- 1Health Management Institute, People's Liberation Army General Hospital, Beijing, China
- 2Department of Clinical Laboratory, Xiamen Huli Guoyu Clinic, Co., Ltd., Xiamen, China
The structurally-related peptides, gastrin and cholecystokinin (CCK), were originally discovered as humoral stimulants of gastric acid secretion and pancreatic enzyme release, respectively. With the aid of methodological advances in biochemistry, immunochemistry, and molecular biology in the past several decades, our concept of gastrin and CCK as simple gastrointestinal hormones has changed considerably. Extensive in vitro and in vivo studies have shown that gastrin and CCK play important roles in several cellular processes including maintenance of gastric mucosa and pancreatic islet integrity, neurogenesis, and neoplastic transformation. Indeed, gastrin and CCK, as well as their receptors, are expressed in a variety of tumor cell lines, animal models, and human samples, and might contribute to certain carcinogenesis. In this review, we will briefly introduce the gastrin and CCK system and highlight the effects of gastrin and CCK in the regulation of cell proliferation and apoptosis in both normal and abnormal conditions. The potential imaging and therapeutic use of these peptides and their derivatives are also summarized.
Introduction
Multicellular organisms have developed a delicate and efficient cellular regulatory system to maintain a balanced cell proliferation, differentiation, and apoptosis. Aberrant cellular regulation usually results in pathophysiology, for instance, carcinogenesis resulting from infinite cell proliferation, escape from apoptosis, and metastatic invasion. The gut is the largest endocrine organ in the body that expresses over 30 gut hormone genes and a wealth of bioactive peptides (1). As two of the first gastrointestinal peptides discovered, gastrin and CCK are known to play important roles in digestive processes including gastric acid secretion, pancreatic enzyme release, gallbladder emptying, gut motility, and energy homeostasis (2–4).
However, accumulating in vitro and in vivo studies have demonstrated that gastrin and CCK integrate and coordinate a rich network of information exchange pathways in cellular processes of proliferation and apoptosis, and under certain circumstances, they contribute to the pathogenesis and progression of some types of tumors (5). Indeed, gastrin, CCK and their cognate receptors (CCK2R and CCK1R, discussed in detail in section The Receptors for Gastrin and CCK) have been reported to be expressed and involved in several adenocarcinomas originated in stomach, colon, pancreas, esophagus, and gallbladder, as well as some tumors in the brain (6, 7). However, the gastrin and CCK regulatory systems are complex and intricate due to wide distribution of the hormone genes, cell-specific alternative splicing and post-translational modifications, and activation of multiple signal transduction pathways. In this review, we aim to briefly introduce the gastrin and CCK systems regarding peptide biosynthesis, cellular expression, receptor activation, downstream signaling pathways, and involvement in proliferative and apoptotic responses in normal and malignant conditions. The underlying mechanisms attributed to the peptide-induced cellular effects and potential imaging and therapeutic applications will be elaborated.
The Gastrin and CCK System
Gastrin and CCK
As two of the first gastrointestinal hormones identified, the gastrin and CCK were originally discovered as hormonal regulators in stomach and small intestine in 1906 and 1928, respectively (8, 9). Subsequent isolation and structure determination of gastrin and CCK in the 1960s (10, 11) attracted researchers to investigate the biology, physiology, and pharmacology of these two peptides. In humans, the genes encoding gastrin and CCK precursors are located on chromosome 17q21 and 3p22-p21.3, respectively (12, 13). Both gastrin and CCK exist in multiple molecular forms following cell-specific post-translational processing. Among these peptides, the biologically active gastrin includes progastrin, Gly-extended gastrin-17 or −34 (Ggly), and the amidated gastrin-17 or −34 (Gamide), whereas the biologically active CCKs include Gly-extended and amidated CCK-33, -58, -22, and -8 (4, 14). In humans, amidated G-17 and CCK-33 predominate in plasma, and amidated CCK-8 predominates in the brain (15, 16). It should be noted that all bioactive gastrin and CCK peptides share the same amidated COOH-terminal pentapeptide (Gly-Trp-Met-Asp-Phe-NH2) motif, which is exceedingly well-conserved during evolution and comprises the minimal sequence (pharmacophore) required for biological activity and receptor activation (17).
In response to food intake, gastrin is synthesized and released by mucosal G cells to stimulate enterochromaffin-like (ECL) cells to secrete histamine, which further induces acid release from parietal cells through activation of H2 histamine receptors, whereas CCK is predominantly produced and secreted by upper small intestinal I cells to stimulate gallbladder contraction and pancreatic enzyme secretion. As classical gut hormones and potent neurotransmitters, gastrin and CCK are widely distributed in gastrointestinal tract, CNS, and peripheral neurons (4, 18). However, CCK has also been suggested to stimulate spermatozoan fertilization, exert anti-inflammatory effects, promote sodium excretion into the urine, and predict the risk of mortality in heart failure, consistent with its expression in male germ cells (19), cells of immune system (20–23), renal cortex and medulla (24), and cardiomyocytes (25), respectively.
In addition to the acute digestive effects, both gastrin and CCK have been suggested to exert potent proliferative and anti-apoptotic effects by contributing to pathogenesis and progression of cancer (26, 27). Indeed, hypergastrinemia induced by proton pump inhibitor (PPI) together with Helicobacter pylori (H. pylori) infection correlates with higher risk of gastric and colorectal cancer in experimental animal models (28). Increased expression of gastrin and/or CCK were observed in human gastric adenocarcinoma (29, 30), colorectal carcinoma (31, 32), and pancreatic cancer (33), over that of the corresponding normal tissues, indicating potential roles of these peptides in promoting carcinogenesis (6).
The Receptors for Gastrin and CCK
The broad range of physiological functions of gastrin and CCK are mediated by two cognate receptors, which belong to rhodopsin-like G protein-coupled receptors characterized with the hallmark structure of seven transmembrane domains and the alternating NH2 terminus and COOH tail. Gastrin receptor is also referred as CCK2 receptor (CCK2R, previously also called CCK-B receptor) based on the facts that (1) gastrin and CCK share the core sequence required for biological activities of both peptides and activation of the receptor; (2) gastrin and CCK have comparable affinity and potency for CCK2R; (3) CCK2R mediates almost all the classical physiological activities of gastrin (34, 35). However, CCK1 receptor (CCK1R, previously also called CCK-A receptor) binds and responds to CCK with a 500- to 1,000-fold higher affinity and potency than gastrin (36). Therefore, CCK1R is considered as the cognate receptor for CCK (35).
Using primarily cultured mucosal cells, Sachs and colleagues showed that both gastrin and CCK exert essentially equal potency in stimulating acid secretion from gastric parietal cells and histamine release from mucosal cells (37, 38), which are in line with their comparable affinity and potency for CCK2R. However, non-sulfated CCK-8 is less potent and less effective than gastrin in inducing DNA synthesis of isolated ECL cells, as assessed by 5-bromo-2′-deoxyuridine incorporation and ELISA (38). In addition, infusion of gastrin or CCK-8 in rats stimulates histamine synthesis and ECL hyperplasia except that CCK-8 fails to induce ECL hyperplasia even the circulating CCK-8 levels are 10-fold above normal (39, 40). The differential growth responses between gastrin and non-sulfated CCK-8 might be due to lower stability of non-sulfated CCK-8 caused by endopeptidases from the cell culture and/or different binding states of CCK2R are existing for gastrin and non-sulfated CCK-8 (38). Additional studies employing molecular cloning and radioligand binding might provide further explanations.
Table 1 summarizes the general distribution of CCK2R and CCK1R in healthy adult mammals. CCK2R is predominantly expressed in the brain and selected regions in the gastrointestinal tract, including gastric epithelial parietal cells, ECL cells, and D cells, pancreatic acinar cells, myenteric neurons, monocytes and T lymphocytes, and human peripheral blood mononuclear cells (18, 41, 42, 47, 48, 56, 57), whereas CCK1R is mainly found in pancreatic acinar cells, gallbladder smooth muscles, gastric mucosal chief and D cells, as well as cerebral and peripheral neurons (6, 46, 50–52).
Table 2 summarizes the localization and actions of gastrin, CCK, and their receptors on different types of human cancers (indicated as percentage of positive tissues expressing the corresponding ligand or receptor). A variety of human adenocarcinomas in the stomach, pancreas, colon, rectum, esophagus, lung, liver, medullary thyroid, overexpress CCK2R and/or CCK1R than the matched normal tissues (61, 62, 71, 79, 81, 82), serving as the basis for CCK2R- or CCK1R-targeted tumor imaging and therapy (84). Indeed, intensive chemical, physiological, and pharmacological studies provide novel diagnostic and therapeutic agents for the tumors overexpressing CCK2R and/or CCK1R (85).
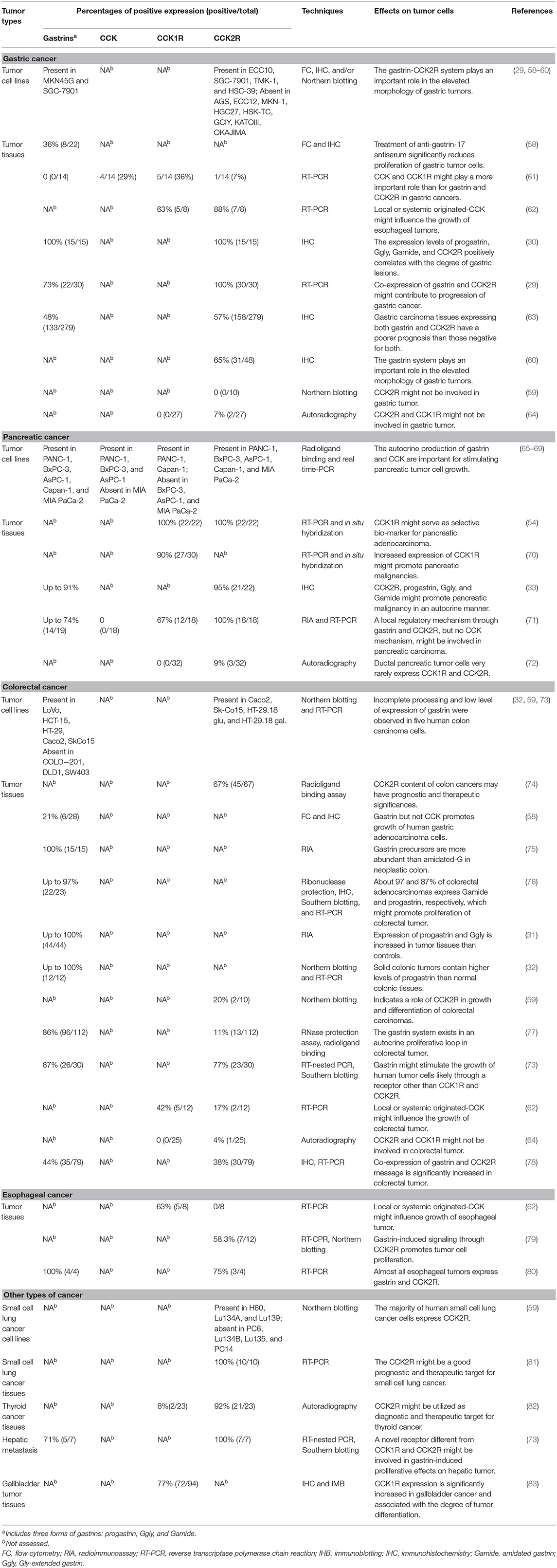
Table 2. Expression and function profiles of gastrin, CCK, and their receptors in human cancer cell lines and tissues.
Another line of evidence, the identification and characterization of the CCK2R splice variants and mutations, further confirmed the involvement of the gastrin and CCK system in cell proliferation and cancer pathogenesis. Indeed, CCK2Ri4sv, an CCK2R splice variant containing intron 4 and therefore additional 69 amino acids in the third intracellular loop, was identified in patients with colorectal cancer (86). Further in vitro functional studies showed that CCK2Ri4sv exhibits constitutive activation of signaling pathways resulting in enhanced Ca2+ levels and cell proliferation in both primary and Balb3T3 human colorectal tumor cells (86). In contrast, in vitro expression of CCK2Ri4sv in human epithelial HEK293 cells does not affect cell growth (87). Interestingly, compared to CCK2R-HEK293-xenografted mice, the CCK2Ri4sv-HEK293-xenografted mice have significantly increased tumor growth, which is associated with a constitutive, Src-dependent increase in the transcription factor hypoxia-inducible factor-1α and secretion of vascular endothelial growth factor (87). Other naturally occurring mutations in the CCK2R gene, such as V287F and R396C, were also shown to promote cell proliferation or angiogenesis through increase in Src-dependent secretion of cytokines (88).
Several cellular models have been utilized to investigate the cellular effects of gastrin and CCK, including (1) cells endogenously expressing CCK2R and CCK1R including human pancreatic tumor PANC-1 and Capan-1 cells (65–67), rat brain E18 neuroblasts (89); (2) cells endogenously expressing only CCK2R, such as rat pancreatic tumor AR42J cells (90), human pancreatic tumor BxPC-3, MIAPaCa-2, and AsPC-1 cells (67, 68, 91), rat pituitary adenoma GH3 cells (92), and human colon cancer HT-29 cells (93); (3) cells ready to be transiently or stably transfected with CCK2R or CCK1R, such as human gastric adenocarcinoma AGS and MKN-45 cells (94, 95), rat intestinal epithelial RIE-1 cells (96), Rat-1 and mouse Swiss 3T3 (also named NIH3T3) fibroblasts (97–99), Chinese hamster ovary CHO cells (99).
Intracellular Signaling Pathways
Once activated by the ligands, the CCK2R and CCK1R located on the cell surface undergo conformational changes and trigger a complex intracellular network of signaling pathways. As shown in Figure 1, both CCK2R and CCK1R can signal through Gq protein to activate phospholipase Cβ (PLCβ), resulting in hydrolysis of phosphatidylinositol 4,5-bisphosphate into inositol trisphosphate and diacylglycerol. Furthermore, second messenger diacylglycerol, together with inositol trisphosphate-induced Ca2+ efflux from endoplasmic reticulum, stimulate the phosphorylation of protein kinase C (PKC) isoforms to activate downstream effector proteins such as mitogen-activated protein kinases (MAPKs, important regulators in cell proliferation, differentiation, survival, and apoptosis) and inflammatory regulator NF-κB (35, 100).
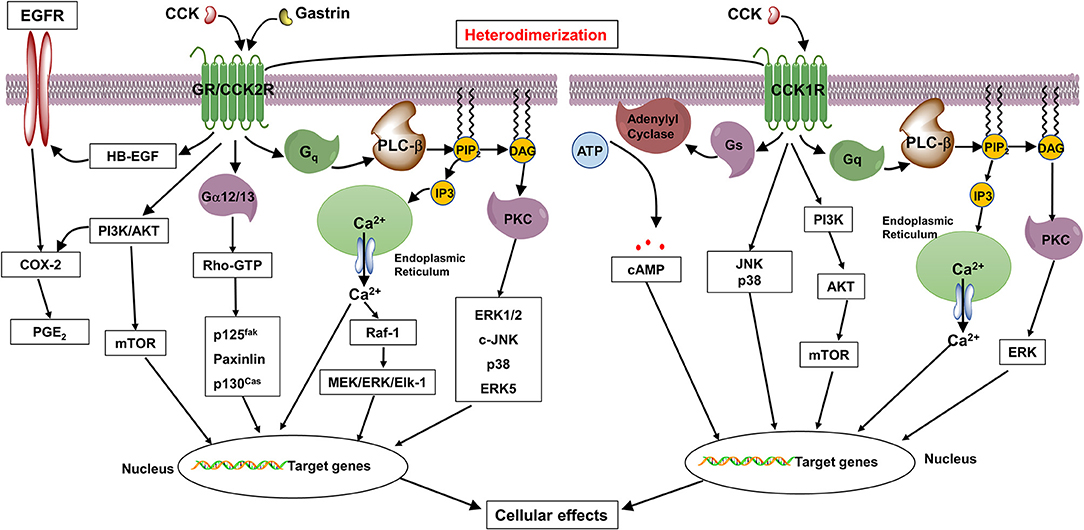
Figure 1. Proposed diagrams of gastrin- and CCK-induced signaling pathways through CCK2R and CCK1R in normal and tumor cells. In response to gastrin and CCK, CCK2R couples to Gq and Gα12/13 proteins to promote cell proliferation and inhibit apoptosis through activation of PLC/Ca2+/PKC, MAPK, p125fak, Src, and PI3K/AKT cascades, as well as transactivation of EGFR, whereas CCK1R couples to Gq and Gs to exhibit trophic effects through activation of PLC/Ca2+/PKC, AC/cAMP/PKA, MAPK, and PI3K/AKT pathways.
In addition, both receptors are able to induce PKC-independent activation of MAPK and PI3K/AKT/mTOR signaling pathways (35, 101). A variety of non-receptor tyrosine kinases, including protooncogene Src kinase, focal adhesion tyrosine kinase (FAK, involved in cell morphology, cell motility, and invasion), and Janus kinase (JAK, involved in cell proliferation, differentiation, apoptosis, and oncogenesis) have also been reported to be activated by CCK2R and/or CCK1R (97, 102, 103). Furthermore, likely through activation of heparin-binding epidermal growth factor (EGF)-like growth factor, CCK2R activation was also reported to lead to transactivation of the EGF receptor (EGFR), a transmembrane tyrosine kinase receptor that plays important roles in cell growth, apoptosis, and migration (96, 104).
However, only CCK1R can couple to Gs protein to activate adenylyl cyclase, a cell membrane enzyme that catalyzes the cytoplasmic ATP into cAMP. The intracellular cAMP acts as second messenger to activate protein kinase A, which further stimulates the phosphorylation of cAMP response element-binding protein, a well-known transcription factor that affects a wealth of downstream genes (105, 106). In addition, the activation of nitric oxide (NO)/cGMP signaling cascade have been reported to be mediated by CCK1R in CHO cells and rodent pancreatic acini cells expressing CCK1R (107, 108).
Gastrin and CCK in Cell Proliferation
Gastrin and CCK Effects in vitro
A number of in vitro studies showed that gastrin and/or CCK induce a significant but modest increase in DNA synthesis in CCK2R-expressing cells, about 1.5-fold in AR42J cells, 1.6-fold in GH3 cells, up to 1.6-fold in HT-29 cells, 1.8-fold in CHO cells, 3-fold in Rat-1 cells, and 4-fold in Swiss 3T3 cells over the corresponding unstimulated control cells (98, 99, 109–111). Although gastrin and CCK exert similar growth-promoting effect in different cell models, the mechanisms attributed for the ligand-stimulated trophic effects seem to be cell specific. In AR42J cells, gastrin was shown to stimulate cell proliferation through MEK/ERK2/Elk-1-induced upregulation of c-fos gene, an early response gene associated with cell growth (94, 109). This activation is PKC-dependent and requires the small GTP-binding RhoA, the CA rich G sequence of the SRE promoter, and transcription factors Elk-1 and Sap-1a which bind to the E26 transformation specific motif (112). Interestingly, in GH3 cells with a similar CCK2R expression and gastrin/CCK2R binding kinetics compared to AR42J cells, the same group further showed that gastrin promotes cell growth in Ca2+-dependent manner, without activation of ERK1/2 (110).
In addition, gastrin and CCK stimulate cell proliferation in ERK1/2- and p74raf-1 kinase-dependent but Gi-independent manner in CCK2R-transfected Rat-1 cells (98), whereas it involves PKC/Ca2+- and Src-dependent activation of p38 pathway in CHO-CCK2R cells (103). In CCK2R-expressing Swiss 3T3 cells, gastrin induces G1/S cell cycle transition and cell proliferation through upregulation of cyclin D1, D3, and E, activation of cyclin-dependent kinases, and hyperphosphorylation of retinoblastoma protein (113). Gastrin-induced cyclin D1 transcription activity is mediated through activation of β-catenin and CREB pathways in AGS-CCK2R cells (114).
Cyclooxygenase-2 (COX-2), an inflammatory regulator critical for prostaglandin synthesis, have been suggested to be a downstream player of CCK2R. Indeed, gastrin increases transcriptional level of COX-2 in several cell lines, although the underlying mechanisms are different. In RIE-1 cells, the mRNA level of COX-2 is increased through activation of ERK5 and transactivation of the EGFR (96), whereas in Swiss 3T3 cells, it does not require PKC activity, activation of ERK1/2, or transactivation of EGFR (115). Gastrin-dependent COX-2 expression is inhibited by pretreatment with CCK2R antagonist L365,260, but not by pretreatment with CCK1R antagonist L364,714, indicating CCK2R but not CCK1R mediates gastrin-induced upregulation of COX-2 (115). In HT-29 cells, gastrin stimulates COX-2 expression via ERK1/2 and PI3K/AKT pathways (93). Together with the evidence that the COX-2 inhibitor L-745,337 reverses gastrin-induced DNA synthesis and cell growth (93), it is reasonable to conclude that COX-2 is responsible for the trophic effects of gastrin.
As the most abundant peptide neurotransmitter in the brain, the effects of gastrin and CCK on neuronal proliferation have been also investigated. In rat brain E18 neuroblasts, the group of Bragado showed that CCK promotes neuroblast proliferation by inducing tyrosine phosphorylation of adaptor proteins p130Cas and paxillin (89), two key components of focal adhesion complexes (116, 117), and phosphorylation of PKB/AKT and ERK1/2, followed by stimulation of DNA-binding activity of AP-1 (89). Furthermore, pharmacological blockade of CCK2R signaling with a potent and selective non-peptide CCK2R antagonist CR2945 inhibits CCK-stimulated ERK1/2 phosphorylation by over 50%, whereas totally antagonizes gastrin-stimulated ERK1/2 phosphorylation, indicating the proliferation-promoting effects of CCK on rat brain neuroblasts are mediated by both CCK1R and CCK2R (89).
Gastrin and CCK Effects in vivo
The trophic actions of gastrin on gastric mucosa were demonstrated by a sequence of in vivo studies. Two early clinical studies reported that inhibition of gastrin synthesis by gastrectomy results in atrophy of the residual mucosa, suggesting the involvement of gastrin in the regulation of mucosal cell growth (118, 119). Indeed, continuous administration of pentagastrin to male Wistar rats results in an increase in both density and population of gastric mucosal parietal cells (120, 121). Inspired by the above discoveries, Willems and colleagues, using histamine immunocytochemistry and autoradiography after labeling of mucosal specimens with 3H-thymidine, first showed sustained administration of porcine gastrin causes a marked increase in DNA synthesis and in the mitotic index in canine fundic mucosa (122). Similarly, endogenous and exogenous hypergastrinemia, induced by antrocolic transposition, antral exclusion, and subcutaneous infusion of G17 were reported to lead to elevated cell proliferation rates of mucosal cells and oxyntic mucosal thickness in rats (123–125). Using BxPC-3 cell-xenografted mice, Smith et al. first demonstrated that gastrin, but not CCK, stimulates pancreatic tumor growth in a tonic and autocrine fashion (67, 68).
Extensive rodent studies have demonstrated that hypergastrinemia induced by continuous treatment of acid-suppressing drugs, including PPIs and H2 histamine receptor antagonists, results in mucosal hyperplasia and ECL cell hyperplasia. In rare cases, ECL cell neuroendocrine tumors (NETs) might develop although these generally remain benign (126, 127). However, Mastomys, a hypergastrinemic rodent model that is genetically susceptible to spontaneous formation of gastric NETs, can develop gastric carcinoid tumor in the presence of normal serum gastrin levels, likely through the constitutive activation of CCK2R (128, 129). In humans, gastrin is known to promote proliferation of ECL cells and pathogenesis of gastric NETs. Indeed, hyperplasia-dysplasia-neoplasia processes in ECL cell populations were reported in hypergastrinemic conditions (130–132). However, it is still of debate whether hypergastrinemia alone is sufficient to result in ECL cell NETs. Patients with sporadic Zollinger-Ellison syndrome (ZES) rarely develop gastric NETs even though their circulating gastrin levels are over 10-fold above normal for a long period of time (133, 134). In contrast, gastric NETs were reported in 13–30% of patients combined with ZES and familial multiple endocrine neoplasia type I (MEN-1, an autosomal dominantly inherited disorder caused by inactivation of MEN-1 gene), indicating that gastrin and genetic factors are both important for the formation of gastric NETs (135, 136).
In addition, the ability of CCK to induce pancreatic hyperplasia and hypertrophy was also reported by several in vivo studies. Sustained subcutaneous injection of cerulein, a structural and functional homolog of CCK, in rats initiates a significant dose- and time-dependent increase in pancreatic weight and DNA, RNA, and protein contents (137, 138). Similar effects of CCK-8 were observed in male Wistar rats by Rosewicz et al., who further reported the trophic actions of CCK analog might be mediated by induction of pancreatic ornithine decarboxylase (ODC) activity and subsequent accumulation of polyamines, which are closely involved in cellular growth and proliferation due to their ability to facilitate almost all aspects of DNA, RNA, and protein syntheses (139, 140). Indeed, the cerulein-induced pancreatic acinar cell growth is inhibited by an irreversible ODC inhibitor α-difluoromethylornithine and this inhibition is further reversed by administration of the small polyamine putrescine (141, 142).
Very recently, an elegant study by Stanic and his colleagues revealed that CCK, signaling through CCK1R, is involved in regulating neurogenesis in the female adult mouse brain (143). In this study, transgenic mice lacking Cck1r (Cck1r−/−) have decreased proliferating cells in the subgranular zone of the dentate gyrus and rostral migratory stream, with 42% and 29% lower number of cells immune-stained with Ki67 (a nuclear protein associated with cell proliferation) and Doublecortin (a microtubule-associated protein expressed in migrating and differentiating neurons), respectively (143–145). The decreased proliferating precursor cells in female Cck1r −/− mice further result in fewer migratory neuroblasts and tyrosine hydroxylase-immunoreactive mature interneurons in the olfactory bulb compared to the wild-type (WT) mice (143). Similarly, the proliferation-promoting effects of CCK on neurons were confirmed by Reisi et al., who showed that intraperitoneal injection of CCK in male Wistar rats promotes neurogenesis, as evidenced by significantly enhanced Ki-67 positive cells in the granular layer of hippocampal dentate gyrus than those treated with placebo (146).
Mechanism of Proliferative Actions of Gastrin and CCK
Physiologically, two sources of gastrin, originating from mucosal G cells and gastrointestinal tumors, have been suggested to act in different fashions to promote the pathogenesis and development of certain types of cancer. However, the signaling pathways mediating gastrin- and CCK-induced growth-promoting effects are still not fully understood. Since the processes of cellular proliferation are regulated by a complex signaling network of phosphorylation events initiated by the interaction of growth factors with their specific cellular receptors (147), we depicted the critical pathways that might be responsible for gastrin- or CCK-induced trophic effects in Figure 1, based on results obtained from various cell models. These include Gq-mediated phosphatidyl inositol turnover, Ca2+ mobilization, and PKC phosphorylation, Gs-mediated intracellular cAMP accumulation, Gα12/13-mediated Rho-dependent tyrosine phosphorylation of FAKs, activation of MAPK, Src, and PI3K/AKT, as well as transactivation of EGFR, which ultimately results in the regulation of target genes and contribute to proliferative actions of gastrin or CCK (5, 85, 148).
Interestingly, heterodimerization of CCK2R and CCK1R has been also implicated in promoting CCK-induced cell proliferation. In CCK1R-tansfected COS cells, the presence of CCK1R oligomeric complexes was demonstrated by Cheng and Miller in bioluminescence resonance energy transfer and co-immunoprecipitation experiments (149). CCK occupation of CCK1R induces the dissociation of those complexes in a concentration-dependent but phosphorylation-independent manner (149). The same group further showed that heterodimers composed of CCK2R and CCK1R display novel functional and regulatory properties with increased intracellular Ca2+ mobilization and delayed receptor internalization in response to CCK stimulation compared to these in cells only expressing individual receptor (150). It should be noted that CCK1R/CCK2R heterodimer-expressing COS cells tend to have enhanced CCK-induced cell proliferation responses, indicating a stimulant role of heterodimerization on the trophic effects of CCK (150).
Gastrin and CCK in Apoptosis
Gastrin and CCK Effects in vitro
Compared to the well-described proliferative actions of gastrin and CCK, their involvement in the regulation of apoptosis is, however, poorly understood. To date, whether gastrin and CCK exert apoptotic or anti-apoptotic effect is still of debate and might be dependent on the exact physiological and pathological conditions.
Using flow cytometry and terminal deoxynucleotidyl transferase-mediated dUTP-FITC nick end labeling (TUNEL) method, Todisco et al. first demonstrated that gastrin reverses serum withdrawal-induced cellular apoptosis and promotes AR42J cell survival through PI3K- and p38-dependent activation of AKT (151). The same group further showed AKT inhibits apoptosis through activation of the pro-apoptotic proteins BAD and caspase-9, and transcriptional inactivation of FOXO forkhead transcription factors (152). In MKN-45 cells, blockade of CCK2R and COX-2 by AG-041R (a CCK2R antagonist) and NS-398 (a selective COX-2 inhibitor), respectively, was shown to synergistically induce apoptosis through downregulation of the anti-apoptotic protein BCL-2 and upregulation of the pro-apoptotic Bax (95). Similarly, gastrin-induced MCL-1 expression through CCK2R/PKC/MAPK pathway was also shown to be responsible for decreased apoptosis of AGS-CCK2R cells (153). In vitro study in human retinal pigment epithelial cells showed that CCK suppresses peroxynitrite-triggered cell apoptosis through inhibiting the expression of Fas, Fas-associated death domain (FADD), caspase-8, and BAX (154). In addition, the level of plasminogen activator inhibitor type 2 (PAI-2), a major gastrin-targeted gene implicated as an inhibitor for cell invasion and apoptosis (155, 156), was shown to be elevated in serum and stomach of hypergastrinemic patients and gastrin-treated media (157). Furthermore, by transfecting AGS-CCK2R cells with PAI-2 promoter-luciferase construct, Dockray and his colleagues showed that gastrin dose- and time-dependently induces the expression of PAI-2 luciferase, likely through Gq/PKC/RhoA-dependent activation of transcription factors CREB and AP1 (157).
Despite the anti-apoptotic effects of gastrin and CCK discussed above, gastrin and CCK were also reported to stimulate apoptosis of various cell lines. In human colorectal cancer Lovo cells expressing endogenous CCK2R and Colo320 cells transfected with WT CCK2R (Colo320wt), 10 nM of gastrin significantly increases the number of apoptotic Lovo cells and Colo320 cells by 21 and 42%, respectively, which is completely abolished in the presence of 500 nM of CCK2R antagonist L365-260 (158). Further In vitro signal transduction studies showed that, in Colo320wt cells but not in Colo320 cells lacking CCK2R or expressing loss-of-function CCK2R mutant, gastrin stimulates apoptosis, induces MAPK/ERK/AP-1 cascade, and suppresses the activity of NF-κB, indicating CCK2R mediates gastrin-induced apoptosis (158). Similarly, gastrin-induced apoptosis was also demonstrated in gastric epithelial RGM-1 cells and cholangiocarcinoma Mz-ChA-1 cells (159, 160).
Gastrin and CCK Effects in vivo
To investigate the in vivo effects of gastrin on apoptosis, several hypergastrinemic rodent models, such as INS-GAS mice expressing human gastrin minigene spliced with insulin promoter in pancreatic islets, Mastomys rodents treated with an H2 histamine receptor antagonist, and FVB/N mice treated with a PPI, have been utilized (160–162). Although the ambivalent actions of gastrin were reported in a number of in vitro studies, the majority of evidence from in vivo studies suggested gastrin signaling through CCK2R stimulates gastric cell apoptosis.
Moss and colleagues, using TUNEL technique, showed that Mastomys rodents treated with loxtidine, an irreversible H2 receptor antagonist, for 8 weeks have a 1.8-fold increase in the apoptotic cells in the hyperplastic mucosa and apoptotic cells return to the control levels upon loxtidine withdraw in 10 days (161). Nevertheless, the ratio of fundic mucosal proliferative to apoptotic cells also increases in the loxtidine-treated Mastomys rodents compared to that of the controls (161). In addition, INS-GAS mice and gastrin-infused GAS-KO mice have significantly elevated apoptotic glandular parietal cells, extraglandular mesenchymal cells and infiltrating immune cells, along with increased expression of proapoptotic BAX and decreased expression of BCL-2 compared to the corresponding controls (160). Sustained H. felis infection of INS-GAS mice results in exacerbated hypergastrinemia, increased apoptosis, as well as accelerated progression to atrophy (160). Treatment of H. felis-infected INS-GAS mice with YF476 (a highly specific CCK2R antagonist) and/or loxtidine for 6 months demonstrated that both agents have equivalent suppressing effects on gastric apoptosis and atrophy, and combination of both drugs exert more profound inhibitory effects on gastric cell apoptosis (160). Using hypergastrinemic mice models including INS-GAS and FVB/N mice treated with omeprazole, Przemeck et al. showed that hypergastrinemia renders gastric epithelial cells more susceptible to induction of apoptosis by 12Gy γ-radiation or H. pylori infection, and in both cases these effects are suppressed by CCK2R antagonist YM022 (162).
Similar results were observed in gastric corpus biopsies obtained from H. pylori-infected humans with moderate hypergastrinemia (162). One possible mechanism by which gastrin-induced apoptosis results in gastrointestinal cancers was proposed by Houghton et al., through establishment of the Helicobacter felis/C57BL/6 mouse model of gastric cancer, who demonstrated that chronic Helicobacter felis-induced hypergastrinemia stimulates the apoptosis of gastric stem cells, followed by recruitment and repopulation of bone marrow–derived cells in the gastric mucosa. Subsequently, the bone marrow–derived cells progress through metaplasia and dysplasia to intraepithelial cancer since they are more susceptible to development of malignancy than the originally inhabited gastric epithelial stem cells (163).
In contrast, CCK has been shown to suppress neuronal apoptosis in several animal models. The pilot study of Sugaya et al. demonstrated the ability of CCK to protect the degeneration of cholinergic neurons in a basal forebrain-lesioned rat model, as evidenced by preserved choline acetyltransferase activity and acetylcholine release (164). Similarly, in cultured rat cortical neurons, CCK was shown to inhibit glutamate-induced neuronal death in a dose-dependent manner at concentrations of 1–100 nM. Furthermore, CCK2R was suggested to mediate CCK-induced neuronal protection since this effect was antagonized by the CCK2R antagonist L-365260 but not by the CCK1R antagonist L-364718 (165). In addition, Reisi et al. showed that intraperitoneal injection of CCK-8S, the octapeptide that can rapidly cross the blood-brain barrier and spread across the brain, into male Wistar rats inhibits neuronal apoptosis in the hippocampus, as evidenced by reduced number of TUNEL-positive cells in granular layer of hippocampal dentate gyrus (146). Interestingly. the pilot studies of Lavine et al. demonstrated that CCK is up-regulated and expressed in the pancreatic islet β-cells of obese mice, and whole-body deletion of Cck results in reduced islet size and β-cell mass through increased β-cell apoptosis (166). In cultured β-cells or isolated islets, CCK also functions as a paracrine or autocrine factor to protect β-cells from cytokine- or ER stress-stimulating agent-induced apoptosis (166). Similar anti-apoptotic effects of islet-derived CCK were observed in lean transgenic mice that endogenously express CCK in the β-cells (167).
Mechanism of Gastrin- and CCK-Mediated Effects in Apoptosis
Due to lack of evidence, the exact roles of gastrin and CCK in the regulation of apoptosis are still of debate. Some in vitro studies demonstrated that gastrin and CCK exert an anti-apoptotic effect through PI3K/AKT- and p38/AKT-dependent activation of BAD and caspase-8, CCK2R/COX-2-dependent and Fas/FADD/caspase-8-dependent upregulation of BCL-2 and downregulation of BAX, CCK2R/PKC/MAPK-dependent upregulation of MCL-1, as well as PKC/RhoA/CREB/AP1-dependent activation of PAI-2. However, other studies showed gastrin induces apoptosis via activation of MAPK/ERK/AP-1, blockade of NF-κB, and Ca2+-dependent activation of PKC-α. Recent in vivo studies showed that CCK protects central neurons and pancreatic β-cells from apoptosis in autocrine and/or paracrine manners, whereas the underling mechanisms remain elusive. Further investigations are needed to elucidate gastrin- and CCK-induced effects in apoptosis in the context of cell types and animal models.
Imaging and Therapeutic Perspectives
It has been well-recognized that both the expression of CCK2R and CCK1R are increased in numerous human NETs over the corresponding normal tissues, suggesting that both receptors might be utilized as molecular targets for localization of certain adenocarcinomas by radiopeptide imaging in vivo, and more recently, for treatment by peptide receptor radiation therapy (168, 169). Indeed, inspired by somatostatin receptor scintigraphy, the diagnostic gold standard procedure for the detection of several tumor entities, Gotthardt et al. developed a novel imaging method, gastrin receptor scintigraphy (GRS), for the detection of metastases of medullary thyroid carcinoma (MTC) (170). By comparing different detection methods in 26 patients with metastasized MTC, it was shown that GRS combined with computed tomography is the most effective in MTC detection, with a tumor detection rate of 96.7% (170). In a 60-patient cohort of carcinoids and other NETs the same group suggested that GRS should be performed in selected patients since it may provide additional information in NET patients with equivocal or absent somatostatin uptake (171).
In this scenario, the search of radiolabeled CCK2R ligands with good tumor-to-kidney pharmacodynamics is of great importance in clinical settings. Thirty-four radiolabeled candidate compounds derived from gastrin were screened by measuring tumor and kidney uptake in several pancreatic xenograft nude mouse models, and the peptide with sequence DOTA-HHEAYGWMDF-NH2 showed the highest tumor-to-kidney ratio with saturable uptake in target organs and low uptake by non-target tissues, indicating a promising candidate for peptide receptor radiation therapy (172).
Accumulating evidence showed that gastrin signaling via CCK2R stimulates the growth of gastrointestinal cancer cells in vitro and in vivo, indicating blockade of CCK2R pathway might present a promising strategy for the treatment of gastrointestinal carcinoma. Indeed, in xenografted nude mice transplanted with the mouse colon adenocarcinoma cell line MC-26, proglumide, a weak CCK2R inhibitor, suppresses growth of MC-26 colon cancer and prolongs survival in tumor-bearing mice (173). In addition, decreased mean tumor area, mean tumor weight, and tumor DNA and RNA contents were also observed in proglumide-treated group compared to the control group (173). However, the beneficial effects of proglumide on survival from gastric carcinoma were abolished in a randomized, controlled study of proglumide in 110 gastric tumor patients (174). The authors proposed that more specific and potent CCK2R antagonists, in combination with agents that block gastrin secretion such as somatostatin analogs or prostaglandin analogs, might exert a greater benefit on survival in humans (174).
Furthermore, Watson et al. developed a gastrin-specific monoclonal antibody G17DT, an immunogen composed of the amino terminal portion of G17 linked to a diphtheria toxoid (175). The colon tumor-xenografted rats treated with G17DT were shown to have significantly reduced median cross-sectional tumor area and weights, and increased degree of necrosis compared to control rats (175). Similar survival-promoting effects were observed in severe combined immune deficient mice xenografted with two human gastric cancer lines MGLVA1 cells and ST16 cells (176). In the MGLVA1asc-xenografted mice, the enhancement in survival induced by G17DT was not significantly different from that achieved by treatment with 5-fluorouracil/leucovorin (177). Mice treated with a combination therapy with G17DT and 5-fluorouracil/leucovorin benefit more in survival compared to those treated with G17DT or 5-fluorouracil/leucovorin alone, indicating additive effects of both treatments (177).
Due to the positive results of G17DT achieved in multiple gastrin-sensitive tumor models, the same group further investigated the therapeutic effectiveness of G17DT in clinical trials. G17DT was shown to elicit functional antibodies against gastrin with safe and well-tolerated profile in 52 patients with gastric carcinomas, with the exception that two patients suffered significant adverse reactions (178). In another open-label, multinational, and multicenter phase II study, sixty-five of 94 advanced gastric cancer patients were successfully vaccinated with G17DT in terms of anti-gastrin antibody production and showed longer time-to-progression and median survival compared to control patients (179). A further international multicenter randomized controlled Phase III clinical trial consisting of 154 patients (79 G17DT and 75 placebo) with advanced pancreatic cancer confirmed improved survival of patients in the G17DT group through an intention-to-treat analysis (180). Therefore, G17DT represents a promising therapeutic option for gastrointestinal malignancy.
Conclusions
Since the discovery of gastrin and CCK a century ago as digestion-related gastrointestinal peptides, our understanding of these peptides have considerably improved. Extensive investigations have demonstrated the expression of gastrin, CCK, and their receptors in a variety of tumor cells and tissues and their involvement in the regulation of cell proliferation and apoptosis, as well as the pathogenesis of cancer. Indeed, a complex network of signaling pathways, including Gq/PLC/Ca2+/PKC, Gs/AC/cAMP, and NO/cGMP cascades, activation of kinases such as MAPK, PI3K/AKT, Src, and FAKs, as well as transactivation of the EGFR, have been suggested to contribute to the trophic effects of these two peptides. Finally, diagnosis and treatment approaches targeting peptides and CCK2R, such as receptor scintigraphy and radiopharmaceuticals, have been utilized in tumor imaging and/or therapy in vitro, in vivo, and in clinical trials. However, it should be noted that the CCK2R- and CCK1R-mediated signal transduction varies in the context of cell types, suggesting that cautions should be taken in future investigations attempting to target the gastrin and CCK system for the treatment of certain types of cancer.
Author Contributions
All authors listed have made a substantial, direct and intellectual contribution to the work, and approved it for publication.
Funding
This study was funded by Xiamen Huli Guoyu Clinic, CO., Ltd.
Conflict of Interest
WW and D-YG are employees of Xiamen Huli Guoyu Clinic, Co., Ltd., Xiamen, China.
The remaining authors declare that the research was conducted in the absence of any commercial or financial relationships that could be construed as a potential conflict of interest.
Abbreviations
CCK, cholecystokinin; CCK1R, CCK1 receptor; CCK2R, CCK2 receptor; COX-2, cyclooxygenase-2; epidermal growth factor; EGFR, epidermal growth factor receptor; FAK, focal adhesion tyrosine kinase; Gamide, amidated gastrin; Ggly, Gly-extended gastrin; GRS, gastrin receptor scintigraphy; H. pylori, Helicobacter pylori; JAK, Janus kinase; MAPK, mitogen-activated protein kinase; MEN-1, multiple endocrine neoplasia type I; MTC, medullary thyroid carcinoma; NETs, neuroendocrine tumors; NO, nitric oxide; ODC, ornithine decarboxylase; PAI-2, plasminogen activator inhibitor type 2; PKB, protein kinase B; PKC, protein kinase C; PPI, proton pump inhibitor; TUNEL, terminal deoxynucleotidyl transferase-mediated dUTP-FITC nick end labeling; WT, wild-type.
References
1. Holst JJ, Fahrenkrug J, Stadil F, Rehfeld JF. Gastrointestinal endocrinology. Scand J Gastroenterol. (1996) 216(Suppl.):27–38. doi: 10.3109/00365529609094558
2. Johnson LR, Gastrointestinal hormones and their functions. Annu Rev Physiol. (1977) 39:135–58. doi: 10.1146/annurev.ph.39.030177.001031
3. Dimaline R, Varro A. Novel roles of gastrin. J Physiol. (2014) 592:2951–8. doi: 10.1113/jphysiol.2014.272435
4. Rehfeld JF. Cholecystokinin-from local gut hormone to ubiquitous messenger. Front Endocrinol. (2017) 8:47. doi: 10.3389/fendo.2017.00047
5. Rozengurt E, Walsh JH. Gastrin, CCK, signaling, and cancer. Annu Rev Physiol. (2001) 63:49–76. doi: 10.1146/annurev.physiol.63.1.49
6. Rai R, Chandra V, Tewari M, Kumar M, Shukla HS. Cholecystokinin and gastrin receptors targeting in gastrointestinal cancer. Surg Oncol. (2012) 21:281–92. doi: 10.1016/j.suronc.2012.06.004
7. Aly A, Shulkes A, Baldwin GS. Gastrins, cholecystokinins and gastrointestinal cancer. Biochim Biophys Acta. (2004) 1704:1–10. doi: 10.1016/j.bbcan.2004.01.004
8. Edkins JS. The chemical mechanism of gastric secretion. J Physiol. (1906) 34:133–44. doi: 10.1113/jphysiol.1906.sp001146
9. Ivy AC, Oldberg E. A hormone mechanism for gallbladder contraction and evacuation. Am J Physiol. (1928) 86:559–613. doi: 10.1152/ajplegacy.1928.86.3.599
10. Gregory H, Hardy PM, Jones DS, Kenner GW, Sheppard RC. The antral hormone gastrin: structure of gastrin. Nature. (1964) 204:931–3. doi: 10.1038/204931a0
11. Jorpes E, Mutt V. Cholecystokinin and pancreozymin, one single hormone? Acta Physiol Scand. (1966) 66:196–202. doi: 10.1111/j.1748-1716.1966.tb03185.x
12. Wiborg O, Berglund L, Boel E, Norris F, Norris K, Rehfeld JF, et al. Structure of a human gastrin gene. Proc Natl Acad Sci USA. (1984) 81:1067–9. doi: 10.1073/pnas.81.4.1067
13. Takahashi Y, Kato K, Hayashizaki Y, Wakabayashi T, Ohtsuka E, Matsuki S, et al. Molecular cloning of the human cholecystokinin gene by use of a synthetic probe containing deoxyinosine. Proc Natl Acad Sci USA. (1985) 82:1931–5. doi: 10.1073/pnas.82.7.1931
14. Dockray GJ, Varro A, Dimaline R, Wang T. The gastrins: their production and biological activities. Annu Rev Physiol. (2001) 63:119–39. doi: 10.1146/annurev.physiol.63.1.119
15. Rehfeld JF, Sun G, Christensen T, Hillingso JG. The predominant cholecystokinin in human plasma and intestine is cholecystokinin-33. J Clin Endocrinol Metab. (2001) 86:251–8. doi: 10.1210/jc.86.1.251
16. Rehfeld JF. The art of measuring gastrin in plasma: a dwindling diagnostic discipline? Scand J Clin Lab Invest. (2008) 68:353–61. doi: 10.1080/00365510701771831
17. Johnsen AH, Phylogeny of the cholecystokinin/gastrin family. Front. Neuroendocrinol. (1998) 19:73–99. doi: 10.1006/frne.1997.0163
18. Dockray G, Dimaline R, Varro A. Gastrin: old hormone, new functions. Pflugers Arch. (2005) 449:344–55. doi: 10.1007/s00424-004-1347-5
19. Persson H, Rehfeld JF, Ericsson A, Schalling M, Pelto-Huikko M, Hokfelt T. Transient expression of the cholecystokinin gene in male germ cells and accumulation of the peptide in the acrosomal granule: possible role of cholecystokinin in fertilization. Proc Natl Acad Sci USA. (1989) 86:6166–70. doi: 10.1073/pnas.86.16.6166
20. Panerai AE, Manfredi B, Locatelli L, Rubboli F, Sacerdote P. Age-related changes of beta-endorphin and cholecystokinin in peripheral blood mononuclear cells. Ann N Y Acad Sci. (1991) 621:174–8. doi: 10.1111/j.1749-6632.1991.tb16978.x
21. Sacerdote P, Breda M, Barcellini W, Meroni PL, Panerai AE. Age-related changes of beta-endorphin and cholecystokinin in human and rat mononuclear cells. Peptides. (1991) 12:1353–6. doi: 10.1016/0196-9781(91)90219-F
22. De la Fuente M, Carrasco M, Del Rio M, Hernanz A. Modulation of murine lymphocyte functions by sulfated cholecystokinin octapeptide. Neuropeptides. (1998) 32:225–33. doi: 10.1016/S0143-4179(98)90041-5
23. Meng AH, Ling YL, Zhang XP, Zhang JL. Anti-inflammatory effect of cholecystokinin and its signal transduction mechanism in endotoxic shock rat. World J Gastroenterol. (2002) 8:712–7. doi: 10.3748/wjg.v8.i4.712
24. Aunapuu M, Roosaar P, Jarveots T, Kurrikoff K, Koks S, Vasar E, et al. Altered renal morphology in transgenic mice with cholecystokinin overexpression. Transgenic Res. (2008) 17:1079–89. doi: 10.1007/s11248-008-9204-5
25. Goetze JP, Johnsen AH, Kistorp C, Gustafsson F, Johnbeck CB, Rehfeld JF. Cardiomyocyte expression and cell-specific processing of procholecystokinin. J Biol Chem. (2015) 290:6837–43. doi: 10.1074/jbc.M114.622670
26. Burkitt MD, Varro A, Pritchard DM. Importance of gastrin in the pathogenesis and treatment of gastric tumors. World J Gastroenterol. (2009) 15:1–16. doi: 10.3748/wjg.15.1
27. Konturek PC, Konturek SJ, Brzozowski T. Helicobacter pylori infection in gastric cancerogenesis. J Physiol Pharmacol. (2009) 60:3–21.
28. Thorburn CM, Friedman GD, Dickinson CJ, Vogelman JH, Orentreich N, Parsonnet J. Gastrin and colorectal cancer: a prospective study. Gastroenterology. (1998) 115:275–80. doi: 10.1016/S0016-5085(98)70193-3
29. Zhou JJ, Chen ML, Zhang QZ, Hu JK, Wang WL. Coexpression of cholecystokinin-B/gastrin receptor and gastrin gene in human gastric tissues and gastric cancer cell line. World J Gastroenterol. (2004) 10:791–4. doi: 10.3748/wjg.v10.i6.791
30. Henwood M, Clarke PA, Smith AM, Watson SA. Expression of gastrin in developing gastric adenocarcinoma. Br J Surg. (2001) 88:564–8. doi: 10.1046/j.1365-2168.2001.01716.x
31. Nemeth J, Taylor B, Pauwels S, Varro A, Dockray GJ. Identification of progastrin derived peptides in colorectal carcinoma extracts. Gut. (1993) 34:90–5. doi: 10.1136/gut.34.1.90
32. Van Solinge WW, Nielsen FC, Friis-Hansen L, Falkmer UG, Rehfeld JF. Expression but incomplete maturation of progastrin in colorectal carcinomas. Gastroenterology. (1993) 104:1099–107. doi: 10.1016/0016-5085(93)90279-L
33. Caplin M, Savage K, Khan K, Brett B, Rode J, Varro A, et al. Expression and processing of gastrin in pancreatic adenocarcinoma. Br J Surg. (2000) 87:1035–40. doi: 10.1046/j.1365-2168.2000.01488.x
34. Shulkes A, Baldwin GS. Biology of gut cholecystokinin and gastrin receptors. Clin Exp Pharmacol Physiol. (1997) 24:209–16. doi: 10.1111/j.1440-1681.1997.tb01809.x
35. Cayrol C, Clerc P, Bertrand C, Gigoux V, Portolan G, Fourmy D, et al. Cholecystokinin-2 receptor modulates cell adhesion through beta 1-integrin in human pancreatic cancer cells. Oncogene. (2006) 25:4421–8. doi: 10.1038/sj.onc.1209484
36. Schjoldager BT, Role of CCK in gallbladder function. Ann N Y Acad Sci. (1994) 713:207–18. doi: 10.1111/j.1749-6632.1994.tb44067.x
37. Reuben M, Rising L, Prinz C, Hersey S, Sachs G. Cloning and expression of the rabbit gastric CCK-A receptor. Biochim Biophys Acta. (1994) 1219:321–7. doi: 10.1016/0167-4781(94)90055-8
38. Prinz C, Scott DR, Hurwitz D, Helander HF, Sachs G. Gastrin effects on isolated rat enterochromaffin-like cells in primary culture. Am J Physiol. (1994) 267:G663–75. doi: 10.1152/ajpgi.1994.267.4.G663
39. Nylander AG, Chen D, Lilja I, Axelson J, Ihse I, Rehfeld JF, et al. Enterochromaffin-like cells in rat stomach respond to short-term infusion of high doses of cholecystokinin but not to long-term, sustained, moderate hyperCCKemia caused by continuous cholecystokinin infusion or pancreaticobiliary diversion. Scand J Gastroenterol. (1993) 28:73–9. doi: 10.3109/00365529309096048
40. Sachs G, Zeng N, Prinz C. Physiology of isolated gastric endocrine cells. Annu Rev Physiol. (1997) 59:243–56. doi: 10.1146/annurev.physiol.59.1.243
41. Kopin AS, Lee YM, McBride EW, Miller LJ, Lu M, Lin HY, et al. Expression cloning and characterization of the canine parietal cell gastrin receptor. Proc Natl Acad Sci USA. (1992) 89:3605–9. doi: 10.1073/pnas.89.8.3605
42. Mantyh CR, Pappas TN, Vigna SR. Localization of cholecystokinin A and cholecystokinin B/gastrin receptors in the canine upper gastrointestinal tract. Gastroenterology. (1994) 107:1019–30. doi: 10.1016/0016-5085(94)90226-7
43. Reubi JC, Waser B, Laderach U, Stettler C, Friess H, Halter F, et al. Localization of cholecystokinin A and cholecystokinin B-gastrin receptors in the human stomach. Gastroenterology. (1997) 112:1197–205. doi: 10.1016/S0016-5085(97)70131-8
44. Kulaksiz H, Arnold R, Goke B, Maronde E, Meyer M, Fahrenholz F, et al. Expression and cell-specific localization of the cholecystokinin B/gastrin receptor in the human stomach. Cell Tissue Res. (2000) 299:289–98. doi: 10.1007/s004410050027
45. Tang C, Biemond I, Lamers CB. Cholecystokinin receptors in human pancreas and gallbladder muscle: a comparative study. Gastroenterology. (1996) 111:1621–6. doi: 10.1016/S0016-5085(96)70025-2
46. Honda T, Wada E, Battey JF, Wank SA. Differential gene expression of CCKA and CCKB receptors in the rat brain. Mol Cell Neurosci. (1993) 4:143–54. doi: 10.1006/mcne.1993.1018
47. Iwata N, Murayama T, Matsumori Y, Ito M, Nagata A, Taniguchi T, et al. Autocrine loop through cholecystokinin-B/gastrin receptors involved in growth of human leukemia cells. Blood. (1996) 88:2683–9. doi: 10.1182/blood.V88.7.2683.bloodjournal8872683
48. Schmitz F, Schrader H, Otte J, Schmitz H, Stuber E, Herzig K, et al. Identification of CCK-B/gastrin receptor splice variants in human peripheral blood mononuclear cells. Regul Pept. (2001) 101:25–33. doi: 10.1016/S0167-0115(01)00281-6
49. Monstein HJ, Nylander AG, Salehi A, Chen D, Lundquist I, Hakanson R. Cholecystokinin-A and cholecystokinin-B/gastrin receptor mRNA expression in the gastrointestinal tract and pancreas of the rat and man. A polymerase chain reaction study. Scand J Gastroenterol. (1996) 31:383–90. doi: 10.3109/00365529609006415
50. Jensen RT, Lemp GF, Gardner JD. Interaction of cholecystokinin with specific membrane receptors on pancreatic acinar cells. Proc Natl Acad Sci USA. (1980) 77:2079–83. doi: 10.1073/pnas.77.4.2079
51. Shaw MJ, Hadac EM, Miller LJ. Preparation of enriched plasma membranes from bovine gallbladder muscularis for characterization of cholecystokinin receptors. J Biol Chem. (1987) 262:14313–8.
52. Qian JM, Rowley WH, Jensen RT. Gastrin and CCK activate phospholipase C and stimulate pepsinogen release by interacting with two distinct receptors. Am J Physiol. (1993) 264:G718–27. doi: 10.1152/ajpgi.1993.264.4.G718
53. Soll AH, Amirian DA, Thomas LP, Park J, Elashoff JD, Beaven MA, et al. Gastrin receptors on nonparietal cells isolated from canine fundic mucosa. Am J Physiol. (1984) 247:G715–23. doi: 10.1152/ajpgi.1984.247.6.G715
54. Weinberg DS, Ruggeri B, Barber MT, Biswas S, Miknyocki S, Waldman SA. Cholecystokinin A and B receptors are differentially expressed in normal pancreas and pancreatic adenocarcinoma. J Clin Invest. (1997) 100:597–603. doi: 10.1172/JCI119570
55. May AA, Liu M, Woods SC, Begg DP. CCK increases the transport of insulin into the brain. Physiol Behav. (2016) 165:392–7. doi: 10.1016/j.physbeh.2016.08.025
56. Innis RB, Snyder SH. Distinct cholecystokinin receptors in brain and pancreas. Proc Natl Acad Sci USA. (1980) 77:6917–21. doi: 10.1073/pnas.77.11.6917
57. Ashurst HL, Varro A, Dimaline R. Regulation of mammalian gastrin/CCK receptor (CCK2R) expression in vitro and in vivo. Exp Physiol. (2008) 93:223–36. doi: 10.1113/expphysiol.2007.040683
58. Watson SA, Durrant LG, Wencyk PM, Watson AL, Morris DL. Intracellular gastrin in human gastrointestinal tumor cells. J Natl Cancer Inst. (1991) 83:866–71. doi: 10.1093/jnci/83.12.866
59. Matsushima Y, Kinoshita Y, Nakata H, Inomoto-Naribayashi Y, Asahara M, Kawanami C, et al. Gastrin receptor gene expression in several human carcinomas. Jpn J Cancer Res. (1994) 85:819–24. doi: 10.1111/j.1349-7006.1994.tb02953.x
60. Ito M, Tanaka S, Maeda M, Takamura A, Tatsugami M, Wada Y, et al. Role of the gastrin-gastrin receptor system in the expansive growth of human gastric neoplasms. Digestion. (2008) 78:163–70. doi: 10.1159/000181146
61. Okada N, Kubota A, Imamura T, Suwa H, Kawaguchi Y, Ohshio G, et al. Evaluation of cholecystokinin, gastrin, CCK-A receptor, and CCK-B/gastrin receptor gene expressions in gastric cancer. Cancer Lett. (1996) 106:257–62. doi: 10.1016/0304-3835(96)04325-X
62. Clerc P, Dufresne M, Saillan C, Chastre E, Andre T, Escrieut C, et al. Differential expression of the CCK-A and CCK-B/gastrin receptor genes in human cancers of the esophagus, stomach and colon. Int J Cancer. (1997) 72:931–6.
63. Hur K, Kwak MK, Lee HJ, Park DJ, Lee HK, Lee HS, et al. Expression of gastrin and its receptor in human gastric cancer tissues. J Cancer Res Clin Oncol. (2006) 132:85–91. doi: 10.1007/s00432-005-0043-y
64. Reubi JC, Waser B, Schmassmann A, Laissue JA. Receptor autoradiographic evaluation of cholecystokinin, neurotensin, somatostatin and vasoactive intestinal peptide receptors in gastro-intestinal adenocarcinoma samples: where are they really located? Int J Cancer. (1999) 81:376–86. doi: 10.1002/(SICI)1097-0215(19990505)81:3<376::AID-IJC11>3.0.CO;2-5
65. Smith JP, Rickabaugh CA, McLaughlin PJ, Zagon IS. Cholecystokinin receptors and PANC-1 human pancreatic cancer cells. Am J Physiol. (1993) 265:G149–55. doi: 10.1152/ajpgi.1993.265.1.G149
66. Smith JP, Fantaskey AP, Liu G, Zagon IS. Identification of gastrin as a growth peptide in human pancreatic cancer. Am J Physiol. (1995) 268:R135–41. doi: 10.1152/ajpregu.1995.268.1.R135
67. Matters GL, McGovern C, Harms JF, Markovic K, Anson K, Jayakumar C, et al. Role of endogenous cholecystokinin on growth of human pancreatic cancer. Int J Oncol. (2011) 38:593–601. doi: 10.3892/ijo.2010.886
68. Smith JP, Shih A, Wu Y, McLaughlin PJ, Zagon IS. Gastrin regulates growth of human pancreatic cancer in a tonic and autocrine fashion. Am J Physiol. (1996) 270:R1078–84. doi: 10.1152/ajpregu.1996.270.5.R1078
69. Smith JP, Liu G, Soundararajan V, McLaughlin PJ, Zagon IS. Identification and characterization of CCK-B/gastrin receptors in human pancreatic cancer cell lines. Am J Physiol. (1994) 266:R277–83. doi: 10.1152/ajpregu.1994.266.1.R277
70. Moonka R, Zhou W, Bell RH Jr. Cholecystokinin-A receptor messenger RNA expression in human pancreatic cancer. J Gastrointest Surg. (1999) 3:134–40. doi: 10.1016/S1091-255X(99)80022-5
71. Goetze JP, Nielsen FC, Burcharth F, Rehfeld JF. Closing the gastrin loop in pancreatic carcinoma: coexpression of gastrin and its receptor in solid human pancreatic adenocarcinoma. Cancer. (2000) 88:2487–94. doi: 10.1002/1097-0142(20000601)88:11<2487::AID-CNCR9>3.0.CO;2-E
72. Reubi JC, Waser B, Gugger M, Friess H, Kleeff J, Kayed H, et al. Distribution of CCK1 and CCK2 receptors in normal and diseased human pancreatic tissue. Gastroenterology. (2003) 125:98–106. doi: 10.1016/S0016-5085(03)00697-8
73. Biagini P, Monges G, Vuaroqueaux V, Parriaux D, Cantaloube JF, De Micco P. The human gastrin/cholecystokinin receptors: type B and type C expression in colonic tumors and cell lines. Life Sci. (1997) 61:1009–18. doi: 10.1016/S0024-3205(97)00605-X
74. Upp JR Jr, Singh P, Townsend CM Jr, Thompson JC. Clinical significance of gastrin receptors in human colon cancers. Cancer Res. (1989)49:488–92.
75. Lu L, Logsdon CD. CCK, bombesin, and carbachol stimulate c-fos, c-jun, and c-myc oncogene expression in rat pancreatic acini. Am J Physiol. (1992) 263:G327–32. doi: 10.1152/ajpgi.1992.263.3.G327
76. Finley GG, Koski RA, Melhem MF, Pipas JM, Meisler AI. Expression of the gastrin gene in the normal human colon and colorectal adenocarcinoma. Cancer Res. (1993) 53:2919–26.
77. Imdahl A, Mantamadiotis T, Eggstein S, Farthmann EH, Baldwin GS. Expression of gastrin, gastrin/CCK-B and gastrin/CCK-C receptors in human colorectal carcinomas. J Cancer Res Clin Oncol. (1995) 121:661–6. doi: 10.1007/BF01218524
78. Schmitz F, Otte JM, Stechele HU, Reimann B, Banasiewicz T, Folsch UR, et al. CCK-B/gastrin receptors in human colorectal cancer. Eur J Clin Invest. (2001) 31:812–20. doi: 10.1046/j.1365-2362.2001.00870.x
79. Haigh CR, Attwood SE, Thompson DG, Jankowski JA, Kirton CM, Pritchard DM, et al. Gastrin induces proliferation in Barrett's metaplasia through activation of the CCK2 receptor. Gastroenterology. (2003) 124:615–25. doi: 10.1053/gast.2003.50091
80. AbdAlla SI, Lao-Sirieix P, Novelli MR, Lovat LB, Sanderson IR, FitzGerald RC. Gastrin-induced cyclooxygenase-2 expression in barrett's carcinogenesis. Clin Cancer Res. (2004) 10:4784–92. doi: 10.1158/1078-0432.CCR-04-0015
81. Matsumori Y, Katakami N, Ito M, Taniguchi T, Iwata N, Takaishi T, et al. Cholecystokinin-B/gastrin receptor: a novel molecular probe for human small cell lung cancer. Cancer Res. (1995) 55:276–9.
82. Reubi JC, Waser B. Unexpected high incidence of cholecystokinin-B/gastrin receptors in human medullary thyroid carcinomas. Int J Cancer. (1996) 67:644–7. doi: 10.1002/(SICI)1097-0215(19960904)67:5<644::AID-IJC9>3.0.CO;2-U
83. Rai R, Tewari M, Kumar M, Singh TB, Shukla HS. Expression profile of cholecystokinin type-A receptor in gallbladder cancer and gallstone disease. Hepatobiliary Pancreat Dis Int. (2011) 10:408–14. doi: 10.1016/S1499-3872(11)60069-
84. Roy J, Putt KS, Coppola D, Leon ME, Khalil FK, Centeno BA, et al. Assessment of cholecystokinin 2 receptor (CCK2R) in neoplastic tissue. Oncotarget. (2016) 7:14605–15. doi: 10.18632/oncotarget.7522
85. Reubi JC. Targeting CCK receptors in human cancers. Curr Top Med Chem. (2007) 7:1239–42. doi: 10.2174/156802607780960546
86. Hellmich MR, Rui XL, Hellmich HL, Fleming RY, Evers BM, Townsend CM Jr. Human colorectal cancers express a constitutively active cholecystokinin-B/gastrin receptor that stimulates cell growth. J Biol Chem. (2000) 275:32122–8. doi: 10.1074/jbc.M005754200
87. Chao C, Goluszko E, Lee YT, Kolokoltsov AA, Davey RA, Uchida T, et al. Constitutively active CCK2 receptor splice variant increases src-dependent HIF-1 expression and tumor growth. Oncogene. (2007) 26:1013–9. doi: 10.1038/sj.onc.1209862
88. Willard MD, Lajiness ME, Wulur IH, Feng B, Swearingen ML, Uhlik MT, et al. Somatic mutations in CCK2R alter receptor activity that promote oncogenic phenotypes. Mol Cancer Res. (2012) 10:739–49. doi: 10.1158/1541-7786.MCR-11-0483
89. Langmesser S, Cerezo-Guisado MI, Lorenzo MJ, Garcia-Marin LJ, Bragado MJ. CCK1 and 2 receptors are expressed in immortalized rat brain neuroblasts: intracellular signals after cholecystokinin stimulation. J Cell Biochem. (2007) 100:851–64. doi: 10.1002/jcb.21193
90. Christophe J. Pancreatic tumoral cell line AR42J: an amphicrine model. Am J Physiol. (1994) 266:G963–71. doi: 10.1152/ajpgi.1994.266.6.G963
91. Kaufmann R, Schafberg H, Rudroff C, Henklein P, Nowak G. Cholecystokinin B-type receptor signaling is involved in human pancreatic cancer cell growth. Neuropeptides. (1997) 31:573–83. doi: 10.1016/S0143-4179(97)90003-2
92. Xu Y, Kaji H, Okimura Y, Matsui T, Abe H, Chihara K. Paracrine stimulation of cell growth by cholecystokinin/gastrin through cholecystokinin-B receptor on GH3 cells in vitro. Neuroendocrinology. (1996) 64:280–5. doi: 10.1159/000127129
93. Colucci R, Blandizzi C, Tanini M, Vassalle C, Breschi MC, Del Tacca M. Gastrin promotes human colon cancer cell growth via CCK-2 receptor-mediated cyclooxygenase-2 induction and prostaglandin E2 production. Br J Pharmacol. (2005) 144:338–48. doi: 10.1038/sj.bjp.0706053
94. Todisco A, Takeuchi Y, Seva C, Dickinson CJ, Yamada T. Gastrin and glycine-extended progastrin processing intermediates induce different programs of early gene activation. J Biol Chem. (1995) 270:28337–41. doi: 10.1074/jbc.270.47.28337
95. Sun WH, Zhu F, Chen GS, Su H, Luo C, Zhao QS, et al. Blockade of cholecystokinin-2 receptor and cyclooxygenase-2 synergistically induces cell apoptosis, and inhibits the proliferation of human gastric cancer cells in vitro. Cancer Lett. (2008) 263:302–11. doi: 10.1016/j.canlet.2008.01.012
96. Guo YS, Cheng JZ, Jin GF, Gutkind JS, Hellmich MR, Townsend CM Jr. Gastrin stimulates cyclooxygenase-2 expression in intestinal epithelial cells through multiple signaling pathways. Evidence for involvement of ERK5 kinase and transactivation of the epidermal growth factor receptor. J Biol Chem. (2002) 277:48755–63. doi: 10.1074/jbc.M209016200
97. Taniguchi T, Matsui T, Ito M, Murayama T, Tsukamoto T, Katakami Y, et al. Cholecystokinin-B/gastrin receptor signaling pathway involves tyrosine phosphorylations of p125FAK and p42MAP. Oncogene. (1994) 9:861–7.
98. Seufferlein T, Withers DJ, Broad S, Herget T, Walsh JH, Rozengurt E. The human CCKB/gastrin receptor transfected into rat1 fibroblasts mediates activation of MAP kinase, p74raf-1 kinase, and mitogenesis. Cell Growth Differ. (1995) 6:383–93.
99. Detjen K, Yule D, Tseng MJ, Williams JA, Logsdon CD. CCK-B receptors produce similar signals but have opposite growth effects in CHO and swiss 3T3 cells. Am J Physiol. (1997) 273:C1449–57. doi: 10.1152/ajpcell.1997.273.5.C1449
100. Ogasa M, Miyazaki Y, Hiraoka S, Kitamura S, Nagasawa Y, Kishida O, et al. Gastrin activates nuclear factor kappaB (NFkappaB) through a protein kinase C dependent pathway involving NFkappaB inducing kinase, inhibitor kappaB (IkappaB) kinase, and tumour necrosis factor receptor associated factor 6 (TRAF6) in MKN-28 cells transfected with gastrin receptor. Gut. (2003) 52:813–9. doi: 10.1136/gut.52.6.813
101. Kowalski-Chauvel A, Pradayrol L, Vaysse N, Seva C. Gastrin stimulates tyrosine phosphorylation of insulin receptor substrate 1 and its association with Grb2 and the phosphatidylinositol 3-kinase. J Biol Chem. (1996) 271:26356–61. doi: 10.1074/jbc.271.42.26356
102. Ferrand A, Kowalski-Chauvel A, Bertrand C, Escrieut C, Mathieu A, Portolan G, et al. A novel mechanism for JAK2 activation by a G protein-coupled receptor, the CCK2R: implication of this signaling pathway in pancreatic tumor models. J Biol Chem. (2005) 280:10710–5. doi: 10.1074/jbc.M413309200
103. Dehez S, Daulhac L, Kowalski-Chauvel A, Fourmy D, Pradayrol L, Seva C. Gastrin-induced DNA synthesis requires p38-MAPK activation via PKC/Ca2+ and Src-dependent mechanisms. FEBS Lett. (2001) 496:25–30. doi: 10.1016/S0014-5793(01)02396-1
104. Miyazaki Y, Shinomura Y, Tsutsui S, Zushi S, Higashimoto Y, Kanayama S, et al. Gastrin induces heparin-binding epidermal growth factor-like growth factor in rat gastric epithelial cells transfected with gastrin receptor. Gastroenterology. (1999) 116:78–89. doi: 10.1016/S0016-5085(99)70231-3
105. Yule DI, Tseng MJ, Williams JA, Logdson CD. A cloned CCK-A receptor transduces multiple signals in response to full and partial agonists. Am J Physiol. (1993) 265:G999–1004. doi: 10.1152/ajpgi.1993.265.5.G999
106. Sjodin L, Gardner JD. Effect of cholecystokinin variant (CCK39) on dispersed acinar cells from guinea pig pancreas. Gastroenterology. (1977) 73:1015–8. doi: 10.1016/S0016-5085(19)31850-5
107. Cordelier P, Esteve JP, Rivard N, Marletta M, Vaysse N, Susini C, et al. The activation of neuronal NO synthase is mediated by G-protein subunit and the tyrosine phosphatase SHP-2. FASEB J. (1999) 13:2037–50. doi: 10.1096/fasebj.13.14.2037
108. Ahn SH, Seo DW, Ko YK, Sung DS, Bae GU, Yoon JW, et al. NO/cGMP pathway is involved in exocrine secretion from rat pancreatic acinar cells. Arch Pharm Res. (1998) 21:657–63. doi: 10.1007/BF02976753
109. Todisco A, Takeuchi Y, Urumov A, Yamada J, Stepan VM, Yamada T. Molecular mechanisms for the growth factor action of gastrin. Am J Physiol. (1997) 273:G891–8. doi: 10.1152/ajpgi.1997.273.4.G891
110. Stepan VM, Dickinson CJ, del Valle J, Matsushima M, Todisco A. Cell type-specific requirement of the MAPK pathway for the growth factor action of gastrin. Am J Physiol. (1999) 276:G1363–72. doi: 10.1152/ajpgi.1999.276.6.G1363
111. Daulhac L, Kowalski-Chauvel A, Pradayrol L, Vaysse N, Seva C. Src-family tyrosine kinases in activation of ERK-1 and p85/p110-phosphatidylinositol 3-kinase by G/CCKB receptors. J Biol Chem. (1999) 274:20657–63. doi: 10.1074/jbc.274.29.20657
112. Stepan VM, Tatewaki M, Matsushima M, Dickinson CJ, del Valle J, Todisco A. Gastrin induces c-fos gene transcription via multiple signaling pathways. Am J Physiol. (1999) 276:G415–24. doi: 10.1152/ajpgi.1999.276.2.G415
113. Zhukova E, Sinnett-Smith J, Wong H, Chiu T, Rozengurt E. CCK(B)/gastrin receptor mediates synergistic stimulation of DNA synthesis and cyclin D1, D3, and E expression in swiss 3T3 cells. J Cell Physiol. (2001) 189:291–305. doi: 10.1002/jcp.10018
114. Pradeep A., Sharma C, Sathyanarayana P, Albanese C, Fleming JV, Wang TC, et al. Gastrin-mediated activation of cyclin D1 transcription involves beta-catenin and CREB pathways in gastric cancer cells. Oncogene. (2004) 23:3689–99. doi: 10.1038/sj.onc.1207454
115. Slice LW, Hodikian R, Zhukova E. Gastrin and EGF synergistically induce cyclooxygenase-2 expression in swiss 3T3 fibroblasts that express the CCK2 receptor. J Cell Physiol. (2003) 196:454–63. doi: 10.1002/jcp.10304
116. Sakai R, Iwamatsu A, Hirano N, Ogawa S, Tanaka T, Mano H, et al. A novel signaling molecule, p130, forms stable complexes in vivo with v-Crk and v-Src in a tyrosine phosphorylation-dependent manner. EMBO J. (1994) 13:3748–56. doi: 10.1002/j.1460-2075.1994.tb06684.x
117. Schaller MD. Paxillin: a focal adhesion-associated adaptor protein. Oncogene. (2001) 20:6459–72. doi: 10.1038/sj.onc.1204786
118. Lees F, Grandjean LC. The gastric and jejunal mucosae in healthy patients with partial gastrectomy. AMA Arch Intern Med. (1958) 101:943–51. doi: 10.1001/archinte.1958.00260170099013
119. Gjeruldsen ST, Myren J, Fretheim B. Alterations of gastric mucosa following a graded partial gastrectomy for duodenal ulcer. Scand J Gastroenterol. (1968) 3:465–70. doi: 10.3109/00365526809179904
120. Crean GP, Marshall MW, Rumsey RD. Parietal cell hyperplasia induced by the administration of pentagastrin (ICI 50,123) to rats. Gastroenterology. (1969) 57:147–55. doi: 10.1016/S0016-5085(19)33930-7
121. Johnson LR, Chandler AM. RNA and DNA of gastric and duodenal mucosa in antrectomized and gastrin-treated rats. Am J Physiol. (1973) 224:937–40. doi: 10.1152/ajplegacy.1973.224.4.937
122. Willems G, Vansteenkiste Y, Limbosch JM. Stimulating effect of gastrin on cell proliferation kinetics in canine fundic mucosa. Gastroenterology. (1972) 62:583–9. doi: 10.1016/S0016-5085(72)80042-8
123. Lehy T, Bonnefond A, Dubrasquet M, Nasca S, Lewin M, Bonfils S. Comparative effects of antrocolic transposition and antrectomy on fundic mucosa and acid secretion of the rat. Gastroenterology. (1973) 64:421–8. doi: 10.1016/S0016-5085(73)80165-9
124. Hakanson R, Blom H, Carlsson E, Larsson H, Ryberg B, Sundler F. Hypergastrinaemia produces trophic effects in stomach but not in pancreas and intestines. Regul Pept. (1986) 13:225–33. doi: 10.1016/0167-0115(86)90041-8
125. Ryberg B, Tielemans Y, Axelson J, Carlsson E, Hakanson R, Mattson H, et al. Gastrin stimulates the self-replication rate of enterochromaffinlike cells in the rat stomach. Effects of omeprazole, ranitidine, and gastrin-17 in intact and antrectomized rats. Gastroenterology. (1990) 99:935–42. doi: 10.1016/0016-5085(90)90610-D
126. Betton GR, Dormer CS, Wells T, Pert P, Price CA, Buckley P. Gastric ECL-cell hyperplasia and carcinoids in rodents following chronic administration of H2-antagonists SK&F 93479 and oxmetidine and omeprazole. Toxicol Pathol. (1988) 16:288–98. doi: 10.1177/019262338801600222
127. Ekman L, Hansson E, Havu N, Carlsson E, Lundberg C. Toxicological studies on omeprazole. Scand J Gastroenterol. (1985) 108(Suppl.):53–69.
128. Modlin IM, Tang LH. The gastric enterochromaffin-like cell: an enigmatic cellular link. Gastroenterology. (1996) 111:783–810. doi: 10.1053/gast.1996.v111.agast961110783
129. Schaffer K, McBride EW, Beinborn M, Kopin AS. Interspecies polymorphisms confer constitutive activity to the mastomys cholecystokinin-B/gastrin receptor. J Biol Chem. (1998) 273:28779–84. doi: 10.1074/jbc.273.44.28779
130. Bordi C, Yu JY, Baggi MT, Davoli C, Pilato FP, Baruzzi G, et al. Gastric carcinoids and their precursor lesions. A histologic and immunohistochemical study of 23 cases. Cancer. (1991) 67:663–72. doi: 10.1002/1097-0142(19910201)67:3<663::AID-CNCR2820670323>3.0.CO;2-L
131. Creutzfeldt W. The achlorhydria-carcinoid sequence: role of gastrin. Digestion. (1988) 39:61–79. doi: 10.1159/000199609
132. Jordan PH Jr, Barroso A, Sweeney J. Gastric carcinoids in patients with hypergastrinemia. J Am Coll Surg. (2004) 199:552–5. doi: 10.1016/j.jamcollsurg.2004.06.019
133. Feurle GE. Argyrophil cell hyperplasia and a carcinoid tumour in the stomach of a patient with sporadic zollinger-ellison syndrome. Gut. (1994) 35:275–7. doi: 10.1136/gut.35.2.275
134. Cadiot G, Vissuzaine C, Potet F, Mignon M. Fundic argyrophil carcinoid tumor in a patient with sporadic-type zollinger-ellison syndrome. Dig Dis Sci. (1995) 40:1275–8. doi: 10.1007/BF02065537
135. Debelenko LV, Emmert-Buck MR, Zhuang Z, Epshteyn E, Moskaluk CA, Jensen RT, et al. The multiple endocrine neoplasia type I gene locus is involved in the pathogenesis of type II gastric carcinoids. Gastroenterology. (1997) 113:773–81. doi: 10.1016/S0016-5085(97)70171-9
136. Lehy T, Cadiot G, Mignon M, Ruszniewski P, Bonfils S. Influence of multiple endocrine neoplasia type 1 on gastric endocrine cells in patients with the zollinger-ellison syndrome. Gut. (1992) 33:1275–9. doi: 10.1136/gut.33.9.1275
137. Solomon TE, Petersen H, Elashoff J, Grossman MI. Interaction of caerulein and secretin on pancreatic size and composition in rat. Am J Physiol. (1978) 235:E714–9. doi: 10.1152/ajpendo.1978.235.6.E714
138. Dembinski AB, Johnson LR. Stimulation of pancreatic growth by secretin, caerulein, and pentagastrin. Endocrinology. (1980) 106:323–8. doi: 10.1210/endo-106-1-323
139. Rosewicz S, Lewis LD, Liddle RA, Logsdon CD. Effects of cholecystokinin on pancreatic ornithine decarboxylase gene expression. Am J Physiol. (1988) 255:G818–21. doi: 10.1152/ajpgi.1988.255.6.G818
140. Morisset J, Benrezzak O. Polyamines and pancreatic growth induced by caerulein. Life Sci. (1984) 35:2471–80. doi: 10.1016/0024-3205(84)90456-9
141. Benrezzak O, Morisset J. Effects of -difluoromethylornithine on pancreatic growth induced by caerulein. Regul Pept. (1984) 9:143–53. doi: 10.1016/0167-0115(84)90067-3
142. Morisset J, Benrezzak O. Reversal of alpha-difluoromethylornithine inhibition of caerulein-induced pancreatic growth by putrescine. Regul Pept. (1985) 11:201–8. doi: 10.1016/0167-0115(85)90051-5
143. Sui Y, Vermeulen R, Hokfelt T, Horne MK, Stanic D. Female mice lacking cholecystokinin 1 receptors have compromised neurogenesis, and fewer dopaminergic cells in the olfactory bulb. Front Cell Neurosci. (2013) 7:13. doi: 10.3389/fncel.2013.00013
144. Gerdes J, Schwab U, Lemke H, Stein H. Production of a mouse monoclonal antibody reactive with a human nuclear antigen associated with cell proliferation. Int J Cancer. (1983) 31:13–20. doi: 10.1002/ijc.2910310104
145. Francis F, Koulakoff A, Boucher D, Chafey P, Schaar B, Vinet MC, et al. Doublecortin is a developmentally regulated, microtubule-associated protein expressed in migrating and differentiating neurons. Neuron. (1999) 23:247–56. doi: 10.1016/S0896-6273(00)80777-1
146. Reisi P, Ghaedamini AR, Golbidi M, Shabrang M, Arabpoor Z, Rashidi B. Effect of cholecystokinin on learning and memory, neuronal proliferation and apoptosis in the rat hippocampus. Adv Biomed Res. (2015) 4:227. doi: 10.4103/2277-9175.166650
147. Hill CS, Treisman R. Transcriptional regulation by extracellular signals: mechanisms and specificity. Cell. (1995) 80:199–211. doi: 10.1016/0092-8674(95)90403-4
148. Reubi JC, Schaer JC, Waser B. Cholecystokinin(CCK)-A and CCK-B/gastrin receptors in human tumors. Cancer Res. (1997) 57:1377–86.
149. Cheng ZJ, Miller LJ. Agonist-dependent dissociation of oligomeric complexes of G protein-coupled cholecystokinin receptors demonstrated in living cells using bioluminescence resonance energy transfer. J Biol Chem. (2001) 276:48040–7. doi: 10.1074/jbc.M105668200
150. Cheng ZJ, Harikumar KG, Holicky EL, Miller LJ. Heterodimerization of type A and B cholecystokinin receptors enhance signaling and promote cell growth. J Biol Chem. (2003) 278:52972–9. doi: 10.1074/jbc.M310090200
151. Todisco A, Ramamoorthy S, Witham T, Pausawasdi N, Srinivasan S, Dickinson CJ, et al. Molecular mechanisms for the antiapoptotic action of gastrin. Am J Physiol Gastrointest Liver Physiol. (2001) 280:G298–307. doi: 10.1152/ajpgi.2001.280.2.G298
152. Ramamoorthy S, Stepan V, Todisco A. Intracellular mechanisms mediating the anti-apoptotic action of gastrin. Biochem Biophys Res Commun. (2004) 323:44–8. doi: 10.1016/j.bbrc.2004.08.059
153. Pritchard DM, Berry D, Przemeck SM, Campbell F, Edwards SW, Varro A. Gastrin increases mcl-1 expression in type I gastric carcinoid tumors and a gastric epithelial cell line that expresses the CCK-2 receptor. Am J Physiol Gastrointest Liver Physiol. (2008) 295:G798–805. doi: 10.1152/ajpgi.00015.2008
154. Liu Y, Zhang Y, Gu Z, Hao L, Du J, Yang Q, et al. Cholecystokinin octapeptide antagonizes apoptosis in human retinal pigment epithelial cells. Neural Regen Res. (2014) 9:1402–8. doi: 10.4103/1673-5374.137596
155. Andreasen PA, Kjoller L, Christensen L, Duffy MJ. The urokinase-type plasminogen activator system in cancer metastasis: a review. Int J Cancer. (1997) 72:1–22. doi: 10.1002/(SICI)1097-0215(19970703)72:1<1::AID-IJC1>3.0.CO;2-Z
156. Kumar S, Baglioni C. Protection from tumor necrosis factor-mediated cytolysis by overexpression of plasminogen activator inhibitor type-2. J Biol Chem. (1991) 266:20960–4.
157. Varro A, Hemers E, Archer D, Pagliocca A, Haigh C, Ahmed S, et al. Identification of plasminogen activator inhibitor-2 as a gastrin-regulated gene: role of rho GTPase and menin. Gastroenterology. (2002) 123:271–80. doi: 10.1053/gast.2002.34162
158. Muerkoster S, Isberner A, Arlt A, Witt M, Reimann B, Blaszczuk E, et al. Gastrin suppresses growth of CCK2 receptor expressing colon cancer cells by inducing apoptosis in vitro and in vivo. Gastroenterology. (2005) 129:952–68. doi: 10.1053/j.gastro.2005.06.059
159. Kanno N, Glaser S, Chowdhury U, Phinizy JL, Baiocchi L, Francis H, et al. Gastrin inhibits cholangiocarcinoma growth through increased apoptosis by activation of Ca2+-dependent protein kinase C-alpha. J Hepatol. (2001) 34:284–91. doi: 10.1016/S0168-8278(00)00025-8
160. Cui G, Takaishi S, Ai W, Betz KS, Florholmen J, Koh TJ, et al. Gastrin-induced apoptosis contributes to carcinogenesis in the stomach. Lab Invest. (2006) 86:1037–51. doi: 10.1038/labinvest.3700462
161. Kidd M, Tang LH, Modlin IM, Zhang T, Chin K, Holt PR, et al. Gastrin-mediated alterations in gastric epithelial apoptosis and proliferation in a mastomys rodent model of gastric neoplasia. Digestion. (2000) 62:143–51. doi: 10.1159/000007806
162. Przemeck SM, Varro A, Berry D, Steele I, Wang TC, Dockray GJ, et al. Hypergastrinemia increases gastric epithelial susceptibility to apoptosis. Regul Pept. (2008) 146:147–56. doi: 10.1016/j.regpep.2007.09.002
163. Houghton J, Stoicov C, Nomura S, Rogers AB, Carlson J, Li H, et al. Gastric cancer originating from bone marrow-derived cells. Science. (2004) 306:1568–71. doi: 10.1126/science.1099513
164. Sugaya K, Takahashi M, Kubota K. Cholecystokinin protects cholinergic neurons against basal forebrain lesion. Jpn J Pharmacol. (1992) 59:125–8. doi: 10.1254/jjp.59.125
165. Akaike A, Tamura Y, Sato Y, Ozaki K, Matsuoka R, Miura S, et al. Cholecystokinin-induced protection of cultured cortical neurons against glutamate neurotoxicity. Brain Res. (1991) 557:303–7. doi: 10.1016/0006-8993(91)90149-P
166. Lavine JA, Raess PW, Stapleton DS, Rabaglia ME, Suhonen JI, Schueler KL, et al. Cholecystokinin is up-regulated in obese mouse islets and expands -cell mass by increasing -cell survival. Endocrinology. (2010) 151:3577–88. doi: 10.1210/en.2010-0233
167. Lavine JA, Kibbe CR, Baan M, Sirinvaravong S, Umhoefer HM, Engler KA, et al. Cholecystokinin expression in the -cell leads to increased -cell area in aged mice and protects from streptozotocin-induced diabetes and apoptosis. Am J Physiol Endocrinol Metab. (2015) 309:E819–28. doi: 10.1152/ajpendo.00159.2015
168. Reubi JC, Macke HR, Krenning EP. Candidates for peptide receptor radiotherapy today and in the future. J Nucl Med. (2005) 1(46 Suppl.):67S−75S.
169. Behr TM, Jenner N, Radetzky S, Behe M, Gratz S, Yucekent S, et al. Targeting of cholecystokinin-B/gastrin receptors in vivo: preclinical and initial clinical evaluation of the diagnostic and therapeutic potential of radiolabelled gastrin. Eur J Nucl Med. (1998) 25:424–30. doi: 10.1007/s002590050241
170. Gotthardt M, Behe MP, Beuter D, Battmann A, Bauhofer A, Schurrat T, et al. Improved tumour detection by gastrin receptor scintigraphy in patients with metastasised medullary thyroid carcinoma. Eur J Nucl Med Mol Imaging. (2006) 33:1273–9. doi: 10.1007/s00259-006-0157-8
171. Gotthardt M, Behe MP, Grass J, Bauhofer A, Rinke A, Schipper ML, et al. Added value of gastrin receptor scintigraphy in comparison to somatostatin receptor scintigraphy in patients with carcinoids and other neuroendocrine tumours. Endocr Relat Cancer. (2006) 13:1203–11. doi: 10.1677/erc.1.01245
172. Mather SJ, McKenzie AJ, Sosabowski JK, Morris TM, Ellison D, Watson SA. Selection of radiolabeled gastrin analogs for peptide receptor-targeted radionuclide therapy. J Nucl Med. (2007) 48:615–22. doi: 10.2967/jnumed.106.037085
173. Beauchamp RD, Townsend CM Jr, Singh P, Glass EJ, Thompson JC. Proglumide, a gastrin receptor antagonist, inhibits growth of colon cancer and enhances survival in mice. Ann Surg. (1985) 202:303–9. doi: 10.1097/00000658-198509000-00005
174. Harrison JD, Jones JA, Morris DL. The effect of the gastrin receptor antagonist proglumide on survival in gastric carcinoma. Cancer. (1990) 66:1449–52.
175. Watson SA, Michaeli D, Grimes S, Morris TM, Robinson G, Varro A, et al. Gastrimmune raises antibodies that neutralize amidated and glycine-extended gastrin-17 and inhibit the growth of colon cancer. Cancer Res. (1996) 56:880–5.
176. Watson SA, Morris TM, Varro A, Michaeli D, Smith AM. A comparison of the therapeutic effectiveness of gastrin neutralisation in two human gastric cancer models: relation to endocrine and autocrine/paracrine gastrin mediated growth. Gut. (1999) 45:812–7. doi: 10.1136/gut.45.6.812
177. Watson SA, Michaeli D, Grimes S, Morris TM, Varro A, Clarke PA, et al. A comparison of an anti-gastrin antibody and cytotoxic drugs in the therapy of human gastric ascites in SCID mice. Int J Cancer. (1999) 81:248–54. doi: 10.1002/(SICI)1097-0215(19990412)81:2<248::AID-IJC14>3.0.CO;2-G
178. Gilliam AD, Watson SA, Henwood M, McKenzie AJ, Humphreys JE, Elder J, et al. A phase II study of G17DT in gastric carcinoma. Eur J Surg Oncol. (2004) 30:536–43. doi: 10.1016/j.ejso.2004.03.009
179. Ajani JA, Hecht JR, Ho L, Baker J, Oortgiesen M, Eduljee A, et al. An open-label, multinational, multicenter study of G17DT vaccination combined with cisplatin and 5-fluorouracil in patients with untreated, advanced gastric or gastroesophageal cancer: the GC4 study. Cancer. (2006) 106:1908–16. doi: 10.1002/cncr.21814
Keywords: gastrin, CCK, G protein-coupled receptor, cancer, imaging, therapeutics
Citation: Zeng Q, Ou L, Wang W and Guo D-Y (2020) Gastrin, Cholecystokinin, Signaling, and Biological Activities in Cellular Processes. Front. Endocrinol. 11:112. doi: 10.3389/fendo.2020.00112
Received: 05 January 2020; Accepted: 20 February 2020;
Published: 06 March 2020.
Edited by:
Dora Reglodi, University of Pécs, HungaryReviewed by:
Grazia Maugeri, University of Catania, ItalyNils Lambrecht, VA Long Beach Healthcare System, United States
Copyright © 2020 Zeng, Ou, Wang and Guo. This is an open-access article distributed under the terms of the Creative Commons Attribution License (CC BY). The use, distribution or reproduction in other forums is permitted, provided the original author(s) and the copyright owner(s) are credited and that the original publication in this journal is cited, in accordance with accepted academic practice. No use, distribution or reproduction is permitted which does not comply with these terms.
*Correspondence: Wei Wang, wzw0019@auburn.edu; Dong-Yu Guo, xiamenhaijin@163.com
†These authors have contributed equally to this work