- 1Department of Internal Medicine, Amsterdam University Medical Center, Amsterdam Movement Sciences, Amsterdam, Netherlands
- 2Department of Osteology and Biomechanics, University Medical Center Hamburg-Eppendorf, Hamburg, Germany
- 3Department of Clinical Genetics, Amsterdam University Medical Center, Amsterdam Movement Sciences, Vrije Universiteit Amsterdam, Amsterdam, Netherlands
- 4Bone and Calcium Metabolism Lab, Department of Clinical Chemistry, Amsterdam University Medical Center, Amsterdam Movement Sciences, Amsterdam, Netherlands
Introduction: Bone biopsies have been obtained for many centuries and are one of the oldest known medical procedures in history. Despite the introduction of new noninvasive radiographic imaging techniques and genetic analyses, bone biopsies are still valuable in the diagnosis of bone diseases. Advanced techniques for the assessment of bone quality in bone biopsies, which have emerged during the last decades, allows in-depth tissue analyses beyond structural changes visible in bone histology. In this review, we give an overview of the application and advantages of the advanced techniques for the analysis of bone biopsies in the clinical setting of various rare metabolic bone diseases.
Method: A systematic literature search on rare metabolic bone diseases and analyzing techniques of bone biopsies was performed in PubMed up to 2019 week 34.
Results: Advanced techniques for the analysis of bone biopsies were described for rare metabolic bone disorders including Paget's disease of bone, osteogenesis imperfecta, fibrous dysplasia, Fibrodysplasia ossificans progressiva, PLS3 X-linked osteoporosis, Loeys-Diets syndrome, osteopetrosis, Erdheim-Chester disease, and Cherubism. A variety of advanced available analytical techniques were identified that may help to provide additional detail on cellular, structural, and compositional characteristics in rare bone diseases complementing classical histopathology.
Discussion: To date, these techniques have only been used in research and not in daily clinical practice. Clinical application of bone quality assessment techniques depends upon several aspects such as availability of the technique in hospitals, the existence of reference data, and a cooperative network of researchers and clinicians. The evaluation of rare metabolic bone disorders requires a repertoire of different methods, owing to their distinct bone tissue characteristics. The broader use of bone material obtained from biopsies could provide much more information about pathophysiology or treatment options and establish bone biopsies as a valuable tool in rare metabolic bone diseases.
Introduction
Trepanning of bone is one of the oldest medical procedures known in history. This procedure was initially carried out for the treatment of headache and mental illness. The first application of trepanning as a diagnostic tool was described early 1900 by Pianese for bone marrow aspiration. During the last century, different needles have been developed, including a type for bone biopsy acquisition (1). The first modern needle for the performance of an iliac crest biopsy was described in 1954 (2). Around 1950, modern embedding techniques were discovered for the microscopic examination of mineralized bone tissue (3). Beside conventional X-rays and biochemical markers, histopathological analyses were considered for many years to be the hallmark for the diagnosis of bone-related disorders. The publication of standardization of histomorphometric nomenclature in 1987, largely improved communication between practitioners of bone histomorphometry, medical doctors, and scientists (4). This led to a broader understanding of histomorphometric data (5). The introduction of more advanced radiographic imaging techniques has complemented the role of bone biopsies are no longer the only diagnostic tool for bone disorders. Dual energy x-ray absorptiometry (DXA) is a widely used method to measure bone mineral density to estimate fracture risk. However, measurement of bone mineral density does neither provide information about the microarchitecture of bone, bone cell activity, and bone remodeling, nor allows the consideration of bone volume and density independently of each other. Histology and quantitative histomorphometric analyses are still the most commonly used method for analyzing bone samples; this is often combined with double-labeling with tetracycline which provides additional information about bone mineralization.
The indication for a bone biopsy has shifted from diagnostics of bone structural changes to an additional assessment of compositional information at the tissue level. This shift has taken place due to the availability of new imaging and analyses techniques, a broader use of biochemical markers, and genetic testing. Nowadays bone biopsies are indicated in patients with early onset osteoporosis, renal osteodystrophy, malignant disorders, suspicion of osteomalacia, or rare (metabolic) bone disorders for the analysis of bone mass, cortical and trabecular structure, bone turnover and mineralization as well as cellular status. All these parameters need to be considered in the thorough evaluation of bone quality. Here, bone quality can be considered as the total sum of characteristics with the ability to resist bone fracture, in particular the combination of structural and compositional bone characteristics, as well as the bone cellular activity. Impaired bone quality or bone fragility leads to fractures. Certainly, bone histomorphometric assessment in addition to T-Scores measured with DXA remains useful to predict fracture risk in different rare metabolic bone disorders. However, specific techniques for analyzing bone quality in bone biopsies have become available to examine the pathophysiology in rare bone diseases in more detail. These additional analysis techniques include Fourier transform infrared spectroscopy (FTIR), Raman spectroscopy, transmission electron microscopy (TEM), quantitative backscattered electron imaging (qBEI), confocal microscopy, microCT (μCT), micro- and nano-indentation, high-performance liquid chromatography (HPLC), atomic force microscopy (AFM), small-angle X-ray scattering (SAXS), wide-angle X-ray scattering (WAXS), nuclear magnetic resonance (NMR), and immunohistochemistry (Table 1). Currently, the aforementioned techniques are predominantly applied in research settings and are mostly not used in routine clinical practice. This review focuses on the state-of-the-art assessment of bone biopsies from rare bone diseases. Furthermore, additional information on comprehensive bone quality analyses and its impact for clinical practice is given as a perspective. Such techniques allowing for high-resolution analyses of osseous changes in combination with traditional bone histomorphometry represent a valued resource for the diagnosis of rare metabolic bone disorders and the refined prediction of bone fragility.
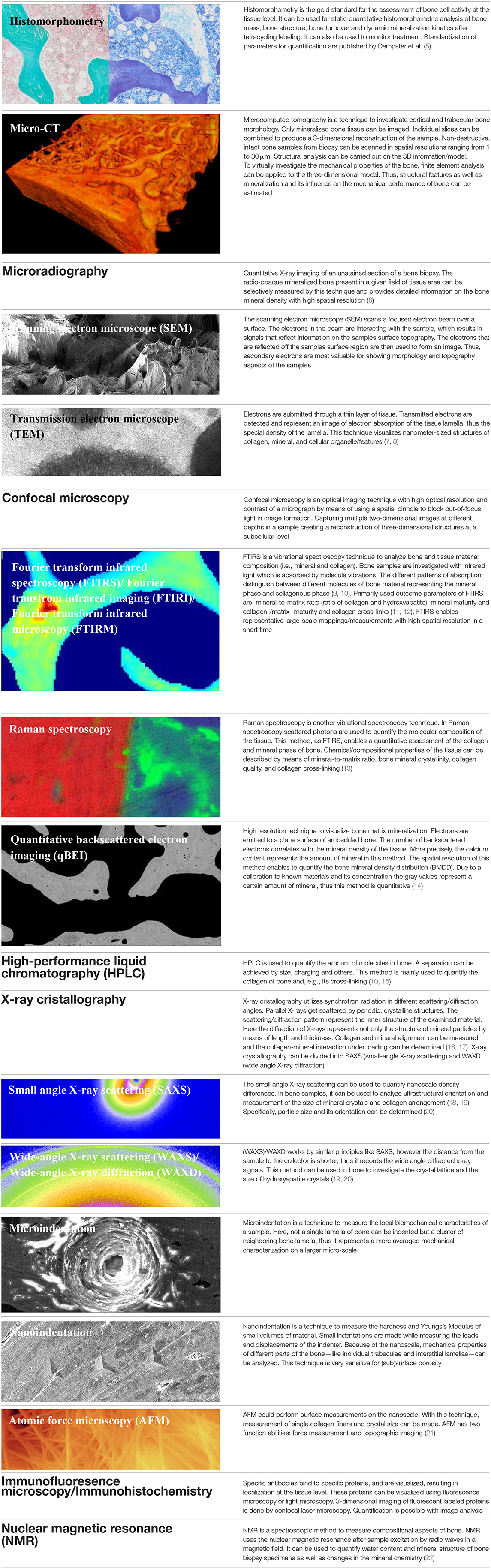
Table 1. Overview of different techniques which can be considered in bone biopsy analysis by clinicians and researchers.
Therefore, the aim of this review is to give an overview of various rare metabolic bone diseases and the possible use of advanced techniques to analyze bone quality in bone biopsies in the clinical setting.
Methods
Literature Search
Literature was systematically reviewed to identify publications on rare metabolic bone diseases and analyzing techniques for bone biopsies. The literature search was performed based on the Preferred Reporting Items for Systematic Reviews and Meta-Analyses (PRISMA)-statement. To identify all relevant publications a systematic search was conducted in the US National Library of Medicine National Institutes of Health (PubMed) for publications from inception to 2019 week 34. The following search terms were used: “(PLS3 mutation) OR (osteogenesis imperfecta) OR (osteopetrosis) OR (fibrodysplasia ossificans progressiva) OR (myositis ossificans) OR (osteosclerosis) OR (osteomalacia) OR (Ehlers-danlos syndrome) OR (fibrous dysplasia of bone) OR (fibrous dysplasia) OR (osteitis deformans) OR (paget disease) OR (morbus paget) OR (m. paget) OR (Loeys-dietz syndrome)” AND “(histology) OR (histochemistry) OR (histomorphometry) OR (Fourier transform infrared spectroscopy) OR (Fourier transform infrared imaging) OR (Raman spectroscopy) OR (Transmission electron microscopy) OR (Quantitative backscattered electron imaging) OR (Confocal microscopy) OR (Micro-CT) OR (contrast enhanced microCT) OR (Microindentation) OR (Nanoindentation) OR (High-performance liquid chromatography) OR (Atomic force microscopy) OR (Immunofluoresence microscopy) OR (Small-angle X-ray scattering) OR (Wide-angle X-ray scattering) OR (synchrotron) OR (“NMR”) OR (“Nuclear magnetic resonance”).”
Inclusion Criteria
The following inclusion criteria were used: (1) studies describing bone biopsies in the following rare metabolic bone disorders: Paget's disease of bone, osteogenesis imperfecta, fibrous dysplasia, fibrodysplasia ossificans progressiva, PLS3 X-linked osteoporosis, Ehlers-Danlos syndrome, Loeys-Diets syndrome, osteopetrosis; (2) study published as an original article; (3) adequate description of bone biopsy analysis; (4) studies were published in either English or Dutch; (5) full text availability; and (6) all types of study design.
Exclusion Criteria
The following exclusion criteria were uses: (1) studies focusing on animal models and (2) studies focusing on in vitro models.
Study Selection
A total of 1,114 papers were identified. All titles and thereafter remaining abstracts were screened for eligibility by two clinical researchers, ST and EE. In case of disagreement consensus was reached by dialogue. Articles were included when they described the analyzing techniques of bone biopsies in the listed rare metabolic bone disorders. A total of 141 studies were included for initial full text analysis. Forty-six studies were excluded when they did not report any results of the analyzed bone biopsies, described no bone biopsies in any of the listed rare metabolic bone diseases, or were used for in vitro studies (Figure 1).
Data Extraction
The extracted data included the following: (1) name of the first author; (2) year of publication; (3) journal; (4) number of patients with a bone biopsy; (5) aim of the article; (6) type of material (biopsy or residual); (7) preparation method; (8) technique of bone material analysis; (9) results.
Study Quality Assessment
Study quality assessment was performed on all articles. The quality assessment was independently conducted by two clinical researchers, ST and EE. In case of disagreement consensus was reached by dialogue. Thirty-two (34%) articles consisted of case reports. The remaining 63 articles were assessed using the Study Quality Assessment Tools created by the NHLBI (23). Two items were added to the tool: “Were the techniques used for analysis of bone biopsy material well-described?” and “Were the histomorphometric parameters well-described?”
Results
A final total of 95 studies were included in this review. Results of the quality assessment (NHLBI) of included studies showed that 10% of the studies were classified as poor, 73% as fair and 17% as good (23). An overview of the included studies and quality assessment is displayed as a supplement. Table 2 shows an overview of the different analysis techniques per rare metabolic disorder as found in the PubMed search.
Atomic force microscopy was not previously described in any of the rare metabolic bone diseases. There were also no studies found describing bone biopsies in Ehlers-Danlos syndrome. Some studies described other methods than the ones used in our PubMed search: microradiography, scanning electron microscopy, electron microscopy, X-ray diffraction, three-point-bending test, in situ fracture toughness test, immunohistochemistry, immunocytochemistry, and histochemistry. These articles were included in this review as well. In addition, Erdheim-Chester disease and Cherubism were included in the results.
Paget's Disease of Bone
Paget's disease is a chronic focal bone disorder of unknown cause, most commonly seen in elderly patients. The hallmark of this disorder is locally increased bone turnover with uncontrolled osteoclast activity, leading to the resorption of bone at specific skeletal sites. As a result of bone resorption and subsequent increase of osteoblast activity, overproduction of newly formed bone takes place. This newly formed bone is disorganized and has a different tissue composition in comparison to bone regions unaffected by Paget's disease of bone (24). Commonly affected areas are the skull, spine, pelvis, and long bones of the lower extremity. Most patients are asymptomatic; however, some patients suffer from pain and fracture risk can be increased. The diagnosis is primarily based on radiologic evaluation of lytic lesions or thickened cortices, accentuated trabeculae, and increased size of bones or by skeletal scintigraphy (25). Biochemical screening shows an elevated serum concentration of alkaline phosphatase in most patients.
Histological examination of lesions shows excessive numbers of multinucleated osteoclasts and abnormal deposition of primarily woven bone. The bone marrow contains an increased number of blood vessels and bone precursor cells (26).
Nineteen articles on Paget's disease were identified with the PubMed search (24, 27–44). Multiple articles described different immunological techniques in search of a viral origin in the disorder. Most of the articles were published before the year 2000. This may be the reason for which most articles mainly describe histomorphometric data. The study of Zimmermann et al. applied different techniques to characterize the composition, structure, and mechanical properties of Pagetic bone. Quantitative backscattered electron microscopy showed a lower degree of mineralization in agreement with the mineral-to-matrix ratio measured by Fourier transform infrared spectroscopy. Structural properties were analyzed by micro-CT, which showed replacement of the typical longitudinal-oriented Haversian canals by disorganized clusters of bone with high porosity. Also, the trabecular thickness was shown to be substantially higher in Pagetic bone. Mechanical properties were tested by nano-indentation, reference point indentation (micro-indentation), and in situ fracture toughness test. These measurements demonstrated a lower plasticity in Pagetic bone (24). Giannini et al. used SAXS and WAXS techniques to characterize the organization of mineral nanocrystals in one patient with Paget's disease. Results showed a disorganized structure of the nanocrystals (44).
Osteogenesis Imperfecta
Osteogenesis imperfecta (OI) is an inherited disorder which is mainly characterized by increased risk of fractures, blue sclerae, dentinogenesis imperfect, and hearing loss. OI is most commonly caused by mutations in the COL1A1 or COL1A2 genes (coding for type I collagen molecules). Type I collagen is the main structural protein in bone, building the mineralizing matrix. OI is subdivided in types I–V, depending on the type and severity of clinical symptoms (45). The production of type I collagen by osteoblasts is abnormal or decreased in patients with OI. In addition to the altered structure, there is hypermineralization of the bone matrix, independent of the type and severity of the disease (46, 47). Histological examination showed low cancellous bone volume. Lamellae appear thinner and less smooth than in healthy people. Histological distinction between OI and other osteoporotic bone disorders by histomorphometric analysis is difficult (48, 49). The diagnosis of OI is based on the described clinical characteristics, radiological findings, and genetic testing.
OI is the most commonly studied rare metabolic bone disorder in our PubMed search with a total of 45 included articles (48, 50–92). This is also the disorder in which high-resolution techniques were employed for in-depth analyses. In particular, quantitative backscattered electron imaging (qBEI), and nanoindentation have been used in multiple studies. Bone mineral density distribution measured by qBEI was increased in different types of OI (58, 59, 61, 62, 68, 69, 76, 83, 90). Only the article by Webb et al. described several OI type XIV patients in which qBEI showed a normal bone mineral density distribution, with the exception of one patient with a higher BMDD compared to healthy controls. This patient had a very low bone turnover (82). In accordance to the high BMDD values found with qBEI, Fratzl-Zelman et al. studied the mineral particle size in children with OI type I with SAXS/WAXD technique. They concluded that high BMDD in children with OI type I is due to increased particle packing density and not to increased particle size (90). Nanoindentation showed a lower Young's modulus in OI in comparison to control bone (50, 53). Comparison between different clinical types of OI was studied. Albert et al. described a higher elastic modulus and hardness in OI type I compared to OI type III (51). Fan et al. showed no significant difference in Young's modulus and hardness between OI type III and OI type IV (52). The data of Weber et al. showed no effect of pamidronate treatment on elastic modulus and hardness (83). In the preliminary study by Abidin et al., the researchers used synchrotron tomography to characterize the 3D structure of cortical bone in children with OI. With these cortical characteristics they successfully performed a machine learning task to distinguish between OI and healthy bone. They conclude that this technique could potentially be used as a future biomarker to detect and quantify the severity of OI, respectively (91).
Fibrous Dysplasia
Fibrous dysplasia (FD) is a disorder in which parts of bone are replaced by fibrous connective tissue and trabecular bone of poor quality. The disease is caused by a mutations in the GNAS1 gene and symptoms commonly present during the second decade of life. It may occur as a single lesion (monostotic) or at multiple sites of the skeleton (polyostotic). A specific polyostotic form is also known as McCune-Albright syndrome (MAS), which is accompanied by skin pigmentation and endocrinopathies. Commonly affected sites are the long bones, ribs, pelvis and the craniofacial skeleton. The GNAS1 gene mutation leads to overproduction of cAMP in the osteoblastic cell lineage which in turn leads to increased proliferation and abnormal differentiation of osteoblastic cells. Most patients with FD are asymptomatic and FD is often an incidental finding on routine X-ray examination. In some patients, the enlargement and distortion of bone lesion(s) can cause pain, swelling, bone deformity, or pathological fractures, which are the main symptoms of FD (93). CT or MRI scans are used to determine the extensiveness of the lesion(s). For the final diagnosis histological examination is required.
A total of nine studies on FD/MAS were identified during our search (94–102). Three out of the nine studies were case-reports. In addition to standardized histomorphometry different study designs included the use of quantitative backscattered electron microscopy (qBEI), immunohistochemistry, and confocal microscopy. A lower mineral content was seen in the more severely affected FD patients measured by qBEI (94). Two immunohistochemical studies were performed to obtain a better understanding of the disease. The most remarkable findings included high expression of c-fos and c-jun in fibroblast-like cells, a negative response to nuclear antigen of cellular proliferation (PCNA), and a positive response to AgNOR (97, 102). The case report by Boyce et al. is the only study reporting the use of immunohistochemistry to evaluate the effect of denosumab treatment on the expression of RANKL. However, the biopsies before and after treatment were not of sufficient quality to compare RANKL expression (100). Two articles describing the use of confocal laser scanning microscopy report the finding of “Sharpey's fibers” in fibrous dysplastic bone (98, 99). The most recent article from 2015 by Laino et al. suggested confocal laser scanning microscopy as a useful tool to investigate FD lesions (99).
Fibrodysplasia Ossificans Progressiva
Fibrodysplasia ossificans progressiva (FOP) is a very rare autosomal inherited disorder caused by a mutation in the ACVR1/ALK2 gene (103). FOP is a clinical diagnosis, confirmed by genetic testing. FOP is characterized by congenital malformation of the great toes and progressive heterotopic ossification of muscles, tendons, and ligaments. Heterotopic ossification is induced by flare-ups (painful inflammatory swelling of soft tissue) (104). Nowadays diagnostic bone biopsies are not performed because of the severe risk of new heterotopic bone formation. Histological examination of bone biopsies in FOP patients has been however performed without awareness of the disease in the past. These patients lacked FOP diagnosis and genetic testing was not yet available.
Only three articles were found describing bone biopsies in FOP (105–107). Kaplan et al. performed a study during the early ‘90s to investigate bone properties by using histomorphometry and immunohistochemistry (105). Histologically, lesions with HO formation appear to be similar to original skeletal bone with regard to lamellar bone formation and resorption and marrow elements (105). In later studies by Piram et al. (106) and Ibarra et al. (107), FOP was identified by coincidence, as the acquired biopsies ruled out malignant disease.
PLS3 X-Linked Osteoporosis
Mutations in the actin-binding protein plastin-3 (PLS3) gene have recently been discovered as a rare cause of monogenetic osteoporosis which is usually reported to present without syndromic features. Due to the X-linked pattern of inheritance, homozygous male patients suffer from fractures from early childhood. Heterozygous women have a milder presentation in age of onset and fracture risk (108). DXA measurements show low BMD values in affected patients. Genetic analysis is needed for the final diagnosis. The cellular functions of PLS3 protein in bone and the pathogenic mechanism causing monogenetic osteoporosis are not well-understood. PLS3 is expressed in almost all cell types, including osteoclasts and osteocytes. It is possible that PLS3 mutations, which affect the function or expression level of PLS3 protein in the osteocyte dendrites, lead to altered mechanosensitivity of these osteocytes.
The literature search yielded six articles, which included a bone biopsy analysis (109–114). All articles except one are case reports or case series describing phenotypical presentation of the PLS3 mutation. Because of the disease rarity patient numbers are very low in all articles, with a maximum of five. Histomorphometric analysis showed a lamellar pattern of cortical and trabecular bone. The amount of trabecular bone is severely reduced and bone formation and resorption parameters are low. Quantitative backscattered electron microscopy showed conflicting results; the study by Fahiminiya et al. (110) described bone mineralization density distribution within the normal range, Balasubramanian et al. (114) showed a hypermineralization distribution and Kampe et al. (115) showed hypomineralization.
Loeys-Diets Syndrome
Loeys-Diets syndrome (LDS) is an autosomal dominant disorder, due to a mutation in the TGFBR1/2-gene. The most typical symptoms are arterial aneurysms, hypertelorism, and bifid uvula or cleft palate. There is a wide variety of clinical presentation with involvement of other organ systems, including the skeleton. Most common skeletal findings are pectus excavatum or carinatum, joint laxity, arachnodactyly, scoliosis, and cervical spine malformation (116, 117). Also, osteoporosis with increased fracture risk has been described (118). The diagnosis of LDS is based on the variety of clinical symptoms and confirmed by genetic testing. TGF-β regulates mechanical properties and bone matrix composition (119). Dysregulation of TGF-β signaling due to the effect of the mutation in TGFBR1/2 and its effect on bone metabolism are poorly understood.
PubMed search identified only one article. The case-series of Ben Amor et al. reported bone histomorphometric and bone material observations in two patients. Histomorphometric findings of thin cortices and high bone turnover without mineralization defects were reported. Quantitative backscattered electron microscopy was used in this case series which showed elevated matrix mineralization on the level of individual trabeculae (120).
Osteopetrosis
Osteopetrosis is a heterogeneous group of disorders characterized by diffuse skeletal sclerosis and high bone mineral density caused by different mutations. There is a wide variety in severity of the disease ranging from asymptomatic to fatal symptoms during childhood. Most patients have an increased fracture risk due to the lack of bone flexibility. X-rays shows a dense appearance of the skeleton. Osteopetrosis is caused by decreased osteoclastic bone resorption due to failure of differentiation or function of the osteoclastic cells. The expansion of bone may cause nerve compression and hematological dysfunction due to reduction of the bone marrow cavity by central bone expansion (121, 122).
A total of six articles were identified (123–128). Histomorphometry showed multinucleated osteoclast-like cells. Four out of this, six articles described the use of transmission electronic microscopy (TEM). The most striking finding of TEM is the absence of ruffled borders and clear zones at sites of bone resorption. The article by Satomura et al. is of recent date (2007), whereas the other articles are written before the year 2000 (123, 124, 127, 128). The primary aim of all studies was focused on improved comprehension of the bone properties associated with the disease.
In addition to the stated rare metabolic bone disorders, our literature search also revealed some other rare bone disorders. Three articles described immunohistochemistry in Erdheim-Chester disease (ECD) (129–131). ECD is a disorder characterized by multi-organ infiltration of non-Langherhans' histiocytes in middle-aged patients. In almost all cases of ECD, the long bones are affected, and half of the patients have extra-skeletal involvement (132). ECD is often challenging to diagnose because of a variety of symptoms. Bone pain of the distal extremities are most often present. Histologic examination of skin or bone is required to establish the diagnosis. All the included articles were case reports or case series. It is striking that only the article by Rivera et al. described histomorphometric data (129). All three articles described findings of CD68 positive and S-100 negative histocytes. The other rare metabolic bone disorder found in the PubMed search is Cherubism. Cherubism is characterized by a bilateral swelling of the cheek caused by fibro-osseus lesions of the mandibular or maxillary bone. Age of onset is usually during childhood and sometimes the lesion regress as the child grows (133). Radiological findings shows regions of a “spongy” appearance of the maxilla and mandibular. Three articles about Cherubism described histomorphometric and immunohistochemistry data (134–136). Histopathological examination showed fibrous tissue in the lesions with multinucleated giant cells. Immunohistochemistry revealed CD68 positive multinucleated giant cells and OPG and RANKL expression.
Discussion
This review provides an overview of the application of advanced techniques in bone biopsies of rare metabolic bone disorders and an outlook on the consideration of advanced techniques. These advanced techniques may help to resolve many gaps in the knowledge of general bone metabolism and the pathophysiology of many different rare metabolic bone disorders. Though, it has to be mentioned that histomorphometric analysis is still the most widely used method. Application of techniques focusing on structural and compositional aspects of bone could provide much more information and make a bone biopsies an even more valuable tool in diagnosis and treatment guidance. In this review, we described a variety of advanced techniques, many of which have rarely been used in clinical practice.
Clinical utility depends on several aspects of the introduction of additional technique. The information obtained from advanced techniques should refine the definition of skeletal status, predict future skeletal complications, or guide treatment in order to be of clinical value. Reference values should be available for informed decision making. The techniques should be (widely) available in diagnostic laboratories. Application of the technique should preserve its properties to allow its use in other diagnostic tools including histology/histomorphometry.
It is important to realize that some of the advanced techniques require special processing, which can hinder the usage of other methods or even make other methods impossible to use. This means that not all techniques are applicable on the same biopsy specimen. Techniques for testing mechanical properties often cause damage to the biopsy specimen by breaking it or causing surface damage. Specimen used for HPLC will be completely destroyed. Standard fixation using formaldehyde 3.5% may cause problems when immune histology assays are performed. TEM may need a different (epoxy) embedding method than acrylate for histology and HPLC may be best performed on untreated samples. Most articles combined one of the advanced analyzing techniques with histomorphometry. Only a limited amount of articles combined more than one advanced analyzing techniques in their research.
Because of the differences in processing, it will be of great importance that doctors provide the biopsy specimen with sufficient comprehensive clinical data and the specified research question to make the right choice for analyzing techniques. Starting at the bed site, a close cooperation of clinicians and researchers is needed to correctly process the biopsy for a maximum gain of information by a variety of different applied methods.
As already mentioned in the introduction of this article, most advanced analysis techniques are currently only used for research purposes only. Although some techniques are available already for many years, reference data does not always exist due to the lack of proper control groups, thus a sufficient comparison to healthy cases is lacking. This is a problem especially in the group of rare metabolic bone diseases, but also in the more common metabolic bone disorders, such as osteoporosis, where treatment-naive age-matched controls are also very limited. Reference data for adults and children have been published for bone mineral density distribution (BMDD) assessed by quantitative backscattered electron imaging (qBEI) (137, 138). Such reference data will ideally come from bone biopsies of a healthy population. Collection of these reference data will be challenging since a bone biopsy is an invasive procedure and cannot routinely be applied to healthy persons. In addition, reference data will differ between adults and children. Certainly, in (young) children ethical justification has to be considered to collect any type of reference data. A feasible option for collecting reference data from a healthy population is the use of residual material after routine surgical intervention.
Different rare metabolic bone diseases will require different methods, since they have different tissue characteristics. Rare metabolic bone disorders can roughly be divided in three groups: disorders with osteoblast dysfunction, disorders with osteoclast dysfunction, and the group of remaining disorders.
Osteoblastic dysfunction is seen in OI and fibrous dysplasia. In this group, a broad spectrum of advanced techniques could be valuable. Techniques to measure structural properties could be effective, especially in the group of OI. Confocal microscopy of polarized light microscopy could give advantageous information about the abnormal collagen fiber organization in OI or arrangement of fibrous tissue in FD. Micro-CT analysis could be of potential interest to explore the microstructural patterns compared to healthy groups. These results could provide critical insight in the interpretation of bone mineral density measurements of a DXA-scan. Also, techniques to measure material composition can potentially be of high scientific value, e.g., FTIR-spectroscopy, Raman-spectroscopy or qBEI. SAXS/WAXS technique could be of beneficial to monitor changes in mineral structure and alignment after treatment of OI patients. In OI, most of these techniques have already been tested and showed promising results. In FD, besides qBEI, none of the other techniques have been applied yet but we believe that this review will steer clinicians toward this direction, which is an effort to gain more information about the composition of the affected bone. Of course, the focal nature of the disease is a complicating factor since the bone specimen needs to be taken from an FD lesion. In OI, the mechanical properties of bone are affected as well. Testing of these properties could help the prediction of fracture risk.
Osteoclast dysfunction is seen in Paget's disorder, PLS3 X-linked osteoporosis, and osteopetrosis. Although the affected material and mechanical parameters of bone share common characteristics, there are distinct differences. Mineral composition and structure quantifying methods such as qBEI, FTIR-spectroscopy, Raman-spectroscopy, or SAXS/WAXS may be a valuable tools to investigate the diversity of the mineral apposition, distribution, density and structure in each disease. Potentially valuable techniques are micro- and nano-indentation for the measurement of the local mechanical alteration of the bone material caused by a changed structure and composition. This has hardly been done in any of the disorder with osteoclast dysfunction. We must take into account that also the Paget's disease of bone is a focal disorder and bone samples should be obtained in the regions of interest, which are not always justifiably reachable for biopsy. PLS3 X-linked osteoporosis is a relatively new discovery with still many uncertainties about the pathophysiological mechanism.
The remaining disorders are fibrodysplasia ossificans progressiva, Loeys-Dietz syndrome, Erdheim-Chester disease, and Cherubism. In all of these disorders, a scarce number of techniques have been tested and a lot of uncertainties about their pathophysiology exist. Analysis of structural properties and material properties will contribute to a higher level of knowledge of the disorders. In FOP, the availability of bone material for research is very low due to the rarity of the disease and the lack of biopsies or surgeries performed. Biopsies or surgery can induce heterotopic bone formation. Postmortem studies are expected to be helpful to optimize a large set of diagnostic methods and gain a deeper understanding of the disorder.
Immunological techniques have been used in the past to explore the possibility of a viral origin of some rare bone disorders. However, no such origin has been found yet. Immunohistology could be in these cases beneficial in identifying critical components of signaling and interaction between osteocytes, osteoblasts, and osteoclasts. Immunohistochemistry could also be helpful in guiding treatment especially with the new developments in biologicals. For instance, detection of RANKL by IHC in a bone biopsy of osteogenesis imperfecta, may indicate denosumab treatment could be beneficial.
The use of advanced techniques is not limited to their application in research and diagnosis; in the future they also hold great promise in the design and evaluation of treatment in patient follow-up. Firstly, techniques providing information on material properties might be able to predict fracture risk, which can be useful to differentiate the patients in need for treatment. Secondly, advanced techniques could be of great advantage to the patient and the clinician, by providing insight in the pathophysiology and giving guidance to treatment strategy. Thirdly, techniques that give insight in mechanisms by which various therapeutic drugs work, at the individual level might help in follow-up. Only a limited amount of papers discussed the use of the advanced techniques to investigate the effect of treatment. This is potentially due to the fact that two biopsies are needed, pre- and post-treatment.
Despite the development of analysis techniques for bone biopsies, innovative less invasive techniques have an obvious benefit and are thus highly sought to complement the diagnosis of metabolic bone disorders now and in the future. The measurement of biochemical bone turnover markers (BMTs) in blood serum or urine has a central role in the diagnostic process and follow-up of rare metabolic bone disorder for many years. New bone turnover markers were developed and measurements became more accurate. However, there is biological variability in BMTs due to age, sex, physical activity, recent fractures, or intercurrent disease (139). Due to these factors, interpretation of BMTs in rare metabolic bone disorders can be difficult. High-resolution peripheral quantitative computed tomography (HR-pQCT) is a new technique to detect microstructural properties at the radius and tibia (140). This non-invasive method uses a very low dose of radiation and is possible to use on a regular basis for follow-up (141, 142). Reference point indentation is a new tool to measure mechanical properties in vivo. Microindentations are made by a hand-held device. Although no bone biopsy is needed for this technique, patients still need local anesthetic because the indentations are painful due to penetration of the skin and periosteum (143). Both techniques are mostly used for the follow-up of more prevalent diseases such as osteoporosis; whether they are useful in the diagnosis of rare metabolic bone disorders needs further investigation.
There were several limitations in this review. The described metabolic bone diseases are rare, which could explain the low number of published articles on this topic. It could be possible that some articles were missed because the advanced analyzing techniques were not described in the title or abstract. We only used the PubMed database for data collection. The majority of the papers included descriptions of histomorphometric results. The number of patients enrolled in most of the studies was very low, especially in the papers describing techniques other than histomorphometry. Even when the number of patients in a study was sufficient, most of the time only a small number of patients underwent a bone biopsy. Also, the quality of the articles differed. One-third of the included studies consisted of case reports, with sometimes limited information. In FOP, PLS3 X-linked osteoporosis, LD, and ECD the majority of the articles consisted of case reports. Also, 10% of the remaining articles were of poor quality (23). However, we decided to not exclude any of these articles. Given the rarity of the disorders, also these articles can help in providing an overview of performed research on bone biopsies with advanced techniques. Description of the used techniques and processing of the biopsy specimens for analysis were not always adequately.
Striking is the lack of articles where advanced techniques have been applied to study rare metabolic bone disorders with a high bone mineral density. Only a few articles describing osteopetrosis were found. Van Buchem disease was not covered in our search, an extra search for this disorder revealed no articles. Application of advanced techniques to disorders with a high bone mineral density could be valuable to accurately describe the full range of bone mineral disorders.
Notwithstanding the relatively limited amount of publications and patients, this review offers valuable insights into possible applications of various analyzing techniques.
Larger studies must establish a reliable database. Using this database, clinicians would be able to assess the need for treatment for the underlying disease as well as monitor the success of the therapy. MicroCT as well as spectroscopy and scanning electron microscopy (e.g., qBEI) are of great importance, as they link research and clinical routine. A further advantage of these techniques is the sample volume saving preparational approach. A combination of the modern techniques and classical pathological histology and histomorphometry has the potential to lead to an improved new, individualized, patient-centered therapy.
This review shows the possibilities for a broader use of bone material obtained from biopsies or residual material after surgery. These advanced techniques could provide important additional information to the new imaging techniques developed in recent years. The use of advanced analyses techniques can provide a better understanding of the pathophysiology and pave the way for new treatment options ultimately aiming optimal patient recovery and prevention of skeletal complications in the rare bone diseases.
Data Availability Statement
All datasets generated for this study are included in the article/Supplementary Material.
Author Contributions
Study design: ST, EE, BB, and NB. Study conduct: ST, EE, FS, BB, and NB. Data collection: ST, EE, FS, BB, and NB. Data analysis: ST, EE, FS, BB, and NB. Data interpretation: ST, EE, FS, DM, BB, and NB. Drafting manuscript: ST, EE, FS, BB, and NB. Revising manuscript content: ST, EE, FS, DM, BB, and NB. Approving final version of manuscript: ST, EE, FS, DM, BB, and NB take responsibility for the integrity of the data analysis. All authors contributed to the article and approved the submitted version.
Conflict of Interest
The authors declare that the research was conducted in the absence of any commercial or financial relationships that could be construed as a potential conflict of interest.
Supplementary Material
The Supplementary Material for this article can be found online at: https://www.frontiersin.org/articles/10.3389/fendo.2020.00399/full#supplementary-material
References
1. Parapia LA. Trepanning or trephines: a history of bone marrow biopsy. Br J Haematol. (2007) 139:14–9. doi: 10.1111/j.1365-2141.2007.06749.x
2. Sacker LS, Nordin BE. A simple bone biopsy needle. Lancet. (1954) 266:347. doi: 10.1016/S0140-6736(54)91095-8
3. Recker RR, Kimmel DB, Dempster D, Weinstein RS, Wronski TJ, Burr DB. Issues in modern bone histomorphometry. Bone. (2011) 49:955–64. doi: 10.1016/j.bone.2011.07.017
4. Parfitt AM, Drezner MK, Glorieux FH, Kanis JA, Malluche H, Meunier PJ, et al. Bone histomorphometry: standardization of nomenclature, symbols, and units. Report of the ASBMR Histomorphometry Nomenclature Committee. J Bone Miner Res. (1987) 2:595–610. doi: 10.1002/jbmr.5650020617
5. Dempster DW, Compston JE, Drezner MK, Glorieux FH, Kanis JA, Malluche H, et al. Standardized nomenclature, symbols, and units for bone histomorphometry: a 2012 update of the report of the ASBMR Histomorphometry Nomenclature Committee. J Bone Miner Res. (2013) 28:2–17. doi: 10.1002/jbmr.1805
6. Farlay D, Bala Y, Rizzo S, Bare S, Lappe JM, Recker R, et al. Bone remodeling and bone matrix quality before and after menopause in healthy women. Bone. (2019) 128:115030. doi: 10.1016/j.bone.2019.08.003
7. Georgiadis M, Muller R, Schneider P. Techniques to assess bone ultrastructure organization: orientation and arrangement of mineralized collagen fibrils. J R Soc Interface. (2016) 13:20160088. doi: 10.1098/rsif.2016.0088
8. Milovanovic P, Zimmermann EA, Vom Scheidt A, Hoffmann B, Sarau G, Yorgan T, et al. The formation of calcified nanospherites during micropetrosis represents a unique mineralization mechanism in aged human bone. Small. (2017) 13. doi: 10.1002/smll.201602215
9. Paschalis EP. Fourier transform infrared imaging of bone. Methods Mol Biol. (2019) 1914:641–9. doi: 10.1007/978-1-4939-8997-3_34
10. Schmidt FN, Zimmermann EA, Campbell GM, Sroga GE, Puschel K, Amling M, et al. Assessment of collagen quality associated with non-enzymatic cross-links in human bone using Fourier-transform infrared imaging. Bone. (2017) 97:243–51. doi: 10.1016/j.bone.2017.01.015
11. Paschalis EP, Mendelsohn R, Boskey AL. Infrared assessment of bone quality: a review. Clin Orthop Relat Res. (2011) 469:2170–8. doi: 10.1007/s11999-010-1751-4
12. Boskey A, Pleshko Camacho N. FT-IR imaging of native and tissue-engineered bone and cartilage. Biomaterials. (2007) 28:2465–78. doi: 10.1016/j.biomaterials.2006.11.043
13. Morris MD, Mandair GS. Raman assessment of bone quality. Clin Orthop Relat Res. (2011) 469:2160–9. doi: 10.1007/s11999-010-1692-y
14. Roschger P, Fratzl P, Eschberger J, Klaushofer K. Validation of quantitative backscattered electron imaging for the measurement of mineral density distribution in human bone biopsies. Bone. (1998) 23:319–26. doi: 10.1016/S8756-3282(98)00112-4
15. Saito M, Marumo K. Collagen cross-links as a determinant of bone quality: a possible explanation for bone fragility in aging, osteoporosis, and diabetes mellitus. Osteoporos Int. (2010) 21:195–214. doi: 10.1007/s00198-009-1066-z
16. Schmidt FN, Zimmermann EA, Walsh F, Plumeyer C, Schaible E, Fiedler IAK, et al. On the origins of fracture toughness in advanced teleosts: how the swordfish sword's bone structure and composition allow for slashing under water to kill or stun prey. Adv Sci. (2019) 6:1900287. doi: 10.1002/advs.201900287
17. Zimmermann EA, Schaible E, Gludovatz B, Schmidt FN, Riedel C, Krause M, et al. Intrinsic mechanical behavior of femoral cortical bone in young, osteoporotic and bisphosphonate-treated individuals in low- and high energy fracture conditions. Sci Rep. (2016) 6:21072. doi: 10.1038/srep21072
18. Fratzl P, Schreiber S, Klaushofer K. Bone mineralization as studied by small-angle x-ray scattering. Connect Tissue Res. (1996) 34:247–54. doi: 10.3109/03008209609005268
19. Pabisch S, Wagermaier W, Zander T, Li C, Fratzl P. Imaging the nanostructure of bone and dentin through small- and wide-angle X-ray scattering. Methods Enzymol. (2013) 532:391–413. doi: 10.1016/B978-0-12-416617-2.00018-7
20. Wagermaier W, Gourrier A, Aichmayer B. Understanding Hierarchy and Functions of Bone Using Scanning X-ray Scattering Methods. (2013). doi: 10.1039/9781849737555-00046
21. Thurner PJ. Atomic force microscopy and indentation force measurement of bone. Wiley Interdiscip Rev Nanomed Nanobiotechnol. (2009) 1:624–49. doi: 10.1002/wnan.56
22. Donnelly E. Methods for assessing bone quality: a review. Clin Orthop Relat Res. (2011) 469:2128–38. doi: 10.1007/s11999-010-1702-0
23. NHLBI. Study Quality Assessment Tools. Available online at: https://www.nhlbi.nih.gov/health-topics/study-quality-assessment-tools
24. Zimmermann EA, Köhne T, Bale HA, Panganiban B, Gludovatz B, Zustin J, et al. Modifications to nano- and microstructural quality and the effects on mechanical integrity in Paget's disease of bone. J Bone Miner Res. (2015) 30:264–73. doi: 10.1002/jbmr.2340
25. Cundy T. Paget's disease of bone. Metab Clin Exp. (2018) 80:5–14. doi: 10.1016/j.metabol.2017.06.010
26. Singer FR. Bone Quality in Paget's disease of bone. Curr Osteoporos Rep. (2016) 14:39–42. doi: 10.1007/s11914-016-0303-6
27. Siris E, Weinstein RS, Altman R, Conte JM, Favus M, Lombardi A, et al. Comparative study of alendronate versus etidronate for the treatment of Paget's disease of bone. J Clin Endocrinol Metab. (1996) 81:961–7. doi: 10.1210/jcem.81.3.8772558
28. Delmas PD, Chapuy MC, Vignon E, Charhon S, Briancon D, Alexandre C, et al. Long term effects of dichloromethylene diphosphonate in Paget's disease of bone. J Clin Endocrinol Metab. (1982) 54:837–44. doi: 10.1210/jcem-54-4-837
29. Fraser TR, Ibbertson HK, Holdaway IM, Rutland M, King A, Dodd G, et al. Effective oral treatment of severe Paget's disease of bone with APD (3-amino-1-hydroxypropylidene-1,1-bisphosphonate); a comparison with combined calcitonin + EHDP (1-hydroxyethylidene-1,1-bisphosphonate). Aust N Z J Med. (1984). 14:811–8. doi: 10.1111/j.1445-5994.1984.tb03778.x
30. Gutteridge DH, Gruber HE, Kermode DG, Worth GK. Thirty cases of concurrent Paget's disease and primary hyperparathyroidism: sex distribution, histomorphometry, and prediction of the skeletal response to parathyroidectomy. Calcif Tissue Int. (1999) 65:427–35. doi: 10.1007/s002239900728
31. Helfrich MH, Hobson RP, Grabowski PS, Zurbriggen A, Cosby SL, Dickson GR, et al. A negative search for a paramyxoviral etiology of Paget's disease of bone: molecular, immunological, and ultrastructural studies in UK patients. J Bone Miner Res. (2000) 15:2315–29. doi: 10.1359/jbmr.2000.15.12.2315
32. Ingram RT, Collazo-Clavell M, Tiegs R, Fitzpatrick LA. Paget's disease is associated with changes in the immunohistochemical distribution of noncollagenous matrix proteins in bone. J Clin Endocrinol Metab. (1996) 81:1810–20. doi: 10.1210/jcem.81.5.8626840
33. Khairi MR, Altman RD, DeRosa GP, Zimmermann J, Schenk RK, Johnston CC. Sodium etidronate in the treatment of Paget's disease of bone. A study of long-term results. Ann Intern Med. (1977) 87:656–63. doi: 10.7326/0003-4819-87-6-656
34. Lauffenburger T, Olah AJ, Dambacher MA, Guncaga J, Lentner C, Haas HG. Bone remodeling and calcium metabolism: a correlated histomorphometric, calcium kinetic, and biochemical study in patients with osteoporosis and Paget's disease. Metab Clin Exp. (1977) 26:589–606. doi: 10.1016/0026-0495(77)90081-6
35. Meunier PJ. Bone histomorphometry and skeletal distribution of Paget's disease of bone. Semin Arthritis Rheum. (1994) 23:219–21. doi: 10.1016/0049-0172(94)90036-1
36. Meunier PJ, Chapuy MC, Delmas P, Charhon S, Edouard C, Arlot M. Intravenous disodium etidronate therapy in Paget's disease of bone and hypercalcemia of malignancy. Effects on biochemical parameters and bone histomorphometry. Am J Med. (1987) 82:71–8. doi: 10.1016/0002-9343(87)90489-X
37. Meunier PJ, Coindre JM, Edouard CM, Arlot ME. Bone histomorphometry in Paget's disease. Quantitative and dynamic analysis of pagetic and nonpagetic bone tissue. Arthritis Rheum. (1980) 23:1095–103. doi: 10.1002/art.1780231005
38. Mills BG, Singer FR, Weiner LP, Suffin SC, Stabile E, Holst P. Evidence for both respiratory syncytial virus and measles virus antigens in the osteoclasts of patients with Paget's disease of bone. Clin Orthop Relat Res. (1984) 183:303–11. doi: 10.1097/00003086-198403000-00044
39. Ralston SH, Boyce BF, Cowan RA, Fogelman I, Smith ML, Jenkins A, et al. The effect of 1 alpha-hydroxyvitamin D3 on the mineralization defect in disodium etidronate-treated Paget's disease–a double-blind randomized clinical study. J Bone Miner Res. (1987) 2:5–12. doi: 10.1002/jbmr.5650020103
40. Seitz S, Priemel M, Zustin J, Beil FT, Semler J, Minne H, et al. Paget's disease of bone: histologic analysis of 754 patients. J Bone Miner Res. (2009) 24:62–9. doi: 10.1359/jbmr.080907
41. Tari AS, Fard MA. Solitary orbital Paget disease: a case report. Orbit. (2010) 29:219–21. doi: 10.3109/01676830.2010.485717
42. Basle MF, Russell WC, Goswami KK, Rebel A, Giraudon P, Wild F, et al. Paramyxovirus antigens in osteoclasts from Paget's bone tissue detected by monoclonal antibodies. J Gen Virol. (1985) 66:2103–10. doi: 10.1099/0022-1317-66-10-2103
43. Rebel A, Basle M, Pouplard A, Malkani K, Filmon R, Lepatezour A. Bone tissue in Paget's disease of bone. Ultrastructure and Immunocytology. Arthritis Rheum. (1980) 23:1104–14. doi: 10.1002/art.1780231006
44. Giannini C, Siliqi D, Bunk O, Beraudi A, Ladisa M, Altamura D, et al. Correlative light and scanning X-ray scattering microscopy of healthy and pathologic human bone sections. Sci Rep. (2012) 2:435. doi: 10.1038/srep00435
45. Van Dijk FS, Sillence DO. Osteogenesis imperfecta: clinical diagnosis, nomenclature and severity assessment. Am J Med Genet A. (2014) 164A:1470–81. doi: 10.1002/ajmg.a.36545
46. Fratzl-Zelman N, Misof BM, Klaushofer K, Roschger P. Bone mass and mineralization in osteogenesis imperfecta. Wien Med Wochensch. (2015) 165:271–7. doi: 10.1007/s10354-015-0369-2
47. Bishop N. Bone Material properties in osteogenesis imperfecta. J Bone Miner Res. (2016) 31:699–708. doi: 10.1002/jbmr.2835
48. Rauch F, Travers R, Parfitt AM, Glorieux FH. Static and dynamic bone histomorphometry in children with osteogenesis imperfecta. Bone. (2000) 26:581–9. doi: 10.1016/S8756-3282(00)00269-6
49. Rauch F, Travers R, Norman ME, Taylor A, Parfitt AM, Glorieux FH. Deficient bone formation in idiopathic juvenile osteoporosis: a histomorphometric study of cancellous iliac bone. J Bone Miner Res. (2000) 15:957–63. doi: 10.1359/jbmr.2000.15.5.957
50. Imbert L, Aurégan J-C, Pernelle K, Hoc T. Mechanical and mineral properties of osteogenesis imperfecta human bones at the tissue level. Bone. (2014) 65:18–24. doi: 10.1016/j.bone.2014.04.030
51. Albert C, Jameson J, Toth JM, Smith P, Harris G. Bone properties by nanoindentation in mild and severe osteogenesis imperfecta. Clin Biomech. (2013) 28:110–6. doi: 10.1016/j.clinbiomech.2012.10.003
52. Fan Z, Smith PA, Harris GF, Rauch F, Bajorunaite R. Comparison of nanoindentation measurements between osteogenesis imperfecta Type III and Type IV and between different anatomic locations (femur/tibia versus iliac crest). Connect Tissue Res. (2007) 48:70–5. doi: 10.1080/03008200601090949
53. Fan Z, Smith PA, Eckstein EC, Harris GF. Mechanical properties of OI type III bone tissue measured by nanoindentation. J Biomed Mater Res A. (2006) 79:71–7. doi: 10.1002/jbm.a.30713
54. Cassella JP, Barrie PJ, Garrington N, Ali SY. A Fourier transform infrared spectroscopic and solid-state NMR study of bone mineral in osteogenesis imperfecta. J Bone Miner Metab. (2000) 18:291–6. doi: 10.1007/PL00010645
55. Albert C, Jameson J, Smith P, Harris G. Reduced diaphyseal strength associated with high intracortical vascular porosity within long bones of children with osteogenesis imperfecta. Bone. (2014) 66:121–30. doi: 10.1016/j.bone.2014.05.022
56. Albert C, Jameson J, Tarima S, Smith P, Harris G. Macroscopic anisotropic bone material properties in children with severe osteogenesis imperfecta. J Biomech. (2017) 64:103–11. doi: 10.1016/j.jbiomech.2017.09.003
57. Baron R, Gertner JM, Lang R, Vignery A. Increased bone turnover with decreased bone formation by osteoblasts in children with osteogenesis imperfecta tarda. Pediatr Res. (1983) 17:204–7. doi: 10.1203/00006450-198303000-00007
58. Blouin S, Fratzl-Zelman N, Glorieux FH, Roschger P, Klaushofer K, Marini JC, et al. Hypermineralization and high osteocyte lacunar density in osteogenesis imperfecta type V bone indicate exuberant primary bone formation. J Bone Miner Res. (2017) 32:1884–92. doi: 10.1002/jbmr.3180
59. Cundy T, Dray M, Delahunt J, Hald JD, Langdahl B, Li C, et al. Mutations that alter the carboxy-terminal-propeptide cleavage site of the chains of type I procollagen are associated with a unique osteogenesis imperfecta phenotype. J Bone Miner Res. (2018) 33:1260–71. doi: 10.1002/jbmr.3424
60. Fiscaletti M, Biggin A, Bennetts B, Wong K, Briody J, Pacey V, et al. Novel variant in Sp7/Osx associated with recessive osteogenesis imperfecta with bone fragility and hearing impairment. Bone. (2018) 110:66–75. doi: 10.1016/j.bone.2018.01.031
61. Fratzl-Zelman N, Barnes AM, Weis M, Carter E, Hefferan TE, Perino G, et al. Non-lethal type VIII osteogenesis imperfecta has elevated bone matrix mineralization. J Clin Endocrinol Metab. (2016) 101:3516–25. doi: 10.1210/jc.2016-1334
62. Fratzl-Zelman N, Schmidt I, Roschger P, Roschger A, Glorieux FH, Klaushofer K, et al. Unique micro- and nano-scale mineralization pattern of human osteogenesis imperfecta type VI bone. Bone. (2015) 73:233–41. doi: 10.1016/j.bone.2014.12.023
63. Glorieux FH, Rauch F, Plotkin H, Ward L, Travers R, Roughley P, et al. Type V osteogenesis imperfecta: a new form of brittle bone disease. J Bone Miner Res. (2000) 15:1650–8. doi: 10.1359/jbmr.2000.15.9.1650
64. Glorieux FH, Ward LM, Rauch F, Lalic L, Roughley PJ, Travers R. Osteogenesis imperfecta type VI: a form of brittle bone disease with a mineralization defect. J Bone Miner Res. (2002) 17:30–8. doi: 10.1359/jbmr.2002.17.1.30
65. Iwamoto J, Takeda T, Ichimura S. Increased bone resorption with decreased activity and increased recruitment of osteoblasts in osteogenesis imperfecta type I. J Bone Miner Metab. (2002) 20:174–9. doi: 10.1007/s007740200025
66. Katti KS, Gu C, Katti DR. Anisotropic properties of human cortical bone with osteogenesis imperfecta. Biomech Model Mechanobiol. (2016) 15:155–67. doi: 10.1007/s10237-015-0727-4
67. Land C, Rauch F, Travers R, Glorieux FH. Osteogenesis imperfecta type VI in childhood and adolescence: effects of cyclical intravenous pamidronate treatment. Bone. (2007) 40:638–44. doi: 10.1016/j.bone.2006.10.010
68. Lindahl K, Astrom E, Dragomir A, Symoens S, Coucke P, Larsson S, et al. Homozygosity for CREB3L1 premature stop codon in first case of recessive osteogenesis imperfecta associated with OASIS-deficiency to survive infancy. Bone. (2018) 114:268–77. doi: 10.1016/j.bone.2018.06.019
69. Lindahl K, Barnes AM, Fratzl-Zelman N, Whyte MP, Hefferan TE, Makareeva E, et al. COL1 C-propeptide cleavage site mutations cause high bone mass osteogenesis imperfecta. Hum Mutat. (2011) 32:598–609. doi: 10.1002/humu.21475
70. McCarthy EF, Earnest K, Rossiter K, Shapiro J. Bone histomorphometry in adults with type IA osteogenesis imperfecta. Clin Orthop Relat Res. (1997) 336:254–62. doi: 10.1097/00003086-199703000-00034
71. Mäkitie RE, Haanpää M, Valta H, Pekkinen M, Laine CM, Lehesjoki A-E, et al. Skeletal characteristics of WNT1 osteoporosis in children and young adults. J Bone Miner Res. (2016) 31:1734–42. doi: 10.1002/jbmr.2841
72. Munns CFJ, Rauch F, Travers R, Glorieux FH. Effects of intravenous pamidronate treatment in infants with osteogenesis imperfecta: clinical and histomorphometric outcome. J Bone Miner Res. (2005) 20:1235–43. doi: 10.1359/JBMR.050213
73. Nerlich AG, Brenner RE, Wiest I, Lehmann H, Yang C, Müller PK, et al. Immunohistochemical localization of interstitial collagens in bone tissue from patients with various forms of osteogenesis imperfecta. Am J Med Genet. (1993) 45:258–9. doi: 10.1002/ajmg.1320450221
74. Rauch F, Lalic L, Roughley P, Glorieux FH. Relationship between genotype and skeletal phenotype in children and adolescents with osteogenesis imperfecta. J Bone Miner Res. (2010) 25:1367–74. doi: 10.1359/jbmr.091109
75. Rauch F, Travers R, Plotkin H, Glorieux FH. The effects of intravenous pamidronate on the bone tissue of children and adolescents with osteogenesis imperfecta. J Clin Invest. (2002) 110:1293–9. doi: 10.1172/JCI0215952
76. Roschger P, Fratzl-Zelman N, Misof BM, Glorieux FH, Klaushofer K, Rauch F. Evidence that abnormal high bone mineralization in growing children with osteogenesis imperfecta is not associated with specific collagen mutations. Calcif Tissue Int. (2008) 82:263–70. doi: 10.1007/s00223-008-9113-x
77. Sarathchandra P, Pope FM. Unexpected ultrastructral changes in bone osteiod collagens in osteogenesis imperfecta. Micron. (2005) 36:696–702. doi: 10.1016/j.micron.2005.07.011
78. Shapiro JR, McCarthy EF, Rossiter K, Ernest K, Gelman R, Fedarko N, et al. The effect of intravenous pamidronate on bone mineral density, bone histomorphometry, and parameters of bone turnover in adults with type IA osteogenesis imperfect. Calcif Tissue Int. (2003) 72:103–12. doi: 10.1007/s00223-001-1055-5
79. Ste-Marie LG, Charhon SA, Edouard C, Chapuy MC, Meunier PJ. Iliac bone histomorphometry in adults and children with osteogenesis imperfecta. J Clin Pathol. (1984) 37:1081–9. doi: 10.1136/jcp.37.10.1081
80. Vardakastani V, Saletti D, Skalli W, Marry P, Allain JM, Adam C. Increased intra-cortical porosity reduces bone stiffness and strength in pediatric patients with osteogenesis imperfecta. Bone. (2014) 69:61–7. doi: 10.1016/j.bone.2014.09.003
81. Vignery A. Bone cell defects in osteogenesis imperfecta. Connect Tissue Res. (1995) 31:275–8. doi: 10.3109/03008209509010822
82. Webb EA, Balasubramanian M, Fratzl-Zelman N, Cabral WA, Titheradge H, Alsaedi A, et al. Phenotypic spectrum in osteogenesis imperfecta due to mutations in TMEM38B: unraveling a complex cellular defect. J Clin Endocrinol Metab. (2017) 102:2019–28. doi: 10.1210/jc.2016-3766
83. Weber M, Roschger P, Fratzl-Zelman N, Schöberl T, Rauch F, Glorieux FH, et al. Pamidronate does not adversely affect bone intrinsic material properties in children with osteogenesis imperfect. Bone. (2006) 39:616–22. doi: 10.1016/j.bone.2006.02.071
84. Zeitlin L, Rauch F, Travers R, Munns C, Glorieux FH. The effect of cyclical intravenous pamidronate in children and adolescents with osteogenesis imperfecta type V. Bone. (2006) 38:13–20. doi: 10.1016/j.bone.2005.07.020
85. Farber CR, Reich A, Barnes AM, Becerra P, Rauch F, Cabral WA, et al. A novel IFITM5 mutation in severe atypical osteogenesis imperfecta type VI impairs osteoblast production of pigment epithelium-derived factor. J Bone Miner Res. (2014) 29:1402–11. doi: 10.1002/jbmr.2173
86. Doty SB, Mathews RS. Electron microscopic and histochemical investigation of osteogenesis imperfecta tarda. Clin Orthop Relat Res. (1971) 80:191–201. doi: 10.1097/00003086-197110000-00027
87. Sarathchandra P, Cassella JP, Ali SY. Enzyme histochemical localisation of alkaline phosphatase activity in osteogenesis imperfecta bone and growth plate: a preliminary study. Micron. (2005) 36:715–20. doi: 10.1016/j.micron.2005.05.014
88. Teitelbaum SL, Kraft WJ, Lang R, Avioli LV. Bone collagen aggregation abnormalities in osteogenesis imperfecta. Calcif Tissue Res. (1974) 17:75–9. doi: 10.1007/BF02547215
89. Riley FC, Jowsey J, Brown DM. Osteogenesis imperfecta: morphologic and biochemical studies of connective tissue. Pediatr Res. (1973) 7:757–68. doi: 10.1203/00006450-197309000-00005
90. Fratzl-Zelman N, Schmidt I, Roschger P, Glorieux FH, Klaushofer K, Fratzl P, et al. Mineral particle size in children with osteogenesis imperfecta type I is not increased independently of specific collagen mutations. Bone. (2014) 60:122–8. doi: 10.1016/j.bone.2013.11.023
91. Abidin AZ, Jameson J, Molthen R, Wismüller A. Classification of micro-CT images using 3D characterization of bone canal patterns in human osteogenesis imperfecta. Proc SPIE Int Soc Opt Eng. (2017) 10134:1013413. doi: 10.1117/12.2254421
92. Sarathchandra P, Pope FM, Ali SY. An ultrastructural and immunogold localization study of proteoglycans associated with the osteocytes of fetal bone in osteogenesis imperfecta. Calcif Tissue Int. (1996) 58:435–42. doi: 10.1007/s002239900072
93. DiCaprio MR, Enneking WF. Fibrous dysplasia. Pathophysiology, evaluation, and treatment. J Bone Joint Surg Am. (2005) 87:1848–64. doi: 10.2106/00004623-200508000-00028
94. Corsi A, Collins MT, Riminucci M, Howell PGT, Boyde A, Robey PG, et al. Osteomalacic and hyperparathyroid changes in fibrous dysplasia of bone: core biopsy studies and clinical correlations. J Bone Miner Res. (2003) 18:1235–46. doi: 10.1359/jbmr.2003.18.7.1235
95. Siadati S, Shafigh E. McCune-Albright syndrome: a case report. Indian J Dermatol Venereol Leprol. (2010) 76:723. doi: 10.4103/0378-6323.72473
96. Gerceker M, Ozgursoy OB, Erdem A, Ekinci C. Fibrous dysplasia in the retropharyngeal area. Ear Nose Throat. (2006) 85:446–7. doi: 10.1177/014556130608500716
97. Sakamoto A, Oda Y, Iwamoto Y, Tsuneyoshi M. A comparative study of fibrous dysplasia and osteofibrous dysplasia with regard to expressions of c-fos and c-jun products and bone matrix proteins: a clinicopathologic review and immunohistochemical study of c-fos, c-jun, type I collagen, osteonectin, osteopontin, and osteocalcin. Hum Pathol. (1999) 30:1418–26. doi: 10.1016/S0046-8177(99)90162-4
98. Riminucci M, Liu B, Corsi A, Shenker A, Spiegel AM, Robey PG, et al. The histopathology of fibrous dysplasia of bone in patients with activating mutations of the Gs alpha gene: site-specific patterns and recurrent histological hallmarks. J Pathol. (1999) 187:249–58. doi: 10.1002/(SICI)1096-9896(199901)187:2<249::AID-PATH222>3.0.CO;2-J
99. Laino L, Favia G, Menditti D, De Francesco F, Salerno C, Scivetti M, et al. Confocal laser scanning microscopy analysis of 10 cases of craniofacial fibrous dysplasia. Ultrastruct Pathol. (2015) 39:231–4. doi: 10.3109/01913123.2014.1002961
100. Boyce AM, Chong WH, Yao J, Gafni RI, Kelly MH, Chamberlain CE, et al. Denosumab treatment for fibrous dysplasia. J Bone Miner Res. (2012) 27:1462–70. doi: 10.1002/jbmr.1603
101. Toyosawa S, Yuki M, Kishino M, Ogawa Y, Ueda T, Murakami S, et al. Ossifying fibroma vs fibrous dysplasia of the jaw: molecular and immunological characterization. Mod Pathol. (2007) 20:389–96. doi: 10.1038/modpathol.3800753
102. Maki M, Saitoh K, Horiuchi H, Morohoshi T, Fukayama M, Machinami R. Comparative study of fibrous dysplasia and osteofibrous dysplasia: histopathological, immunohistochemical, argyrophilic nucleolar organizer region and DNA ploidy analysis. Pathol Int. (2001) 51:603–11. doi: 10.1046/j.1440-1827.2001.01252.x
103. Bravenboer N, Micha D, Triffit JT, Bullock AN, Ravazollo R, Bocciardi R, et al. Clinical utility gene card for: fibrodysplasia ossificans progressiva. Eur J Hum Genet. (2015) 23:1431. doi: 10.1038/ejhg.2014.274
104. Kaplan FS, Le Merrer M, Glaser DL, Pignolo RJ, Goldsby RE, Kitterman JA, et al. Fibrodysplasia ossificans progressiva. Best Pract Res Clin Rheumatol. (2008) 22:191–205. doi: 10.1016/j.berh.2007.11.007
105. Kaplan FS, Tabas JA, Gannon FH, Finkel G, Hahn GV, Zasloff MA. The histopathology of fibrodysplasia ossificans progressiva. An endochondral process. J Bone Joint Surg Am. (1993) 75:220–30. doi: 10.2106/00004623-199302000-00009
106. Piram M, Le Merrer M, Bughin V, De Prost Y, Fraitag S, Bodemer C. Scalp nodules as a presenting sign of fibrodysplasia ossificans progressiva: a register-based study. J Am Acad Dermatol. (2011) 64:97–101. doi: 10.1016/j.jaad.2010.04.025
107. Ibarra M, Chou PM, Pachman LM, Zhao Y-D, Boskey AL. Calcification in a case of circumscribed myositis ossificans. J Rheumtol. (2010) 37:876. doi: 10.3899/jrheum.090833
108. van Dijk FS, Zillikens MC, Micha D, Riessland M, Marcelis CL, de Die-Smulders CE, et al. PLS3 mutations in X-linked osteoporosis with fractures. N Engl J Med. (2013) 369:1529–36. doi: 10.1056/NEJMoa1308223
109. Kannu P, Mahjoub A, Babul-Hirji R, Carter MT, Harrington J. PLS3 mutations in X-linked osteoporosis: clinical and bone characteristics of two novel mutations. Horm Res Paediatr. (2017) 88:298–304. doi: 10.1159/000477242
110. Fahiminiya S, Majewski J, Al-Jallad H, Moffatt P, Mort J, Glorieux FH, et al. Osteoporosis caused by mutations in PLS3: clinical and bone tissue characteristics. J Bone Miner Res. (2014) 29:1805–14. doi: 10.1002/jbmr.2208
111. Kämpe AJ, Costantini A, Levy-Shraga Y, Zeitlin L, Roschger P, Taylan F, et al. PLS3 deletions lead to severe spinal osteoporosis and disturbed bone matrix mineralization. J Bone Miner Res. (2017) 32:2394–404. doi: 10.1002/jbmr.3233
112. Laine CM, Wessman M, Toiviainen-Salo S, Kaunisto MA, Mäyränpää MK, Laine T, et al. A novel splice mutation in PLS3 causes X-linked early onset low-turnover osteoporosis. J Bone Miner Res. (2015) 30:510–8. doi: 10.1002/jbmr.2355
113. Wesseling-Perry K, Mäkitie RE, Välimäki V-V, Laine T, Laine CM, Välimäki MJ, et al. Osteocyte protein expression is altered in low-turnover osteoporosis caused by mutations in WNT1 and PLS3. J Clin Endocrinol Metab. (2017) 102:2340–8. doi: 10.1210/jc.2017-00099
114. Balasubramanian M, Fratzl-Zelman N, O'Sullivan R, Bull M, Fa Peel N, Pollitt RC, et al. Novel PLS3 variants in X-linked osteoporosis: exploring bone material properties. Am J Med Genet A. (2018) 176:1578–86. doi: 10.1002/ajmg.a.38830
115. Kampe AJ, Costantini A, Levy-Shraga Y, Zeitlin L, Roschger P, Taylan F, et al. PLS3 deletions lead to severe spinal osteoporosis and disturbed bone matrix mineralization. J Bone Miner Res. (2017) 32:2394–404. doi: 10.1002/jbmr.3233
116. Loeys BL, Chen J, Neptune ER, Judge DP, Podowski M, Holm T, et al. A syndrome of altered cardiovascular, craniofacial, neurocognitive and skeletal development caused by mutations in TGFBR1 or TGFBR2. Nat Genet. (2005) 37:275–81. doi: 10.1038/ng1511
117. Van Laer L, Dietz H, Loeys B. Loeys-dietz syndrome. Adv Exp Med Biol. (2014) 802:95–105. doi: 10.1007/978-94-007-7893-1_7
118. Kirmani S, Tebben PJ, Lteif AN, Gordon D, Clarke BL, Hefferan TE, et al. Germline TGF-β receptor mutations and skeletal fragility: a report on two patients with Loeys-Dietz syndrome. Am J Med Genet A. (2010) 152A:1016–9. doi: 10.1002/ajmg.a.33356
119. Balooch G, Balooch M, Nalla RK, Schilling S, Filvaroff EH, Marshall GW, et al. TGF-β regulates the mechanical properties and composition of bone matrix. Proc Natl Acad Sci U S A. (2005) 102:18813–8. doi: 10.1073/pnas.0507417102
120. Ben Amor IM, Edouard T, Glorieux FH, Chabot G, Tischkowitz M, Roschger P, et al. Low bone mass and high material bone density in two patients with Loeys-Dietz syndrome caused by transforming growth factor beta receptor 2 mutations. J Bone Miner Res. (2012) 27:713–8. doi: 10.1002/jbmr.1470
121. Tolar J, Teitelbaum SL, Orchard PJ. Osteopetrosis. N Engl J Med. (2004) 351:2839–49. doi: 10.1056/NEJMra040952
122. Stark Z, Savarirayan R. Osteopetrosis. Orphanet J Rare Dis. (2009) 4:5. doi: 10.1186/1750-1172-4-5
123. Teti A, Migliaccio S, Taranta A, Bernardini S, De Rossi G, Luciani M, et al. Mechanisms of osteoclast dysfunction in human osteopetrosis: abnormal osteoclastogenesis and lack of osteoclast-specific adhesion structures. J Bone Miner Res. (1999) 14:2107–17. doi: 10.1359/jbmr.1999.14.12.2107
124. Bollerslev J, Marks SC, Pockwinse S, Kassem M, Brixen K, Steiniche T, et al. Ultrastructural investigations of bone resorptive cells in two types of autosomal dominant osteopetrosis. Bone. (1993) 14:865–9. doi: 10.1016/8756-3282(93)90316-3
125. Bollerslev J, Steiniche T, Melsen F, Mosekilde L. Structural and histomorphometric studies of iliac crest trabecular and cortical bone in autosomal dominant osteopetrosis: a study of two radiological types. Bone. (1989) 10:19–24. doi: 10.1016/8756-3282(89)90142-7
126. Cournot G, Trubert-Thil CL, Petrovic M, Boyle A, Cormier C, Girault D, et al. Mineral metabolism in infants with malignant osteopetrosis: heterogeneity in plasma 1,25-dihydroxyvitamin D levels and bone histology. J Bone Miner Res. (1992) 7:1–10. doi: 10.1002/jbmr.5650070103
127. Shapiro F, Key LL, Anast C. Variable osteoclast appearance in human infantile osteopetrosis. Calcif Tissue Int. (1988) 43:67–76. doi: 10.1007/BF02555149
128. Satomura K, Kon M, Tokuyama R, Tomonari M, Takechi M, Yuasa T, et al. Osteopetrosis complicated by osteomyelitis of the mandible: a case report including characterization of the osteopetrotic bone. Int J Oral Maxillofac Surg. (2007) 36:86–93. doi: 10.1016/j.ijom.2006.06.009
129. Rivera TL, Irish RD, Hoda SA, Steiner GC, Rackoff PJ, Fischer HD. Erdheim-Chester disease–clinical pathological case discussion. Bull Hosp Jt Dis. (2013) 71:152–5.
130. Stoppacciaro A, Ferrarini M, Salmaggi C, Colarossi C, Praderio L, Tresoldi M, et al. Immunohistochemical evidence of a cytokine and chemokine network in three patients with Erdheim-Chester disease: implications for pathogenesis. Arthritis Rheum. (2006) 54:4018–22. doi: 10.1002/art.22280
131. Kim NR, Ko YH, Choe YH, Lee HG, Huh B, Ahn GH. Erdheim-Chester disease with extensive marrow necrosis: a case report and literature review. Int J Surg Pathol. (2001) 9:73–9. doi: 10.1177/106689690100900115
132. Cavalli G, Guglielmi B, Berti A, Campochiaro C, Sabbadini MG, Dagna L. The multifaceted clinical presentations and manifestations of Erdheim-Chester disease: comprehensive review of the literature and of 10 new cases. Ann Rheum Dis. (2013) 72:1691–5. doi: 10.1136/annrheumdis-2012-202542
133. Kannu P, Baskin B, Bowdin S. Cherubism. “Cherubism.” In: Adam MP, Ardinger HH, Pagon RA, Wallace SE, Bean LJH, Stephens K, et al., editors. GeneReviews((R)). Seattle, WA: University of Washington, Seattle University of Washington, Seattle. GeneReviews is a registered trademark of the University of Washington, Seattle. All rights reserved. (1993) 1–17.
134. Kadlub N, Sessiecq Q, Mandavit M, L'Hermine AC, Badoual C, Galmiche L, et al. Molecular and cellular characterizations of human cherubism: disease aggressiveness depends on osteoclast differentiation. Orphan J Rare Dis. (2018) 13:166. doi: 10.1186/s13023-018-0907-2
135. Chavali LV, Bhimalingam RMR, Sudhakar PV. Cherubism-a case report with long term follow up. Indian J Pathol Microbiol. (2011) 54:793–5. doi: 10.4103/0377-4929.91509
136. Wang C, Song Y, Peng B, Fan M, Li J, Zhu S, et al. Expression of c-Src and comparison of cytologic features in cherubism, central giant cell granuloma and giant cell tumors. Oncol Rep. (2006) 15:589–94. doi: 10.3892/or.15.3.589
137. Roschger P, Gupta HS, Berzlanovich A, Ittner G, Dempster DW, Fratzl P, et al. Constant mineralization density distribution in cancellous human bone. Bone. (2003) 32:316–23. doi: 10.1016/S8756-3282(02)00973-0
138. Fratzl-Zelman N, Roschger P, Misof BM, Pfeffer S, Glorieux FH, Klaushofer K, et al. Normative data on mineralization density distribution in iliac bone biopsies of children, adolescents and young adults. Bone. (2009) 44:1043–8. doi: 10.1016/j.bone.2009.02.021
139. Guañabens NP, Monegal A. Bone turnover markers: a clinical review. Clin Rev Bone Min Metab. (2015) 13:83–97. doi: 10.1007/s12018-015-9185-x
140. Milovanovic P, Adamu U, Simon MJ, Rolvien T, Djuric M, Amling M, et al. Age- and sex-specific bone structure patterns portend bone fragility in radii and tibiae in relation to osteodensitometry: a high-resolution peripheral quantitative computed tomography study in 385 individuals. J Gerontol Ser A Biol Sci Med Sci. (2015) 70:1269–75. doi: 10.1093/gerona/glv052
141. Cheung AM, Adachi JD, Hanley DA, Kendler DL, Davison KS, Josse R, et al. High-resolution peripheral quantitative computed tomography for the assessment of bone strength and structure: a review by the Canadian Bone Strength Working Group. Curr Osteoporos Rep. (2013) 11:136–46. doi: 10.1007/s11914-013-0140-9
142. Rolvien T, Schmidt T, Schmidt FN, von Kroge S, Busse B, Amling M, et al. Recovery of bone mineralization and quality during asfotase alfa treatment in an adult patient with infantile-onset hypophosphatasia. Bone. (2019) 127:67–74. doi: 10.1016/j.bone.2019.05.036
Keywords: bone biopsy, histomorphometry, bone quality, rare bone disorders, advanced techniques
Citation: Treurniet S, Eekhoff EMW, Schmidt FN, Micha D, Busse B and Bravenboer N (2020) A Clinical Perspective on Advanced Developments in Bone Biopsy Assessment in Rare Bone Disorders. Front. Endocrinol. 11:399. doi: 10.3389/fendo.2020.00399
Received: 31 January 2020; Accepted: 18 May 2020;
Published: 23 June 2020.
Edited by:
Guillaume Mabilleau, Université d'Angers, FranceReviewed by:
Natalie A. Sims, St. Vincents Institute of Medical Research, AustraliaStéphane Blouin, Ludwig Boltzmann Institute of Osteology (LBIO), Austria
Copyright © 2020 Treurniet, Eekhoff, Schmidt, Micha, Busse and Bravenboer. This is an open-access article distributed under the terms of the Creative Commons Attribution License (CC BY). The use, distribution or reproduction in other forums is permitted, provided the original author(s) and the copyright owner(s) are credited and that the original publication in this journal is cited, in accordance with accepted academic practice. No use, distribution or reproduction is permitted which does not comply with these terms.
*Correspondence: Nathalie Bravenboer, bi5icmF2ZW5ib2VyJiN4MDAwNDA7YW1zdGVyZGFtdW1jLm5s