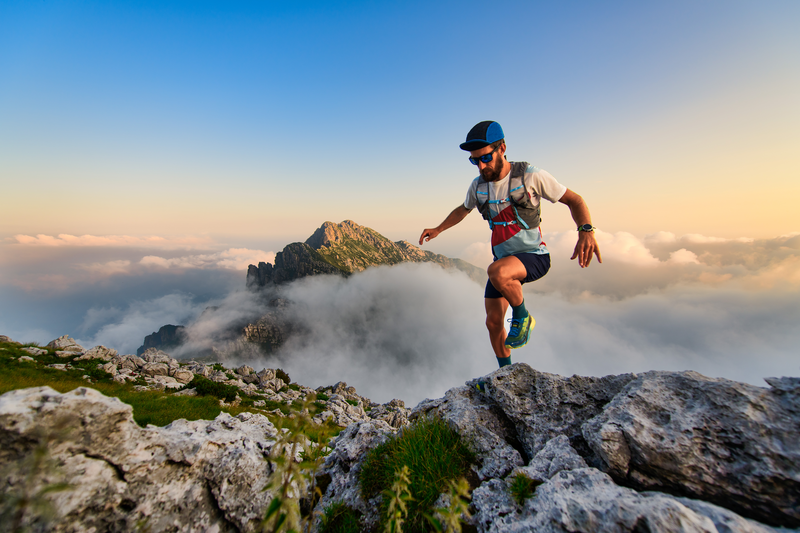
94% of researchers rate our articles as excellent or good
Learn more about the work of our research integrity team to safeguard the quality of each article we publish.
Find out more
ORIGINAL RESEARCH article
Front. Endocrinol. , 29 September 2020
Sec. Obesity
Volume 11 - 2020 | https://doi.org/10.3389/fendo.2020.565065
Thyroid hormones control both metabolic pathways and body composition, whereas little knowledge is available about the possible influence of skeletal muscle mass (MM) on thyroid hormone metabolism and circulating levels. This was a cross-sectional study conducted at the Population Health Unit of the National Institute of Gastroenterology IRCCS “S. de Bellis” (Italy) and investigating the extent to which skeletal MM affects thyroid function in obesity. Two hundred twenty-seven consecutive healthy volunteers (155 women and 72 men) with overweight and obesity (BMI ≥ 25 kg/m2) and taking no medication or supplement were assessed for hormone, metabolic and routine laboratory parameters. Body composition parameters were collected by using bioelectrical impedance analysis (BIA). MM was directly related to the body mass index (BMI), waist circumference (WC), insulin, triglycerides, uric acid and free-triiodothyronine (FT3) serum levels, FT3 to the free-thyroxine (FT4) ratio, and insulin-resistance (HOMA-IR), and inversely related to age, total, and HDL-cholesterol serum levels. Multiple regression models confirmed the relationship between MM and the FT3 to FT4 ratio, independently of age, BMI, TSH, triglycerides, and insulin serum levels. The same analyses run by gender showed that this relationship maintained significance only in men. Increased skeletal MM in obesity results in improved thyroid activity mediated by increased T4 conversion to T3, and higher FT3 circulating levels, particularly in men. In conclusion, preserving a greater skeletal MM in obesity helps to enhance thyroid activity.
Clinical Trial Registration: ClinicalTrials.gov, identifier NCT04327375.
In recent decades, obesity rates have more than doubled, to the extent that nearly one third of the world population is now classified as overweight or obese (1). Despite its debatable sensitivity, the body mass index (BMI) is still the parameter most commonly used to define obesity in clinical practice. According to the latest data from the Global Burden of Disease Study (GBD), a high BMI showed the greatest relative increase in exposure since 1990, and is thus actually ranked among the top five risk factors in terms of attributable deaths and disability-adjusted life-years (DALYs) (2). Obesity is a multifactorial condition characterized by an excessive accumulation of body fat resulting from the interaction between environmental and genetic factors (3, 4). Among lifestyle contributory factors, dietary habits play a leading role in weight gain in terms of energy balance. Indeed, a chronic positive energy balance resulting from an excessive dietary energy intake versus energy expenditure leads to a form of metabolic failure deriving from the inability of oxidative processes to cope with the surplus calories intake (5).
Thyroid hormones play an important role in regulating many metabolic pathways, since they are involved in accelerating thermogenesis and oxygen consumption, as well as controlling lipid and glucose metabolism. On this basis, hypothyroidism is usually associated with a decreased thermogenesis and metabolic rate, inducing a modest weight gain, whereas hyperthyroidism leads to weight loss despite an increased appetite and elevated metabolic rate (6).
Obesity is responsible for a moderate increase in serum thyrotropin (TSH) concentrations, due to insulin resistance and elevated leptin levels (7, 8), but also for slightly above-normal free plasma levels of triiodothyronine (T3). This latter is widely acknowledged as a compensatory mechanism reducing further weight gain, since higher thyroid hormone concentrations physiologically increase resting energy expenditure (REE) (9, 10).
To date, the large volume of research investigating body modifications in obesity is consistent with the conviction that gaining weight elicits several adaptations in all body compartments, especially fat storage (11). Besides the increase in fat mass, obesity also induces a simultaneous growth of fat-free and muscle mass (MM) (12). In particular, an increased lower limb skeletal MM has been described as a way to compensate for the increased fat mass, in order to support standing and ambulation (13). Thus, since the skeletal muscle is a major site for thermogenesis, glucose utilization and lipid oxidation, the possibility of a close interplay between thyroid gland function, and MM is quite viable.
Moreover, it should be remembered that human skeletal muscle is the site of type II iodothyronine deiodinase activity, converting T4 to T3 (14, 15). Given that obesity is characterized by an increase of both MM and FT3 levels, it may well be that a higher MM is responsible for higher type II iodothyronine deiodinase, thus increasing free T3 production and circulating hormone levels.
To the best of our knowledge, studies clarifying thyroid performance in response to body MM changes in obesity are lacking. The purpose of this study was to examine the relationship between skeletal MM and thyroid hormone levels among subjects with overweight and obesity, focusing on FT4 conversion to FT3, as well as on changes in FT3 serum levels.
From January 2018 to December 2019, 227 consecutive patients (155 women and 72 men, aged 42.4 ± 13.3 years) were recruited at the Population Health Unit of the National Institute of Gastroenterology “S. de Bellis”, Research Hospital, Castellana Grotte, Italy. In the power analysis, to detect a variance of the FT3/FT4 ratio parameter between the reference population (10) compared to our population used in the general linear model, a sample size at least of 19 was required, with 90% power for a two-sided alpha error probability of 5%.
All data collection was done at the baseline examination. Inclusion criteria were overweight or obesity (BMI ≥ 25 kg/m2), and taking no supplements or medication, including oral contraceptives or medicines for osteoporosis. Exclusion criteria were any history of endocrinological diseases (diabetes mellitus, hypo or hyperthyroidism, hypopituitarism, etc.), chronic inflammatory diseases, stable hypertension, angina pectoris, stroke, transient ischemic attack, heart infarction, atrial fibrillation, congenital heart disease, any malignancies, renal and liver failure and inherited thrombocytopenia.
The study protocol (ClinicalTrials.gov Identifier: NCT04327375) met the principles of the Declaration of Helsinki and was approved by the Ethics Committee of the National Institute of Gastroenterology “S. De Bellis” Research Hospital (Castellana Grotte, Italy). All participants gave informed consent prior to enrollment in accordance with the Helsinki Declaration of 1964 and subsequent revisions.
At baseline, subjects were closely examined for hormone, metabolic and routine biochemistry parameters. A brief interview, including questions on medical history and lifestyle, was conducted by a physician. Extemporaneous outpatients diastolic (DBP) and systolic blood pressure (SBP) was determined in a sitting position after at least a 10-min rest, a minimum of three different times, using an OMRON M6 automatic Blood Pressure monitor. Blood samples were drawn between 08:00 h and 09:00 h after overnight fasting. Fasting blood glucose, insulin, total cholesterol, high- and low-density lipoprotein (HDL, LDL) cholesterol, and triglycerides serum levels were assayed. Serum insulin concentrations were measured by radioimmunoassay (Behring, Scoppito, Italy) and all samples were analyzed in duplicate. Plasma glucose was determined using the glucose oxidase method (Sclavus, Siena, Italy), while the concentrations of plasma lipids (triglycerides, total cholesterol, HDL cholesterol) were quantified by automated colorimetric method (Hitachi; Boehringer Mannheim, Mannheim, Germany). LDL cholesterol was calculated using the Friedewald equation.
TSH, FT3, and FT4 serum concentrations were measured by a competitive luminometric assay based on the SPALT (solid-phase antigen luminescence technique) principle (LIAISON FT3, FT4, TSH, DiaSorin, Saluggia, Italy). Serum uric acid was measured by the URICASE/POD method implemented in an autoanalyzer (Boehringer Mannheim, Mannheim, Germany). Insulin resistance was assessed using the Homeostasis Model Assessment for Insulin Resistance (HOMA-IR) (16).
The metabolic syndrome was assessed according to IDF criteria (17), consisting of abdominal obesity (WC ≥ 94 cm for men and ≥ 80 cm for women) together with at least 2 of the following parameters: hypertension (SBP ≥ 130 mm Hg and/or DBP ≥ 85 mm Hg) or a history of antihypertensive drugs, hypertriglyceridemia (≥ 150 mg/dl) or treatment for this disorder, low HDL-C (< 40 mg/dl in men and < 50 mg/dl in women) or treatment for this disorder, and high fasting plasma glucose ≥ 100 mg/dl) or a diagnosis of T2DM.
Clinical procedures were carried out by two certified nutritionists (R.Z. and F.C.) both previously trained to equivalent measuring performances. All anthropometric measurements were taken with participants wearing lightweight clothing and no shoes. Variables were all collected at the same time between 7:00 and 10:00 a.m., following an overnight fast.
Height was measured to the nearest 0.5 cm using a wall-mounted stadiometer (Seca 711; Seca, Hamburg, Germany). Body weight was determined to the nearest 0.1 kg using a calibrated balance beam scale (Seca 711; Seca, Hamburg, Germany). BMI was calculated by dividing body weight (kg) by the square of height (m2) and classified according to World Health Organization criteria for normal weight (18.5–24.9 kg/m2), overweight (25.0–29.9 kg/m2), grade I obesity (30.0–34.9 kg/m2), grade II obesity (35.0–39.9 kg/m2), and grade III obesity (≥40.0 kg/m2) (18). Waist circumference (WC) was measured at the narrowest part of the abdomen, or in the area between the tenth rib and the iliac crest (minimum circumference).
Bioelectrical Impedance Analysis (BIA) was performed using a single-frequency bioimpedance analyzer (BIA‐101 analyzer, 50‐kHz frequency; Akern Bioresearch, Florence, Italy). The instrument is routinely checked with resistors and capacitors of known values. In accordance with the European Society of Parenteral and Enteral Nutrition (ESPEN) guidelines (19), all participants were examined supine with their legs spread slightly apart, and had refrained from eating, drinking, and exercising for six hours and drunk no alcohol within 24 h before examination. Shoes and socks were removed and contact areas scrubbed with alcohol prior to electrode placement. Electrodes (BIATRODES Akern, Florence, Italy) were placed proximal to the phalangeal-metacarpal joint on the dorsal surface of the right hand and distal to the transverse arch on the superior surface of the right foot. Sensor electrodes were placed at the midpoint between the distal prominence of the radius and ulna of the right wrist, and between the medial and lateral malleoli of the right ankle (20). All measurements were performed by a senior nutritionist (SP) under strictly standardized conditions. Whole‐body impedance vector components, resistance (R, Ω) and reactance (Xc, Ω), were derived and recorded when stable.
Then, according to age, gender, weight and height of each subject, body composition parameters were obtained using the software provided by the manufacturer, which included validated (19) predictive equations for total body water (TBW, L), extracellular water (ECW, L), intracellular water (ICW, L), fat-free mass (FFM, kg), MM (kg), Appendicular skeletal muscle mass (ASMM, kg), and body cell mass (BCM, kg). Phase angle (PhA, °) values, derived from the ratio of reactance versus electric resistance, were collected as well. A more detailed description of all principles applied to derive bioimpedance measurements has been previously made elsewhere (21, 22).
We performed statistical analysis of baseline variables as the mean ± standard deviation (SD) for continuous variables and proportion (%) for the frequency of categorical variables. The normality of distribution was assessed for each variable using Shapiro’s test. Wilcoxon sum rank test and independent samples t-test were performed on the basis of methodological suitability, to assess differences of collected variables between groups. A Spearman’s correlation matrix was applied to all continuous biochemical and anthropometrics variables in order to assess the presence of significant correlations. P-values less than or equal to 0.05 were considered statistically significant, with 95% confidence intervals. Three different linear regression models were performed on the FT3/FT4 ratio as dependent variable and MM as regressor, as follows: 1) raw model; 2) partially adjusted model including age, BMI, TSH, insulin, and triglycerides levels as confounders; and 3) fully adjusted model adding gender to the previous parameters. Fully adjusted model performed by applying the previous parameters separately in women and men. The selection of confounders was made considering both the univariate significance with the FT3/FT4 ratio and major covariates such as age, BMI, and gender. The principle for structuring each model was derived from the assumption of a key role of gender in affecting body composition, as well as of an unbalanced distribution of the sample. Statistical analyses were performed using RStudio software, Version 1.2.5001 (RStudio, Inc., Boston, MA, USA).
There was a greater proportion of women in the whole sample (68.3%). Median age for both genders was around 42 years (range 18–72 years). The mean BMI was 33 ± 5.38 kg/m2. Metabolic syndrome showed a higher prevalence in men (40.3%), although a minority of the whole sample was affected (N = 60 of 227 subjects). Table 1 summarizes the general, anthropometric, hormone, metabolic, and routine biochemistry parameters of the enrolled subjects expressed as mean ± SD and subdivided by gender. Men showed significantly higher cell and lean mass parameters (PhA, BCM, FFM, MM, ASMM), body fluids (ECW, ICW, TBW), WC, systolic and diastolic BP, biomarkers of glucose metabolism (FBG, insulin, HOMA-IR), triglycerides, uric acid, FT3 serum levels and FT3 to FT4 ratio (all showing a P value less than 0.02). TSH and HDL-cholesterol serum levels were significantly higher in women (P = 0.043 and P < 0.01, respectively).
Spearman’s correlation analysis (Table 2) showed univariate associations between skeletal MM, FT3, FT3 to FT4 ratio, and all investigated variables. MM parameters, including FFM, MM, BCM, and ASMM, resulted directly associated with FT3 levels and the FT3 to FT4 ratio. Moreover, MM was found to be inversely associated with age (P = 0.003, R = −0.196), total (P = 0.035, R = −0.14) and HDL-cholesterol (P < 0.01, R = −0.422) serum levels. By contrast, MM showed a direct relationship with BMI (P = 0.01, R = 0.171), WC (P < 0.01, R = 0.363), insulin-resistance (HOMA-IR) (P = 0.001, R = 0.221), triglycerides (P < 0.01, R = 0.238), uric acid (P < 0.01, R = 0.424), insulin (P = 0.001, R = 0.21), FT3 (P = 0.006, R = 0.18) serum levels, and FT3 to FT4 ratio (P = 0.023, R = 0.151).
Table 2 Spearman’s correlation rank matrix between MM, FT3, and FT3 to FT4 ratio and other continuous collected variables.
Three sets of linear multiple regression models were built on the FT3 to FT4 ratio in order to investigate the association with MM (Table 3). The selection of confounders was made considering both the univariate significance with the FT3 to FT4 ratio and major covariates such as age, gender, and BMI. Raw and semi-adjusted models confirmed the relationship between MM and the FT3 to FT4 ratio independently of age, BMI, TSH, triglycerides, and insulin serum levels (P = 0.040, 95% CI 0.0001 to 0.0024). This association was not maintained when gender was entered into the model, as shown in the fully adjusted model (P = 0.312, 95% CI −0.0008 to 0.0025). However, after dividing the sample by gender, the independent relationship was only maintained in men (P = 0.003, 95% CI 0.0009 to 0.0034) (Table 4).
Table 4 Linear regression models applying the FT3/FT4 ratio as dependent variable, subdivided by gender.
This study, carried out in a population of subjects with overweight and obesity, was conceived to investigate the hypothesis that a higher MM is responsible for higher FT3 production and circulating levels in a preexisting condition of obesity which, per se, triggers increased FT3 concentrations as a possible compensatory mechanism for a thermogenic defect (10). Our findings show, for the first time, that a preserved skeletal MM within a framework of excess weight has a direct influence on thyroid function, in terms of a higher conversion of FT4 to FT3, independently of age, BMI, TSH, triglycerides, and insulin serum levels, but is affected by gender.
As amply described (23, 24), type II iodothyronine deiodinase (DIO2) activity has been shown to be expressed in skeletal muscle cells, thus opening an interesting research perspective on MM involvement in local and systemic T3 production. As the skeletal muscle is a major site for thermogenesis, glucose utilization and lipid oxidation, our findings reflect the possibility of a biological attempt to counteract overfeeding and weight gain by accelerating oxidative processes through the stimulation of thermogenesis. In agreement with this line of thinking, we found a significant correlation between MM and FT3 levels, as well as the FT3 to FT4 ratio, without any significant relationship between MM and FT4 levels. Only a few studies have previously described a similar but opposite mechanism, suggesting that after a period of calorie restriction, our body tends to adopt a thrifty attitude, altering the local muscle metabolism of thyroid hormones and decreasing the availability of T3, thus basically ensuring a kind of quick restoration of fat reserves (25).
Equally conceivable, though needful of further investigations, is the mechanism involving myokines. From this perspective, irisin is widely known to be increased in subjects with obesity (26), and was recently found to be released also by skeletal muscle. In both cases, its major tasks consist of suppressing appetite while increasing energy expenditure so as to slow down weight gain. Noteworthily, leptin was very recently demonstrated to stimulate FT3 via activation of the conversion of FT4 to FT3, and this mechanism supports the hypothesis of a crosstalk between thyroid and skeletal muscle mediated by the muscle-myokine irisin (8, 27). Furthermore, our data suggest that gender has a key role in influencing the association between MM and the conversion of FT4 to FT3, since this relationship appeared to be more pronounced in men, who are well-known to have a higher MM than women. This is a clear effect due to the marked physiological discrepancy in sex hormones secretion and adipose tissue metabolism (28, 29). In other words, while women have higher amounts of body fat storage due to the higher energy demands of their reproductive nature, men exhibit a quantitatively higher amount of fat-free body mass, including bone as well as lean body mass.
In addition, consistently with previous findings (10, 29), this study confirms the FT3 to FT4 ratio as an indicator of an unfavorable metabolic profile, given its association with higher serum triglycerides and insulin levels, as well as insulin resistance (HOMA-IR), all factors with the common effect of strengthening the DIO2 activity. Additional findings of a significantly lower MM in older subjects, as well as a higher BMI and WC in subjects with higher MM, strengthen the internal validity of our results. Equally in line with literature data is the descriptive finding of the prevalence of metabolic syndrome; in fact, this has already been described as predominant in males, in a large sample drawn from the Italian population (30).
Of note, our results are in apparent contrast with those of a previous study (31) showing a negative correlation between the skeletal MM index (SMI) and FT3 levels. However, the authors investigated normal weight Asian subjects, without excluding any use of medications, whereas we examined only Caucasian individuals with a BMI of more than 25 and taking no medications. We also applied a different parameter to quantify MM. Thus, there is insufficient basis for a comparison of these conflicting results.
Some limitations of this study should be considered. Because of the cross-sectional setting, the direction of any causal relationship could not be established, and prospective studies are needed to clarify a causal relationship. Moreover, no evaluation of dietary habits was made and, therefore, we cannot establish any effect of previous diet on anthropometrics and metabolic parameters. This may represent a weak point of our study, since caloric intake may influence directly DIO2 pathway and indirectly thyroid hormones (32). It should also be pointed out that BIA tends to overestimate FFM, however, under stable hydration, as in our case, this method may be useful as an alternative to DXA in a selected clinical setting (33). Furthermore, low correlation coefficients, despite being significant, decreased the biological value of correlations, because they were less than 0.5, a well-recognized cut-off (34) to define a scientifically robust correlation, independently of the p value. Lastly, to complete the picture, further research in this field should take into account pre- and post-menopausal status, since the relative drop in estrogen leads to a decrease in bone mass density and MM/strength (35, 36).
By contrast, a strong point is that we examined only individuals taking no medication, thus avoiding a possible interference with outcomes.
In conclusion, this study shows, for the first time, that a preserved skeletal MM in excess weight conditions helps to stimulate thyroid function, by increasing T4 conversion to T3, and hence T3 availability. The phenomenon is more pronounced in men, due to their greater advantage in terms of MM.
The raw data supporting the conclusions of this article will be made available by the authors, without undue reservation.
The studies involving human participants were reviewed and approved by IRCCS Istituto Tumori Giovanni Paolo II Bari. The patients/participants provided their written informed consent to participate in this study.
Conceptualization: GDP and GG. Methodology: RS. Software: FC and RS. Validation: RS. Formal analysis: FC. Investigation: LLa and SP. Resources: GG. Data curation: FC. Writing—original draft preparation: RZ. Writing—review and editing: GDP, VT, LDL, and VG. Visualization: GDP. Supervision: GDP and RS. Project Administration: GG.
The authors declare that the research was conducted in the absence of any commercial or financial relationships that could be construed as a potential conflict of interest.
1. Tremmel M, Gerdtham U-G, Nilsson PM, Saha S. Economic Burden of Obesity: A Systematic Literature Review. Int J Environ Res Public Health (2017) 14(4):435. doi: 10.3390/ijerph14040435
2. GBD 2017 Risk Factor Collaborators. Global, regional, and national comparative risk assessment of 84 behavioural, environmental and occupational, and metabolic risks or clusters of risks for 195 countries and territories, 1990-2017: a systematic analysis for the Global Burden of Disease Study 2017. Lancet (2018) 392(10159):1923–94.
3. Giskes K, van Lenthe F, Avendano-Pabon M, Brug J. A systematic review of environmental factors and obesogenic dietary intakes among adults: are we getting closer to understanding obesogenic environments? Obes Rev (2011) 12(5):e95–e106.
4. González-Muniesa P, Mártinez-González M-A, Hu FB, Després J-P, Matsuzawa Y, Loos RJF, et al. Obesity. Nat Rev Dis Primers (2017) 3(1):17034. doi: 10.1038/nrdp.2017.34
5. Astrup A. The relevance of increased fat oxidation for body-weight management: metabolic inflexibility in the predisposition to weight gain. Obes Rev (2011) 12(10):859–65.
6. Mullur R, Liu Y-Y, Brent GA. Thyroid hormone regulation of metabolism. Physiol Rev (2014) 94(2):355–82.
7. Bétry C, Challan-Belval MA, Bernard A, Charrié A, Drai J, Laville M, et al. Increased TSH in obesity: Evidence for a BMI-independent association with leptin. Diabetes Metab (2015) 41(3):248–51.
8. Zimmermann-Belsing T, Brabant G, Holst JJ, Feldt-Rasmussen U. Circulating leptin and thyroid dysfunction. Eur J Endocrinol (2003) 149(4):257–71.
10. De Pergola G, Ciampolillo A, Paolotti S, Trerotoli P, Giorgino R. Free triiodothyronine and thyroid stimulating hormone are directly associated with waist circumference, independently of insulin resistance, metabolic parameters and blood pressure in overweight and obese women. Clin Endocrinol (2007) 67(2):265–9.
11. Goossens GH. The Metabolic Phenotype in Obesity: Fat Mass, Body Fat Distribution, and Adipose Tissue Function. Obes Facts (2017) 10(3):207–15. doi: 10.1159/000471488
12. Guillet C. Skeletal Muscle in Obesity and Chronic Overfeeding. In: Nutrition and Skeletal Muscle. Academic Press (2019). p. 107–15.
13. Leon B, Jenkins S, Pepin K, Chaudhry H, Smith K, Zalos G, et al. Insulin and extremity muscle mass in overweight and obese women. Int J Obes (2013) 37(12):1560–4.
14. Arrojo E Drigo R, Bianco AC. Type 2 deiodinase at the crossroads of thyroid hormone action. Int J Biochem Cell Biol (2011) 43(10):1432–41.
15. Larsen PR. Type 2 iodothyronine deiodinase in human skeletal muscle: new insights into its physiological role and regulation. J Clin Endocrinol Metab (2009) 94(6):1893–5.
16. Matthews DR, Hosker JP, Rudenski AS, Naylor BA, Treacher DF, Turner RC. Homeostasis model assessment: insulin resistance and beta-cell function from fasting plasma glucose and insulin concentrations in man. Diabetologia (1985) 28(7):412–9.
17. Alberti KGMM, Zimmet P, Shaw J. Metabolic syndrome—a new world-wide definition. A consensus statement from the international diabetes federation. Diabet Med (2006) 23(5):469–80.
18. Ulijaszek SJ. Obesity: Preventing and Managing the Global Epidemic. Report of a WHO Consultation. WHO Technical Report Series 894. Pp. 252. (World Health Organization, Geneva, 2000.) SFr 56.00, ISBN 92-4-120894-5, paperback. J Biosocial Sci (2003) 35(4):624–5.
19. Beaudart C, Bruyère O, Geerinck A, Hajaoui M, Scafoglieri A, Perkisas S, et al. Belgian Aging Muscle Society (BAMS). Equation models developed with bioelectric impedance analysis tools to assess muscle mass: A systematic review. Clin Nutr ESPEN (2020) 35:47–62.
20. Kushner RF. Bioelectrical impedance analysis: a review of principles and applications. J Am Coll Nutr (1992) 11(2):199–209.
21. Kyle UG, Bosaeus I, De Lorenzo AD, Deurenberg P, Elia M, Manuel Gómez J, et al. Bioelectrical impedance analysis—part II: utilization in clinical practice. Clin Nutr (2004) 23(6):1430–53.
22. Kyle UG, Bosaeus I, De Lorenzo AD, Deurenberg P, Elia M, Gómez JM, et al. Bioelectrical impedance analysis—part I: review of principles and methods. Clin Nutr (2004) 23(5):1226–43.
23. Mebis L, Langouche L, Visser TJ, Van den Berghe G. The type II iodothyronine deiodinase is up-regulated in skeletal muscle during prolonged critical illness. J Clin Endocrinol Metab (2007) 92(8):3330–3.
24. Hosoi Y, Murakami M, Mizuma H, Ogiwara T, Imamura M, Mori M. Expression and regulation of type II iodothyronine deiodinase in cultured human skeletal muscle cells. J Clin Endocrinol Metab (1999) 84(9):3293–3300.24.
25. Shen W, Punyanitya M, Silva AM, Chen J, Gallagher D, Sardinha LB, et al. Sexual dimorphism of adipose tissue distribution across the lifespan: a cross-sectional whole-body magnetic resonance imaging study. Nutr Metab (2009) 6:17.
26. Arhire LI, Mihalache L, Covasa M. Irisin: A Hope in Understanding and Managing Obesity and Metabolic Syndrome. Front Endocrinol (Lausanne) (2019) 10:524. doi: 10.3389/fendo.2019.00524
27. Stratigou T, Dalamaga M, Antonakos G, Marinou I, Vogiatzakis E, Christodoulatos GS, et al. Hyperirisinemia is independently associated with subclinical hypothyroidism: correlations with cardiometabolic biomarkers and risk factors. Endocrine (2018) 61(1):83–93.
28. Rosenbaum M, Leibel RL. Role of Gonadal Steroids in the Sexual Dimorphisms in Body Composition and Circulating Concentrations of Leptin. J Clin Endocrinol Metab (1999) 84(6):1784–9.
29. Park SY, Park SE, Jung SW, Jin HS, Park IB, Ahn SV, et al. Free triiodothyronine/free thyroxine ratio rather than thyrotropin is more associated with metabolic parameters in healthy euthyroid adult subjects. Clin Endocrinol (2017) 87(1):87–96.
30. Lafortuna CL, Agosti F, De Col A, Pera F, Adorni F, Sartorio A. Prevalence of the metabolic syndrome and its components among obese men and women in Italy. Obes facts (2012) 5(1):127–37.
31. Kwon H, Cho JH, Lee DY, Park SE, Park CY, Lee WY, et al. Association between thyroid hormone levels, body composition and insulin resistance in euthyroid subjects with normal thyroid ultrasound: The Kangbuk Samsung Health Study. Clin Endocrinol (2018) 89(5):649–55.
32. Lartey LJ, Werneck-de-Castro JP, O-Sullivan I, Unterman TG, Bianco AC. Coupling between Nutrient Availability and Thyroid Hormone Activation. J Biol Chem (2015) 290:30551–61.
33. Savastano S, Belfiore A, Di Somma C, Mauriello C, Rossi A, Pizza G, et al. Validity of bioelectrical impedance analysis to estimate body composition changes after bariatric surgery in premenopausal morbidly women. Obes Surg (2010) 20(3):332–9.
34. Mukaka MM. Statistics corner: A guide to appropriate use of correlation coefficient in medical research. Malawi Med J (2012) 24(3):69–71.
35. Maltais ML, Desroches J, Dionne IJ. Changes in muscle mass and strength after menopause. J Musculoskelet Neuronal Interact (2009) 9(4):186–97.
Keywords: obesity, body composition, skeletal muscle mass, thyroid hormone, Italy
Citation: Zupo R, Castellana F, Sardone R, Lampignano L, Paradiso S, Giagulli VA, Triggiani V, Di Lorenzo L, Giannelli G and De Pergola G (2020) Higher Muscle Mass Implies Increased Free-Thyroxine to Free-Triiodothyronine Ratio in Subjects With Overweight and Obesity. Front. Endocrinol. 11:565065. doi: 10.3389/fendo.2020.565065
Received: 23 May 2020; Accepted: 10 September 2020;
Published: 29 September 2020.
Edited by:
Carla Lubrano, Sapienza University of Rome, ItalyReviewed by:
Hidetaka Hamasaki, Hamasaki Clinic, JapanCopyright © 2020 Zupo, Castellana, Sardone, Lampignano, Paradiso, Giagulli, Triggiani, Di Lorenzo, Giannelli and De Pergola. This is an open-access article distributed under the terms of the Creative Commons Attribution License (CC BY). The use, distribution or reproduction in other forums is permitted, provided the original author(s) and the copyright owner(s) are credited and that the original publication in this journal is cited, in accordance with accepted academic practice. No use, distribution or reproduction is permitted which does not comply with these terms.
*Correspondence: Roberta Zupo, enVwb3JvYmVydGFAZ21haWwuY29t
†ORCID: Roberta Zupo, orcid.org/0000-0001-9885-1185
Fabio Castellana, orcid.org/0000-0002-6439-8228
Rodolfo Sardone, orcid.org/0000-0003-1383-1850
Luisa Lampignano, orcid.org/0000-0001-9299-3211
Silvia Paradiso, orcid.org/0000-0003-1213-0331
Vito Angelo Giagulli, orcid.org/0000-0002-7073-1795
Vincenzo Triggiani, orcid.org/0000-0001-6308-0528
Luigi Di Lorenzo, orcid.org/0000-0002-4696-1435
Gianluigi Giannelli, orcid.org/0000-0002-5140-8060
Giovanni De Pergola, orcid.org/0000-0003-0020-3273
Disclaimer: All claims expressed in this article are solely those of the authors and do not necessarily represent those of their affiliated organizations, or those of the publisher, the editors and the reviewers. Any product that may be evaluated in this article or claim that may be made by its manufacturer is not guaranteed or endorsed by the publisher.
Research integrity at Frontiers
Learn more about the work of our research integrity team to safeguard the quality of each article we publish.