Correspondence: María Victoria Delpino, bWRlbHBpbm9AZmZ5Yi51YmEuYXI=; Jorge Quarleri, cXVhcmxlcmlAZm1lZC51YmEuYXI=
†ORCID: María Victoria Delpino, orcid.org/0000-0003-2077-8509; Jorge Quarleri, orcid.org/0000-0001-5110-8773