- 1Department of Kinesiology, Brock University, St. Catharines, ON, Canada
- 2Centre for Bone and Muscle Health, Brock University, St. Catharines, ON, Canada
- 3School of Kinesiology and Health Science, York University, Toronto, ON, Canada
Background: In adults, excess adiposity has been associated with low-grade, chronic inflammation and compromised bone health, but less is known about these linkages in children. The purpose of this study was to compare the circulating levels of inflammatory cytokines, adipokines, osteokines, and bone markers at rest and in response to plyometric exercise between obese and normal weight adolescent females.
Methods: Ten normal weight (BMI = 21.3 ± 2) and 10 obese (BMI = 32.9 ± 4), postmenarcheal females, aged 13–17 years, performed one bout of plyometric exercise (5 circuits; 120 jumps). Blood samples were taken at rest, 5 min, 1 h, and 24 h post-exercise. Tumor necrosis factor alpha (TNF-α), interleukin 6 (IL-6), insulin, leptin, osteocalcin, carboxy-terminal telopeptide (CTX), sclerostin, and parathyroid hormone (PTH) were measured in serum.
Results: Cytokines were not different between groups at rest or over time with IL-6 increasing (+31%; p = 0.04) 5 min post-exercise and TNF-α decreasing (-9%; p = 0.001) 1 h post-exercise. Insulin and leptin were higher in the obese compared to the normal weight females. In both groups, insulin significantly increased 5 min post-exercise but remained elevated 1 h post-exercise only in the obese group. Leptin did not change in response to exercise. Osteocalcin was lower in the obese group across time and increased (+12%; p = 0.02) 24 h post-exercise in both groups. CTX was similar between groups at rest and decreased (-24%; p < 0.001) 1 h post-exercise. Sclerostin was similar between groups at rest, but there was a significant interaction reflecting a significant increase (+29%; p = 0.04) 5 min post-exercise in the obese group and a non-significant decrease (-13%; p = 0.08) in normal weight controls. PTH increased 5 min post-exercise, dropped 1 h post-exercise to lower than pre-exercise, and returned to baseline 24 h post-exercise in both groups.
Conclusion: Obese adolescent females from our study had no evidence of resting inflammation or differences in bone resorption but show blunted bone formation when compared to normal weight controls. The direction and temporal changes in inflammatory cytokines, adipokines, and bone turnover markers to exercise were similar in both groups, reflecting an overall bone anabolic response for most biomarkers, except sclerostin, which increased only in the obese females immediately post-exercise, suggesting a different systemic regulation of sclerostin depending on adiposity.
Introduction
Previous studies have suggested that obesity is beneficial to bone due to the increased loading with higher body mass (1). However, there is likely a limit to such benefit, as individuals with over 33% body fat have a negative relationship with bone mineral density (BMD) (2), and overweight adolescent females appear to have lower BMD compared to normal weight females (3, 4). This impairment of the normal growth response of the skeleton (e.g., lower peak bone mass) due to excess adiposity also appears to increase the risk of fracture (5, 6). Thus, understanding the mechanisms that regulate adiposity and bone growth are important as they may aid in mitigating the risk of bone-related diseases and issues, such as osteopenia, osteoporosis, and fractures with increased age.
There are several mechanisms that can explain how obesity may impact bone. Obesity can lead to a state of low grade systemic inflammation, which is associated with increased circulating pro-inflammatory cytokines [e.g. tumor necrosis factor alpha (TNF-α), interleukin 6 (IL-6)] and adipokines (e.g., leptin) and an increase in pro-inflammatory macrophages within adipose tissue (7–9). This inflammation may perpetuate bone loss with aging or attenuate bone growth during adolescence, as higher circulating levels of pro-inflammatory cytokines (10, 11) and adipokines, such as leptin (12, 13), promote bone resorption. Also, obesity promotes the preferential increase in adipocyte formation, which may be at the cost of osteoblastogenesis, since these cells are derived from the same multi-potential mesenchymal stem cells (14). Bone may in turn impact adipose tissue through an endocrine function or molecular crosstalk. For example, in vivo ablation of osteocytes leads to a loss of white adipose tissue in murine models (15, 16), suggesting secretory factors from these bone cells (i.e., osteokines) are likely involved in adipose tissue regulation.
Sclerostin is an osteokine that may be implicated in this crosstalk. It is an osteocyte secreted factor that inhibits bone formation by inhibiting Wnt signaling. The Wnt signaling cascade is a critical pathway for the regulation of cell fate and function. Specifically, in bone, Wnt signaling promotes bone formation (17), while in adipose tissue it inhibits adipogenesis (18). Recently, studies have shown that sclerostin promotes adipogenesis in vitro (19), and regulates white and brown adipose tissue growth and development in murine models (20–23). In humans, serum sclerostin levels appear to be elevated in individuals with prediabetes and correlate with insulin resistance in skeletal muscle, liver, and adipose tissue (24). In adult females, circulating sclerostin is positively correlated with fat free mass (25). Additionally, systemic low grade inflammation may perpetuate bone loss by inducing the secretion of pro-inflammatory cytokines that are known to stimulate osteoclast activity and subsequent bone resorption (10, 11). Indeed, TNF-α has been shown to increase the expression of sclerostin, which indicates a coordinated control over bone by the induction of inflammation (26, 27). Taken together, these findings suggest a dynamic and coordinated communication between bone and adipose tissue that is likely regulated by inflammation, metabolism, energy demands or adiposity, and may involve the osteokine sclerostin.
Exercise can reduce adiposity and improve bone accrual in adolescence (28–30). Acutely, exercise has also been shown to increase bone formation and decrease bone resorption in both children and adults (31–34). In addition, sclerostin levels have been shown to increase following exercise in adults, while normal weight children show no response (35–38), suggesting children may be protected from sclerostin increasing post-exercise. Cytokine levels can also be altered both acutely and chronically by exercise, resulting in a net-improved, long term, pro-inflammatory profile (39–41). In fact, the acute inflammatory state post-exercise seems to aid, over time, the long-term beneficial muscle adaptations to exercise (42–44). This may be the case for bone and adipose tissue as well. We have previously demonstrated correlations between inflammatory cytokines and bone markers in normal weight young men following a bout of high intensity cycling (34). However, in female adolescents, no data exist examining linkages between the cytokine, adipokine and osteokine response with bone turnover, i.e., the balance between bone formation and bone resorption, either at rest or following exercise, and certainly no data exist examining this relationship in youth with obesity. Thus, the overall purpose of this study was to compare the serum levels of inflammatory cytokines, adipokines, osteokines and bone turnover markers at rest and in response to one bout of plyometric exercise in obese postmenarcheal adolescent females (ObAF) and normal weight postmenarcheal adolescent females (NwAF).
Materials and Methods
Participants
This study represents a secondary analysis that includes data from 20 adolescent females who participated in two larger studies conducted at our institution; half of the NwAF participants were from Dekker et al. (32) with the other half recruited later and all ObAF participants were from Josse et al. (45). Both studies and all procedures received ethical clearance from our University’s Biosciences Research Ethics Board. Further details from both these studies have been published elsewhere (32, 45). For the purpose of the current study, data from 10 NwAF (BMI < 85th percentile, 15.0 ± 1 years of age) and 10 ObAF (BMI ≥ 95th percentile, 14.7 ± 1 years of age), matched for age, menstrual status and somatic maturity, met the inclusion and matching criteria and were selected for the final analysis. Although there were more ObAF participants in the original study (45), we chose 10 of the ObAF participants with the highest BMI percentile who matched appropriately with the 10 available NwAF in all other concordant characteristics to balance the comparison. All participants were postmenarcheal and were tested during the early follicular phase (days 1–7) of their menstrual cycle. In addition, both groups had no previous or current fractures and did not use any pharmaceuticals or nutraceuticals/supplements that may affect bone. Baseline characteristics of the participants are presented in Table 1.
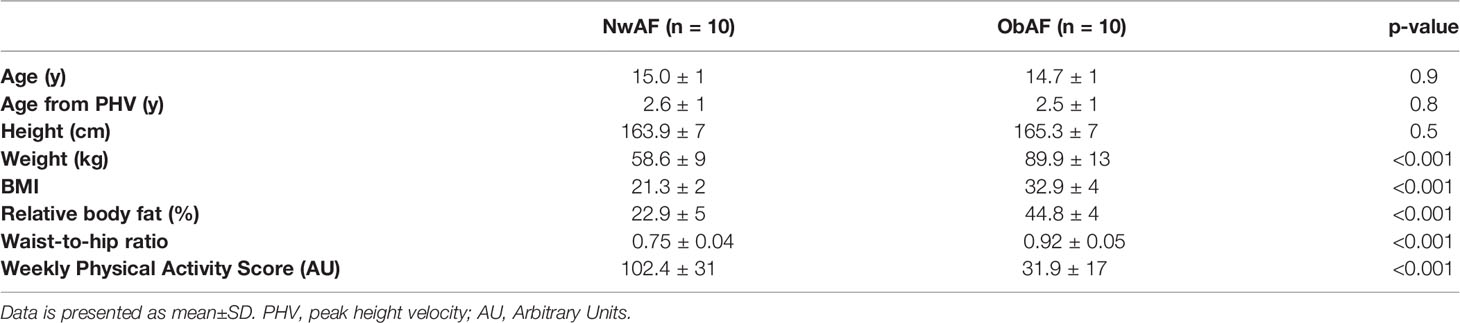
Table 1 Comparison of anthropometry, body composition and physical activity levels between normal weight and obese girls.
Study Protocol
Both studies followed the same protocol, which involved three sessions in the laboratory. The first session was to inform participants of the study including the risks and benefits, to have them view the laboratory and to obtain informed consent and assent from both the parents/guardians and the participant, respectively. The next two sessions were for testing and took place during the morning hours (between 0800 and 1000 h) to minimize any circadian rhythm variation in the serum biomarkers. For visits two and three, participants were asked to come to the laboratory fasted (~10–12h), and to avoid any vigorous or high-impact exercise for at least 24 h. Upon arrival to the laboratory, if requested, the topical anesthetic cream, Emla (25 mg/g lidocaine + 25 mg/g prilocaine), was applied to the antecubital fossa of the participants arm prior to blood sampling. Subsequently, height, weight and body composition were measured. Participants then sat down and rested for 10 min and had a resting/pre-exercise fasted venous blood sample, which was drawn using a standard venipuncture procedure using a 23G winged infusion set and vacutainers. Following the resting blood sample, participants were provided with a standardized light breakfast (1 granola bar, 1 banana, and water). The breakfast was consistent across both groups and was low in protein, since protein is known to influence markers of bone turnover (46, 47). Within 20 min, they began the 30 min plyometric exercise protocol followed by two more blood samples at 5 min and 1 h post-exercise. Between these two post-exercise blood samples, participants filled out the Godin Shephard Leisure-time physical activity questionnaire, which was used to calculate the weekly leisure activity score (arbitrary units) as previously described (48). Lastly, as participants were postmenarcheal, information regarding menstrual cycles and age of menarche were recorded. Participants returned to the laboratory for the third visit 24 h following the exercise protocol for the last blood sample.
Exercise Protocol
The plyometric exercise protocol was designed to provide high-impact, weight-bearing loads. It involved 120 jumps organized into five circuit training stations (three sets of eight repetitions, with 3 min of recovery between sets) (49). The five circuit stations included box, lunge, and tuck jumps, single leg hopping, and jumping jacks. The same protocol has been previously used in our laboratory and was successful in eliciting responses in bone biomarkers in pediatric populations (31, 32, 35, 38).
Participants began the exercise session with a warm-up that included 5 min of low-intensity cycling (~40W of resistance) on a cycle ergometer. Once the warm-up was completed, participants were given a comprehensive explanation and demonstration of each of the circuits. They were also allowed to familiarize themselves with each circuit to ensure proper technique and form to minimize injury. Jump height for box jumps was set at 40 cm for the NwAF and 25 cm for the ObAF. The height difference between the boxes was meant to control for differences in ground reaction forces between the two groups due to the obese girls having significantly higher body mass. Though the precise load required to elicit an osteogenic response in youth is still unknown, the jump height was adjusted for the adolescent females to reflect differences in weight (e.g., have comparable ground reaction forces) and to ensure safety. Each plyometric testing protocol was carried out by 2 research/study staff to ensure safety, and form/technique was watched closely to minimize any risk of injury. There were no adverse events because of the exercise protocol in this study.
Measurements
Height and seated height were measured with a stadiometer (Ellard Instruments, Monroe WA, USA) to the nearest 0.1 cm with no shoes and light clothes. These measures were then used to assess somatic maturity, expressed as years from age of peak height velocity (aPHV) as previously described (50). Body mass (kg) and body composition, including lean body mass (LBM), fat mass (FM), and relative body fat percent (%BF), were measured using bioelectrical impedance analysis (BIA; InBody520 bioelectrical impedance analysis system; Biospace Co. Inc. Los Angeles, CA, USA).
Venous blood was collected into serum separator vacutainers (cat#: 367983-1, BD, Mississauga, ON) and clotted for ~15 min before being centrifuged at ≤1400 RCF (g) for 15 min. The serum was separated and aliquoted into polyethylene tubes for storage at –80°C until analysis upon study completion. Immediately following each blood sample, hematocrit was measured to test for potential post-exercise hemoconcentration (shifts in plasma volume) using the microhematocrit method (51). Relative change in plasma volume (%ΔPV) from pre- to post-exercise was estimated using the Van Beaumont formula (51). No changes in plasma volume between pre- and post-exercise were observed, therefore unadjusted concentrations were presented.
Blood Biomarkers
All samples used for the purpose of this secondary analysis were reanalyzed together. Serum sclerostin was analyzed in duplicate using an enzyme linked Immunosorbent assay (ELISA; cat# DSST00; R&D, Minneapolis, MN). The average intra-assay coefficient of variation for sclerostin was 3.8%, and the inter-assay coefficient of variation was 5.1%. Osteocalcin, parathyroid hormone (PTH), insulin, leptin, IL-6 and TNF-α were analyzed in duplicate using Milliplex MAP human bone magnetic bead panels (HBNMAG-51K; EMD, Millipore Corporation, Etobicoke, ON). The average intra-assay coefficient of variations for IL-6, TNF-α, osteocalcin, PTH, insulin and leptin were 9.5, 5.0, 5.4, 7.9, 6.5, and 6.5%, respectively. The average inter-assay coefficient of variation for all analytes was 6.9%. β−isomerized Carboxy−terminal cross-linking telopeptides (CTX) (cat#: 11972308 122, β-CrossLaps) was measured from serum at the Mount Sinai Hospital Core Laboratory (Toronto, Ontario) using a Roche Cobas e602 Modular Analytics automated analyzer. Lower and upper detection limits were 0.010–6.00 ng/ml (quality control standard CV: 4.8%).
Statistical Analysis
Normality was confirmed using the Kolmogorov-Smirnov test, z-scores for skewness and kurtosis and visual screening of box plots. Unpaired/independent t-tests were used to assess physical characteristics and maturity between groups. A series of two-way repeated measures ANOVAs were used to assess time and group effects and time-by-group interactions for each biomarker and confidence intervals were adjusted based on Least Significant Difference (LSD), as all biomarkers did not pass Mauchly’s Test of Sphericity. In the event of a significant time-by-group interaction, further pairwise comparisons were made using one-way repeated measures ANOVAs for each group with Tukey’s multiple comparison test. Significance was accepted at an alpha level of <0.05 for all analyses. Statistical Analysis was performed using SPSS version 25 for Windows.
Results
As per the study design, there were no significant differences in age, height and somatic maturity between NwAF and ObAF, while ObAF had significantly higher body mass, BMI, %BF and waist-to-hip ratio, and lower weekly leisure activity score than NwAF (Table 1).
There was a significant main effect for time for IL-6, with no main effect for group or time-by-group interaction. Specifically, IL-6 increased 5 min following the plyometric protocol compared to pre-exercise [+0.6 pg/ml (+31%), p = 0.02], then returned to baseline 24 h post-exercise in both groups (Figure 1A). TNF-α also showed a significant main effect for time, but no significant main effect for group or time-by-group interaction. In both groups, TNF-α was significantly lower at 1h post-exercise compared to both pre-exercise [-0.16 pg/ml (-9%), p = 0.001] and 5 min post-exercise [-0.15 pg/ml (-8%), p = 0.001] concentrations (Figure 1B).
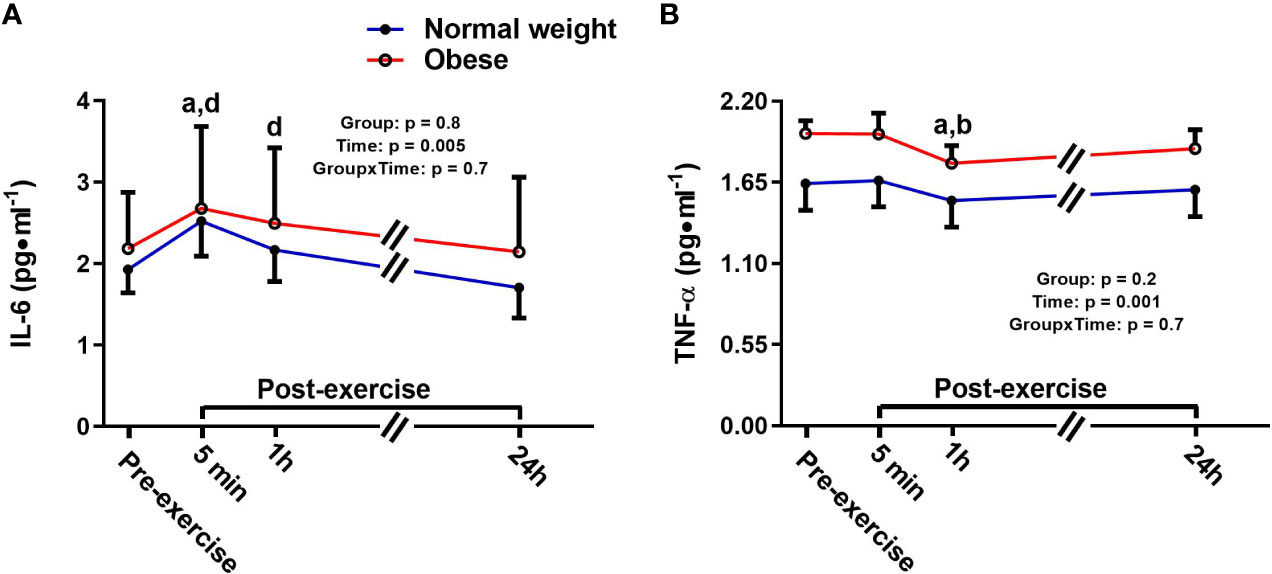
Figure 1 Serum concentrations (mean±SEM) of interleukin 6 (IL-6, A) and tumor necrosis factor alpha (TNF-α, B) pre- and post-exercise in normal weight (NwAF) and obese adolescent (ObAF) females. For post-hoc analysis for combined groups (black letters): a = significant difference from pre-exercise (p ≤ 0.05); b = significant difference from 5 min post-exercise (p ≤ 0.05); d = significant difference from 24 h post-exercise (p ≤ 0.05).
There were significant main effects for time and group, as well as a significant time-by-group interaction for insulin, indicating that the ObAF had higher insulin levels than NwAF across all tome points [mean difference: 787 pg/ml (174%), p = 0.03] and responded differently over time to exercise and feeding (Figure 2A). Insulin significantly increased from pre- to 5 min post-exercise [+345 pg/ml (+108%), p = 0.001] and returned to baseline 1h post-exercise in NwAF (Figure 2A). The ObAF also increased from pre- to post-exercise [+1,091 pg/ml (+129%), p = 0.02], but their levels remained elevated 1 h post-exercise and returned to baseline 24 h post-exercise, indicating the exercise and feeding-induced increase in insulin was higher and more prolonged in ObAF compared to NwAF (Figure 2A). There was a significant main effect for group for leptin, with the ObAF having significantly higher leptin levels than NwAF at all time points [mean difference: 13,579 pg/ml (+2.6-fold), p = 0.02] with no significant time effect and no significant interaction (Figure 2B).
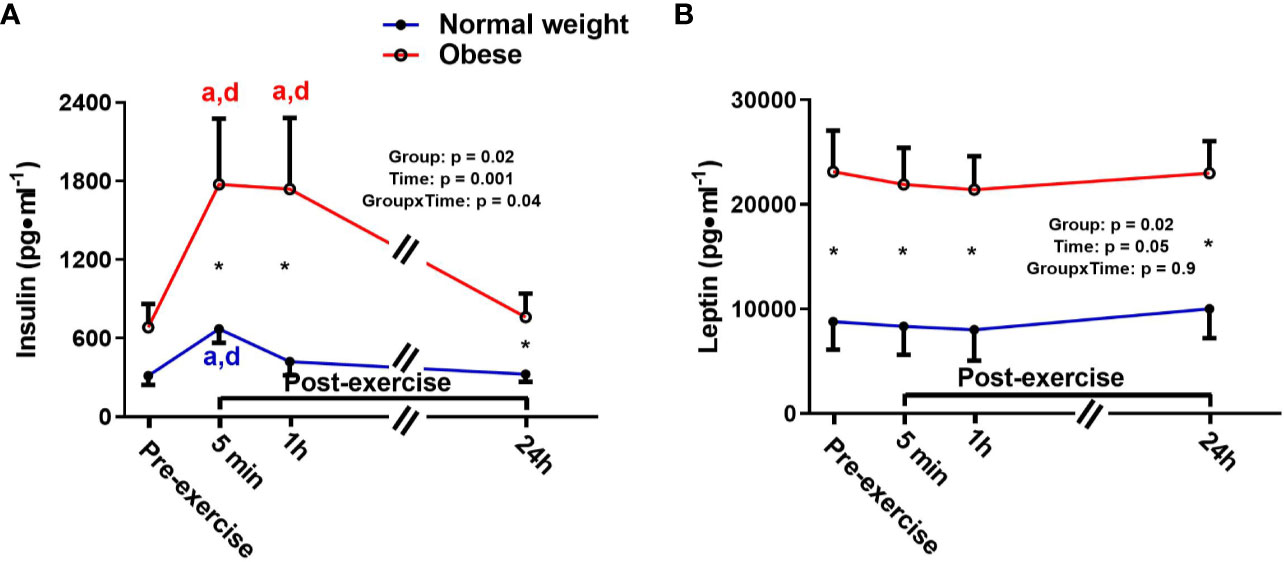
Figure 2 Serum concentrations (mean±SEM) of insulin (A) and leptin (B) pre- and post-exercise in normal weight (NwAF) and obese adolescent (ObAF) females. For post-hoc analysis within normal weight females (blue letters) and obese females (red letters): a = significant difference from pre-exercise (p ≤ 0.05); b = significant difference from 5 min post-exercise (p ≤ 0.05); c = significant difference from 1 h post-exercise (p ≤ 0.05); d = significant difference from 24 h post-exercise (p ≤ 0.05). *Significant mean difference between groups (p ≤ 0.05).
Osteocalcin showed a significant main effect for group and time, but no time-by-group interaction. The main time effect reflected a similar exercise induced increase in bone formation/turnover following plyometric exercise in both groups, and both groups had a progressive increase in osteocalcin after exercise, reaching a significant difference 24 h post-exercise compared to pre-exercise [+1,464.6 pg/ml (+12%), p = 0.04]. The main group effect demonstrated that the ObAF had significantly lower osteocalcin levels compared to NwAF [mean difference: 3,326 pg/ml (-23%), p = 0.04] (Figure 3A). CTX showed a significant main effect for time, but no group effect or time-by-group interaction, suggesting similar exercise-induced bone resorption changes between ObAF and NwAF. Compared to pre-exercise, CTX significantly decreased 5 min [-156 pg/ml (-19%), p < 0.001] and 1 h following the plyometric exercise protocol [-204 pg/ml (-24%), p < 0.001], then returned to baseline 24 h post-exercise in both groups (Figure 3B).
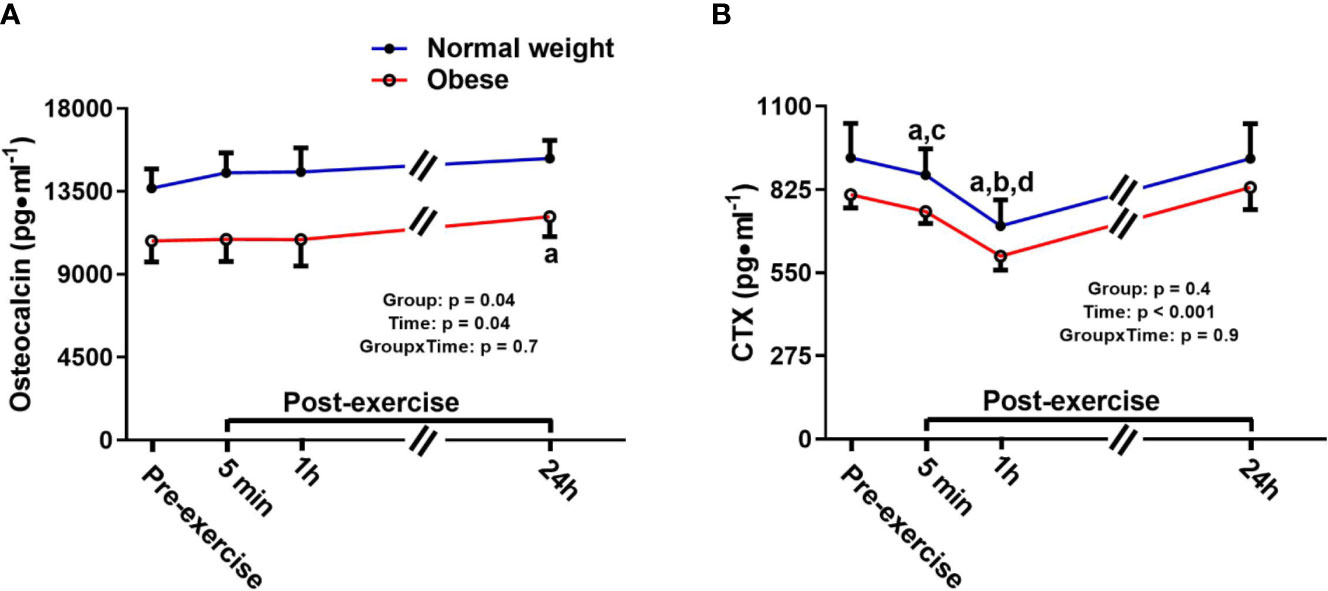
Figure 3 Serum concentrations (mean±SEM) of osteocalcin and CTX pre- and post-exercise in normal weight (NwAF) and obese adolescent (ObAF) females. For post-hoc analysis for combined groups (black letters): (A) significant difference from pre-exercise (p < 0.05); (B) significant difference from 5 min post-exercise (p ≤ 0.05); c = significant difference from 1 h post-exercise (p ≤ 0.05); d = significant difference from 24 h post-exercise (p ≤ 0.05). *Significant mean difference between groups (p ≤ 0.05).
There was a significant main effect for time and a significant time-by-group interaction for sclerostin, but no significant group effect (Figure 4). The interaction reflected that the groups responded differently to the exercise bout. Upon further inspection, this differential response was mostly driven by the ObAF that had a significant increase in sclerostin from pre- to 5 min post-exercise [+73 pg/ml (+26%), p = 0.04] that returned to baseline 1 h post-exercise, while the NwAF had no change in sclerostin immediately post-exercise (Figure 4). PTH showed a significant main effect for time, but no group effect or time-by-group interaction (Figure 5). Specifically, PTH levels significantly increased 5 min post-exercise compared to pre-exercise [+15.7 pg/ml (+38%), p = 0.05], dropped 1 h post-exercise to lower than pre-exercise [-14.8 pg/ml (-36%), p = 0.001], and returned to baseline 24 h post-exercise in both groups combined (Figure 5).
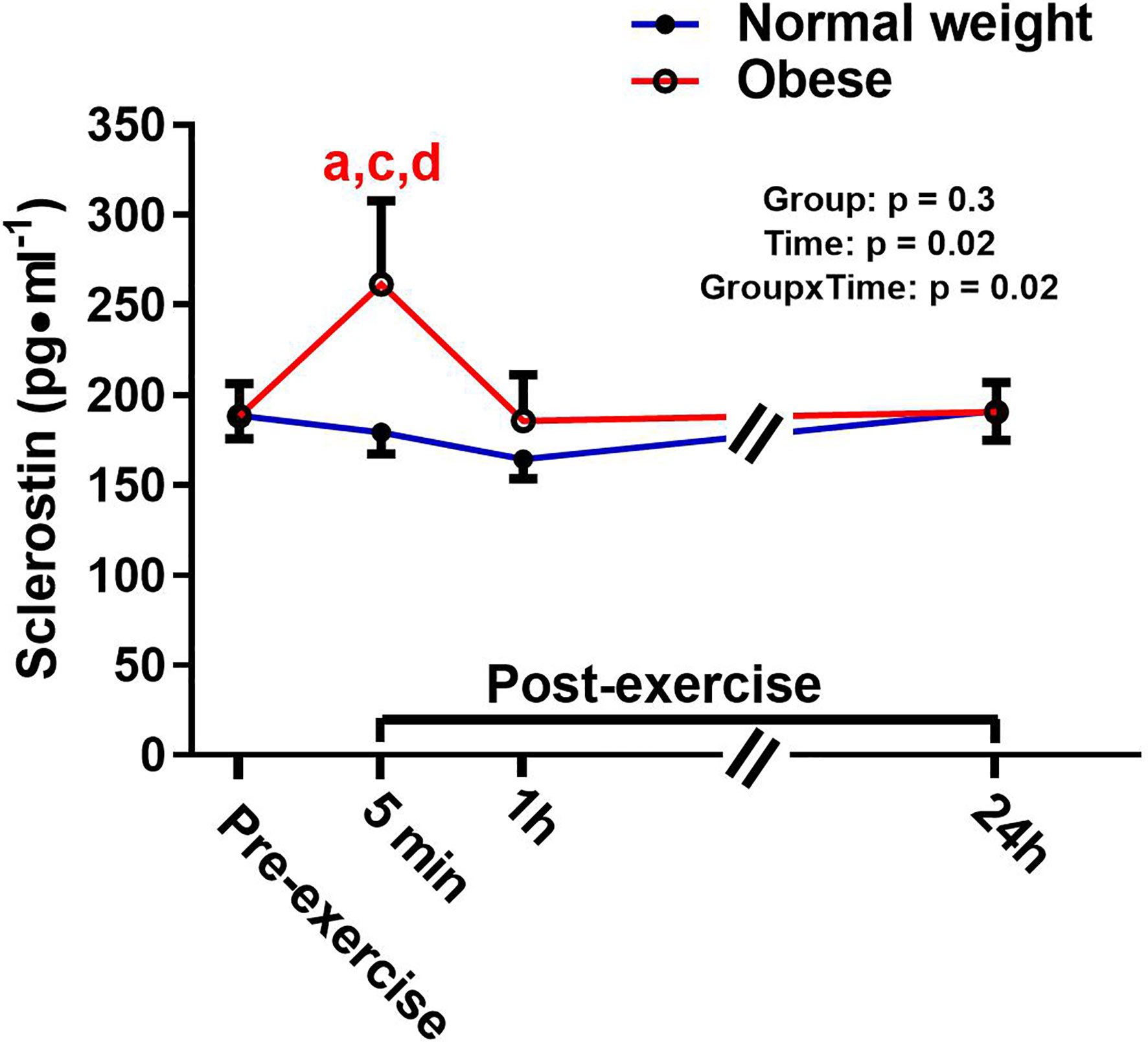
Figure 4 Serum concentrations (mean±SEM) of sclerostin pre- and post-exercise in normal weight (NwAF) and obese adolescent (ObAF) females. For post-hoc analysis within normal weight females (blue letters) and obese females (red letters): a = significant difference from pre-exercise (p ≤ 0.05); c = significant difference from 1 h post-exercise (p ≤ 0.05); d = significant difference from 24 h post-exercise (p ≤ 0.05).
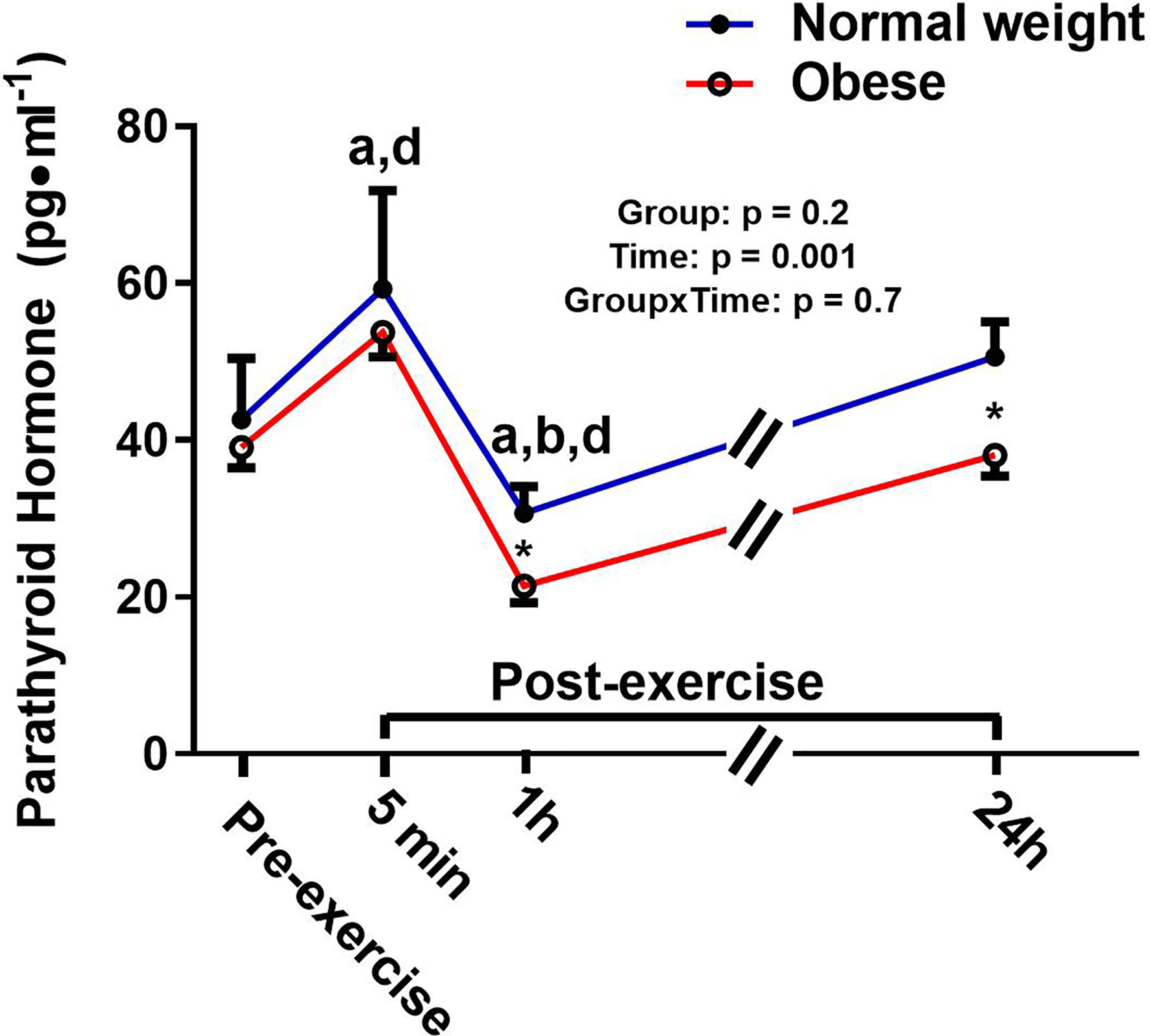
Figure 5 Serum concentrations (mean±SEM) of parathyroid hormone (PT) pre- and post-exercise in normal weight (NwAF) and obese adolescent (ObAF) females. For post-hoc analysis for combined groups (black letters): a = significant difference from pre-exercise (p ≤ 0.05); b = significant difference from 5 min post-exercise (p ≤ 0.05); d = significant difference from 24 h post-exercise (p ≤ 0.05). *Significant mean difference between groups (p ≤ 0.05).
Percent changes are presented in Supplementary Table 1 for all serum biomarker concentrations at 5 min, 1 h, and 24 h post-exercise relative to pre-exercise values within each group.
Discussion
This is the first study to compare the resting levels of serum osteokines, inflammatory cytokines, and adipokines and their responses to a single bout of high-impact, plyometric exercise in postmenarcheal adolescent females with normal weight (NwAF) and obesity (ObAF). There were three main findings from this study. First, there was no evidence of chronic low-grade inflammation in these ObAF. In addition, the similar cytokine response to exercise between groups suggests an exercise induced anti-inflammatory response that is intact even in the presence of excess adiposity. Second, compared to the NwAF, the ObAF had a significantly lower concentration of osteocalcin, both at rest and in response to acute exercise, suggesting that obesity during adolescence may blunt bone turnover, and thus prevent normal bone growth over time. Despite a global mean difference in osteocalcin levels and no difference in CTX, the response to plyometric exercise in these markers were similar between NwAF and ObAF, as both showed a transitory decrease in bone resorption (i.e., CTX) 1 h post-exercise and an increase in bone formation/turnover (i.e., osteocalcin) 24 h following plyometric exercise. Third, there was a differential response of sclerostin to exercise both directionally and temporally, as ObAF had an increase 5 min post-exercise while NwAF had no significant response.
Inflammatory Cytokines at Rest and in Response to Plyometric Exercise
There was no difference in the resting levels of IL-6 or TNF-α between NwAF and ObAF. These findings are in contrast to previous studies that have reported small, yet statistically significant, elevations in resting IL-6 and TNF-α levels in inactive 10–13 year old children with obesity compared to normal weight controls (52, 53). In contrast, when children with obesity were as active as children with healthy body fat levels, there were no differences in systemic inflammation (53). Our findings do not support this protective mechanism of inflammation with higher physical activity levels, since the ObAF reported significantly lower leisure time activity than the NwAF despite having similar cytokine profiles. In contrast, the ObAF may have surpassed a “threshold” of weekly physical activity that was sufficient to protect them despite their levels being significantly lower than the NwAF, as there levels appear to be similar to normal weight adults (54). Alternatively, our results may simply indicate that there was no detectable systemic low-grade inflammation in this cohort of ObAF independent of physical activity/exercise training. This is in line with the idea that overt systemic inflammation is not always detectable in children, but that an early, potentially local (e.g., in adipose depots), induction of inflammation in children with obesity may precede detectable levels within the circulation that are typically observed in adults with obesity (41, 55). The accumulation of (visceral) adipose tissue from childhood to young adulthood likely precedes the induction of chronic low-grade systemic inflammation seen more overtly in adult populations (56). Thus, our findings reinforce that adolescence is a critical time for intervening to reduce adiposity in hopes of preventing systemic inflammation and associated chronic diseases later in life (41, 44, 57).
There were no differences in the cytokine response to the acute plyometric exercise bout between ObAF and NwAF. Despite the significant differences in body mass and fat between the groups there was an identical transient response in IL-6 in direction and magnitude, which indicates that this type of exercise was sufficient at eliciting an IL-6 response to exercise in both groups. Although this has not been confirmed, the IL-6 increase post-exercise was likely secreted by the muscle and driven by changes in calcium, reactive oxygen species and/or substrate utilization [e.g., glycogen (58)] (56) in response to exercise. This implies that these mechanisms resulting in the release of IL-6 are still intact in adolescents with obesity. In contrast, the TNF-α response to plyometric exercise in our adolescent females was not similar to what is typically observed in adults. Specifically, TNF-α has been shown to increase significantly or remain unchanged post-exercise in adults (44, 59, 60). Here we report that TNF-α significantly decreased 1h post-exercise. While mechanistically this may make sense, given the increase in IL-6 is known to increase anti-inflammatory cytokines (e.g., IL-10) and reduce TNF-α production (39, 44, 55, 61), this small decrease (-5 and -9% from pre- to 1 h post exercise in NwAF and ObAF, respectively) may not be clinically relevant.
Leptin and Insulin at Rest and in Response to Plyometric Exercise
Leptin is an adipokine that acts to stimulate appetite and diminishes energy expenditure to maintain fat stores, thus playing a role in energy homeostasis (62). In this study, leptin; was significantly higher at all time points in the ObAF (consistent with their greater fat mass) compared to NwAF and unaffected by an acute bout of exercise in both groups. Exercise training induced reductions in fat mass are normally needed to see reductions in circulating leptin (63, 64). Moreover, elevated leptin levels with obesity are likely also a result of leptin resistance, causing its overproduction (65). Increased leptin levels are associated with insulin resistance, which, in turn, can lead to hyperinsulinemia (66). Indeed, we did find evidence of hyperinsulinemia post-exercise only in the ObAF (after consuming a high carbohydrate breakfast and exercising), which remained elevated for longer compared to NwAF. This indicates a potential impairment in postprandial glucose metabolism/handling in the ObAF. Leptin also has immune modulating effects (67), which include inducing the production of pro-inflammatory cytokine (e.g., TNF-α and IL-6), reactive oxygen species, and lymphopoiesis (65, 68–73). While there were no differences in circulating inflammatory cytokines between groups in this study, chronically elevated leptin may induce systemic inflammation that will be detectable over time (74). Thus, elevated leptin in youth may trigger local/tissue level inflammation that has not yet manifested as systemic inflammation, but still has adverse consequences.
Bone Turnover and Sclerostin at Rest and in Response to Exercise
NwAF had a significantly higher concentration of osteocalcin compared to ObAF at rest, suggesting that obesity during adolescence may blunt bone turnover and prevent normal bone growth over time (75). Despite this difference at baseline, the direction and magnitude of the response of these markers to plyometric exercise was similar between NwAF and ObAF, as both showed a transitory decrease in bone resorption (i.e., CTX) 1 h post-exercise and an increase in bone turnover/formation (i.e., osteocalcin) 24 h following high impact exercise. These findings are in line with previous studies that highlight a similar osteogenic response to acute exercise (31, 34) in male adults and children and a lower rate of bone turnover, and particularly lower bone formation, at rest in adolescents and young adults with obesity (5, 76). Taken together, it appears obesity in adolescence decreases bone turnover at rest, which may explain why children with obesity tend to present with an earlier achievement, and lower, peak height velocity, ultimately resulting in impaired linear growth (77). It is important to point out that our participants were matched for somatic maturity; hence, there was no significant difference in the age from peak height velocity between groups. Alternatively, the lower rate of bone turnover may affect bone quality. Studies have demonstrated that while adolescents with obesity tend to have increased bone mass, they also have an increased risk of fracture (78, 79) and a relatively lower bone mass for their weight (80). While there were differences in bone turnover at rest, the osteogenic bone turnover (i.e., CTX and OC) response to high-impact plyometric exercise was similar between groups. This suggests that higher impact loading exercise is beneficial for promoting positive bone turnover responses during this critical period for bone accrual (i.e. adolescence) (81) independent of adiposity or body mass.
There was a differential response in sclerostin post-exercise in the NwAF and ObAF. Specifically, NwAF had no change in sclerostin, while the ObAF showed a significant increase 5 min post-exercise which returned to baseline 1h post-exercise. The response in the NwAF was consistent with what we have previously reported in pre- and postmenarcheal females (32). Interestingly, the ObAF response was similar to what we have previously observed in adult females of normal weight (37). This finding supports previous reports that obesity in adolescence may promote advanced linear growth and skeletal maturation (i.e., “bone aging”) (82), which may have a negative impact on normal linear bone growth and development (77). Although we are unclear of what may be driving this differential response, the fact that adolescents with obesity have similar bone responses to adults following impact exercise warrants further investigation.
Research suggests that PTH and TNF-α may play a role in regulating sclerostin’s response to exercise since they have been shown to decrease (83, 84) and increase (27, 59) sclerostin expression, respectively. While both PTH and TNF-α fluctuated with exercise in this study, their responses were similar between the two groups, suggesting that PTH and/or TNF-α cannot explain the differential response of sclerostin post-exercise between our two groups. Alternatively, the exercise response in the ObAF may merely reflect higher sclerostin content within the bone microenvironment (e.g., canalicular-lacunar network) (35) that is released/secreted during mechanical loading (i.e., acute exercise). We initially hypothesized that there may be tissue crosstalk from adipose tissue to bone, in the form of inflammation, that would result in increasing sclerostin levels, both at rest and in response to exercise. This would be more apparent in the ObAF compared to NwAF. While we did not observe any differences in inflammation between ObAF and NwAF, there is an influence of obesity on the regulation of sclerostin post-exercise. This finding is intriguing given that humans who have mutations that inhibit Wnt signaling (i.e., sclerostin’s mechanism of action) show increased bone loss and alterations in the regulation of fat mass and energy balance (85, 86). Moreover, murine models highlight an important role of sclerostin in regulating adipose tissue metabolism and growth and development (20–23). This further points towards sclerostin as being an endocrine factor that likely plays a role in regulating tissues beyond bone (e.g., adipose tissue).
This study adds to a body of literature examining the effect of obesity on factors that regulate inflammation, bone turnover, and adiposity in adolescent females, which is an understudied population and a critical period of growth and development. While there were several novel findings in this study, there were also some limitations; first, the small sample size may have contributed to the lack of detection of significant differences post-exercise, particularly in inflammatory cytokines. Second, we did not measure all bone-related biomarkers, including, for example, blood calcium levels, which could have provided some insight regarding the fluctuations we observed in serum PTH. In addition, participants ate a small standardized breakfast before performing the exercise bout, which is known to impact some of the serum biomarkers we measured [e.g., IL-6, insulin, and bone turnover markers (CTX)]. There were also differences between groups in other variables such as habitual physical activity levels in addition to body mass and body composition (by design). While we believe that differences in adiposity are most responsible for driving these differences, it is unclear whether other differences between groups, such as habitual physical activity levels, may also have played a role. Lastly, the exercise mode used in this study may have provided small differences in mechanical loading between the groups. This was a result of the ObAF having a larger body mass and the NwAF having a higher vertical jump. While we tried to reduce the height of the box jumps for the ObAF compared to the NwAF to account for this, there still may have been small differences. Despite this potential difference, both groups responded similarly to the plyometric protocol, therefore we can assume both groups had a sufficient loading stimulus. Examination of the influence of longer-term exercise and dietary interventions (i.e. lifestyle modification) on the acute response of cytokines, osteokines and adipokines in pediatric populations should be done to see if these differences persist/change over time with interventions designed to reduce adiposity and improve fitness and overall health. Mechanistic studies should also be done (in animal models) to examine the importance of sclerostin to the exercise induced adaptations of adipose tissue.
Conclusion
This study found no detectable differences in inflammatory cytokines at rest or post-exercise between the adolescent females with normal weight and obesity. This study also provided evidence that a single bout of plyometric exercise can lead to an acute anti-inflammatory response post-exercise, as both ObAF and NwAF showed an increase in IL-6 at 5 min post-exercise and a decrease in TNF-α 1h post-exercise. There was also a steady decrease in CTX 1h post-exercise and a similar increase in osteocalcin 24 h post-exercise in both groups of adolescent females, suggesting an overall anabolic bone response to loading exercise in this population. Additionally, in the fasted rested state, osteocalcin was significantly lower in participants with obesity, indicating an overall lowering of bone turnover, which overtime may impact normal bone growth and development. Finally, we observed no difference in resting sclerostin levels, but there was a differential response post-exercise where the ObAF had a significant increase in sclerostin immediately post-exercise while the NwAF had no response. The impact of this differential regulation post-exercise is not known but is a cause for concern given sclerostin’s role in positively regulating adipogenesis and negatively regulating osteogenesis.
Data Availability Statement
The datasets generated for this study are available on request to the corresponding author.
Ethics Statement
The studies involving human participants were reviewed and approved by Brock University Research Ethics Board. Written informed consent to participate in this study was provided by the participants’ legal guardian/next of kin.
Author Contributions
NK contributed to data collection, performed the analysis of blood samples, completed the statistical analysis and prepared the first draft of the paper. KM and MC contributed to data collection and analysis. AJ contributed to the experimental design and the interpretation of the data. PK was the principal investigator of the research and contributed to the experimental design, data analysis and interpretation of the data. She is the corresponding author. All authors contributed to the article and approved the submitted version.
Funding
This study was funded by a Natural Sciences and Engineering Research Council of Canada (NSERC) grant to PK (grant # 2015-04424). This study was also partially funded by a Dairy Farmers of Canada grant to AJ and PK. NK holds an NSERC doctoral scholarship. Support for the open access publication fees was received through the Brock University Library Open Access Publishing Fund.
Conflict of Interest
The authors declare that the research was conducted in the absence of any commercial or financial relationships that could be construed as a potential conflict of interest.
Supplementary Material
The Supplementary Material for this article can be found online at: https://www.frontiersin.org/articles/10.3389/fendo.2020.531926/full#supplementary-material
References
1. Fassio A, Idolazzi L, Rossini M, Gatti D, Adami G, Giollo A, et al. The obesity paradox and osteoporosis. Eating weight Disord EWD (2018) 23(3):293–302. doi: 10.1007/s40519-018-0505-2
2. Liu P-Y, Ilich JZ, Brummel-Smith K, Ghosh S. New insight into fat, muscle and bone relationship in women: determining the threshold at which body fat assumes negative relationship with bone mineral density. Int J Prevent Med (2014) 5(11):1452–63.
3. Janicka A, Wren TA, Sanchez MM, Dorey F, Kim PS, Mittelman SD, et al. Fat mass is not beneficial to bone in adolescents and young adults. J Clin Endocrinol Metab (2007) 92(1):143–7. doi: 10.1210/jc.2006-0794
4. Pollock NK, Laing EM, Baile CA, Hamrick MW, Hall DB, Lewis RD. Is adiposity advantageous for bone strength? A peripheral quantitative computed tomography study in late adolescent females. Am J Clin Nutr (2007) 86(5):1530–8. doi: 10.1093/ajcn/86.5.1530
5. Dimitri P, Wales JK, Bishop N. Adipokines, bone-derived factors and bone turnover in obese children; evidence for altered fat-bone signalling resulting in reduced bone mass. Bone (2011) 48(2):189–96. doi: 10.1016/j.bone.2010.09.034
6. Dimitri P, Wales JK, Bishop N. Fat and bone in children: differential effects of obesity on bone size and mass according to fracture history. J Bone Miner Res (2010) 25(3):527–36. doi: 10.1359/jbmr.090823
7. Rasouli N, Kern PA. Adipocytokines and the metabolic complications of obesity. J Clin Endocrinol Metab (2008) 93(11 Suppl 1):S64–73. doi: 10.1210/jc.2008-1613
8. Wisse BE. The inflammatory syndrome: the role of adipose tissue cytokines in metabolic disorders linked to obesity. J Am Soc Nephrol (2004) 15(11):2792–800. doi: 10.1097/01.ASN.0000141966.69934.21
9. Saltiel AR, Olefsky JM. Inflammatory mechanisms linking obesity and metabolic disease. J Clin Invest (2017) 127(1):1–4. doi: 10.1172/JCI92035
10. Takayanagi H. Osteoimmunology: shared mechanisms and crosstalk between the immune and bone systems. Nat Rev Immunol (2007) 7(4):292–304. doi: 10.1038/nri2062
11. Khosla S. Minireview: the OPG/RANKL/RANK system. Endocrinology (2001) 142(12):5050–5. doi: 10.1210/endo.142.12.8536
12. Feresin RG, Johnson SA, Elam ML, Jo E, Arjmandi BH, Hakkak R. Effects of obesity on bone mass and quality in ovariectomized female Zucker rats. J Obes (2014) 2014:690123–3. doi: 10.1155/2014/690123
13. Caffarelli C, Alessi C, Nuti R, Gonnelli S. Divergent effects of obesity on fragility fractures. Clin Interventions Aging (2014) 9:1629–36. doi: 10.2147/CIA.S64625
14. Rosen CJ, Bouxsein ML. Mechanisms of disease: is osteoporosis the obesity of bone? Nat Clin Pract Rheumatol (2006) 2(1):35–43. doi: 10.1038/ncprheum0070
15. Sato M, Asada N, Kawano Y, Wakahashi K, Minagawa K, Kawano H, et al. Osteocytes regulate primary lymphoid organs and fat metabolism. Cell Metab (2013) 18(5):749–58. doi: 10.1016/j.cmet.2013.09.014
16. Sato M, Katayama Y. Osteocytes and Homeostasis of Remote Organs : Bone-Buried Osteocytes Talk to Remote Organs. Curr Osteoporos Rep (2015) 13(4):193–7. doi: 10.1007/s11914-015-0269-9
17. Poole KE, van Bezooijen RL, Loveridge N, Hamersma H, Papapoulos SE, Lowik CW, et al. Sclerostin is a delayed secreted product of osteocytes that inhibits bone formation. FASEB J (2005) 19(13):1842–4. doi: 10.1096/fj.05-4221fje
18. Chen N, Wang J. Wnt/β-Catenin Signaling and Obesity. Front Physiol (2018) 9:792–2. doi: 10.3389/fphys.2018.00792
19. Ukita M, Yamaguchi T, Ohata N, Tamura M. Sclerostin Enhances Adipocyte Differentiation in 3T3-L1 Cells. J Cell Biochem (2016) 117(6):1419–28. doi: 10.1002/jcb.25432
20. Kim SP, Da H, Li Z, Kushwaha P, Beil C, Mei L, et al. Lrp4 expression by adipocytes and osteoblasts differentially impacts sclerostin’s endocrine effects on body composition and glucose metabolism. J Biol Chem (2019) 294:6899–911. doi: 10.1074/jbc.RA118.006769
21. Kim SP, Frey JL, Li Z, Kushwaha P, Zoch ML, Tomlinson RE, et al. Sclerostin influences body composition by regulating catabolic and anabolic metabolism in adipocytes. Proc Natl Acad Sci U States America (2017) 114(52):E11238–47. doi: 10.1073/pnas.1707876115
22. Fulzele K, Lai F, Dedic C, Saini V, Uda Y, Shi C, et al. Osteocyte-Secreted Wnt Signaling Inhibitor Sclerostin Contributes to Beige Adipogenesis in Peripheral Fat Depots. J Bone Mineral Res (2017) 32(2):373–84. doi: 10.1002/jbmr.3001
23. Fairfield H, Falank C, Harris E, Demambro V, McDonald M, Pettitt JA, et al. The skeletal cell-derived molecule sclerostin drives bone marrow adipogenesis. J Cell Physiol (2018) 233(2):1156–67. doi: 10.1002/jcp.25976
24. Daniele G, Winnier D, Mari A, Bruder J, Fourcaudot M, Pengou Z, et al. Sclerostin and Insulin Resistance in Prediabetes: Evidence of a Cross Talk Between Bone and Glucose Metabolism. Diabetes Care (2015) 38(8):1509–17. doi: 10.2337/dc14-2989
25. Sheng Z, Tong D, Ou Y, Zhang H, Zhang Z, Li S, et al. Serum sclerostin levels were positively correlated with fat mass and bone mineral density in central south Chinese postmenopausal women. Clin Endocrinol (2012) 76(6):797–801. doi: 10.1111/j.1365-2265.2011.04315.x
26. Kim BJ, Bae SJ, Lee SY, Lee YS, Baek JE, Park SY, et al. TNF-alpha mediates the stimulation of sclerostin expression in an estrogen-deficient condition. Biochem Biophys Res Commun (2012) 424(1):170–5. doi: 10.1016/j.bbrc.2012.06.100
27. Baek K, Hwang HR, Park H-J, Kwon A, Qadir AS, Ko S-H, et al. TNF-α Upregulates Sclerostin Expression in Obese Mice Fed a High-Fat Diet. J Cell Physiol (2014) 229(5):640–50. doi: 10.1002/jcp.24487
28. Yuan Y, Chen X, Zhang L, Wu J, Guo J, Zou D, et al. The roles of exercise in bone remodeling and in prevention and treatment of osteoporosis. Prog Biophys Mol Biol (2016) 122(2):122–30. doi: 10.1016/j.pbiomolbio.2015.11.005
29. Goodman CA, Hornberger TA, Robling AG. Bone and skeletal muscle: Key players in mechanotransduction and potential overlapping mechanisms. Bone (2015) 80:24–36. doi: 10.1016/j.bone.2015.04.014
30. Rosa N, Simoes R, Magalhaes FD, Marques AT. From mechanical stimulus to bone formation: A review. Med Eng Phys (2015) 37(8):719–28. doi: 10.1016/j.medengphy.2015.05.015
31. Kish K, Mezil Y, Ward WE, Klentrou P, Falk B. Effects of plyometric exercise session on markers of bone turnover in boys and young men. Eur J Appl Physiol (2015) 115(10):2115–24. doi: 10.1007/s00421-015-3191-z
32. Dekker J, Nelson K, Kurgan N, Falk B, Josse A, Klentrou P. Wnt Signaling-Related Osteokines and Transforming Growth Factors Before and After a Single Bout of Plyometric Exercise in Child and Adolescent Females. Pediatr Exerc Sci (2017) 29(4):504–12. doi: 10.1123/pes.2017-0042
33. Sapir-Koren R, Livshits G. Osteocyte control of bone remodeling: is sclerostin a key molecular coordinator of the balanced bone resorption-formation cycles? Osteoporos Int (2014) 25(12):2685–700. doi: 10.1007/s00198-014-2808-0
34. Mezil YA, Allison D, Kish K, Ditor D, Ward WE, Tsiani E, et al. Response of Bone Turnover Markers and Cytokines to High-Intensity Low-Impact Exercise. Med Sci Sports Exerc (2015) 47(7):1495–502. doi: 10.1249/MSS.0000000000000555
35. Falk B, Haddad F, Klentrou P, Ward W, Kish K, Mezil Y, et al. Differential sclerostin and parathyroid hormone response to exercise in boys and men. Osteoporos Int (2016) 27(3):1245–9. doi: 10.1007/s00198-015-3310-z
36. Kouvelioti R, Kurgan N, Falk B, Ward WE, Josse AR, Klentrou P. Response of Sclerostin and Bone Turnover Markers to High Intensity Interval Exercise in Young Women: Does Impact Matter? BioMed Res Int (2018) 2018:4864952. doi: 10.1155/2018/4864952
37. Kouvelioti R, LeBlanc P, Falk B, Ward WE, Josse AR, Klentrou P. Effects of High-Intensity Interval Running Versus Cycling on Sclerostin, and Markers of Bone Turnover and Oxidative Stress in Young Men. Calcif Tissue Int (2019) 104(6):582–90. doi: 10.1007/s00223-019-00524-1
38. Klentrou P, Angrish K, Awadia N, Kurgan N, Kouvelioti R, Falk B. Wnt Signaling-Related Osteokines at Rest and Following Plyometric Exercise in Prepubertal and Early Pubertal Boys and Girls. Pediatr Exerc Sci (2018) 30(4):457–65. doi: 10.1123/pes.2017-0259
39. Ullum H, Haahr PM, Diamant M, Palmo J, Halkjaer-Kristensen J, Pedersen BK. Bicycle exercise enhances plasma IL-6 but does not change IL-1 alpha, IL-1 beta, IL-6, or TNF-alpha pre-mRNA in BMNC. J Appl Physiol (1985) 1994. 77(1):93–7. doi: 10.1152/jappl.1994.77.1.93
40. You T, Arsenis NC, Disanzo BL, Lamonte MJ. Effects of exercise training on chronic inflammation in obesity : current evidence and potential mechanisms. Sports Med (2013) 43(4):243–56. doi: 10.1007/s40279-013-0023-3
41. Gleeson M, Bishop NC, Stensel DJ, Lindley MR, Mastana SS, Nimmo MA. The anti-inflammatory effects of exercise: mechanisms and implications for the prevention and treatment of disease. Nat Rev Immunol (2011) 11(9):607–15. doi: 10.1038/nri3041
42. Fischer CP. Interleukin-6 in acute exercise and training: what is the biological relevance? Exerc Immunol Rev (2006) 12:6–33.
43. Pedersen BK, Fischer CP. Physiological roles of muscle-derived interleukin-6 in response to exercise. Curr Opin Clin Nutr Metab Care (2007) 10(3):265–71. doi: 10.1097/MCO.0b013e3280ebb5b3
44. Pedersen BK, Febbraio MA. Muscles, exercise and obesity: skeletal muscle as a secretory organ. Nat Rev Endocrinol (2012) 8(8):457–65. doi: 10.1038/nrendo.2012.49
45. Josse AR, Ludwa IA, Kouvelioti R, Calleja M, Falk B, Ward WE, et al. Dairy product intake decreases bone resorption following a 12-week diet and exercise intervention in overweight and obese adolescent girls. Pediatr Res (2020) 88:910–6. doi: 10.1038/s41390-020-0834-5
46. Shams-White MM, Chung M, Du M, Fu Z, Insogna KL, Karlsen MC, et al. Dietary protein and bone health: a systematic review and meta-analysis from the National Osteoporosis Foundation. Am J Clin Nutr (2017) 105(6):1528–43. doi: 10.3945/ajcn.116.145110
47. Bhattoa HP. Laboratory aspects and clinical utility of bone turnover markers. Ejifcc (2018) 29(2):117–28.
48. Godin G, Shephard RJ. A simple method to assess exercise behavior in the community. Canadian journal of applied sport sciences. J Canadien Des Sci Appliquees au Sport (1985) 10(3):141–6.
49. MacKelvie KJ, Khan KM, Petit MA, Janssen PA, McKay HA. A school-based exercise intervention elicits substantial bone health benefits: a 2-year randomized controlled trial in girls. Pediatrics (2003) 112(6 Pt 1):e447–7. doi: 10.1542/peds.112.6.e447
50. Mirwald RL, Baxter-Jones ADG, Bailey DA, Beunen GP. An assessment of maturity from anthropometric measurements. Med Sci Sports Exercise (2002) 34(4):689–94. doi: 10.1249/00005768-200204000-00020
51. Van Beaumont W. Evaluation of hemoconcentration from hematocrit measurements. J Appl Physiol (1972) 32(5):712–3. doi: 10.1152/jappl.1972.32.5.712
52. Reinehr T, Stoffel-Wagner B, Roth CL, Andler W. High-sensitive C-reactive protein, tumor necrosis factor α, and cardiovascular risk factors before and after weight loss in obese children. Metabolism (2005) 54(9):1155–61. doi: 10.1016/j.metabol.2005.03.022
53. Halle M, Korsten-Reck U, Wolfarth B, Berg A. Low-grade systemic inflammation in overweight children: impact of physical fitness. Exercise Immunol Rev (2004) 10:66–74.
54. Amireault S, Godin G. The Godin-Shephard Leisure-Time Physical Activity Questionnaire: Validity Evidence Supporting its Use for Classifying Healthy Adults into Active and Insufficiently Active Categories. Perceptual Motor Skills (2015) 120(2):604–22. doi: 10.2466/03.27.PMS.120v19x7
55. Petersen AMW, Pedersen BK. The anti-inflammatory effect of exercise. J Appl Physiol (2005) 98(4):1154–62. doi: 10.1152/japplphysiol.00164.2004
56. Pedersen BK. Muscle as a secretory organ. Compr Physiol (2013) 3(3):1337–62. doi: 10.1002/cphy.c120033
57. Booth FW, Roberts CK, Laye MJ. Lack of exercise is a major cause of chronic diseases. Compr Physiol (2012) 2(2):1143–211. doi: 10.1002/cphy.c110025
58. Starkie R, Ostrowski SR, Jauffred S, Febbraio M, Pedersen BK. Exercise and IL-6 infusion inhibit endotoxin-induced TNF-alpha production in humans. FASEB J Off Publ Fed Am Societies Exp Biol (2003) 17(8):884–6. doi: 10.1096/fj.02-0670fje
59. Kouvelioti R, Kurgan N, Falk B, Ward WE, Josse AR, Klentrou P. Cytokine and Sclerostin Response to High-Intensity Interval Running versus Cycling. Med Sci Sports Exerc (2019) 51(12):2458–64. doi: 10.1249/MSS.0000000000002076
60. Contrepois K, Wu S, Moneghetti KJ, Hornburg D, Ahadi S, Tsai M-S, et al. Molecular Choreography of Acute Exercise. Cell (2020) 181(5):1112–30.e16. doi: 10.1016/j.cell.2020.04.043
61. Ostrowski K, Rohde T, Zacho M, Asp S, Pedersen BK. Evidence that interleukin-6 is produced in human skeletal muscle during prolonged running. J Physiol (1998) 508( Pt 3):949–53. doi: 10.1111/j.1469-7793.1998.949bp.x
62. Pan WW, Myers MG. Leptin and the maintenance of elevated body weight. Nat Rev Neurosci (2018) 19(2):95–105. doi: 10.1038/nrn.2017.168
63. Bouassida A, Chamari K, Zaouali M, Feki Y, Zbidi A, Tabka Z. Review on leptin and adiponectin responses and adaptations to acute and chronic exercise. Br J Sports Med (2010) 44(9):620–30. doi: 10.1136/bjsm.2008.046151
64. Josse AR, Atkinson SA, Tarnopolsky MA, Phillips SM. Diets higher in dairy foods and dietary protein support bone health during diet- and exercise-induced weight loss in overweight and obese premenopausal women. J Clin Endocrinol Metab (2012) 97(1):251–60. doi: 10.1210/jc.2011-2165
65. Tilg H, Moschen AR. Adipocytokines: mediators linking adipose tissue, inflammation and immunity. Nat Rev Immunol (2006) 6(10):772–83. doi: 10.1038/nri1937
66. D’Elia L, Strazzullo P, Iacone R, Russo O, Galletti F. Leptin levels predict the development of insulin resistance in a sample of adult men-The Olivetti Heart Study. Nutr Metab Cardiovasc Dis (2019) 29(1):39–44. doi: 10.1016/j.numecd.2018.10.003
67. La Cava A, Matarese G. The weight of leptin in immunity. Nat Rev Immunol (2004) 4(5):371–9. doi: 10.1038/nri1350
68. Romeo J, Martinez-Gomez D, Diaz LE, Gómez-Martinez S, Marti A, Martin-Matillas M, et al. Changes in cardiometabolic risk factors, appetite-controlling hormones and cytokines after a treatment program in overweight adolescents: preliminary findings from the EVASYON study. Pediatr Diabetes (2011) 12(4 Pt 2):372–80. doi: 10.1111/j.1399-5448.2010.00753.x
69. Howard JK, Lord GM, Matarese G, Vendetti S, Ghatei MA, Ritter MA, et al. Leptin protects mice from starvation-induced lymphoid atrophy and increases thymic cellularity in ob/ob mice. J Clin Invest (1999) 104(8):1051–9. doi: 10.1172/JCI6762
70. Zhao T, Hou M, Xia M, Wang Q, Zhu H, Xiao Y, et al. Globular adiponectin decreases leptin-induced tumor necrosis factor-alpha expression by murine macrophages: involvement of cAMP-PKA and MAPK pathways. Cell Immunol (2005) 238(1):19–30. doi: 10.1016/j.cellimm.2005.12.002
71. Matarese G, Moschos S, Mantzoros CS. Leptin in immunology. J Immunol (Baltimore Md. 1950) (2005) 174(6):3137–42. doi: 10.4049/jimmunol.174.6.3137
72. Tian Z, Sun R, Wei H, Gao B. Impaired natural killer (NK) cell activity in leptin receptor deficient mice: leptin as a critical regulator in NK cell development and activation. Biochem Biophys Res Commun (2002) 298(3):297–302. doi: 10.1016/S0006-291X(02)02462-2
73. Gainsford T, Willson TA, Metcalf D, Handman E, McFarlane C, Ng A, et al. Leptin can induce proliferation, differentiation, and functional activation of hemopoietic cells. Proc Natl Acad Sci U States America (1996) 93(25):14564–8. doi: 10.1073/pnas.93.25.14564
74. Iikuni N, Lam QLK, Lu L, Matarese G, La Cava A. Leptin and Inflammation. Curr Immunol Rev (2008) 4(2):70–9. doi: 10.2174/157339508784325046
75. Russell M, Mendes N, Miller KK, Rosen CJ, Lee H, Klibanski A, et al. Visceral Fat Is a Negative Predictor of Bone Density Measures in Obese Adolescent Girls. J Clin Endocrinol Metab (2010) 95(3):1247–55. doi: 10.1210/jc.2009-1475
76. Viljakainen H, Ivaska KK, Paldánius P, Lipsanen-Nyman M, Saukkonen T, Pietiläinen KH, et al. Suppressed bone turnover in obesity: a link to energy metabolism? A case-control study. J Clin Endocrinol Metab (2014) 99(6):2155–63. doi: 10.1210/jc.2013-3097
77. De Leonibus C, Marcovecchio ML, Chiavaroli V, de Giorgis T, Chiarelli F, Mohn A. Timing of puberty and physical growth in obese children: a longitudinal study in boys and girls. Pediatr Obes (2014) 9(4):292–9. doi: 10.1111/j.2047-6310.2013.00176.x
78. Manias K, McCabe D, Bishop N. Fractures and recurrent fractures in children; varying effects of environmental factors as well as bone size and mass. Bone (2006) 39(3):652–7. doi: 10.1016/j.bone.2006.03.018
79. Fornari ED, Suszter M, Roocroft J, Bastrom T, Edmonds EW, Schlechter J. Childhood obesity as a risk factor for lateral condyle fractures over supracondylar humerus fractures. Clin Orthop Relat Res (2013) 471(4):1193–8. doi: 10.1007/s11999-012-2566-2
80. Goulding A, Taylor RW, Jones IE, McAuley KA, Manning PJ, Williams SM. Overweight and obese children have low bone mass and area for their weight. Int J Obes Relat Metab Disord (2000) 24(5):627–32. doi: 10.1038/sj.ijo.0801207
81. Weaver CM, Gordon CM, Janz KF, Kalkwarf HJ, Lappe JM, Lewis R, et al. The National Osteoporosis Foundation’s position statement on peak bone mass development and lifestyle factors: a systematic review and implementation recommendations. Osteoporos Int (2016) 27(4):1281–386. doi: 10.1007/s00198-015-3440-3
82. de Groot CJ, van den Berg A, Ballieux BEPB, Kroon HM, Rings EHHM, Wit JM, et al. Determinants of Advanced Bone Age in Childhood Obesity. Hormone Res Paediatr (2017) 87(4):254–63. doi: 10.1159/000467393
83. Bellido T, Ali AA, Gubrij I, Plotkin LI, Fu Q, O’Brien CA, et al. Chronic Elevation of Parathyroid Hormone in Mice Reduces Expression of Sclerostin by Osteocytes: A Novel Mechanism for Hormonal Control of Osteoblastogenesis. Endocrinology (2005) 146(11):4577–83. doi: 10.1210/en.2005-0239
84. Mirza FS, Padhi ID, Raisz LG, Lorenzo JA. Serum sclerostin levels negatively correlate with parathyroid hormone levels and free estrogen index in postmenopausal women. J Clin Endocrinol Metab (2010) 95(4):1991–7. doi: 10.1210/jc.2009-2283
85. Styrkarsdottir U, Thorleifsson G, Sulem P, Gudbjartsson DF, Sigurdsson A, Jonasdottir A, et al. Nonsense mutation in the LGR4 gene is associated with several human diseases and other traits. Nature (2013) 497(7450):517–20. doi: 10.1038/nature12124
Keywords: exercise, adolescent girls, obesity, inflammation, carboxy-terminal telopeptide, osteocalcin, parathyroid hormone, sclerostin
Citation: Kurgan N, McKee K, Calleja M, Josse AR and Klentrou P (2020) Cytokines, Adipokines, and Bone Markers at Rest and in Response to Plyometric Exercise in Obese vs Normal Weight Adolescent Females. Front. Endocrinol. 11:531926. doi: 10.3389/fendo.2020.531926
Received: 01 February 2020; Accepted: 12 November 2020;
Published: 11 December 2020.
Edited by:
Katherine Samaras, St Vincent’s Hospital Sydney, AustraliaReviewed by:
Monique Francois, University of Wollongong, AustraliaReina Villareal, Baylor College of Medicine, United States
Copyright © 2020 Kurgan, McKee, Calleja, Josse and Klentrou. This is an open-access article distributed under the terms of the Creative Commons Attribution License (CC BY). The use, distribution or reproduction in other forums is permitted, provided the original author(s) and the copyright owner(s) are credited and that the original publication in this journal is cited, in accordance with accepted academic practice. No use, distribution or reproduction is permitted which does not comply with these terms.
*Correspondence: Panagiota Klentrou, bmtsZW50cm91QGJyb2NrdS5jYQ==