- 1Reproductive Medicine Center, The First Affiliated Hospital of Wenzhou Medical University, Wenzhou, China
- 2Department of Obstetrics and Gynecology, The First Affiliated Hospital of Wenzhou Medical University, Wenzhou, China
Levels of circulating adipokines in nonobese polycystic ovary syndrome (PCOS) patients have been reported in many studies. However, the results are inconsistent. The aim of this meta-analysis is to assess whether the levels of circulating adipokines are changed in nonobese PCOS relative to nonobese healthy controls. To identify eligible studies, a literature research was performed in the PubMed, Embase, and Web of Science databases without restricting by region, journal, or language. A total of 81 studies met the eligibility criteria. The meta-analysis showed that the circulating level of adiponectin (standardized mean difference [SMD]: -0.95; 95% CI: -1.36 to -0.53) was significantly decreased in nonobese PCOS patients. In contrast, the circulating levels of chemerin (SMD: 1.13; 95% CI: 0.08 to 2.18), leptin (SMD: 0.47; 95% CI: 0.13 to 0.81), resistin (SMD: 0.45; 95% CI: 0.03 to 0.88), and visfatin (SMD: 1.38; 95% CI: 0.68 to 2.09) were significantly increased in nonobese PCOS patients. There were no significant changes in the circulating levels of apelin (SMD: 0.32; 95% CI: -1.34 to 1.99), irisin (SMD: 1.01; 95% CI: -0.68 to 2.70), omentin (SMD: -0.37; 95% CI: -1.05 to 0.31), or vaspin (SMD: 0.09; 95% CI: -0.14 to 0.32). Thus, scientific evidence suggests that the circulating adipokine levels are altered in nonobese PCOS patients compared to nonobese healthy controls. Therefore, independent of the degree of obesity, dysregulated circulating adipokine levels might play important roles in the occurrence and development of PCOS.
Introduction
Polycystic ovary syndrome (PCOS) is a common endocrine and metabolic disease. It affects 6%–10% of reproductive-aged women, according to various diagnostic criteria (1). It is diagnosed based on sparse ovulation or anovulation, hyperandrogenism, and/or polycystic ovaries (2). Research has shown that the obesity rate among PCOS patients is significantly increased, which suggests that obesity may be related to the occurrence and symptoms of PCOS (3).
Fat tissue, which we often think of as a storage site for energy, is a crucial endocrine tissue in the body (4). Adipokines are active hormones and other factors that are secreted by adipocytes. Examples include adiponectin, apelin, chemerin, irisin, leptin, omentin, resistin, vaspin, and visfatin. As adipokines are involved in many critical physiological processes in the body, dysregulation of adipokines may lead to endocrine diseases (5). Additionally, research has shown that adipokines play an essential role in the pathogenesis of obesity and obesity-related diseases (6).
PCOS patients commonly have metabolic complications, such as type 2 diabetes, insulin resistance (IR), and adipose tissue dysfunction (7–9). IR affects about 10%–25% of the general population, but PCOS patients have 2–3 times the risk of IR (10, 11). In addition, the incidence of obesity is higher in patients with PCOS (relative risk [RR]: 2.77; 95% CI: 1.88 to 4.10) than in those without PCOS (12). Furthermore, PCOS symptoms in patients with both PCOS and obesity are significantly aggravated. However, the mechanism of PCOS has not been determined.
Adipose tissue dysfunction can lead to changes in adipokine levels (13). Many studies show that there are changes in adipokine levels in obese PCOS patients, indicating that adipokines may play a role in obese PCOS patients (14, 15). However, studies on adipokine levels in nonobese PCOS patients have been inconsistent. Moreover, it is not known whether the changes in adipokines are related directly to PCOS, to obesity, or to both. Thus, we aimed to perform this systematic review and meta-analysis to evaluate the levels of various adipokines in nonobese women with PCOS and in nonobese healthy controls.
Materials and Methods
Reporting Guidelines
This systematic review and meta-analysis was designed according to the Preferred Reporting Items for Systematic Reviews and Meta-Analyses (PRISMA) statement (16) and the Meta-analysis of Observational Studies in Epidemiology (MOOSE) guidelines (17).
Eligibility Criteria
Studies published before July 2019 that define PCOS in line with the Rotterdam Criteria or other compatible criteria were considered for eligibility. The following studies were excluded: 1) studies on obese (BMI ≥30.0) women with PCOS; 2) reviews, nonhuman studies, and conference proceedings; 3) studies without control groups; and 4) studies without extractable data (data not provided as mean ± standard deviation [SD]).
Databases
To identify eligible studies, an exhaustive literature search was performed in the PubMed, Embase, and Web of Science databases without restricting by location, journal, or language.
Search Strategy
The search strategy involved the identification of studies on the levels of adiponectin, apelin, chemerin, irisin, leptin, omentin, resistin, vaspin, and/or visfatin, using the following keywords: (“polycystic ovary syndrome” OR “PCOS”) AND (“adiponectin” OR “apM-1 protein” OR “ACRP30 protein”) OR (“apelin”) OR (“chemerin” OR “TIG2 protein”) OR (“irisin” OR “FRCP2 protein”) OR (“leptin” OR “obese protein”) OR (“omentin” OR “intelectin 1”) OR (“resistin”) OR (“vaspin” OR “SERPINA12 protein”) OR (“visfatin” OR “NAMPT protein”).
Study Selection and Data Extraction
Two reviewers independently reviewed the studies and determined whether they met the eligibility criteria. Disagreements between the two reviewers were resolved by discussion and, if necessary, consultation with a third reviewer. The following information was extracted: general study characteristics (name of the first author, year of publication, and study location), age and body mass index (BMI) of participants, summary of study conclusions, and data on adipokine levels.
Assessment of Risk of Bias and Publication Bias
We used the Cochrane Collaboration tool to assess the risk of bias in the included studies. This tool involves seven domains: random sequence generation, allocation concealment, blinding of participants and personnel, outcome assessment, incomplete outcome data, selective reporting, and other biases. Each domain was independently evaluated (as having a high, low, or unclear risk of bias) by two reviewers, and disagreements were resolved by discussion. The possibility of publication bias was assessed by visual inspection of a funnel plot and Egger’s test.
Statistical Analysis
Review Manager version 5.3 was used to perform the meta-analysis of the differences in mean adipokine levels between nonobese PCOS patients and nonobese healthy controls and to construct the forest plots. Due to the different measurement methods among studies, Review Manager was used to calculate the standardized mean difference (SMD) with 95% confidence intervals (CIs). Cochran’s Q test and the I2 test were used to assess the heterogeneity among studies. We selected random or fixed effects models according to whether high/moderate or low heterogeneity, respectively, was detected. STATA version 15.0 was used to conduct Egger’s test to assess the publication bias (with P<0.05 indicating publication bias), and the trim-and-fill method was used to recalculate the SMD if publication bias was detected.
Results
Study Selection
Our search strategy identified 1540 articles, but 1256 were excluded after screening the titles and abstracts. Thereafter, 284 potentially relevant articles were assessed by reviewing the full text. Among these articles, 203 were excluded due to lack of a control group or inclusion of obese subjects and 10 were excluded because the data were not presented as mean ± SD. Thus, 71 studies (comprising 2495 PCOS patients and 2520 controls) met our eligibility criteria. Figure 1 shows the flow diagram of study selection.
Characteristics of Included Studies
The features of the included studies are presented in Table 1. Among the 81 included studies, several sets of PCOS diagnostic criteria were used (53 studies used the Rotterdam criteria, 9 used the National Institutes of Health (NIH) criteria; 3 used the ESHRE/ASRM consensus criteria, 2 used the National Institute of Child Health and Human Disease criteria, and the other studies used specific standards set out in the studies themselves). The studies were conducted in 26 countries (19 in Turkey, 8 in China, 8 in Poland, 7 in Greece, 4 in Egypt, 4 in Italy, 4 in Iran, 3 in India, 3 in South Korea, 3 in Taiwan, 2 in Brazil, 2 in Saudi Arabia, 1 in Croatia, 1 in the Netherlands, 1 in Pakistan, 1 in Denmark, 1 in France, 1 in the United States, 1 in Israel, 1 in Indonesia, 1 in Iraq, 1 in Germany, 1 in Japan, 1 in Australia, 1 in Spain, and 1 in Qatar). In the vast majority of the studies, the age of most participants was between 20 and 25 years. As there were no significant differences in age between the PCOS patients and the controls in any of the studies, we can exclude the influence of age on the results. The participants all had a BMI <30.0 (the World Health Organization criterion for obesity is BMI ≥30.0). Table 1 also briefly lists the primary conclusions of each study.
Associations of PCOS With Adipokine Levels
Thirty studies (n=2565 participants) compared the adiponectin level between nonobese PCOS patients and controls (Figure 2A), and there was significant heterogeneity among the studies (I2 = 95%; P<0.00001). PCOS was significantly associated with a decreased adiponectin level (SMD: -0.95; 95% CI: -1.36 to -0.53; P<0.00001).
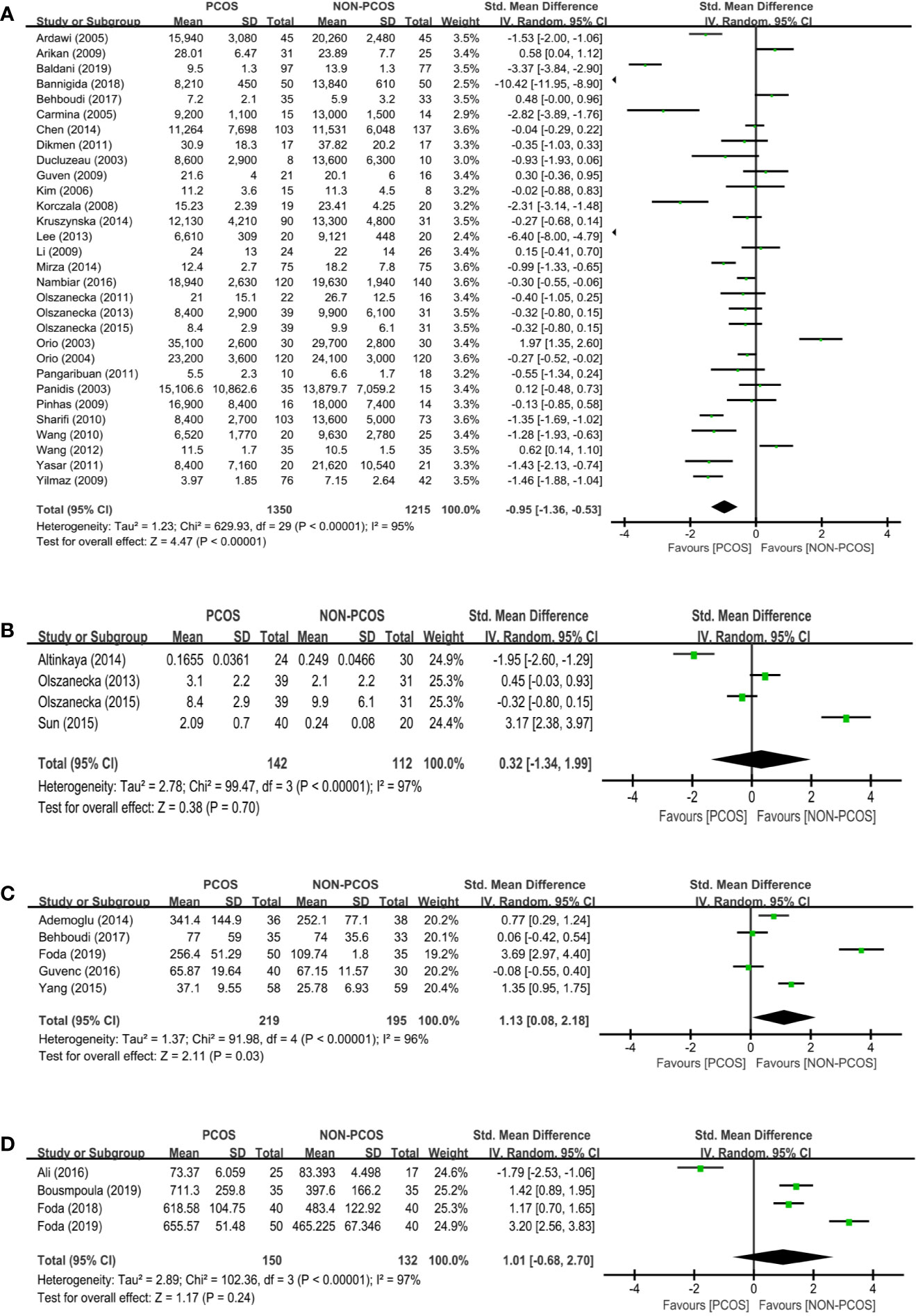
Figure 2 Meta-analysis of the association between the levels of adipokines in nonobese patients and PCOS. Weights are from random effects analysis. (A) Meta-analysis of adiponectin. (B) Meta-analysis of apelin. (C) Meta-analysis of chemerin. (D) Meta-analysis of irisin. PCOS, polycystic ovary syndrome; CI, confidence interval; SD, standard difference.
Four studies (n=254 participants) compared the apelin level between nonobese PCOS patients and controls (Figure 2B). There was no significant association between PCOS and apelin (SMD: 0.32; 95% CI: -1.34 to 1.99; P=0.70).
Five studies (n=414 participants) compared the chemerin level between nonobese PCOS patients and controls (Figure 2C), and there was significant heterogeneity among the studies (I2 = 96%; P<0.00001). PCOS was significantly associated with an increased chemerin level (SMD: 1.13; 95% CI: 0.08 to 2.18; P=0.03).
Four studies (n=282 participants) compared the irisin level between nonobese PCOS patients and controls (Figure 2D), and there was significant heterogeneity among the studies (I2 = 97%; P<0.00001). There was no significant association between PCOS and irisin (SMD: 1.01; 95% CI: -0.68 to 2.70; P=0.24).
Twenty-five studies (n=2148 participants) compared the leptin level between nonobese PCOS patients and controls (Figure 3A). PCOS was significantly associated with an increased leptin level (SMD: 0.47; 95% CI: 0.13 to 0.81; P=0.007).
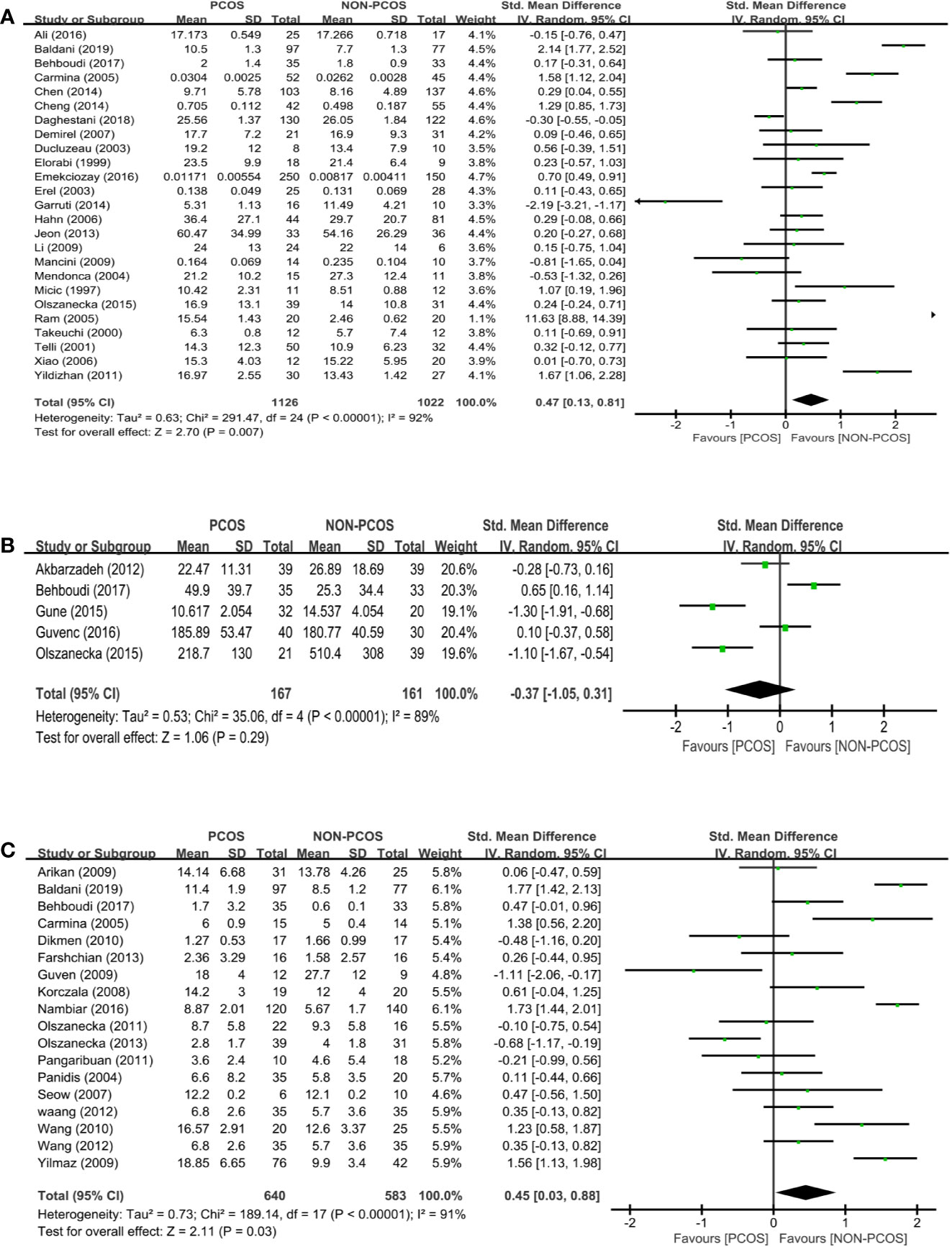
Figure 3 Meta-analysis of the association between the levels of adipokines in nonobese patients and PCOS. Weights are from random effects analysis. (A) Meta-analysis of leptin. (B) Meta-analysis of omentin. (C) Meta-analysis of resistin. PCOS, polycystic ovary syndrome; CI, confidence interval; SD, standard difference.
Five studies (n=328 participants) compared the omentin level between nonobese PCOS patients and controls (Figure 3B), and there was significant heterogeneity among the studies (I2 = 89%; P<0.00001). There was no significant association between PCOS and omentin (SMD: -0.37; 95% CI: -1.05 to 0.31; P=0.29).
Eighteen studies (n=1223 participants) compared the resistin level between nonobese PCOS patients and controls (Figure 3C), and there was significant heterogeneity among the studies (I2 = 91%; P<0.00001). PCOS was associated with an increased resistin level (SMD: 0.45; 95% CI: 0.03 to 0.88; P=0.03; n=18 studies).
Three studies (n=348 participants) compared the vaspin level between nonobese PCOS patients and controls (Figure 4A). There was no significant association between PCOS and vaspin (SMD: 0.09; 95% CI: -0.14 to 0.32; P=0.43).
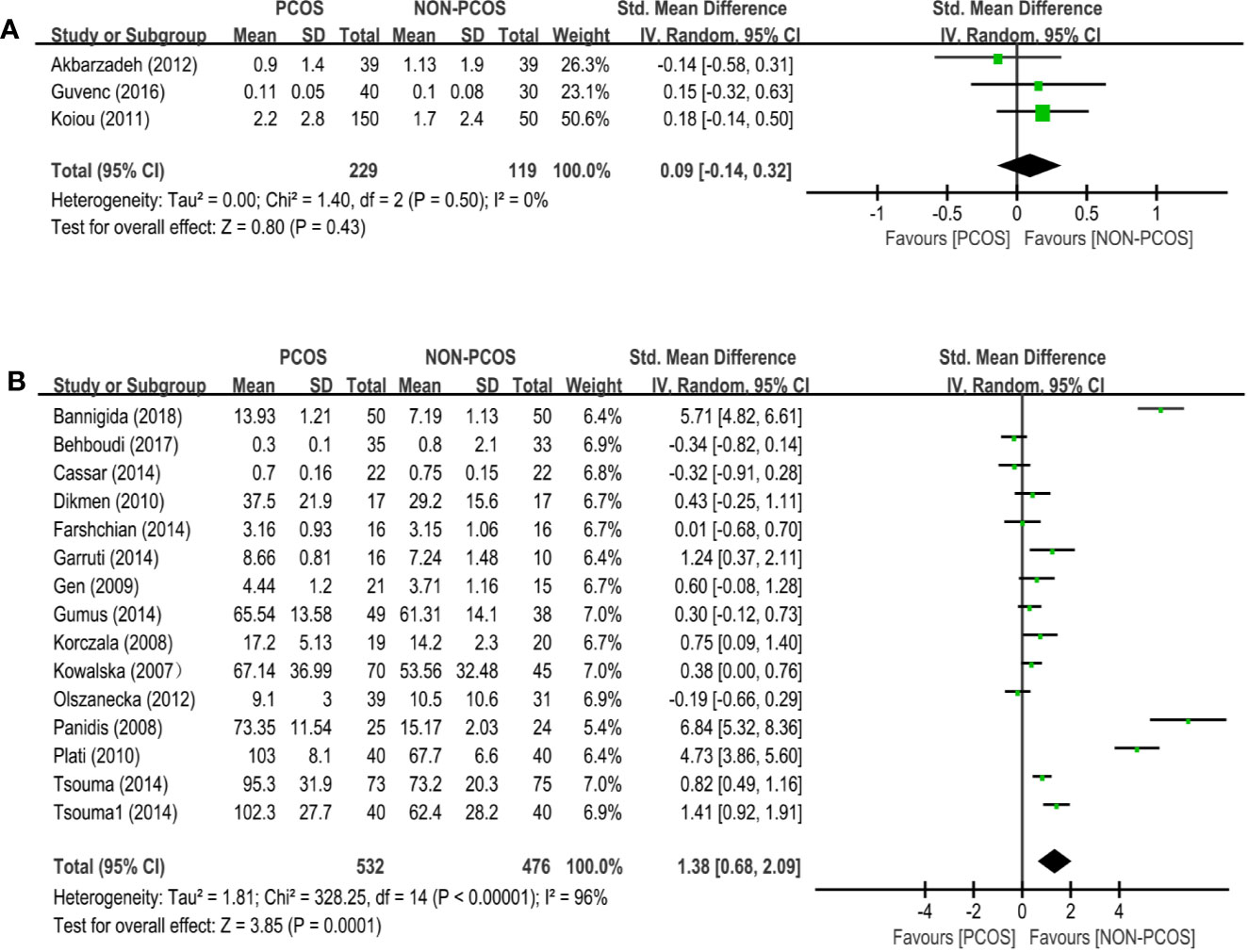
Figure 4 Meta-analysis of the association between the levels of adipokines in nonobese patients and PCOS. Weights are from random effects analysis. (A) Meta-analysis of vaspin. (B) Meta-analysis of visfatin. PCOS, polycystic ovary syndrome; CI, confidence interval; SD, standard difference.
Twenty-six studies (n=1008 participants) compared the visfatin level between nonobese PCOS patients and controls (Figure 4B), and there was significant heterogeneity among the studies (I2 = 96%; P<0.00001). PCOS was associated with an increased visfatin level (SMD: 1.38; 95% CI: 0.68 to 2.09; P=0.0001).
Publication Bias
The funnel plot method was used to detect any publication bias, and Egger’s test was used to quantify the publication bias. Publication bias can be due to systematic reviewers who, by not including unpublished literature, lead to final statistical conclusions that differ from actual results. Regarding adiponectin and resistin, the shape of the funnel plots was not obviously asymmetric. However, regarding leptin and visfatin, the funnel plots indicated observable publication bias (Figures 5A–D). Egger’s test shows no evidence of publication bias regarding adiponectin and leptin, but there is possible publication bias regarding resistin and visfatin (Table 2). We used the trim-and-fill method and recalculated our pooled SMD values for resistin and visfatin, and the corrected and uncorrected SMD values did not differ, which suggested that publication bias did not affect the final results. We did not assess the publication bias for apelin, chemerin, irisin, omentin, or vaspin, as the Cochrane Handbook for Systematic Reviews of Interventions (www.cochranehandbook.org) states that the test for publication bias yields unreliable results when <10 studies are included.
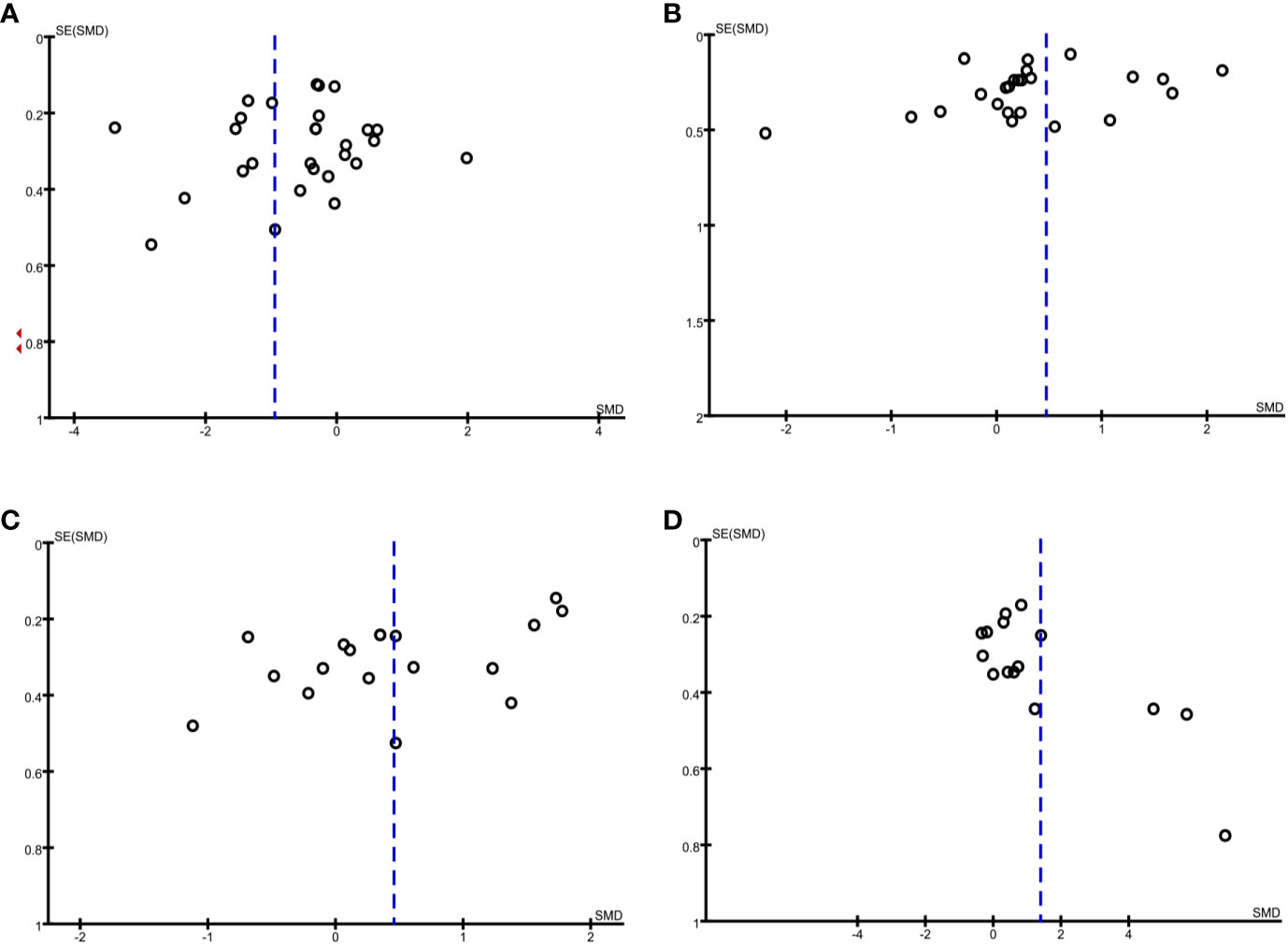
Figure 5 Funnel plot analysis to detect publication bias under a dominant model. (A) Funnel plot for adiponectin. (B) Funnel plot for leptin. (C) Funnel plot for resistin. (D) Funnel plot for visfatin.
Discussion
To our knowledge, this is the first systematic review and meta-analysis quantifying the levels of nine adipokines in nonobese PCOS patients. This systematic review and meta-analysis of 81 studies demonstrates that nonobese PCOS patients have a significantly decreased adiponectin level and significantly increased leptin, visfatin, chemerin, and resistin levels. Thus, our results indicate that the concentration of common adipokines in plasma or serum were remarkably altered in nonobese PCOS patients.
Over the past decade, many studies have investigated circulating adipokine levels in nonobese PCOS patients. The results of the studies are inconsistent, with adipokine levels differing by geographical region, ethnicity, and age. For example, a study by Alfaqih et al. shows that adipokine levels in PCOS patients were significantly lower than those in controls (20), whereas a study by Arikan et al. shows significantly higher levels (21). The purpose of this meta-analysis was to integrate these results in a statistical analysis. Combining study findings is an attractive option to enhance the credibility of the evidence (22). Therefore, we performed a meta-analysis to reach a reliable conclusion on the changes in adipokine levels in nonobese PCOS patients.
Changes in adipokine levels in PCOS patients might be caused by obesity rather than the PCOS itself. This should be considered because adipokine levels are related to BMI (23), and PCOS patients tend to have a higher BMI (24) with a meta-analysis reporting higher rates of overweight (RR: 1.95; 95% CI: 1.52 to 2.50), obesity (RR: 2.77; 95% CI: 1.88 to 4.10), and central obesity (RR: 1.73; 95% CI: 1.31 to 2.30) in women with PCOS compared with women without PCOS (25). To exclude the influence of obesity and directly analyze the relationships between PCOS and adipokine levels, we only included studies on nonobese PCOS patients in our meta-analysis.
Understanding the dysregulation of adipokine levels in nonobese PCOS patients may help to explain the pathophysiology and symptoms of PCOS, which are thought to involve hyperandrogenism, IR, and chronic inflammation. 1) Hyperandrogenism: the occurrence of PCOS is closely related to hyperandrogenism (26). After androgen receptor knockdown in adipocytes in mice, the adipokine levels were altered, and the impaired insulin sensitivity and poor glucose tolerance were found not to be associated with obesity. Therefore, androgen receptors in adipocytes may influence adipokine levels, and they may control insulin sensitivity and glucose tolerance independently of obesity (27). Additionally, in nonobese PCOS patients, androgen hypersecretion and androgen receptor dysfunction may underlie the changes in the adipokine levels as they are the main signs of the condition (28). Moreover, sex hormones influence adipokine levels, which provides further evidence that the increase in androgens in PCOS patients might influence the adipokine levels (29). Furthermore, a study involving triptorelin treatment provided evidence that hyperandrogenemia may increase the level of the adipokine leptin (in addition to leptin influencing androgens). More precisely, although leptin may influence the gonadal axis, the gonadal axis itself may also dramatically upregulate leptin secretion as indicated by the significant associations in women between the percentage increase in circulating leptin levels (relative to baseline) and the percentage increases in both the free androgen index and the testosterone levels following triptorelin treatment (30). 2) IR: the occurrence of PCOS is closely related to IR (31). In vitro research has shown that, in fat cells, certain adipokines (such as omentin) activate protein kinases that increase insulin-mediated glucose transport, thus increasing insulin sensitivity (32). Additionally, high leptin levels inhibit proinsulin mRNA expression, involving decreased transcription activity of the insulin gene promoter and decreased phosphorylation of insulin receptors in peripheral tissues (33, 34). High leptin levels may lead to IR in nonobese PCOS patients by promoting the following events: (i) inhibition of phosphoenolpyruvate carboxykinase, causing decreased hepatic glucose oxidation and increased hepatic glycogen reserve; (ii) fat deposition in skeletal muscle cells; (iii) fat breakdown and free fatty acid production; and (iv) direct inhibition of basal insulin secretion and glucose-stimulated insulin secretion. Furthermore, the insulin sensitizer thiazolidinedione upregulates adiponectin mRNA expression and promotes the differentiation and apoptosis of adipocytes, thus reducing IR. Therefore, dysregulated adipokine levels may play important roles in IR in nonobese PCOS patients, and PCOS treatment could involve correcting the dysregulated adipokine levels. In particular, we need to consider the role of low serum adiponectin levels in causing IR in women with PCOS as adiponectin (i) decreases muscle triglyceride content, (ii) regulates the insulin receptor, (iii) activates peroxisome proliferator-activated receptor α (PPARα) to increase fatty acid oxidation, (iv) reduces hepatic glucose production by activating protein kinases, (v) increases fat oxidation in muscle, and (vi) inhibits the expression of gluconeogenic enzymes (35). 3) Chronic inflammation: The proinflammatory adipokine chemerin was discovered to promote the chemotaxis of immature dendritic cells and macrophages via its receptor CMKLR1 (36). Chemerin and CMKLR1 (which is expressed on immature dendritic cells, myeloid dendritic cells, macrophages, and natural killer cells) were later shown to recruit immune cells to injury sites and might affect the occurrence and development of inflammation (37, 38). Adipokines and their receptors are elevated in many inflammatory states, and adipokine levels might be predictors of PCOS. Additionally, obesity involves a chronic low-grade inflammatory state, and this long-term inflammatory stimulation may partly underlie the downregulation of omentin (which is an adipokine with anti-inflammatory, anticardiovascular disease, and antidiabetic effects) (39, 40).
Research shows that both environmental and genetic factors play important roles in the development of PCOS. Studies on whether insulin receptor gene mutations play a role in the pathogenesis of PCOS all yield negative results, indicating that these mutations are unlikely to be the main cause of IR in PCOS. However, CYP11A promoter polymorphisms are shown to be associated with the pathogenesis of PCOS. The CYP11A protein catalyzes the rate-limiting step of the pathway that produces pregnenolone (an androgen precursor) from cholesterol, so CYP11A promoter polymorphisms that upregulate its expression may upregulate ovarian or adrenal androgen secretion. However, the specific mechanism remains unclear, and there is conflicting experimental evidence regarding the fact that the polymorphisms cause increased androgen secretion (41).
In addition, the roles of adipokine gene polymorphisms in the pathogenesis of PCOS may be important. A Finnish study suggests that adiponectin gene polymorphisms may increase susceptibility to PCOS as the T allele studied is significantly reduced in PCOS patients compared to controls (odds ratio: 0.72; 95% CI: 0.52 to 0.99; P=0.047) (42). However, the adiponectin gene polymorphisms 45 T→G and 276 G→T are not significantly different between PCOS patients and controls. Nevertheless, these polymorphisms are associated with adiponectin levels and the levels of IR-associated metabolic variables. These polymorphisms may be in linkage disequilibrium with several other loci that primarily function to produce and polymerize adiponectin, thereby affecting adipokine levels (43). A follow-up study reports similar results, demonstrating that PCOS is not associated with the adiponectin 45 T→G and 276 G→T polymorphisms. In addition, hyperandrogenemia and abdominal obesity are the main causes of low serum adiponectin levels in PCOS patients, which may explain the link between the former two factors and the IR experienced by PCOS patients (as decreased adiponectin may cause IR) (44). Mice with a mutant leptin receptor (Y123F mice with three tyrosine residues [Tyr985, Tyr1077, and Tyr1138] replaced with phenylalanine) had atrophied ovaries and abnormal follicle development along with fewer ovarian leptin receptors, which may involve effects on JAK-STAT signaling and on hormone biosynthetic pathways (45). A study of PCOS patients and their parents reports that the resistin gene is near the D19S884 polymorphic marker, which is closely linked to PCOS, and both the resistin gene and D19S884 are reasonable candidate susceptibility loci for PCOS (46). A study of women in India shows that the resistin gene 420 C→G (promoter region) and 299 G→A polymorphisms are positively associated with the incidence of PCOS (with the heterozygous CG genotype, regarding the former polymorphism, being more common in PCOS patients than controls) (19). Both polymorphisms are significantly associated with cerebrovascular disease in type 2 diabetes patients and with the development of IR, which may explain the development of IR in PCOS patients (47). Another study shows that vaspin gene rs2236242 polymorphism is significantly different between PCOS patients and controls (odds ratio: 0.59; 95% CI: 0.37 to 0.95; P=0.03). However, after adjusting for BMI, the results are not significant (48). Overall, studies on the role of adipokine gene polymorphisms in PCOS are still relatively rare and inconsistent, so more research is needed to demonstrate their roles.
Nonobese PCOS patients with dysregulated adipokine levels can develop related diseases over time, such as type 2 diabetes, coronary heart disease, and hypertension (49). Type 2 diabetes is almost 10 times more common in PCOS patients than in the normal population, and glucose intolerance is 30%–50% higher in obese PCOS patients (50). The risk of cardiovascular disease in PCOS patients should also not be ignored. As PCOS is closely related to IR, this can lead to a variety of abnormalities, such as hypertension, glucose intolerance, diabetes, metabolic syndrome, and dyslipidemia, which increase the risk of cardiovascular disease in women (51).
Adipokine levels may be of great significance in the diagnosis and treatment of PCOS, and increased understanding of the abnormal changes in adipokine levels is required. Metformin might alleviate the symptoms and treat PCOS by correcting the abnormal levels of certain adipokines, such as resistin, visfatin, irisin, and chemerin levels (18, 52–54). For example, metformin may alleviate abnormal visfatin levels in PCOS patients by increasing peripheral insulin sensitivity and thereby decreasing the IR-induced hyperinsulinemia, which, in turn, may lower visfatin secretion because insulin and glucose affect visfatin secretion via the phosphatidylinositol 3-kinase/protein kinase B pathway (55). It is believed that adipokines and hyperandrogenemia form a vicious endocrine metabolism cycle, increasing the risk of PCOS and other related endocrine diseases. Relatedly, metformin may also alleviate PCOS symptoms via reducing high testosterone levels (56). However, more studies are needed to prove whether metformin can correct dysregulated adipokine levels and, thus, treat PCOS.
There is significant statistical heterogeneity among the studies that assess adiponectin, apelin, chemerin, irisin, leptin, omentin, resistin, and visfatin levels. This might reflect clinical heterogeneity and, more specifically, differences in the PCOS diagnostic criteria used. Differences in location, ethnicity, age, and/or BMI may also have played a role. For example, the BMI thresholds used to determine obesity differ among the studies. Caution should be exercised when extrapolating the results to other situations. However, the analysis of apelin shows no heterogeneity, indicating that the included studies are homogeneous and the study results are accurate.
Furthermore, we find evidence of publication bias. Publication bias is a phenomenon in the medical literature that involves positive findings having a greater chance of being published. Performing a meta-analysis after using the trim-and-fill method to correct for funnel plot asymmetry arising from publication bias can indicate whether publication bias has impacted the results. Our results for resistin and visfatin after using the trim-and-fill method suggest that publication bias did not affect the conclusions (i.e., PCOS remained significantly associated with increased resistin and visfatin levels). However, some studies came to a different conclusion (i.e., PCOS is not associated with resistin or visfatin levels), so more consideration is still needed before potentially applying the results in clinical practice.
Our literature search was comprehensive, and we did not apply any region, journal, or language restrictions that would limit the assessment of the relationships between the adipokine levels and PCOS. However, our meta-analysis still has several limitations. First, many different sets of criteria are used for the identification of PCOS, so the diagnosis of PCOS is associated with heterogeneity. Second, different regions have different definitions of obesity; for example, the World Health Organization developed the world standard of BMI >30 for obesity although BMI >28 is considered to be obese in China. Given this, not all obese PCOS patients (e.g., based on the less stringent criteria of BMI >28) may have been excluded, so obesity may have influenced the results. Third, many different methods are used to determine the adipokine levels in the blood, which may have led to some deviations among the studies. Last, many studies demonstrate that PCOS patients are much more likely to develop hyperandrogenemia, and we did not rule out the effect of androgen levels on adipokine levels. Despite these limitations, our meta-analysis increased the statistical power by pooling the results of single studies, which meant that the total number of subjects was sufficiently large to support more robust conclusions.
In summary, this systematic review and meta-analysis demonstrates that the circulating adipokine levels in nonobese PCOS patients are changed to varying degrees relative to those in nonobese healthy controls. This may help to identify the pathogenesis of PCOS and new biochemical diagnostic criteria for PCOS. Moreover, these results might provide insights into new PCOS treatment approaches involving correcting dysregulated adipokine levels. However, further studies are needed to explore the potential mechanisms underlying the dysregulation in adipokine levels and to determine whether the PCOS could be treated by correcting this dysregulation.
Author Contributions
KL conducted the literature search, compiled the data, and drafted the manuscript. XS and XW contributed to the literature search and data interpretation. HW reviewed the manuscript and provided advice. XC contributed to critical discussion, provided extensive advice, and reviewed and revised the manuscript drafts. All authors contributed to the article and approved the submitted version.
Funding
This work was supported by Wenzhou Municipal Science and Technology Bureau (Y20180271) and the Health and Family Planning Commission of Zhejiang Province (2019317125).
Conflict of Interest
The authors declare that the research was conducted in the absence of any commercial or financial relationships that could be construed as a potential conflict of interest.
Acknowledgments
The authors would like to thank Liangshan Mu for his excellent technical support and for critically reviewing the manuscript. Thanks to Charlesworth Author Services for language retouching and formatting corrections.
References
1. Dean HJ, Sellers EAC, Bozdag G, Mumusoglu S, Zengin D, Karabulut E, et al. The prevalence and phenotypic features of polycystic ovary syndrome: a systematic review and meta-analysis. Hum Reprod (2016) 31(12):2841–55. doi: 10.1093/humrep/dew218
2. Palomba S, de Wilde MA, Falbo A, Koster MP, La Sala GB, Fauser BC. Pregnancy complications in women with polycystic ovary syndrome. Hum Reprod Update (2015) 21(5):575–92. doi: 10.1093/humupd/dmv029
3. Joham AE, Palomba S, Hart R. Polycystic Ovary Syndrome, Obesity, and Pregnancy. Semin Reprod Med (2016) 34(2):93–101. doi: 10.1055/s-0035-1571195
4. Holst D, Grimaldi PA. New factors in the regulation of adipose differentiation and metabolism. Curr Opin Lipidol (2002) 13(3):241–5. doi: 10.1097/00041433-200206000-00002
5. Havel PJ. Control of energy homeostasis and insulin action by adipocyte hormones: leptin, acylation stimulating protein, and adiponectin. Curr Opin Lipidol (2002) 13(1):51–9. doi: 10.1097/00041433-200202000-00008
6. Chen T, Wang F, Chu Z, Shi X, Sun L, Lv H, et al. Serum CTRP3 Levels In Obese Children: A Potential Protective Adipokine Of Obesity, Insulin Sensitivity And Pancreatic β Cell Function. Diabetes Metab Syndr Obes Targets Ther (2019) 12:1923–30. doi: 10.2147/dmso.S222066
7. Legro RS, Kunselman AR, Dodson WC, Dunaif A. Prevalence and predictors of risk for type 2 diabetes mellitus and impaired glucose tolerance in polycystic ovary syndrome: A prospective, controlled study in 254 affected women. J Clin Endocrinol Metab (1999) 84(1):165–9. doi: 10.1210/jc.84.1.165
8. Stepto NK, Cassar S, Joham AE, Hutchison SK, Harrison CL, Goldstein RF, et al. Women with polycystic ovary syndrome have intrinsic insulin resistance on euglycaemic-hyperinsulaemic clamp. Hum Reprod (2013) 28(3):777–84. doi: 10.1093/humrep/des463
9. Spritzer PM, Lecke SB, Satler F, Morsch DM. Adipose tissue dysfunction, adipokines, and low-grade chronic inflammation in polycystic ovary syndrome. Reprod (Cambridge Engl) (2015) 149(5):R219–R27. doi: 10.1530/rep-14-0435
10. Sirmans SM, Pate KA. Epidemiology, diagnosis, and management of polycystic ovary syndrome. Clin Epidemiol (2014) 6:1–13. doi: 10.2147/clep.S37559
11. Dean HJ, Sellers EA. Type 2 diabetes, polycystic ovary syndrome and the insulin resistance syndrome in adolescents: Are they one big iceberg? Paediatr Child Health (2002). doi: 10.1093/pch/7.5.333
12. Lim SS, Davies MJ, Norman RJ, Moran LJ. Overweight, obesity and central obesity in women with polycystic ovary syndrome: a systematic review and meta-analysis. Hum Reprod Update (2012) 18(6):618–37. doi: 10.1093/humupd/dms030
13. Maury E, Brichard SM. Adipokine dysregulation, adipose tissue inflammation and metabolic syndrome. Mol Cell Endocrinol (2010) 314(1):1–16. doi: 10.1016/j.mce.2009.07.031
14. Itoh H, Kawano Y, Furukawa Y, Matsumoto H, Yuge A, Narahara H. The role of serum adiponectin levels in women with polycystic ovarian syndrome. Clin Exp Obstet Gynecol (2013) 40(4):531–5.
15. Kumawat M, Ram M, Agarwal S, Singh V. Role of serum Leptin, insulin and other hormones in women with Polycystic ovarian syndrome. Indian J Clin Biochem (2018) 33:S88–S9. doi: 10.1007/s12291-018-0795-1
16. Knobloch K, Yoon U, Vogt PM. Preferred reporting items for systematic reviews and meta-analyses (PRISMA) statement and publication bias. J Craniomaxillofac Surg (2009) 18: (3):e123.
17. Stroup DF, Berlin JA, Morton SC, Olkin I, Williamson GD, Rennie D, et al. Meta-analysis of observational studies in epidemiology: a proposal for reporting. Meta-analysis Of Observational Studies in Epidemiology (MOOSE) group. JAMA (2000) 283(15):2008–12. doi: 10.1001/jama.283.15.2008
18. Foda AA, Foda EA, El-Negeri MA, El-Said ZH. Serum chemerin levels in Polycystic Ovary Syndrome after metformin therapy. Diabetes Metab Syndr (2019) 13(2):1309–15. doi: 10.1016/j.dsx.2019.01.050
19. Nambiar V, Vijesh VV, Lakshmanan P, Sukumaran S, Suganthi R. Association of adiponectin and resistin gene polymorphisms in South Indian women with polycystic ovary syndrome. Eur J Obstet Gynecol Reprod Biol (2016) 200:82–8. doi: 10.1016/j.ejogrb.2016.02.031
20. Alfaqih MA, Khader YS, Al-Dwairi AN, Alzoubi A, Al-Shboul O, Hatim A. Correction: Lower Levels of Serum Adiponectin and the T Allele of rs1501299 of the ADIPOQ Gene Are Protective against Polycystic Ovarian Syndrome in Jordan. Korean J Family Med (2018) 39(3):207. doi: 10.4082/kjfm.2018.39.3.207
21. Arikan S, Bahceci M, Tuzcu A, Kale E, Gokalp D. Serum resistin and adiponectin levels in young non-obese women with polycystic ovary syndrome. Gynecol Endocrinol (2010) 26(3):161–6. doi: 10.3109/09513590903247816
22. DerSimonian R, Laird N. Meta-analysis in clinical trials. Controlled Clin Trials (1986) 7(3):177–88. doi: 10.1016/0197-2456(86)90046-2
23. Saremi A, Asghari M, Ghorbani A. Effects of aerobic training on serum omentin-1 and cardiometabolic risk factors in overweight and obese men. J Sports Sci (2010) 28(9):993–8. doi: 10.1080/02640414.2010.484070
24. Barrea L, Arnone A, Annunziata G, Muscogiuri G, Laudisio D, Salzano C, et al. Adherence to the Mediterranean Diet, Dietary Patterns and Body Composition in Women with Polycystic Ovary Syndrome (PCOS). Nutrients (2019) 11(10):22–78. doi: 10.3390/nu11102278
25. Lim SS, Davies MJ, Norman RJ, Moran LJ. Overweight, obesity and central obesity in women with polycystic ovary syndrome: a systematic review and meta-analysis. Hum Reprod Update (2012) 18(6):618–37. doi: 10.1093/humupd/dms030
26. Wang X, Wang H, Liu W, Zhang Z, Zhang Y, Zhang W, et al. High level of C-type natriuretic peptide induced by hyperandrogen-mediated anovulation in polycystic ovary syndrome mice. Clin Sci (Lond Engl 1979) (2018) 132(7):759–76. doi: 10.1042/cs20171394
27. McInnes KJ, Smith LB, Hunger NI, Saunders PT, Andrew R, Walker BR. Deletion of the androgen receptor in adipose tissue in male mice elevates retinol binding protein 4 and reveals independent effects on visceral fat mass and on glucose homeostasis. Diabetes (2012) 61(5):1072–81. doi: 10.2337/db11-1136
28. Cree-Green M, Torres M, Pyle L, Scherzinger A, Kelsey MM, Nadeau KJ. Extreme hyperandrogenism worsens metabolic but not dermatologic findings within obese adolescents with polycystic ovarian syndrome. Horm Res Paediatr (2017) 88:58–9. doi: 10.1159/000481424
29. Martínez-García M, Montes-Nieto R, Fernández-Durán E, Insenser M, Luque-Ramírez M, Escobar-Morreale HF. Evidence for masculinization of adipokine gene expression in visceral and subcutaneous adipose tissue of obese women with polycystic ovary syndrome (PCOS). J Clin Endocrinol Metab (2013) 98(2):E388–96. doi: 10.1210/jc.2012-3414
30. Escobar-Morreale HF, Serrano-Gotarredona J, Varela C, García-Robles R, Sancho JM. Circulating leptin concentrations in women with hirsutism. Fertil steril (1997) 68(5):898–906. doi: 10.1016/s0015-0282(97)00336-1
31. Wang J, Wu D, Guo H, Li M. Hyperandrogenemia and insulin resistance: The chief culprit of polycystic ovary syndrome. Life Sci (2019) 236:116940. doi: 10.1016/j.lfs.2019.116940
32. Yang RZ, Lee MJ, Hu H, Pray J, Wu HB, Hansen BC, et al. Identification of omentin as a novel depot-specific adipokine in human adipose tissue: possible role in modulating insulin action. Am J Physiol Endocrinol Metab (2006) 290(6):E1253–61. doi: 10.1152/ajpendo.00572.2004
33. Tan BK, Adya R, Farhatullah S, Lewandowski KC, O’Hare P, Lehnert H, et al. Omentin-1, a novel adipokine, is decreased in overweight insulin-resistant women with polycystic ovary syndrome - Ex vivo and in vivo regulation of omentin-1 by insulin and glucose. Diabetes (2008) 57(4):801–8. doi: 10.2337/db07-0990
34. Barzilai N, Wang J, Massilon D, Vuguin P, Hawkins M, Rossetti L. Leptin selectively decreases visceral adiposity and enhances insulin action. J Clin Invest (1997) 100: (12):3105–10. doi: 10.1172/JCI119865
35. Kadowaki T, Yamauchi T. Adiponectin and adiponectin receptors. Endocr Rev (2005) 26(3):439–51. doi: 10.1210/er.2005-0005
36. Wittamer V, Franssen J-D, Vulcano M, Mirjolet J-F, Le Poul E, Migeotte I, et al. Specific Recruitment of Antigen-presenting Cells by Chemerin, a Novel Processed Ligand from Human Inflammatory Fluids. J Exp Med (2003) 198: (7):977–85. doi: 10.1084/jem.20030382
37. Bondue B, Vosters O, de Nadai P, Glineur S, De Henau O, Luangsay S, et al. ChemR23 Dampens Lung Inflammation and Enhances Anti-viral Immunity in a Mouse Model of Acute Viral Pneumonia. PLoS Pathog (2011) 7(11):e1002358. doi: 10.1371/journal.ppat.1002358
38. Benjamin B, Valérie M, Parmentier B. Chemerin and its receptors in leukocyte trafficking, inflammation and metabolism. doi: 10.1016/j.cytogfr.2011.11.004
39. Kim JY, Xue K, Cao M, Wang Q, Liu JY, Leader A, et al. Chemerin suppresses ovarian follicular development and its potential involvement in follicular arrest in rats treated chronically with dihydrotestosterone. Endocrinology (2013) 154(8):2912–23. doi: 10.1210/en.2013-1001
40. Reverchon M, Cornuau M, Ramé C, Guerif F, Royère D, Dupont J. Chemerin inhibits IGF-1-induced progesterone and estradiol secretion in human granulosa cells. Hum Reprod (2012) 27(6):1790–800. doi: 10.1093/humrep/des089
41. Urbanek M, Legro RS, Driscoll DA, Azziz R, Ehrmann DA, Norman RJ, et al. Thirty-seven candidate genes for polycystic ovary syndrome: strongest evidence for linkage is with follistatin. Proc Natl Acad Sci USA (1999) 96(15):8573–8. doi: 10.1073/pnas.96.15.8573
42. Heinonen S, Korhonen S, Helisalmi S, Koivunen R, Tapanainen J, Hippeläinen M, et al. Associations between two single nucleotide polymorphisms in the adiponectin gene and polycystic ovary syndrome. Gynecol Endocrinol (2005) 21(3):165–9. doi: 10.1080/09513590500238796
43. Xita N, Georgiou I, Chatzikyriakidou A, Vounatsou M, Papassotiriou GP, Papassotiriou I, et al. Effect of adiponectin gene polymorphisms on circulating adiponectin and insulin resistance indexes in women with polycystic ovary syndrome. Clin Chem (2005) 51(2):416–23. doi: 10.1373/clinchem.2004.043109
44. Escobar-Morreale HF, Villuendas G, Botella-Carretero JI, Alvarez-Blasco F, Sanchón R, Luque-Ramírez M, et al. Adiponectin and resistin in PCOS: a clinical, biochemical and molecular genetic study. Hum Reprod (Oxford Engl) (2006) 21(9):2257–65. doi: 10.1093/humrep/del146
45. Tu X, Kuang Z, Gong X, Shi Y, Yu L, Shi H, et al. The Influence of LepR Tyrosine Site Mutations on Mouse Ovary Development and Related Gene Expression Changes. PLoS One (2015) 10(11):e0141800. doi: 10.1371/journal.pone.0141800
46. Urbanek M, Du Y, Silander K, Collins FS, Steppan CM, Strauss JF3, et al. Variation in resistin gene promoter not associated with polycystic ovary syndrome. Diabetes (2003) 52(1):214–7. doi: 10.2337/diabetes.52.1.214
47. Kunnari A, Ukkola O, Kesäniemi YA. Resistin polymorphisms are associated with cerebrovascular disease in Finnish Type 2 diabetic patients. Diabet Med (2005) 22(5):583–9. doi: 10.1111/j.1464-5491.2005.01480.x
48. Kohan L, Zarei A, Fallahi S, Tabiee O. Association between vaspin rs2236242 gene polymorphism and polycystic ovary syndrome risk. Gene (2014) 539(2):209–12. doi: 10.1016/j.gene.2014.01.078
49. Mangge H, Almer G, Truschnig-Wilders M, Schmidt A, Gasser R, Fuchs D. Inflammation, adiponectin, obesity and cardiovascular risk. Curr Med Chem (2010) 17(36):4511–20. doi: 10.2174/092986710794183006
50. Jakubowicz D, Wainstein J, Homburg R. The link between polycystic ovarian syndrome and type 2 diabetes: preventive and therapeutic approach in Israel. Isr Med Assoc J (2012) 14(7):442–7.
51. Osibogun O, Ogunmoroti O, Michos ED. Polycystic ovary syndrome and cardiometabolic risk: Opportunities for cardiovascular disease prevention. Trends Cardiovasc Med (2019) 30(7):399–404. doi: 10.1016/j.tcm.2019.08.010
52. Tarkun I, Dikmen E, Cetinarslan B, Cantürk Z. Impact of treatment with metformin on adipokines in patients with polycystic ovary syndrome. Eur Cytokine Netw (2010) 21(4):272–7. doi: 10.1684/ecn.2010.0217
53. Ozkaya M, Cakal E, Ustun Y, Engin-Ustun Y. Effect of metformin on serum visfatin levels in patients with polycystic ovary syndrome. Fertil Steril (2010) 93(3):880–4. doi: 10.1016/j.fertnstert.2008.10.058
54. Li M, Yang M, Zhou X, Fang X, Hu W, Zhu W, et al. Elevated Circulating Levels of Irisin and the Effect of Metformin Treatment in Women With Polycystic Ovary Syndrome. J Clin Endocrinol Metab (2015) 100(4):1485–93. doi: 10.1210/jc.2014-2544
55. Haider DG, Schaller G, Kapiotis S, Maier C, Luger A, Woltz M. The release of the adipocytokine visfatin is regulated by glucose and insulin. Diabetologia. (2006) 49(8):1909–14. doi: 10.1007/s00125-006-0303-7
Keywords: polycystic ovary syndrome, adipokine, systematic review, meta-analysis, non-obese
Citation: Lin K, Sun X, Wang X, Wang H and Chen X (2021) Circulating Adipokine Levels in Nonobese Women With Polycystic Ovary Syndrome and in Nonobese Control Women: A Systematic Review and Meta-Analysis. Front. Endocrinol. 11:537809. doi: 10.3389/fendo.2020.537809
Received: 20 September 2020; Accepted: 18 November 2020;
Published: 07 January 2021.
Edited by:
Pieter de Lange, University of Campania Luigi Vanvitelli, ItalyReviewed by:
Gloria Cerrone, University of Buenos Aires, ArgentinaStuart Maudsley, University of Antwerp, Belgium
Copyright © 2021 Lin, Sun, Wang, Wang and Chen. This is an open-access article distributed under the terms of the Creative Commons Attribution License (CC BY). The use, distribution or reproduction in other forums is permitted, provided the original author(s) and the copyright owner(s) are credited and that the original publication in this journal is cited, in accordance with accepted academic practice. No use, distribution or reproduction is permitted which does not comply with these terms.
*Correspondence: Xia Chen, chuangchi@163.com; Hanchu Wang, 76540557@qq.com
†These authors have contributed equally to this work