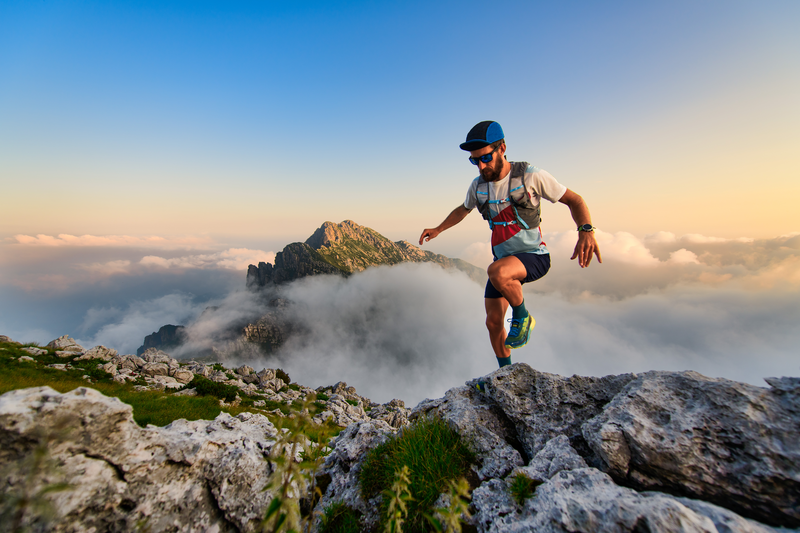
94% of researchers rate our articles as excellent or good
Learn more about the work of our research integrity team to safeguard the quality of each article we publish.
Find out more
REVIEW article
Front. Endocrinol. , 26 June 2020
Sec. Neuroendocrine Science
Volume 11 - 2020 | https://doi.org/10.3389/fendo.2020.00420
This article is part of the Research Topic Recent Progress and Perspectives in Neurosteroid Research View all 20 articles
Neural circuits in female rats sequentially exposed to estradiol and progesterone underlie so-called estrogen positive feedback that induce the surge release of pituitary luteinizing hormone (LH) leading to ovulation and luteinization of the corpus hemorrhagicum. It is now well-established that gonadotropin releasing hormone (GnRH) neurons express neither the reproductively critical estrogen receptor-α (ERα) nor classical progesterone receptor (PGR). Estradiol from developing ovarian follicles acts on ERα-expressing kisspeptin neurons in the rostral periventricular region of the third ventricle (RP3V) to induce PGR expression, and kisspeptin release. Circulating estradiol levels that induce positive feedback also induce neuroprogesterone (neuroP) synthesis in hypothalamic astrocytes. This local neuroP acts on kisspeptin neurons that express PGR to augment kisspeptin expression and release needed to stimulate GnRH release, triggering the LH surge. In vitro and in vivo studies demonstrate that neuroP signaling in kisspeptin neurons occurs through membrane PGR activation of Src family kinase (Src). This signaling cascade has been also implicated in PGR signaling in the arcuate nucleus of the hypothalamus, suggesting that Src may be a common mode of membrane PGR signaling. Sexual maturation requires that signaling between neuroP synthesizing astrocytes, kisspeptin and GnRH neurons be established. Prior to puberty, estradiol does not facilitate the synthesis of neuroP in hypothalamic astrocytes. During pubertal development, levels of membrane ERα increase in astrocytes coincident with an increase of PKA phosphorylation needed for neuroP synthesis. Currently, it is not clear whether these developmental changes occur in existing astrocytes or are due to a new population of astrocytes born during puberty. However, strong evidence suggests that it is the former. Blocking new cell addition during puberty attenuates the LH surge. Together these results demonstrate the importance of pubertal maturation involving hypothalamic astrocytes, estradiol-induced neuroP synthesis and membrane-initiated progesterone signaling for the CNS control of ovulation and reproduction.
Successful reproduction in female rodents depends on the interaction of steroidogenesis in the ovaries and brain. Almost 40 years ago Baulieu's group discovered that nervous tissue synthesizes steroids de novo from cholesterol and named them neurosteroids (1–4). Unraveling the physiology and actions of neurosteroids in the nervous system has been challenging because they are synthesized in specific locations, their actions must be differentiated from actions of circulating steroids, and in many cases the actions of peripheral steroids and neurosteroids are interdependent. Neurosteroids have been implicated in the myelination of peripheral nerves (5–8) neurogenesis (9) [reviewed in (10)], epilepsy, traumatic brain injury (11–13), and memory (14–18). Our research has concentrated on the role of the neurosteroid, neuroprogesterone (neuroP), which is synthesized de novo in hypothalamic astrocytes as part of the mechanism of estrogen positive feedback needed to stimulate the luteinizing hormone (LH) surge, inducing ovulation. This review considers estradiol signaling in the context of facilitating neuroP synthesis in astrocytes, and the integration of estradiol and neuroP signaling in regulating kisspeptin neurons in the rostral periventricular region of the third ventricle (RP3V). As with other steroid receptors, more recent findings indicate that in addition of nuclear localization and action, these receptors are trafficked to the plasma membrane where they are coupled to cell signaling cascades. The activation of nuclear progesterone receptor (PGR) at the cell membrane has recently been reviewed (19). In this review, we are primarily concerned with experimental evidence gathered in rodents. When appropriate, we indicate that the results were from different species. Kisspeptin is the most potent activator of neurons that release gonadotropin releasing hormone (GnRH) into the hypothalmo-hypophyseal portal circulation, generating a surge of pituitary LH into the systemic circulation. An LH surge is the trigger for ovulation and the formation of the corpus luteum—central events for reproduction.
Hormones of the hypothalamic-pituitary-gonadal axis coordinate events that lead to maturation of ovarian follicles. The pivotal event is the LH surge that induces ovulation and reprograms the ovary to produce large amounts of progesterone as well as estradiol. These ovarian hormones are necessary to: (i) facilitate female sexual receptivity to maximize the potential of fertilization, (ii) induce the secretory phase of the stratum functionale completing the preparation of the uterine endometrium for implantation of the zygote should fertilization occur, and (iii) supporting the initial stage of pregnancy until the placenta develops.
Orchestrated actions of estradiol, progesterone and kisspeptin in the brain are critical for triggering the LH surge. GnRH neurons of the diagonal band of Broca (DBB) and medial septum project to the median eminence and release GnRH into the hypothalamo-hypophyseal portal system. GnRH regulates the release of follicle stimulating hormone (FSH) and LH from gonadotrophin cells in the anterior pituitary. Differential regulation of LH and FSH is accomplished by changes in GnRH release: low frequency and amplitude favor FSH release, whereas elevated amplitude and frequency preferentially release LH. Within the ovary, gonadotropins are critical for maturation of follicles, which become dependent on their stimulation. LH acts on the thecal and granular cells of the ovarian follicles and later the corpora lutea to regulate estradiol and progesterone synthesis throughout the cycle. At the beginning of the estrous cycle (diestrus I and II) as ovarian follicles mature, circulating estradiol levels slowly rise and produce negative feedback in the hypothalamus and pituitary retarding the release of gonadotropins. The main effects of negative feedback regulating GnRH release appear to be mediated through kisspeptin, neurokinin B, and dynorphin expressing (KNDy) neurons of the arcuate nucleus of the hypothalamus (ARH) (20) [reviewed in (21, 22)]. The mechanism of estrogen positive feedback requires the action of estradiol and progesterone, and yet, GnRH neurons do not express ERα or classical PGR (23–25). Therefore, estradiol and progesterone must signal through neurons upstream of the GnRH neuron. The majority of anterior hypothalamic kisspeptin neurons express ERα and PGR, providing a platform for integrating steroid actions that modulate the excitation of GnRH neurons (26–28). In rodents, positive feedback actions of steroids are mediated by kisspeptin neurons in the RP3V, which contains the anteroventral periventricular (AVPV) and rostral periventricular zone (25, 29–33). The AVPV is a site critical for estrogen positive feedback signaling in rodents. Lesioning or implanting anti-estrogens into the AVPV blocks the LH surge (34–36).
GnRH neurons in the DBB receive input from RP3V (including AVPV) kisspeptin neurons and are activated by kisspeptin to increase the frequency and amplitude of GnRH release inducing an LH surge from the pituitary (23–27, 29–31, 37–39). Infusion of exogenous kisspeptin excites GnRH neurons and induces levels of LH that mimic surge levels. GnRH neurons in the DBB express Kiss1R (formerly GPR54), the cognate receptor for kisspeptin (40–42). GnRH neuronal activation and the LH surge are lacking in female Kiss1R knockout mice (37). Activation of Kiss1R in GnRH neurons produces robust depolarizing currents and induces GnRH release (43–47). The timing of the LH surge requires the stimulatory action of kisspeptin and the removal of RFamide-related peptide 3 (RFRP-3; also known as gonadotropin-inhibitory hormone—GnIH) (48–53). In this model, the daily afternoon increase in GnRH and LH is due to suppression of the RFRP-3 inhibitory input to the GnRH neurons by the suprachiasmatic nucleus (SCN). We propose that estrogen positive feedback surge release of LH requires an amalgamation of circadian and kisspeptin models. It is only when the diurnal release of RFRP-3 inhibition of GnRH coincides with estradiol and neuroP stimulation of kisspeptin release that a GnRH–LH surge occurs–once every 4 days during the estrous cycle (52).
As estradiol levels rise rapidly and peak on the afternoon of proestrus, positive feedback predominates (54). Because estradiol treatments induce the LH surge in ovariectomized and adrenalectomized (OVX/ADX) rats, progesterone was not thought to be required for the LH surge and the phenomenon was called “estrogen positive feedback” (55, 56). A large number of studies unequivocally demonstrated that in addition to estradiol, “estrogen” positive feedback requires PGR and progesterone (55, 57–64). It turned out that the needed progesterone, neuroP, is synthesized in the hypothalamus (32, 65–68). Rising estradiol levels during diestrus 1 to proestrus induce the expression of PGR and kisspeptin in RP3V neurons that are critical for the LH surge (26, 28, 33, 37, 63, 69–71). This initial kisspeptin induction is dependent on ERα (70, 71). In vivo experiments did not differentiate between effects of estradiol that induced PGR and kisspeptin since both require ERα. Moreover, in vivo experiments did not segregate estradiol effects directly on kisspeptin neurons from neuroP-PGR effects on kisspeptin neurons. Our in vitro experiments allowed us to tease apart these overlapping effects. Proestrous (positive feedback) levels of estradiol stimulate hypothalamic astrocytes to synthesize neuroP that acts on the estradiol-induced PGR in kisspeptin neurons, which augments the synthesis and release of kisspeptin needed for the GnRH-LH surge (19, 32, 33, 65, 68, 72) [reviewed in (73)]. Thus, a critical component of positive feedback is estrogen-facilitated neuroP signaling through ERα and PGR expressing kisspeptin neurons.
Depending on the final bioactive steroid, neurosteroidogenesis may involve one or a combination of astrocytes, oligodendrocytes, and neurons (74). This is because each cell type expresses certain enzymes within the steroidogenic pathway (74). The de novo synthesis of neurosteroids that are further down the pathway from cholesterol (e.g., estradiol) require shuttling through multiple cell types in order to be synthesized. However, neuroP only requires two enzymes to be synthesized from cholesterol, and astrocytes express both of these enzymes (4, 74) (Figure 1). The synthesis of progesterone is initiated by transport of cholesterol into the inner mitochondrial membrane through the interaction of translocator protein (TSPO) and steroid acute regulatory protein (StAR) (76–78) [but see (79–81)]. Cholesterol is converted to pregnenolone by the enzyme CYP11A1 (previously P450 side chain cleavage; P450scc) that is associated with the inner mitochondrial membrane. 3β-hydroxysteroid dehydrogenase (3β-HSD or HSD3B1) converts pregnenolone to progesterone, which diffuses out of astrocytes to activate local PGR-expressing kisspeptin neurons of the RP3V (19, 82) facilitating the LH surge.
Figure 1. Proposed estradiol-induced hypothalamic astrocyte steroidogenesis of neuroP that activates a membrane classical progesterone receptor (PGR)-Src tyrosine kinase (Src) signaling pathway in RP3V kisspeptin neurons to trigger the luteinizing (LH) surge. In hypothalamic astrocytes, proestrous levels of estradiol (E2) activate membrane estrogen receptor-α (mERα) that complex with and signal through metabotropic glutamate receptor-1a type (mGluR1a). mERα-mGluR1a signals through a PKC-IP3 pathway to increase intracellular calcium concentrations [(Ca2+)]. This releases Ca2+ from the smooth endoplasmic reticulum (sER). Within the mitochondrion, cholesterol (CHOL) is converted to pregnenolone (PREG) by P450 side chain cleavage (P450scc). PREG is then converted to neuroprogesterone (neuroP) by 3β-hydroxysteroid dehydrogenase (3β-HSD). The neuroP is secreted from the astrocytes to activate ERα-mediated, E2-induced PGR in RP3V kisspeptin neurons. Concurrently, E2 increases Kiss1 mRNA and kisspeptin expression via a mERα initiated mechanism [but see (70)]. neuroP rapidly augments the E2-induced Kiss1 mRNA and kisspeptin expression, potentially through PGR-Src signaling. PGR complexes with and signals through Src to activate a MAPK pathway. Further, a membrane PGR can initiate signaling that increases intracellular Ca2+ from sER stores. PGR-Src signaling also mediates the release of kisspeptin from neurons that project to diagonal band of Broca (DBB) GnRH neurons. Kisspeptin then binds to its cognate receptor, Kiss1R stimulating GnRH release into the median eminence that triggers the LH surge from gonadotrophs in the anterior pituitary (AP). Steroid acute regulatory protein (StAR), translocator protein (TSPO). Modified from Micevych et al. (75).
Positive feedback levels of estradiol induce neuroP synthesis in hypothalamic astrocytes. Proestrous levels of estradiol activate membrane ERα (mERα) that is complexed with and transactivates metabotropic glutamate receptor-1a (mGluR1a; Figure 1) to rapidly induce phosphorylation events that regulate cholesterol transport (65, 76, 83, 84). Estradiol activation of the mERα-mGluR1a complex, signaling through Gαq, activates the phospholipase C-inositol trisphosphate (IP3) signaling pathway that produces a robust release/increase of intracellular free calcium ([Ca2+]i) from intracellular stores (83, 85). This activates a calcium-sensitive adenylate cyclase-protein kinase A pathway that increases the phosphorylation of TSPO and StAR in hypothalamic astrocytes, which is necessary for inducing neuroP synthesis (83, 84, 86). These results suggest that proestrous levels of estradiol increase neuroP synthesis by increasing the cholesterol transport into mitochondria and access to P450scc for conversion to pregnenolone. In vivo, estradiol increases hypothalamic expression and activity of the second enzyme in the neuroP synthesis, 3β-HSD (85, 87). The estradiol-induced increase in brain progesterone levels are sex- and site-specific: present in the adult female hypothalamus but absent in the male hypothalamus (72). Moreover, blocking 3β-HSD activity in the hypothalamus of adult OVX/ADX rats prevents the estradiol-induced LH surge (72). We further demonstrated that neuroP is important for the LH surge in gonadally intact rats by blocking hypothalamic neuroP synthesis on the morning of proestrus by third ventricular (3V) administration of aminoglutethimide (AGT), a P450scc inhibitor (67). The estrous cycle is arrested in proestrus prior to the LH surge even though peripheral estradiol levels, a marker of ovarian steroidogenesis, are unaffected in AGT-treated rats. In these animals, the uterus is swollen with fluid and there are no corpora lutea in the ovaries—all indicating the absence of an LH surge (67). Thus, estradiol-induced hypothalamic neuroP, rather than ovarian or adrenal progesterone, mediates the triggering of the LH surge during positive feedback. Dose and duration of estradiol exposure during negative and positive feedback regulate the mechanisms of neuroP synthesis by astrocytes, and properly coordinate the timing of neuroP synthesis with the priming of the rest of the Kisspeptin-GnRH-LH system.
neuroP actions appear to be mediated through PGR signaling in RP3V kisspeptin neurons. Estradiol-induced RP3V PGRs are required to initiate and reach the full magnitude and duration of the LH surge (62, 88). Likewise, the LH surge cannot be induced in PGR knockout mice (89). Female mice with PGR knocked out specifically in kisspeptin neurons are less fertile (i.e., fewer births with smaller litters), and lack an estradiol-induced LH surge and the associated AVPV c-Fos induction (90, 91). Furthermore, activation of RP3V PGR with R5020 (PGR specific agonist) induced an LH surge in estradiol-primed rats (33). Accumulating evidence supports that it is neuroP signaling through PGR in kisspeptin neurons that is required for the LH surge. While blocking neuroP synthesis with a 3β-HSD inhibitor attenuates the estradiol-induced LH surge in OVX/ADX rats, progesterone treatment or site-specific injections of kisspeptin into the DBB rescued the LH surge, demonstrating that estradiol induction of neuroP synthesis and the actions of neuroP occur first and are required for kisspeptin release (32).
RP3V kisspeptin neurons are modeled in vitro using mHypoA51 cells that are derived from adult female hypothalamic kisspeptin neurons (19, 82). Estradiol induces PGR expression in these cells (19), which is observed in the RP3V where PGR expression is increased in areas that overlap with kisspeptin neurons by estradiol treatment in OVX rats or on proestrus (28, 33). Estradiol increases kisspeptin expression in mHypoA51 neurons, and subsequent progesterone further augments this expression (19). In co-culture experiments where mHypoA51 and adult female hypothalamic astrocytes are separated (i.e., not in direct contact) but share media, estradiol treatment induces neuroP synthesis in astrocytes and increases kisspeptin expression in the mHypoA51 neurons. neuroP secretion from astrocytes stimulates mHypoA51 neurons to rapidly increase kisspeptin release (19, 82). Importantly, mHypoA51 neurons express membrane progesterone receptors (mPR), including mPRα, mPRβ (see more below and Figure 2), and membrane-localized PGR. This membrane-localized PGR increases with estradiol treatment (19).
Figure 2. Modes of progesterone signaling in the rodent. Classical progesterone receptor (PGR) can mediate progesterone signaling classically (A), by binding to DNA progesterone response elements. PGR can also be trafficked to the plasma membrane [as in (B)] where it can activate rapid intracellular signaling cascades involving kinases such as Src. It is unknown whether membrane PGR transactivates another receptor like an mGluR as estrogen receptors have been shown to do. Multiple novel membrane progesterone receptors (mPRs) have been recently discovered and described, such as mPRs α, β, δ, and γ (C). mPRs can activate signaling cascades via G proteins, which go on to affect cyclic AMP (cAMP) pathways. Finally, progestins can bind to progesterone receptor membrane component 1 [PGRMC1 (D)]. PGRMC1 can work in concert with SERBP1 to affect cAMP, Jak/Stat, and multiple kinase pathways (73).
Although classified as a transcription factor and normally thought to be associated with the nucleus, PGR can be trafficked to the plasma membrane via palmitoylation, a mechanism seen in ERα trafficking (92). At the membrane, PGR can interact with and signal through other proteins to initiate rapid signaling, altering neuronal activity (33, 93, 94) (Figure 2). PGRs that are trafficked to the plasma membrane complex with and signal through Src kinase, a non-receptor tyrosine kinase (Src) (33, 93–95). PGRs have two distinct isoforms that are transcribed from a single gene: PGR-A and PGR-B. PGR-A lacks 164 amino acids in the N-domain, and is considered the truncated form of PGR-B (96). A poly-proline rich region (amino acids 421-428, PPPPLPPR) near the N-domain of PGRs is responsible for binding and signaling through the Src SH3 domain (93). Although this region is conserved in PGR-A, and both isoforms display hormone-dependent binding to SH3, only PGR-B activates Src (94). Significantly, PGR-B is also the reproductively relevant isoform.
In vitro and in vivo experiments indicate that neuroP signals through PGR-Src complexes to activate kisspeptin neurons, and that the PGR-Src signaling is interdependent. Nearly all mHypoA51 neurons express kisspeptin, and most express PGR and Src (19, 82). Activation of either PGR or Src in mHypoA51 neurons induces kisspeptin release while inhibiting Src activation blocks progesterone activation of MAPK and kisspeptin release, implying that progesterone and Src interact to stimulate kisspeptin release via activation of a MAPK pathway (Figure 1) (19). Another potential rapid PGR initiated pathway for kisspeptin release is through release on intracellular stores of [Ca2+] (Figure 1) (82). In mHypoA51 neurons, progesterone induced a rapid increase in [Ca2+] that was blocked by pretreatment with RU486, a PGR antagonist (82). However, further studies are required to determine the physiological outcomes of both of these signaling pathways. In vivo data further support that neuroP induces Src-mediated PGR signaling. PGR and Src are co-expressed in neurons of the RP3V of female rats (33). Further, using the Duolink proximity ligation assay that uses specific antibodies to two selected proteins/antigens and then produces punctate staining if these proteins are in close proximity (<40 nm), we observed that estradiol-priming increases the levels of PGR and Src staining in RP3V neurons suggestive of an estradiol-induced increase in PGR-Src interactions (33). Similarly, in the ARH, a region important for facilitation of lordosis, we have observed a similar colocalization and estradiol-induced increase in PGR-Src proximity (97). In the RP3V, PGR and Src exhibit interdependent signaling in the induction of the LH surge. Bilateral infusion of either a classical PGR agonist (R5020) or Src family activator induced a robust LH surge in estradiol-primed OVX/ADX rats (97). However, bilateral RP3V infusion of either a PGR antagonist (RU486) or a Src inhibitor (PP2) blocked the induction of the LH surge by activation of either PGR (progesterone or R5020) or Src (Src activator). The ability of antagonizing either PGR or Src to block the signaling of both PGR and Src indicates that PGR-Src signaling is interdependent. It is likely that PGR is transactivating Src to initiate signaling. Even though Src is “downstream” of PGR, and activation of either one will induce the LH surge, for signaling to occur neither can be occupied by an antagonist, which likely produces a conformational change that prevents Src activation and signaling. This antagonist effect was also seen with interactions of PGR and dopamine receptors (98). However, in the absence of antagonist binding either PGR or Src, it appears the activation of either PGR or Src can initiate the signaling cascade. The similarities of the PGR-Src signaling cascade in the ARH, RP3V and mHypoA51 neurons suggests that PGR-Src signaling may be a common mode of membrane PGR signaling. Together, the in vivo and in vitro findings indicate that membrane PGR-Src signaling mediates the neuro P activation of kisspeptin neurons to activate GnRH neurons to trigger the LH surge.
Although PGRs are essential to induce the LH surge, other types of mPRs have been proposed to modulate neuroP/progesterone actions through membrane initiated signaling [reviewed in (73); see Figure 2]. For example, during progesterone negative feedback, PGR knockout mice respond to positive feedback levels of progesterone to suppress GnRH release, suggesting that progesterone may also signal through non-classical mPRs (99). However, the role of non-classical mPR in the LH surge remains unknown (19, 100). Two families of mPRs have been discovered that initiate progesterone signaling at the plasma membrane. One group of these mPRs is in the Class II progestin and adipoQ receptor (PAQR) family (101–103). These mPRs have a classic 7-transmembrane protein structure and behave similarly to G protein-coupled receptors by rapidly facilitating progesterone action. In vitro, mHypoA51a neurons express subtypes of mPRs: mPRα and mPRβ (19). In vivo, estradiol upregulates mPRβ expression in the anterior hypothalamus (104). The distribution and estrogen regulation of mPRβ in the female rat brain (104), but not in mHypoA51 neurons (19), suggests that this in vivo upregulation occurs in non-kisspeptin cells. Although these mPRs are expressed in the RP3V, little is known about the role of these non-classical mPRs in regulating the neuroP induction of the LH surge. Another protein that binds progesterone and initiates signaling at the plasma membrane is progesterone receptor membrane components (PGRMC) (105–109). Two PGRMC subtypes have been discovered: PGRMC1 (aka 25-DX) and PGRMC2 (105, 106, 108, 110, 111). These PGRMC have been implicated in normal mammalian ovarian function including primordial follicle development, luteal vascularization and normal onset reproductive senescence (107, 110, 112). Young women with reduced PGRMC2 expression in granulosa cells have been diagnosed with diminished ovarian reserve (113). Similarly, reduced expression of PGRMC1 (via point mutation) has been associated with women exhibiting primary ovarian insufficiency (114). PGRMC mRNAs are expressed in the AVPV (109, 111, 115). However, only PGRMC2 mRNA levels are upregulated by the sequential treatment with estradiol and progesterone (115). Although their expression in the AVPV does not appear to be essential, mPRs and PGRMC's may influence PGR actions. A resolution of this issue requires further experimentation.
Maturation of reproductive circuits in females that results in ovulation involves a multitude of changes in the brain during puberty. This is represented by the increase in GnRH pulsatility throughout puberty, which is needed for the surge release of LH [reviewed in (116)]. As with estrogen positive feedback and the facilitation of neuroP synthesis, kisspeptin neurons in the rodent AVPV are sexually dimorphic; females have more kisspeptin neurons in the AVPV compared with males (30). In female mice, the number of presumptive kisspeptin neurons in the AVPV increases across pubertal development (117). The increase in GnRH pulsatility and the increase in the number of kisspeptin neurons are crucial to the development of estrogen positive feedback, but the maturation of these two systems do not fully explain the development of estrogen positive feedback during puberty.
Much like the rest of the system that controls estrogen positive feedback, the ability of the hypothalamus to coordinate estradiol-induced neuroP synthesis is something that develops across puberty in the rodent. It was previously observed that primary hypothalamic astrocyte cultures did not increase progesterone synthesis in response to estradiol if harvested from neonatal female or male mice of any age, and maturation in vitro did not make these astrocytes respond to estradiol with neuroP synthesis (65). At the time it was thought that something about the pubertal transition made astrocytes competent to respond to estradiol, but this idea was not formally tested until recently. Mohr et al., showed that estradiol-facilitated neuroP synthesis in the hypothalamus develops during puberty in the female rat (118). First, hypothalamic tissues, collected from gonadally intact rats either on postnatal day 17, (PND 17; prepuberty), PND 35, (peripuberty), or on the afternoon of proestrus around PND 60 (adulthood), were assayed for neuroP levels with liquid chromatography tandem mass spectrometry (LC-MS/MS). NeuroP significantly increases during puberty in gonad-intact female rats, from prepuberty to adulthood. Then, in OVX rats of the same ages, estradiol treatment only in adulthood reliably facilitates neuroP synthesis. The prepubertal female hypothalamus is insensitive to estradiol in terms of neuroP synthesis. However, during puberty (peripuberty) the ability of estradiol to stimulate neuroP synthesis develops. The pubertal development of neuroP synthesis is yet another way that the brain changes during puberty to allow for estrogen positive feedback signaling.
Adult female hypothalamic astrocytes are the source of estradiol-facilitated neuroP synthesis that contributes to estrogen positive feedback (68). Corresponding to the pubertal increase in estradiol-facilitated neuroP synthesis in vivo, there is an increase in the amount of mERα in hypothalamic astrocyte cultures. In these primary female astrocyte cultures, there is also an increase in caveolin-1 protein, a scaffolding protein that participates in the trafficking of ERα to the cell membrane and coupling with mGluR1a (118). Because membrane-initiated estradiol signaling is necessary to augment neuroP synthesis in astrocytes in adulthood [reviewed in (119)], the lack of mERα provides an explanation as to why pre-pubertal hypothalamic astrocytes are incapable of estradiol-induced neuroP synthesis. It appears that pubertal expression of caveolin-1 that shepherds ERα to the membrane may be key to the development of estrogen positive feedback that induces neuroP synthesis to trigger the LH surge.
Another explanation of how estradiol-induced neuroP synthesis develops in the hypothalamus is the “new astrocyte model” (Figure 3). Accordingly, estradiol-responsive astrocytes are not present in the prepubertal hypothalamus. During and after puberty, populations of new cells are added to the female rat AVPV, and a large majority of these newly born cells express markers for astrocytes (GFAP) (120, 121). These newborn AVPV cells are more numerous in females compared with males, and this sex difference in pubertal cell addition is dependent on gonadal hormones (122). Pubertal cell addition to the AVPV mirrors the overall sex difference observed in the rodent AVPV, considering that the female AVPV is larger and contains more neurons in females compared with males (123). This sex difference in structure likely contributes to the functional sex difference of this brain region, because only female rodents are capable of estrogen positive feedback (124). That females have higher amounts of cells added to the AVPV compared with males during peripuberty may indicate that these newborn cells are needed for estrogen positive feedback signaling.
Figure 3. The new astrocyte model for the pubertal development of rodent estrogen positive feedback. Prior to puberty, kisspeptin expression is low in the female AVPV (green neuron), and mERα expression (maroon diamond) in hypothalamic astrocytes (dark blue stars) is low. The LH surge does not occur naturally and cannot be induced with exogenous estradiol administration. After puberty occurs and once estrogen signaling begins, estrogen-responsive newborn astrocytes are added to the AVPV (light blue stars), which are necessary for the LH surge in adulthood. There is also an increase in mERα expression in hypothalamic astrocytes, and estradiol-induced neuroP synthesis occurs, which acts on membrane-associated PGR (orange oval) to stimulate kisspeptin release. Together, the changes in cellular machinery across pubertal development alter estradiol responsiveness and permit the LH surge to occur, indicating that reproductive maturation is complete.
Indeed, these newly born cells are crucially important for estrogen positive feedback induction of the LH surge. When cell proliferation is blocked with cytrabine (AraC), a pyrimidine analog, either during puberty or in early adulthood, the estradiol + progesterone-induced LH surge is diminished in female rats (120). A majority of these newborn cells are astrocytes, suggesting that these newborn AVPV astrocytes are the source of estradiol-responsive hypothalamic astrocytes that synthesize neuroP necessary for estrogen positive feedback. In the 2017 study by Mohr et al., both estradiol and progesterone were used to elicit the LH surge (120). In this study, the LH surge was not eliminated entirely, which may be explained by several factors: AraC did not eliminate all newborn AVPV cells, and therefore, some neuroP-producing astrocytes were present to produce neuroP and elicit some LH release, or more likely, administration of progesterone on the morning of the day of the surge bypassed hypothalamic neuroP, eliciting some LH release. Had only estrogen been used, which can also elicit the LH surge in rats (72), the effect of AraC may have been more dramatic on the LH surge because the only source of progesterone would have been from hypothalamic astrocytes (neuroP).
More studies are required to determine the exact role of pubertally born astrocytes in the development of estrogen positive feedback signaling. However, it seems likely that the birth of astrocytes contributes to the maturation of reproductive circuits controlling estrogen positive feedback signaling. There may be a developmental difference in the birth and maturation of astrocytes if they are born while circulating estradiol is elevated (i.e., after puberty compared with before puberty) that makes them competent to respond to estradiol with neuroP synthesis. These newborn astrocytes could have higher levels of mERα, and caveolin-1, resulting in increased PKA phosphorylation, making them more proficient in estradiol-induced neuroP synthesis.
The regulation of ovulation is the central event in mammalian reproduction and during puberty. Indeed, neural circuits controlling reproduction in females are considered mature when ovulation can occur. In rodents, at the very least, this critical physiological process requires the coordination of the hypothalamo-pituitary-ovarian axis with the SCN circadian clock. Regulation of the surge release of LH requires a complex neuronal and glial circuitry that directs various peripheral and central hormones onto kisspeptin neurons. In turn, circadian-regulated inputs interact with GnRH neurons, activating the anterior pituitary to release a surge of LH. This mechanism has been dubbed “estrogen positive feedback” for the importance of estrogen, but it is far from the only critical hormonal participant in this process. Developing ovarian follicles synthesize ever increasing levels of estradiol that induce PGR expression in kisspeptin neurons. As estradiol levels peak on the afternoon of proestrus, neuroP synthesis is rapidly facilitated in hypothalamic astrocytes, many of which may be born after the initiation of puberty. Together, estradiol and neuroP stimulate kisspeptin expression and release. When this hormonal activation of kisspeptin coincides with the release of circadian inhibition–a physiological LH surge occurs (i.e., one that stimulates ovulation). We now understand the signaling involved in regulating both the synthesis of neuroP in astrocytes and the neuroP signaling in kisspeptin neurons. In astrocytes, mERα transactivates mGluR1a to induce neuroP synthesis. In kisspeptin neurons, a portion of estradiol-induced PGR are trafficked to the cell membrane where neuroP activates them, augmenting both kisspeptin expression and release. NeuroP signaling in kisspeptin neurons involves Src activation and the release of intracellular calcium. Thus, the brain does not passively respond to ovarian hormones but is an active participant in triggering the LH surge to induce ovulation.
All authors contributed to the writing and editing of this manuscript.
Research was supported by NIH grants HD042635 (PM), HD058638 (KS), 5R25GM071638 (NIH RISE – CSULB), NIH grants HD04612, and HD097965 (MM).
The authors declare that the research was conducted in the absence of any commercial or financial relationships that could be construed as a potential conflict of interest.
We thank Timbora Chuon for his technical support.
1. Baulieu EE. Steroid hormones in the brain: several mechanisms? In: Fuxe K, Gustafsson JA, editors. Steroid Hormone Regulation of the Brain. Oxford: Pergamon (1981). p. 3–14. doi: 10.1016/B978-0-08-026864-4.50007-4
2. Baulieu EE. Neurosteroids: a new function in the brain. Biol Cell. (1991) 71:3–10. doi: 10.1016/0248-4900(91)90045-O
3. Baulieu EE. Neurosteroids: a novel function of the brain. Psychoneuroendocrinology. (1998) 23:963–87. doi: 10.1016/S0306-4530(98)00071-7
4. Corpechot C, Young J, Calvel M, Wehrey C, Veltz JN, Touyer G, et al. Neurosteroids: 3 alpha-hydroxy-5 alpha-pregnan-20-one and its precursors in the brain, plasma, and steroidogenic glands of male and female rats. Endocrinology. (1993) 133:1003–9. doi: 10.1210/endo.133.3.8365352
5. Schumacher M, Akwa Y, Guennoun R, Robert F, Labombarda F, Desarnaud F, et al. Steroid synthesis and metabolism in the nervous system: trophic and protective effects. J Neurocytol. (2000) 29:307–26. doi: 10.1023/A:1007152904926
6. Schumacher M, Guennoun R, Robert F, Carelli C, Gago N, Ghoumari A, et al. Local synthesis and dual actions of progesterone in the nervous system: neuroprotection and myelination. Growth Hormone IGF Res. (2004) 14(Suppl A):S18–33. doi: 10.1016/j.ghir.2004.03.007
7. Schumacher M, Weill-Engerer S, Liere P, Robert F, Franklin RJ, Garcia-Segura LM, et al. Steroid hormones and neurosteroids in normal and pathological aging of the nervous system. Prog Neurobiol. (2003) 71:3–29. doi: 10.1016/j.pneurobio.2003.09.004
8. Guennoun R, Labombarda F, Gonzalez Deniselle MC, Liere P, De Nicola AF, Schumacher M. Progesterone and allopregnanolone in the central nervous system: response to injury and implication for neuroprotection. J Ster Biochem Mol Biol. (2015) 146:48–61. doi: 10.1016/j.jsbmb.2014.09.001
9. Zhang P, Xie MQ, Ding YQ, Liao M, Qi SS, Chen SX, et al. Allopregnanolone enhances the neurogenesis of midbrain dopaminergic neurons in APPswe/PSEN1 mice. Neuroscience. (2015) 290:214–26. doi: 10.1016/j.neuroscience.2015.01.019
10. Cai H, Cao T, Zhou X, Yao JK. Neurosteroids in schizophrenia: pathogenic and therapeutic implications. Front Psychiatry. (2018) 9:73. doi: 10.3389/fpsyt.2018.00073
11. Reddy DS, Rogawski MA. Neurosteroid replacement therapy for catamenial epilepsy. Neurotherapeutics. (2009) 6:392–401. doi: 10.1016/j.nurt.2009.01.006
12. Morrow AL. Recent developments in the significance and therapeutic relevance of neuroactive steroids–Introduction to the special issue. Pharmacol Therap. (2007) 116:1–6. doi: 10.1016/j.pharmthera.2007.04.003
13. Dubrovsky BO. Steroids, neuroactive steroids and neurosteroids in psychopathology. Prog Neuropsychopharmacol Biol Psychiatry. (2005) 29:169–92. doi: 10.1016/j.pnpbp.2004.11.001
14. Mayo W, Dellu F, Robel P, Cherkaoui J, Le Moal M, Baulieu EE, et al. Infusion of neurosteroids into the nucleus basalis magnocellularis affects cognitive processes in the rat. Brain Res. (1993) 607:324–8. doi: 10.1016/0006-8993(93)91524-V
15. Vallee M, Mayo W, Darnaudery M, Corpechot C, Young J, Koehl M, et al. Neurosteroids: deficient cognitive performance in aged rats depends on low pregnenolone sulfate levels in the hippocampus. Proc Natl Acad Sci USA. (1997) 94:14865–70. doi: 10.1073/pnas.94.26.14865
16. Vallee M, Mayo W, Le Moal M. Role of pregnenolone, dehydroepiandrosterone and their sulfate esters on learning and memory in cognitive aging. Brain Res Brain Res Rev. (2001) 37:301–12. doi: 10.1016/S0165-0173(01)00135-7
17. Vallee M, Mayo W, Koob GF, Le Moal M. Neurosteroids in learning and memory processes. Int Rev Neurobiol. (2001) 46:273–320. doi: 10.1016/S0074-7742(01)46066-1
18. Ladurelle N, Eychenne B, Denton D, Blair-West J, Schumacher M, Robel P, et al. Prolonged intracerebroventricular infusion of neurosteroids affects cognitive performances in the mouse. Brain Res. (2000) 858:371–9. doi: 10.1016/S0006-8993(00)01953-3
19. Mittelman-Smith MA, Wong AM, Micevych PE. Estrogen and progesterone integration in an in vitro model of RP3V kisspeptin neurons. Neuroendocrinology. (2018) 106:101–15. doi: 10.1159/000471878
20. Mittelman-Smith MA, Williams H, Krajewski-Hall SJ, Lai J, Ciofi P, McMullen NT, et al. Arcuate kisspeptin/neurokinin B/dynorphin (KNDy) neurons mediate the estrogen suppression of gonadotropin secretion and body weight. Endocrinology. (2012) 153:2800–12. doi: 10.1210/en.2012-1045
21. Rance NE, Dacks PA, Mittelman-Smith MA, Romanovsky AA, Krajewski-Hall SJ. Modulation of body temperature and LH secretion by hypothalamic KNDy (kisspeptin, neurokinin B and dynorphin) neurons: a novel hypothesis on the mechanism of hot flushes. Front Neuroendocrinol. (2013) 34:211–27. doi: 10.1016/j.yfrne.2013.07.003
22. Navarro VM. New insights into the control of pulsatile GnRH release: the role of Kiss1/neurokinin B neurons. Front Endocrinol. (2012) 3:48. doi: 10.3389/fendo.2012.00048
23. Herbison AE, Theodosis DT. Localization of oestrogen receptors in preoptic neurons containing neurotensin but not tyrosine hydroxylase, cholecystokinin or luteinizing hormone-releasing hormone in the male and female rat. Neuroscience. (1992) 50:283–98. doi: 10.1016/0306-4522(92)90423-Y
24. Shivers BD, Harlan RE, Morrell JI, Pfaff DW. Absence of oestradiol concentration in cell nuclei of LHRH-immunoreactive neurones. Nature. (1983) 304:345–7. doi: 10.1038/304345a0
25. Wintermantel TM, Campbell RE, Porteous R, Bock D, Grone HJ, Todman MG, et al. Definition of estrogen receptor pathway critical for estrogen positive feedback to gonadotropin-releasing hormone neurons and fertility. Neuron. (2006) 52:271–80. doi: 10.1016/j.neuron.2006.07.023
26. Smith JT, Cunningham MJ, Rissman EF, Clifton DK, Steiner RA. Regulation of Kiss1 gene expression in the brain of the female mouse. Endocrinology. (2005) 146:3686–92. doi: 10.1210/en.2005-0488
27. Lehman MN, Merkley CM, Coolen LM, Goodman RL. Anatomy of the kisspeptin neural network in mammals. Brain Res. (2010) 1364:90–102. doi: 10.1016/j.brainres.2010.09.020
28. Zhang J, Yang L, Lin N, Pan X, Zhu Y, Chen X. Aging-related changes in RP3V kisspeptin neurons predate the reduced activation of GnRH neurons during the early reproductive decline in female mice. Neurobiol Aging. (2014) 35:655–68. doi: 10.1016/j.neurobiolaging.2013.08.038
29. Han SK, Gottsch ML, Lee KJ, Popa SM, Smith JT, Jakawich SK, et al. Activation of gonadotropin-releasing hormone neurons by kisspeptin as a neuroendocrine switch for the onset of puberty. J Neurosci. (2005) 25:11349–56. doi: 10.1523/JNEUROSCI.3328-05.2005
30. Clarkson J, Herbison AE. Postnatal development of kisspeptin neurons in mouse hypothalamus; sexual dimorphism and projections to gonadotropin-releasing hormone neurons. Endocrinol. (2006) 147:5817–25. doi: 10.1210/en.2006-0787
31. Liu X, Lee K, Herbison AE. Kisspeptin excites gonadotropin-releasing hormone neurons through a phospholipase C/calcium-dependent pathway regulating multiple ion channels. Endocrinology. (2008) 149:4605–14. doi: 10.1210/en.2008-0321
32. Delhousay LK, Chuon T, Mittleman-Smith M, Micevych P, Sinchak K. RP3V kisspeptin neurons mediate neuroprogesterone induction of the luteinizing hormone surge in female rat. bioRxiv. (2019) 2019:700435. doi: 10.1101/700435
33. Chuon T, Feri M, Carlson C, Ondrejik S, Micevych P, Sinchak K. Neuroprogesterone signals through Src kinase within RP3V neurons to induce the luteinizing hormone surge in female rats. bioRxiv. (2019) 2019:835470. doi: 10.1101/835470
34. Petersen SL, Barraclough CA. Suppression of spontaneous LH surges in estrogen-treated ovariectomized rats by microimplants of antiestrogens into the preoptic brain. Brain Res. (1989) 484:279–89. doi: 10.1016/0006-8993(89)90371-5
35. Wiegand SJ, Terasawa E, Bridson WE. Persistent estrus and blockade of progesterone-induced LH release follows lesions which do not damage the suprachiasmatic nucleus. Endocrinology. (1978) 102:1645–8. doi: 10.1210/endo-102-5-1645
36. Wiegand SJ, Terasawa E. Discrete lesions reveal functional heterogeneity of suprachiasmatic structures in regulation of gonadotropin secretion in the female rat. Neuroendocrinology. (1982) 34:395–404. doi: 10.1159/000123335
37. Clarkson J, d'Anglemont de Tassigny X, Moreno AS, Colledge WH, Herbison AE. Kisspeptin-GPR54 signaling is essential for preovulatory gonadotropin-releasing hormone neuron activation and the luteinizing hormone surge. J Neurosci. (2008) 28:8691–7. doi: 10.1523/JNEUROSCI.1775-08.2008
38. Adachi S, Yamada S, Takatsu Y, Matsui H, Kinoshita M, Takase K, et al. Involvement of anteroventral periventricular metastin/kisspeptin neurons in estrogen positive feedback action on luteinizing hormone release in female rats. J Reprod Dev. (2007) 53:367–78. doi: 10.1262/jrd.18146
39. Williams WP 3rd, Jarjisian SG, Mikkelsen JD, Kriegsfeld LJ. Circadian control of kisspeptin and a gated GnRH response mediate the preovulatory luteinizing hormone surge. Endocrinology. (2011) 152:595–606. doi: 10.1210/en.2010-0943
40. Herbison AE, de Tassigny X, Doran J, Colledge WH. Distribution and postnatal development of Gpr54 gene expression in mouse brain and gonadotropin-releasing hormone neurons. Endocrinology. (2010) 151:312–21. doi: 10.1210/en.2009-0552
41. Parhar IS, Ogawa S, Sakuma Y. Laser-captured single digoxigenin-labeled neurons of gonadotropin-releasing hormone types reveal a novel G protein-coupled receptor (Gpr54) during maturation in cichlid fish. Endocrinology. (2004) 145:3613–8. doi: 10.1210/en.2004-0395
42. Bosch MA, Tonsfeldt KJ, Ronnekleiv OK. mRNA expression of ion channels in GnRH neurons: subtype-specific regulation by 17beta-estradiol. Mol Cell Endocrinol. (2013) 367:85–97. doi: 10.1016/j.mce.2012.12.021
43. Kotani M, Detheux M, Vandenbogaerde A, Communi D, Vanderwinden JM, Le Poul E, et al. The metastasis suppressor gene KiSS-1 encodes kisspeptins, the natural ligands of the orphan G protein-coupled receptor GPR54. J Biol Chem. (2001) 276:34631–6. doi: 10.1074/jbc.M104847200
44. Li Q, Jimenez-Krassel F, Ireland JJ, Smith GW. Gene expression profiling of bovine preovulatory follicles: gonadotropin surge and prostanoid-dependent up-regulation of genes potentially linked to the ovulatory process. Reproduction. (2009) 137:297–307. doi: 10.1530/REP-08-0308
45. Roseweir AK, Millar RP. The role of kisspeptin in the control of gonadotrophin secretion. Hum Reprod Update. (2009) 15:203–12. doi: 10.1093/humupd/dmn058
46. Kirilov M, Clarkson J, Liu X, Roa J, Campos P, Porteous R, et al. Dependence of fertility on kisspeptin-Gpr54 signaling at the GnRH neuron. Nat Commun. (2013) 4:2492. doi: 10.1038/ncomms3492
47. Novaira HJ, Sonko ML, Hoffman G, Koo Y, Ko C, Wolfe A, et al. Disrupted kisspeptin signaling in GnRH neurons leads to hypogonadotrophic hypogonadism. Mol Endocrinol. (2014) 28:225–38. doi: 10.1210/me.2013-1319
48. Gotlieb N, Moeller J, Kriegsfeld LJ. Circadian control of neuroendocrine function: implications for health and disease. Curr Opin Physiol. (2018) 5:133–40. doi: 10.1016/j.cophys.2018.11.001
49. Gotlieb N, Baker CN, Moeller J, Kriegsfeld LJ. Time-of-day-dependent sensitivity of the reproductive axis to RFamide-related peptide-3 inhibition in female Syrian hamsters. J Neuroendocrinol. (2019) 31:e12798. doi: 10.1111/jne.12798
50. Rizwan MZ, Poling MC, Corr M, Cornes PA, Augustine RA, Quennell JH, et al. RFamide-related peptide-3 receptor gene expression in GnRH and kisspeptin neurons and GnRH-dependent mechanism of action. Endocrinology. (2012) 153:3770–9. doi: 10.1210/en.2012-1133
51. Poling MC, Quennell JH, Anderson GM, Kauffman AS. Kisspeptin neurones do not directly signal to RFRP-3 neurones but RFRP-3 may directly modulate a subset of hypothalamic kisspeptin cells in mice. J Neuroendocrinol. (2013) 25:876–86. doi: 10.1111/jne.12084
52. Gibson EM, Humber SA, Jain S, Williams WP 3rd, Zhao S, Bentley GE, et al. Alterations in RFamide-related peptide expression are coordinated with the preovulatory luteinizing hormone surge. Endocrinology. (2008) 149:4958–69. doi: 10.1210/en.2008-0316
53. Kriegsfeld LJ, Mei DF, Bentley GE, Ubuka T, Mason AO, Inoue K, et al. Identification and characterization of a gonadotropin-inhibitory system in the brains of mammals. Proc Natl Acad Sci USA. (2006) 103:2410–5. doi: 10.1073/pnas.0511003103
54. Chazal G, Faudon M, Gogan F, Laplante E. Negative and positive effects of oestradiol upon luteinizing hormone secretion in the female rat. J Endocrinol. (1974) 61:511–2. doi: 10.1677/joe.0.0610511
55. Mann DR, Korowitz CD, Macfarland LA, Cost MG. Interactions of the light-dark cycle, adrenal glands and time of steroid administration in determining the temporal sequence of LH and prolactin release in female rats. Endocrinol. (1976) 99:1252–62. doi: 10.1210/endo-99-5-1252
56. Sridaran R, Blake CA. Effects of long-term adrenalectomy on periovulatory increases in serum gonadotrophins and ovulation in rats. J Endocrinol. (1980) 84:75–82. doi: 10.1677/joe.0.0840075
57. DePaolo LV, Barraclough CA. Dose dependent effects of progesterone on the facilitation and inhibition of spontaneous gonadotropin surges in estrogen treated ovariectomized rats. BiolReprod. (1979) 21:1015–23. doi: 10.1095/biolreprod21.4.1015
58. Brom GM, Schwartz NB. Acute changes in the estrous cycle following ovariectomy in the golden hamster. Neuroendocrinology. (1968) 3:366–77. doi: 10.1159/000121725
59. Ferin M, Tempone A, Zimmering PE, Van de Wiele RL. Effect of antibodies to 17beta-estradiol and progesterone on the estrous cycle of the rat. Endocrinology. (1969) 85:1070–8. doi: 10.1210/endo-85-6-1070
60. Labhsetwar AP. Role of estrogens in ovulation: a study using the estrogen-antagonist, I.C.I. 46,474. Endocrinology. (1970) 87:542–51. doi: 10.1210/endo-87-3-542
61. Rao IM, Mahesh VB. Role of progesterone in the modulation of the preovulatory surge of gonadotropins and ovulation in the pregnant mare's serum gonadotropin-primed immature rat and the adult rat. Biol Reprod. (1986) 35:1154–61. doi: 10.1095/biolreprod35.5.1154
62. Mahesh VB, Brann DW. Regulation of the preovulatory gonadotropin surge by endogenous steroids. Steroids. (1998) 63:616–29. doi: 10.1016/S0039-128X(98)00075-0
63. Chappell PE, Levine JE. Stimulation of gonadotropin-releasing hormone surges by estrogen. I. Role of hypothalamic progesterone receptors. Endocrinology. (2000) 141:1477–85. doi: 10.1210/endo.141.4.7428
64. Nath R, Bhakta A, Moudgil VK. ZK98299–a new antiprogesterone: biochemical characterization of steroid binding parameters in the calf uterine cytosol. Arch Biochem Biophys. (1992) 292:303–10. doi: 10.1016/0003-9861(92)90083-9
65. Micevych PE, Chaban V, Ogi J, Dewing P, Lu JK, Sinchak K. Estradiol stimulates progesterone synthesis in hypothalamic astrocyte cultures. Endocrinology. (2007) 148:782–9. doi: 10.1210/en.2006-0774
66. Micevych P, Sinchak K. Synthesis and function of hypothalamic neuroprogesterone in reproduction. Endocrinology. (2008) 149:2739–42. doi: 10.1210/en.2008-0011
67. Micevych P, Sinchak K. Estradiol regulation of progesterone synthesis in the brain. Mol Cell Endocrinol. (2008) 290:44–50. doi: 10.1016/j.mce.2008.04.016
68. Micevych PE, Sinchak K. The neurosteroid progesterone underlies estrogen positive feedback of the LH surge. Front Endocrinol. (2011) 2:90. doi: 10.3389/fendo.2011.00090
69. Smith JT, Popa SM, Clifton DK, Hoffman GE, Steiner RA. Kiss1 neurons in the forebrain as central processors for generating the preovulatory luteinizing hormone surge. J Neurosci. (2006) 26:6687–94. doi: 10.1523/JNEUROSCI.1618-06.2006
70. Gottsch ML, Navarro VM, Zhao Z, Glidewell-Kenney C, Weiss J, Jameson JL, et al. Regulation of Kiss1 and dynorphin gene expression in the murine brain by classical and nonclassical estrogen receptor pathways. J Neurosci. (2009) 29:9390–5. doi: 10.1523/JNEUROSCI.0763-09.2009
71. Glidewell-Kenney C, Hurley LA, Pfaff L, Weiss J, Levine JE, Jameson JL. Nonclassical estrogen receptor alpha signaling mediates negative feedback in the female mouse reproductive axis. Proc Natl Acad Sci USA. (2007) 104:8173–7. doi: 10.1073/pnas.0611514104
72. Micevych P, Sinchak K, Mills RH, Tao L, LaPolt P, Lu JK. The luteinizing hormone surge is preceded by an estrogen-induced increase of hypothalamic progesterone in ovariectomized and adrenalectomized rats. Neuroendocrinol. (2003) 78:29–35. doi: 10.1159/000071703
73. Mittelman-Smith MA, Rudolph LM, Mohr MA, Micevych PE. Rodent models of non-classical progesterone action regulating ovulation. Front Endocrinol. (2017) 8:165. doi: 10.3389/fendo.2017.00165
74. Zwain IH, Yen SS. Neurosteroidogenesis in astrocytes, oligodendrocytes, and neurons of cerebral cortex of rat brain. Endocrinol. (1999) 140:3843–52. doi: 10.1210/endo.140.8.6907
75. Micevych PE, Wong AM, Mittelman-Smith MA. Estradiol membrane-initiated signaling and female reproduction. Compr Physiol. (2015) 5:1211–22. doi: 10.1002/cphy.c140056
76. Chen C, Kuo J, Wong A, Micevych P. Estradiol Modulates Translocator Protein (TSPO) and Steroid Acute Regulatory Protein (StAR) via Protein Kinase A (PKA) signaling in hypothalamic astrocytes. Endocrinology. (2014) 155:2976–85. doi: 10.1210/en.2013-1844
77. Itzhak Y, Baker L, Norenberg MD. Characterization of the peripheral-type benzodiazepine receptors in cultured astrocytes: evidence for multiplicity. Glia. (1993) 9:211–8. doi: 10.1002/glia.440090306
78. Papadopoulos V, Baraldi M, Guilarte TR, Knudsen TB, Lacapere JJ, Lindemann P, et al. Translocator protein (18kDa): new nomenclature for the peripheral-type benzodiazepine receptor based on its structure and molecular function. Trends Pharmacol Sci. (2006) 27:402–9. doi: 10.1016/j.tips.2006.06.005
79. Tu LN, Morohaku K, Manna PR, Pelton SH, Butler WR, Stocco DM, et al. Peripheral benzodiazepine receptor/translocator protein global knock-out mice are viable with no effects on steroid hormone biosynthesis. J Biol Chem. (2014) 289:27444–54. doi: 10.1074/jbc.M114.578286
80. Fan J, Campioli E, Midzak A, Culty M, Papadopoulos V. Conditional steroidogenic cell-targeted deletion of TSPO unveils a crucial role in viability and hormone-dependent steroid formation. Proc Natl Acad Sci USA. (2015) 112:7261–6. doi: 10.1073/pnas.1502670112
81. Barron AM, Ji B, Kito S, Suhara T, Higuchi M. Steroidogenic abnormalities in translocator protein knockout mice and significance in the aging male. Biochem J. (2018) 475:75–85. doi: 10.1042/BCJ20170645
82. Mittelman-Smith MA, Wong AM, Kathiresan AS, Micevych PE. Classical and membrane-initiated estrogen signaling in an in vitro model of anterior hypothalamic kisspeptin neurons. Endocrinology. (2015) 156:2162–73. doi: 10.1210/en.2014-1803
83. Chaban VV, Lakhter AJ, Micevych P. A membrane estrogen receptor mediates intracellular calcium release in astrocytes. Endocrinology. (2004) 145:3788–95. doi: 10.1210/en.2004-0149
84. Kuo J, Hariri OR, Bondar G, Ogi J, Micevych P. Membrane estrogen receptor-alpha interacts with metabotropic glutamate receptor type 1a to mobilize intracellular calcium in hypothalamic astrocytes. Endocrinology. (2009) 150:1369–76. doi: 10.1210/en.2008-0994
85. Micevych P, Soma KK, Sinchak K. Neuroprogesterone: Key to estrogen positive feedback? Brain Res Rev. (2008) 57:470–80. doi: 10.1016/j.brainresrev.2007.06.009
86. Kuo J, Hamid N, Bondar G, Prossnitz ER, Micevych P. Membrane estrogen receptors stimulate intracellular calcium release and progesterone synthesis in hypothalamic astrocytes. J Neurosci. (2010) 30:12950–7. doi: 10.1523/JNEUROSCI.1158-10.2010
87. Soma KK, Sinchak K, Lakhter A, Schlinger BA, Micevych PE. Neurosteroids and female reproduction: estrogen increases 3beta-HSD mRNA and activity in rat hypothalamus. Endocrinology. (2005) 146:4386–90. doi: 10.1210/en.2005-0569
88. Micevych PE, Matt DW, Go VLW. Concentrations of cholecystokinin, substance P, and bombesin in discrete regions of male and female rat brain: Sex differences and estrogen effects. Exp Neurol. (1981) 100:416–26. doi: 10.1016/0014-4886(88)90119-7
89. Chappell PE, Schneider JS, Kim P, Xu M, Lydon JP, O'Malley BW, et al. Absence of gonadotropin surges and gonadotropin-releasing hormone self- priming in ovariectomized (OVX), estrogen (E2)-treated, progesterone receptor knockout (PRKO) mice. Endocrinology. (1999) 140:3653–8. doi: 10.1210/endo.140.8.6895
90. Gal A, Lin PC, Cacioppo JA, Hannon PR, Mahoney MM, Wolfe A, et al. Loss of fertility in the absence of progesterone receptor expression in kisspeptin neurons of female mice. PLoS ONE. (2016) 11:e0159534. doi: 10.1371/journal.pone.0159534
91. Stephens SB, Tolson KP, Rouse ML Jr, Poling MC, Hashimoto-Partyka MK, et al. Absent progesterone signaling in kisspeptin neurons disrupts the LH surge and impairs fertility in female mice. Endocrinology. (2015) 156:3091–7. doi: 10.1210/en.2015-1300
92. Pedram A, Razandi M, Sainson RC, Kim JK, Hughes CC, Levin ER. A conserved mechanism for steroid receptor translocation to the plasma membrane. J Biol Chem. (2007) 282:22278–88. doi: 10.1074/jbc.M611877200
93. Boonyaratanakornkit V, Scott MP, Ribon V, Sherman L, Anderson SM, Maller JL, et al. Progesterone receptor contains a proline-rich motif that directly interacts with SH3 domains and activates c-Src family tyrosine kinases. Mol cell. (2001) 8:269–80. doi: 10.1016/S1097-2765(01)00304-5
94. Boonyaratanakornkit V, McGowan E, Sherman L, Mancini MA, Cheskis BJ, Edwards DP. The role of extranuclear signaling actions of progesterone receptor in mediating progesterone regulation of gene expression and the cell cycle. Mol Endocrinol. (2007) 21:359–75. doi: 10.1210/me.2006-0337
95. Pang DT, Wang JK, Valtorta F, Benfenati F, Greengard P. Protein tyrosine phosphorylation in synaptic vesicles. Proc Natl Acad Sci USA. (1988) 85:762–6. doi: 10.1073/pnas.85.3.762
96. Kastner P, Krust A, Turcotte B, Stropp U, Tora L, Gronemeyer H, et al. Two distinct estrogen-regulated promoters generate transcripts encoding the two functionally different human progesterone receptor forms A and B. EMBO J. (1990) 9:1603–14. doi: 10.1002/j.1460-2075.1990.tb08280.x
97. Micevych PE, Sinchak K. Extranuclear signaling by ovarian steroids in the regulation of sexual receptivity. Hormones Behav. (2018) 104:4–14. doi: 10.1016/j.yhbeh.2018.05.001
98. Mani SK, Allen JM, Lydon JP, Mulac-Jericevic B, Blaustein JD, DeMayo FJ, et al. Dopamine requires the unoccupied progesterone receptor to induce sexual behavior in mice [published erratum appears in Mol Endocrinol 1997 Apr;11:423]. MolEndocrinol. (1996) 10:1728–37. doi: 10.1210/mend.10.12.8961281
99. Sleiter N, Pang Y, Park C, Horton TH, Dong J, Thomas P, et al. Progesterone receptor A (PRA) and PRB-independent effects of progesterone on gonadotropin-releasing hormone release. Endocrinology. (2009) 150:3833–44. doi: 10.1210/en.2008-0774
100. Mani SK, Blaustein JD. Neural progestin receptors and female sexual behavior. Neuroendocrinology. (2012) 96:152–61. doi: 10.1159/000338668
101. Zhu Y, Bond J, Thomas P. Identification, classification, and partial characterization of genes in humans and other vertebrates homologous to a fish membrane progestin receptor. Proc Natl Acad Sci USA. (2003) 100:2237–42. doi: 10.1073/pnas.0436133100
102. Zhu Y, Rice CD, Pang Y, Pace M, Thomas P. Cloning, expression, and characterization of a membrane progestin receptor and evidence it is an intermediary in meiotic maturation of fish oocytes. Proc Natl Acad Sci USA. (2003) 100:2231–6. doi: 10.1073/pnas.0336132100
103. Pang Y, Dong J, Thomas P. Characterization, neurosteroid binding and brain distribution of human membrane progesterone receptors delta and {epsilon} (mPRdelta and mPR{epsilon) and mPRdelta involvement in neurosteroid inhibition of apoptosis. Endocrinology. (2013) 154:283–95. doi: 10.1210/en.2012-1772
104. Zuloaga DG, Yahn SL, Pang Y, Quihuis AM, Oyola MG, Reyna A, et al. Distribution and estrogen regulation of membrane progesterone receptor-beta in the female rat brain. Endocrinology. (2012) 153:4432–43. doi: 10.1210/en.2012-1469
105. Peluso JJ, Gawkowska A, Liu X, Shioda T, Pru JK. Progesterone receptor membrane component-1 regulates the development and Cisplatin sensitivity of human ovarian tumors in athymic nude mice. Endocrinology. (2009) 150:4846–54. doi: 10.1210/en.2009-0730
106. Peluso JJ, Liu X, Gawkowska A, Johnston-MacAnanny E. Progesterone activates a progesterone receptor membrane component 1-dependent mechanism that promotes human granulosa/luteal cell survival but not progesterone secretion. J Clin Endocrinol Metab. (2009) 94:2644–9. doi: 10.1210/jc.2009-0147
107. Peluso JJ, Liu X, Uliasz T, Pru CA, Kelp NC, Pru J. PGRMC1/2 promotes luteal vascularization and maintains the primordial follicles of mice. Reproduction. (2018) 156:365–73. doi: 10.1530/REP-18-0155
108. Peluso JJ, Romak J, Liu X. Progesterone receptor membrane component-1 (PGRMC1) is the mediator of progesterone's antiapoptotic action in spontaneously immortalized granulosa cells as revealed by PGRMC1 small interfering ribonucleic acid treatment and functional analysis of PGRMC1 mutations. Endocrinology. (2008) 149:534–43. doi: 10.1210/en.2007-1050
109. Intlekofer KA, Petersen SL. Distribution of mRNAs encoding classical progestin receptor, progesterone membrane components 1 and 2, serpine mRNA binding protein 1, and progestin and ADIPOQ receptor family members 7 and 8 in rat forebrain. Neuroscience. (2011) 172:55–65. doi: 10.1016/j.neuroscience.2010.10.051
110. Clark NC, Pru CA, Yee SP, Lydon JP, Peluso JJ, Pru JK. Conditional ablation of progesterone receptor membrane component 2 causes female premature reproductive senescence. Endocrinology. (2017) 158:640–51. doi: 10.1210/en.2016-1701
111. Krebs CJ, Jarvis ED, Chan J, Lydon JP, Ogawa S, Pfaff DW. A membrane-associated progesterone-binding protein, 25-Dx, is regulated by progesterone in brain regions involved in female reproductive behaviors. Proc Natl Acad Sci USA. (2000) 97:12816–21. doi: 10.1073/pnas.97.23.12816
112. Guo M, Zhang C, Wang Y, Feng L, Wang Z, Niu W, et al. Progesterone receptor membrane component 1 mediates progesterone-induced suppression of oocyte meiotic prophase i and primordial folliculogenesis. Sci Rep. (2016) 6:36869. doi: 10.1038/srep36869
113. Skiadas CC, Duan S, Correll M, Rubio R, Karaca N, Ginsburg ES, et al. Ovarian reserve status in young women is associated with altered gene expression in membrana granulosa cells. Mol Hum Reprod. (2012) 18:362–71. doi: 10.1093/molehr/gas008
114. Mansouri MR, Schuster J, Badhai J, Stattin EL, Losel R, Wehling M, et al. Alterations in the expression, structure and function of progesterone receptor membrane component-1 (PGRMC1) in premature ovarian failure. Hum Mol Genet. (2008) 17:3776–83. doi: 10.1093/hmg/ddn274
115. Intlekofer KA, Petersen SL. 17beta-estradiol and progesterone regulate multiple progestin signaling molecules in the anteroventral periventricular nucleus, ventromedial nucleus and sexually dimorphic nucleus of the preoptic area in female rats. Neuroscience. (2011) 176:86-92. doi: 10.1016/j.neuroscience.2010.12.033
116. Richter TA, Terasawa E. Neural mechanisms underlying the pubertal increase in LHRH release in the rhesus monkey. Trends Endocrinol Metab. (2001) 12:353–9. doi: 10.1016/S1043-2760(01)00442-8
117. Mayer C, Acosta-Martinez M, Dubois SL, Wolfe A, Radovick S, Boehm U, et al. Timing and completion of puberty in female mice depend on estrogen receptor alpha-signaling in kisspeptin neurons. Proc Natl Acad Sci USA. (2010) 107:22693–8. doi: 10.1073/pnas.1012406108
118. Mohr MA, Falcy BA, Laham BJ, Wong A, Micevych PE. Neuroprogesterone synthesis in hypothalamic astrocytes is associated with a pubertal increase in membrane ERα levels. bioRxiv. (2019) 2019:833434. doi: 10.1101/833434
119. Micevych PE, Mermelstein PG, Sinchak K. Estradiol membrane-initiated signaling in the brain mediates reproduction. Trends Neurosci. (2017) 40:654–66. doi: 10.1016/j.tins.2017.09.001
120. Mohr MA, DonCarlos LL, Sisk CL. Inhibiting production of new brain cells during puberty or adulthood blunts the hormonally induced surge of luteinizing hormone in female rats. eNeuro. (2017) 4, 1–13. doi: 10.1523/ENEURO.0133-17.2017
121. Mohr MA, Garcia FL, DonCarlos LL, Sisk CL. Neurons and glial cells are added to the female rat anteroventral periventricular nucleus during puberty. Endocrinology. (2016) 157:2393–402. doi: 10.1210/en.2015-2012
122. Ahmed EI, Zehr JL, Schulz KM, Lorenz BH, DonCarlos LL, Sisk CL. Pubertal hormones modulate the addition of new cells to sexually dimorphic brain regions. Nat Neurosci. (2008) 11:995–7. doi: 10.1038/nn.2178
123. Davis EC, Shryne JE, Gorski RA. Structural sexual dimorphisms in the anteroventral periventricular nucleus of the rat hypothalamus are sensitive to gonadal steroids perinatally, but develop peripubertally. Neuroendocrinology. (1996) 63:142–8. doi: 10.1159/000126950
Keywords: neuroprogesterone, astrocyte, Src kinase, kisspeptin, RP3V, AVPV, estradiol, LH surge
Citation: Sinchak K, Mohr MA and Micevych PE (2020) Hypothalamic Astrocyte Development and Physiology for Neuroprogesterone Induction of the Luteinizing Hormone Surge. Front. Endocrinol. 11:420. doi: 10.3389/fendo.2020.00420
Received: 24 March 2020; Accepted: 26 May 2020;
Published: 26 June 2020.
Edited by:
Takayoshi Ubuka, Waseda University, JapanReviewed by:
William Henry Colledge, University of Cambridge, United KingdomCopyright © 2020 Sinchak, Mohr and Micevych. This is an open-access article distributed under the terms of the Creative Commons Attribution License (CC BY). The use, distribution or reproduction in other forums is permitted, provided the original author(s) and the copyright owner(s) are credited and that the original publication in this journal is cited, in accordance with accepted academic practice. No use, distribution or reproduction is permitted which does not comply with these terms.
*Correspondence: Kevin Sinchak, S2V2aW4uc2luY2hha0Bjc3VsYi5lZHU=
Disclaimer: All claims expressed in this article are solely those of the authors and do not necessarily represent those of their affiliated organizations, or those of the publisher, the editors and the reviewers. Any product that may be evaluated in this article or claim that may be made by its manufacturer is not guaranteed or endorsed by the publisher.
Research integrity at Frontiers
Learn more about the work of our research integrity team to safeguard the quality of each article we publish.