- 1Department of Biological Sciences, Graduate School of Science and Technology, Kumamoto University, Kumamoto, Japan
- 2Department of Biochemistry, Asahikawa Medical University, Asahikawa, Japan
- 3Spectrography and Bioimaging Facility, National Institute for Basic Biology Core Research Facilities, National Institute for Basic Biology, Okazaki, Japan
Medaka (Oryzias latipes) are teleost fish with a XX/XY sex determination system. Recently, it was reported that high temperature (HT) induced the masculinization of XX medaka by increasing the levels of cortisol, a major glucocorticoid produced by interrenal cells in teleosts. Cortisol secretion is regulated by adrenocorticotropic hormone (ACTH) secreted from the pituitary gland, which is partly regulated by corticotropin-releasing hormone (CRH) secreted from the hypothalamus. In teleosts, two crh paralogs, named crha and crhb, have been identified. Recently, the expression of crhb but not crha was upregulated by HT during gonadal sex differentiation period in medaka and loss-of-functions of its receptors under HT suppressed masculinization of XX medaka and increase of cortisol levels, suggesting that crhb is involved in masculinization induced by HT. However, the transcriptional regulation of crhb under HT has not been elucidated. We analyzed the gene expression pattern in the hypothalamus of medaka embryos incubated under HT using DNA microarray. The expressions of heat shock protein (hsp) genes, such as hsp70.1 and hsp30, were increased. Overexpression of hsp70.1 or hsp30 in cultured rat hypothalamic 4B cells significantly induced crh gene expression. Moreover, hypothalamic hsp70.1-overexpressing transgenic medaka also showed increased crhb gene expression that increased cortisol levels compared with fish incubated at a normal temperature. These results provide the first evidence that HSPs induce cortisol levels by elevating crhb gene expression in the hypothalamus.
Introduction
In vertebrates, sex is determined primarily by a combination of sex chromosomes at fertilization. However, the mechanism of sex determination in vertebrates is diverse, and environmental stresses such as temperature and individual interaction affect sex determination in vertebrates other than mammals (1). The elucidation of sex determination and sexual differentiation mechanisms is expected to be the basis of applied research for the preservation of biological species and reproductive medicine.
Medaka (Oryzias latipes) are small fish with several desirable features for use as a model organism, including a short generation time, small genome size, and the availability of numerous useful strains (2). Transgenic (Tg) techniques and gene knockout system using the clustered regularly interspaced short palindromic repeats (CRISPR)/CRISPR-associated protein 9 (Cas9), have already been established in this fish (3–5). Furthermore, the medaka sex-determining gene dmy/dmrt1bY, which is found on the Y chromosome, has been identified (6–8). Thus, medaka provides an excellent vertebrate model for investigating various biological phenomena including embryonic development and sex differentiation. Recently, it was reported in medaka that exposure to water of a high temperature (HT) during the sex differentiation period induced female-to-male sex reversal (9, 10). Additionally, HT caused the masculinization of XX medaka by elevating the levels of cortisol (11, 12), the major glucocorticoid produced by the interrenal cells in teleosts (13). However, it remains unclear how HT-elevated cortisol induces the masculinization of XX fish.
Cortisol is a glucocorticoid produced in the adrenal cortex that regulates the expression of various stress response genes via a glucocorticoid receptor (13). In addition, cortisol secretion is regulated by adrenocorticotropic hormone (ACTH) secreted from the pituitary gland, and which is partly regulated by corticotropin-releasing hormone (CRH) secreted from the hypothalamus (14). In teleosts, two crh paralogs, named crha and crhb, have been identified (15). The expressions of crha and crhb have been mainly observed in the retina and brain, respectively (15, 16). Recently, the expression of crhb but not crha was upregulated by HT during gonadal sex differentiation period in medaka and loss-of-functions of its receptors under HT suppressed masculinization of XX medaka and increase of cortisol levels, suggesting that crhb is involved in masculinization induced by HT (17). However, the transcriptional regulation of crhb under HT has not been elucidated.
Here, we performed DNA microarray analysis using the hypothalamic regions of medaka embryos reared under HT to identify novel factors regulating crh expression. We confirmed an increase in the gene expressions of heat shock proteins (hsps) such as hsp70 and hsp30. HSPs function as molecular chaperones under physical (18) and environmental stress to maintain homeostasis of the higher order structures of various proteins (19). We performed a functional analysis of HSPs on the transcriptional regulation of crh using cultured rat hypothalamus cells and medaka brains.
Materials and Methods
Animals
The FLFII medaka stock was used (20), which allows the identification of genotypic sex by the appearance of leucophores at 2 days post-fertilization (dpf), before the onset of sex differentiation. The neurogenin3 (ngn3)-green fluorescent protein (GFP) Tg medaka line was generated by injecting the pEGFP-1 vector (Clontech, Palo Alto, CA) fused to the regulatory region of medaka ngn3, which is expressed strongly in the hypothalamus (21), into one-cell stage embryos of FLFII stock as previously described (22). All injected embryos were bred to adults and only F1 embryos possessing GFP fluorescence were selected and used to produce succeeding generations.
To generate hsp70.1-overexpressing Tg medaka that overexpressed HSP70.1 in the hypothalamus, both ngn3-hsp70.1, where full-length medaka hsp70.1 cDNA (23) was exchanged with EGFP cDNA in an ngn3-GFP plasmid, and the olvas-DsRedExpress plasmid, which is used to visualize germ cells by DsRed fluorescence (11), were injected into one-cell stage embryos of FLFII stock (Supplementary Figure 1). All embryos were bred to adults and mated with each other to obtain heterozygous Tg medaka (F1). Only embryos possessing DsRed fluorescence were selected and homozygous Tg medaka produced by mating F1 fish with each other were used to perform the experiments.
Medaka embryos and larvae were maintained in ERM (17 mM NaCl, 0.4 mM KCl, 0.27 mM CaCl2.2H2O, 0.66 mM MgSO4, pH 7) at 26°C (normal temperature) or 33°C (HT) under a 14-h light and 10-h dark cycle. Fish images were captured using a BZ-9000 BioRevo fluorescence microscope (Keyence Co., Osaka, Japan). Survival rates were investigated by counting individual numbers with a heartbeat. Developmental stages of the embryos were determined as described previously (24).
Immunohistochemistry
The ngn3-GFP Tg embryos at 4 dpf (stage 33) and adults at about 6 months of age were fixed in Bouin's solution at 4°C overnight, dehydrated in graded ethanol, embedded in paraffin, and sectioned serially at 5 μm as previously described (25). After deparaffinization and rehydration, sections were incubated at 4°C overnight with primary antibodies (1:500 dilution), mouse monoclonal anti-GFP (Roche Diagnostics, Indianapolis, IN). After washing, the sections were treated for 1 h with secondary antibodies (1:500 dilution), anti-mouse IgG conjugated with biotin (Molecular Probes, Eugene, OR). Then, they were developed using a Histofine SAB-PO kit (Nichirei Co., Tokyo, Japan) and counterstained with hematoxylin.
DNA Microarray Analysis
Total RNA was extracted from the GFP-positive hypothalamic regions cut off from ngn3-GFP Tg embryos (50 pooled fishes) incubated at normal temperature (control) or HT at 4-dpf, before the onset of the elevation in cortisol levels (11), using RNeasy Mini Kit (Qiagen, Hilden, Germany). The DNA microarray analysis was conducted as shown in our previous paper (26). Briefly, DNA microarray hybridization and analyses following the protocol of the manufacturer for one color, microarray-based gene expression analysis (version 5.5) were performed with a medaka microarray tip (44 K; Agilent Technologies, Santa Clara, CA) in the Chemical Evaluation and Research Institute (Tokyo, Japan). Based on Agilent Feature Extraction 12.0, the median of the selected raw data was calculated for each array, and normalization to the median was then applied to the raw intensities across the arrays. Differentially expressed genes from the HT group were selected by t-tests with P-values <0.05 and based on a change that was 2-fold greater than that of the control group. The up-regulated or down-regulated genes were annotated by biological process and cellular component based on Gene Ontology (GO) using the GO consortium (http://www.geneontology.org/) and UniProtKB/Swiss-Prot (http://www.uniprot.org/).
Cell Culture
Rat hypothalamic 4B cells were cultured in Dulbecco's modified eagle's medium (DMEM; Invitrogen Co., Carlsbad, CA) supplemented with 10% charcoal/dextran-treated fetal bovine serum (CDFBS; HyClone, Logan, UT), 100 mg/ml streptomycin, and 100 U/ml penicillin at 37°C in 5% CO2 and passaged based as previously described (27). The cells were cultured in the medium for 2 h at 37 or 45°C with or without 1 × 10−5 M HSP inhibitor (KNK 437, Calbiochem). KNK437 inhibited heat shock factor activity and heat-induced hsp expression in human and Xenopus cultured cells (28, 29). After treatment, the cells were collected, and total RNA was extracted for the analysis of gene expression.
Constructs and Transfection
The hsp70.1- and hsp30-expression plasmids were constructed by cloning the full-length medaka hsp70.1 and hsp30 cDNAs into pcDNA3.1 (Invitrogen). 4B cells were plated in 24-well plates 24 h before transfection with 100 ng of the hsp70.1- or hsp30-expression plasmids, or an empty plasmid (control) using Lipofectamine reagent (Invitrogen) as previously described (25). After 24 h transfection, the cells were collected, and total RNA was extracted for the analysis of gene expression.
Organ Culture
Brains dissected from hsp70.1-overexpressing Tg and wild-type XX medaka embryos (4 dpf) incubated at 26°C were used for organ culture. The brains were cultured in Leibovitz's L-15 medium (Invitrogen) for 16 h at 26 or 33°C with or without 1 × 10−5 M HSP70 functional inhibitor (PES: 2-phenylethynesulfonamide, Calbiochem). PES is a functional inhibitor that selectively acts on HSP70 to inhibit the association between HSP70 and its co-chaperone (30). After culturing, the brains were used for the analysis of gene expression.
Quantitative Reverse Transcription-Polymerase Chain Reaction (qRT-PCR)
Total RNA was extracted from medaka tissues and cultured rat cells using ISOGEN (Nippon Gene, Tokyo, Japan). Then, 0.2 μg of the extracted RNA was reverse-transcribed using an RNA PCR kit (Applied Biosystems) at 42°C for 30 min. Quantitative real-time PCR was performed in a LightCycler 480 (Roche Diagnostics GmbH, Mannheim, Germany) using SYBR Green I Master (Roche) as previously described (12). Primers used are shown in Supplementary Table 1. The conditions were as follows: preheating PCR was carried out at 95°C for 5 min, 95°C for 10 s, 59°C for 10 s, and 72°C for 10 s for 45 cycles. Evaluation of the copy number of the target genes was calculated based on ef1α in medaka cells or b2mg in rat cells. All experiments were performed in triplicates.
Measurement of Cortisol
Steroid hormones in XX medaka larvae (5 pooled fishes) at 0 days post-hatching (dph) (stage 39) were extracted with diethyl ether as previously described (11, 31). Cortisol levels were measured using a Cortisol EIA kit (Cayman Chemical, Ann Arbor, MI) in accordance with the manufacturer's instructions.
Statistical Analysis
Experimental results were tested using Levene's test for homogeneity of variance. Data were analyzed by the Student's t-test or one-way ANOVA followed by Tukey's multiple comparison test using SPSS statistics 20 (IBM Corp., Armonk, NY).
Results
Generation of a Tg Medaka Line to Visualize the Hypothalamus by GFP Fluorescence
To visualize the hypothalamus in medaka embryos, we generated a ngn3-GFP Tg medaka line by injecting a pEGFP-1 vector fused to the regulatory region of medaka ngn3, which is expressed strongly in the hypothalamus (21), into the fertilized eggs of FLFII stock (Figures 1A,B). Immunohistochemical staining using anti-GFP antibody showed positive signals were detected mainly in the eyes and hypothalamus of embryos incubated at 26°C (control; Figure 1C) and 33°C (HT; Figure 1D). Moreover, immunohistochemical staining of the adult brain showed signals were detected strongly in the hypothalamus containing the nucleus ventral tuberis (Figures 1E–I), where medaka crha and crhb mRNAs are co-expressed (16), the area pretectalis and the ventral part of the optic tectum. Thus, this Tg line allows visualization of the hypothalamus by GFP fluorescence in living individuals.
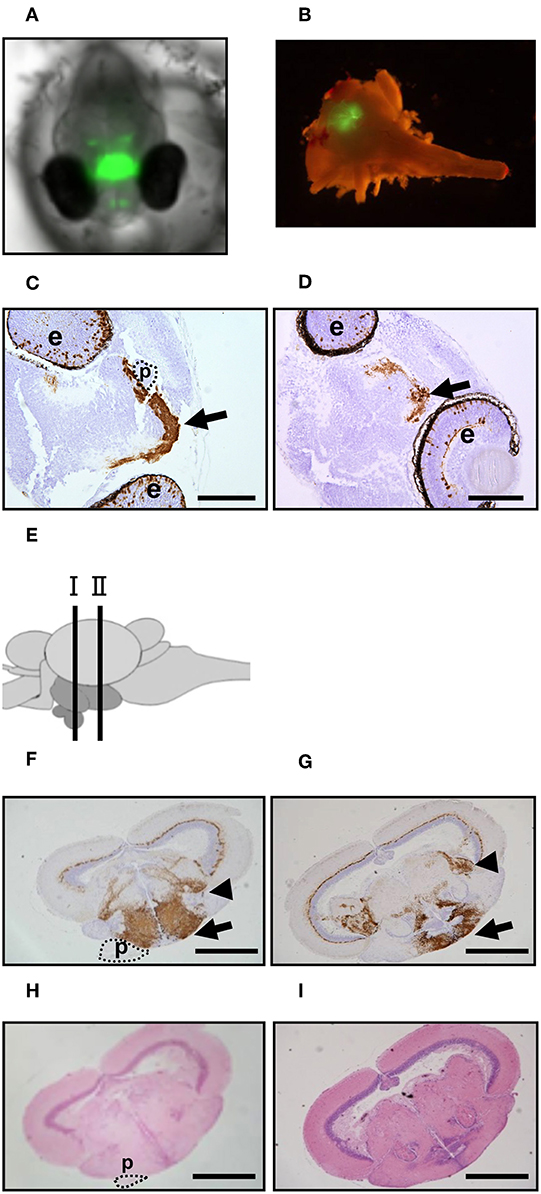
Figure 1. GFP expression in ngn3-GFP Tg medaka. (A,B) GFP fluorescence in the Tg XX medaka at 4 dpf (A) and adult brain (B). (C,D) Immunohistochemistry to detect GFP was performed using head tissues of the embryos treated at normal temperature (C) or high temperature (D). Arrow: hypothalamus. Scale bar: 100 μm. e, eye; p, pituitary. (E) Schematic illustration of the section sites in adult medaka brains. Site I contain the pituitary and site II does not contain the pituitary. (F–I) Immunohistochemistry to detect GFP (F,G) and HE staining (H,I) were performed using brain tissues of the adults reared at normal temperature. (F,H) Site I and (G,I) Site II. Arrow: hypothalamus. Arrowhead: area pretectalis. Scale bar: 500 μm. p, pituitary.
Analysis of Gene Expression Changes in Medaka Embryos by HT
To identify novel factors regulating CRH expression in the hypothalamus, we performed DNA microarray analysis using the hypothalamic regions (containing the pituitary) of medaka embryos reared at 26 and 33°C. HT treatment induced the expressions of 2,512 genes more than 2-fold in 44,000 genes, of which 65 genes had expressions increased more than 10-fold (Supplementary Figure 2). These 65 genes included hsp70.1, hsp30, cholecystokinin (cck), and somatostatin 3 (ppss3). HT treatment reduced the expression of 1,981 genes <1/2, of which 58 genes were reduced <1/10 (Supplementary Figure 2). These 58 genes included keratin, and choriolysin H (hce). Furthermore, qRT-PCR analysis of the hypothalamus regions of medaka embryos at 4 dpf confirmed that crhb, proopiomelanocortin (pomc), hsp70.1, hsp30, cck, and ppss3 expressions were significantly induced by HT (Figures 2A–F), while keratin and hce expressions were significantly reduced by HT (Figures 2G,H), similar to the DNA microarray analysis.
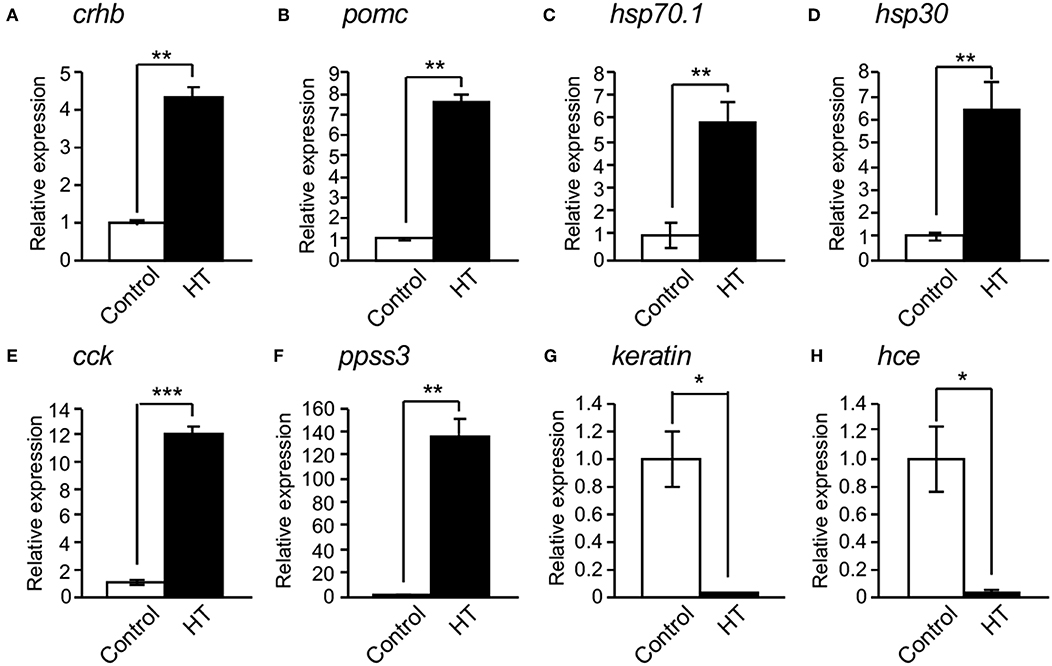
Figure 2. Confirmation of mRNA expression changed by high temperature. (A–F) qRT-PCR showed that high temperature (HT) increased the expressions of crhb (A), pomc (B), hsp70.1 (C), hsp30 (D), cck (E), and ppss3 mRNAs (F) in the hypothalamic region of XX embryos at 4 dpf. (G,H) qRT-PCR showed that HT decreased the expressions of keratin (G) and hce mRNAs (H) in the hypothalamic region of XX embryos at 4 dpf. Vertical bar: mean ± standard error of triplicates, *p < 0.05, **p < 0.01, ***p < 0.001.
Effects of HSPs on crh Expression in Cultured Hypothalamic Cells
We investigated the effect of HSPs on crh expression in rat hypothalamus-derived 4B cells. hsp70 and crh expressions were markedly increased by heat shock (45°C) of the cells, and their expressions were significantly inhibited by treatment with a HSP inhibitor (KNK 437) under heat shock conditions (Figures 3A,B). crh expression was significantly induced by the overexpression of hsp70.1 or hsp30 in 4B cells, and was further induced by their co-overexpression (Figure 3C).
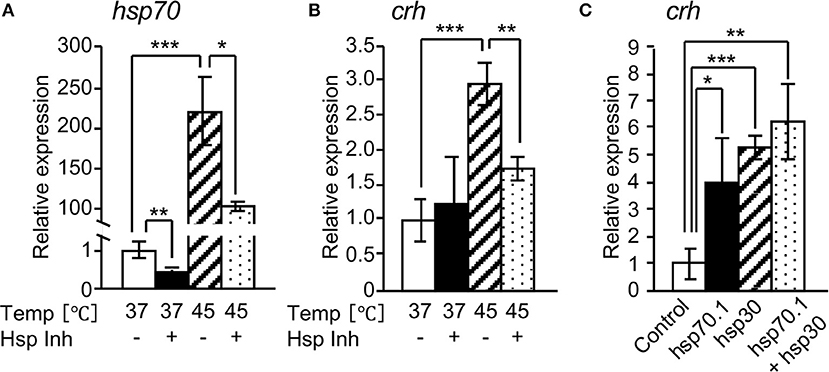
Figure 3. Effects of HSPs on crh expression in 4B cells. qRT-PCR shows that HSP inhibitor (Hsp Inh) significantly attenuated the expressions of hsp70 (A) and crh mRNAs (B). (C) qRT-PCR showed that the forced expression of hsp70.1 and/or hsp30 by transfection of an expression vector significantly induced the expression of crh mRNA. Vertical bar: mean ± standard error of triplicates, *p < 0.05, **p < 0.01, ***p < 0.001.
Analysis of Hypothalamic Hsp70.1-Overexpressing Tg Medaka
We investigated the effects of HSP70.1 on the expressions of cortisol-regulated genes in hypothalamic hsp70.1-overexpressing Tg medaka. qRT-PCR showed that the expression of hsp70.1 mRNA was significantly increased in the hypothalamic regions of both sexes of Tg larvae at 4 dpf and 0 dph compared with controls (Figures 4A,D), while all expressions of crhb and pomc mRNAs were not increased in the hypothalamic regions of the larvae at 4 dpf and 0 dph compared with controls (Figures 4B,C,E,F). Whole-body levels of cortisol in both sexes of Tg larvae at 0 dph were significantly elevated compared with controls, similar to those in HT-treated larvae (Figure 4G). Survival rates in Tg medaka incubated at 26°C were 100% over 5 days, similar to those in controls, whereas they were higher in Tg fish incubated at 33°C than in controls incubated at 33°C (Supplementary Table 2).
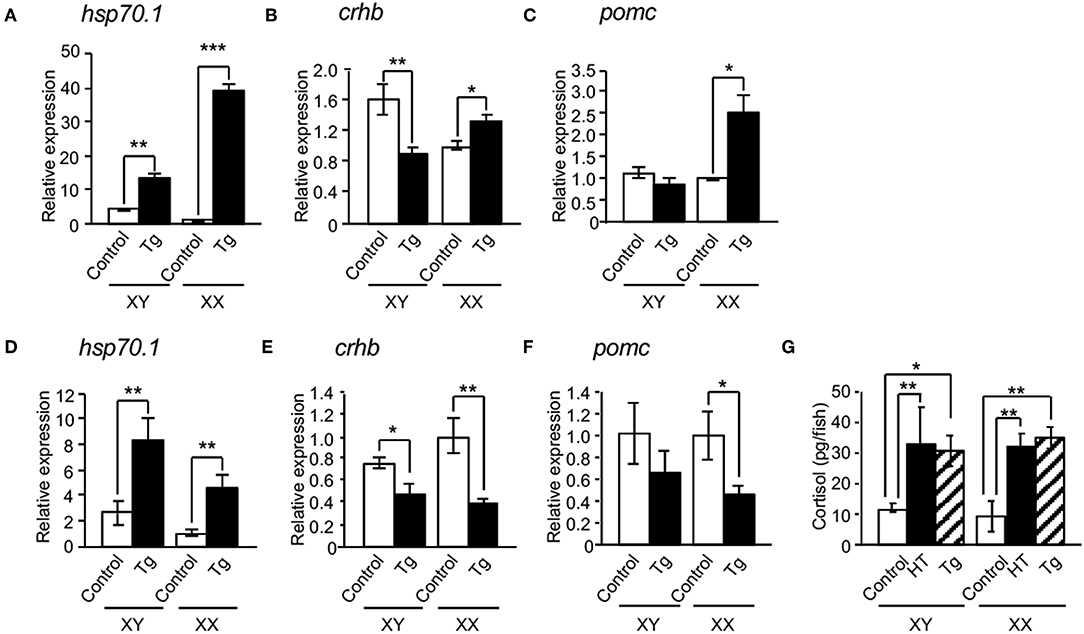
Figure 4. Effects of HSP70 on the expressions of cortisol-regulated genes in hsp70.1-overexpressing Tg medaka. In the hypothalamic regions of XX medaka at 4 dpf (A–C) and 0 dph (D–F), the expressions of hsp70.1 (A,D), crhb (B,E), and pomc mRNAs (C,F) were investigated by qRT-PCR. (G) Whole-body levels of cortisol in the larvae at 0 dph. HT: high temperature treatment, Tg: hsp70.1-overexpressing Tg fish. Vertical bar: mean ± standard error of triplicates, *p < 0.05, **p < 0.01, ***p < 0.001.
To bypass the effects of the negative feedback system through the hypothalamic-pituitary-adrenal (HPA) axis in hsp70.1-overexpressing Tg medaka, we performed organ culture experiments using brains dissected from embryos at 4 dpf. qRT-PCR showed that the expressions of hsp70.1, crhb and pomc mRNAs were significantly increased in the brains of Tg embryos compared with controls (Figures 5A,C,D), while the expressions of crhb and pomc mRNAs were significantly inhibited by treatment with an HSP70 functional inhibitor (PES), similar to HT-treated embryos (Figures 5C,D). PES treatment increased the expressions of hsp70.1 and hsp30 mRNAs in the brains of Tg embryos similar to HT-treated embryos (Figures 5A,B).
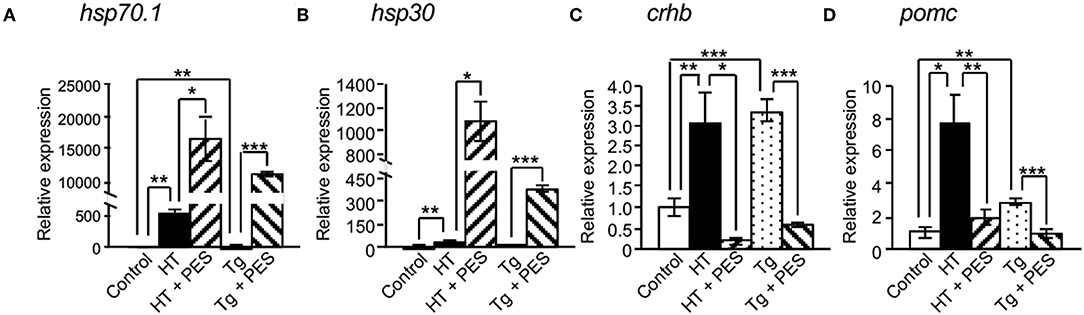
Figure 5. Effects of HSP70 on the expressions of cortisol-regulated genes in medaka brains treated with or without an HSP70 functional inhibitor (PES). The expressions of hsp70.1 (A), hsp30 (B), crhb (C), and pomc mRNAs (D) in brains of XX embryos at 4 dpf were investigated by qRT-PCR. HT: high temperature treatment, Tg: hsp70.1-overexpressing Tg fish. Vertical bar: mean ± standard error of triplicates, *p < 0.05, **p < 0.01, ***p < 0.001.
Discussion
In this study, we identified novel factors regulating CRH expression in the hypothalamus by DNA microarray analysis using the hypothalamic regions of ngn3-GFP Tg medaka embryos reared at normal temperature and HT. Sixty-five genes (including hsp70.1 and hsp30) had expression levels increased more than 10-fold in medaka reared at HT compared with those reared at normal temperature. HSPs function as molecular chaperones under physical (18) and environmental stress to maintain homeostasis of the higher order structures of various proteins (19). Recently, it was reported that some HSPs in medaka were regulated by heat shock factor 1, which is activated by heat stress (32), suggesting HSPs may have common regulating mechanisms in vertebrates. Therefore, we focused on the effects of HSPs on crh expression.
We investigated the effect of HSPs on crh expression in rat hypothalamus-derived 4B cells. hsp70.1 and crh expressions were markedly elevated by heat shock, and significantly inhibited by treatment with an HSP inhibitor under heat shock. This suggested that change in the level of HSPs modulate the crh expression in hypothalamic cells. Moreover, crh expression was significantly induced by the overexpression of hsp70.1 or hsp30 in 4B cells, and further increased by their co-overexpression. Therefore, hsp70.1 and hsp30 are likely to have capacity for inducing crh expression in hypothalamic cells.
Next, we investigated the effect of HSP70.1 on the expression of cortisol-regulated genes in medaka hypothalamic cells using hypothalamic hsp70.1-overexpressing Tg medaka. qRT-PCR showed that the expression of hsp70.1 mRNA was significantly increased in hypothalamic regions of Tg larvae compared with controls, while the expressions of crhb and pomc mRNAs were decreased in hypothalamic regions of the larvae. This indicated the existence of a negative feedback system mediated through the HPA axis (33). To bypass the effects of this system through the HPA axis in Tg medaka, we performed organ culture experiments using embryo brains. qRT-PCR showed that the expressions of hsp70.1, crhb, and pomc mRNAs were significantly increased in the brains of Tg embryos compared with controls, while the expressions of crhb and pomc mRNAs were significantly inhibited by treatment with an HSP70 functional inhibitor, similar to those in HT-treated embryos. These results strongly suggest that HSP70.1 induces crhb expression in the medaka hypothalamus. The human CRH gene contains a cyclic AMP-responsive element (CRE) and several partial 12-O-tetradecanoyl phorbol 13-acetate (TPA) response elements (TREs) (34). Numerous studies have suggested that protein kinase A (PKA) and protein kinase C (PKC) stimulators increase the expression of CRH. Moreover, HSP70 binds to the carboxyl terminus of at least three AGC kinase family members: PKC isozymes, Akt, and PKA (35). This interaction is mediated primarily by the peptide-binding domain on HSP70 and the dephosphorylated turn motif on protein kinases (35), suggesting that HSP70 activates these kinases. Although it remains unclear whether CRE and TREs are present in the medaka crhb gene promoter, HSP70 may regulate crhb expression through the activation of these kinases in vertebrates.
Previously, restraint stress which activates the HPA axis, has induced hsp70 expression by elevating ACTH levels in rat adrenal cortex (36). Long-term dexamethasone treatment has reduced adrenal restraint-induced hsp70 expression (37), suggesting a functional interrelationship between HPA activity and hsp70 expression in the adrenals. Moreover, it was reported that ubiquitously HSP70-overexpressing Tg mice suppressed apoptosis and had increased levels of serum corticosterone (38). In this study, whole-body levels of cortisol in the hypothalamic hsp70.1-overexpressing Tg larvae at 0 dph were significantly elevated compared with controls, similar to those in HT-treated larvae. Therefore, HSP70 appears to activate the HPA axis via crh expression in common among vertebrates.
We previously showed that HT inhibited the female-type proliferation of germ cells and induced the masculinization of XX medaka by elevating the levels of cortisol (11). Preliminary results showed that although this hsp70.1-overexpressing Tg adults did not undergo female-to-male sex reversal, germ cell proliferation in Tg XX larvae at 0 dph was partially inhibited compared with wild-type XX fish (data not shown), suggesting that overexpression of HSP70.1 in the hypothalamus may inhibit the female-type proliferation of germ cells by increasing the levels of cortisol. Taken together, these results strongly suggest that hypothalamic HSP70.1 induced by heat stress inhibits the female-type proliferation of germ cells to induce masculinization of XX medaka by increasing the levels of cortisol. Future studies should focus on the phenotypic analysis of this Tg medaka.
In summary, DNA microarray analysis showed that the expressions of hsp genes, such as hsp70.1 and hsp30, were increased in the hypothalamus of medaka embryos incubated under HT compared with those at normal temperature. The overexpression of hsp70.1 or hsp30 in cultured rat hypothalamic 4B cells significantly induced crh gene expression. Moreover, hsp70.1-overexpressing Tg medaka had elevated crhb gene expression and increased cortisol levels compared with medaka incubated at normal temperature. These results provide the first evidence that HSPs induce cortisol levels by elevating crh gene expression in the hypothalamus.
Data Availability
The datasets generated for this study can be found in the Supplementary Table 1.
Ethics Statement
The animal study was reviewed and approved by Kumamoto University. Written informed consent was obtained from the owners for the participation of their animals in this study.
Author Contributions
TK and YK obtained funding and designed the study. TU, SH, TY, YK, and TK performed the experiments and collected the data. TU and TK wrote the manuscript.
Funding
This work was supported in part by the individual Collaborative Research Program 16-331 (to TK) from the National Institute for Basic Biology, by JSPS KAKENHI Grant Number 16H04983 (to TK), 16H02984 (to TK), and 17H06258 (to YK) from the Ministry of Education, Culture, Sports, Science and Technology of Japan, and by JST CREST Grant Number JPMJCR1754 (to YK).
Conflict of Interest Statement
The authors declare that the research was conducted in the absence of any commercial or financial relationships that could be construed as a potential conflict of interest.
Acknowledgments
We thank Drs. John Kasckow (Univ. of Cincinnati School of Medicine) and Kazunori Kageyama (Hirosaki Univ. Graduate School of Medicine) for the gift of 4B cells. We thank Edanz Group (www.edanzediting.com/ac) for editing a draft of this manuscript.
Supplementary Material
The Supplementary Material for this article can be found online at: https://www.frontiersin.org/articles/10.3389/fendo.2019.00529/full#supplementary-material
Abbreviations
HT, high temperature; ACTH, adrenocorticotropic hormone; CRH, corticotropin-releasing hormone; HPA, hypothalamic-pituitary-adrenal; HSP, heat shock protein; qRT-PCR, quantitative reverse transcription-polymerase chain reaction; GFP, green fluorescent protein.
References
1. Baroiller JF, Guiguen Y. Endocrine and environmental aspects of sex differentiation in gonochoristic fish. EXS. (2001) 91:177–201. doi: 10.1007/978-3-0348-7781-7_9
2. Ishikawa Y. Medakafish as a model system for vertebrate developmental genetics. BioEssays. (2000) 22:487–95. doi: 10.1002/(SICI)1521-1878(200005)22:5<487::AID-BIES11>3.0.CO;2-8
3. Ozato K, Kondoh H, Inohara H, Iwamatsu T, Wakamatsu Y, Okada TS. Production of transgenic fish: introduction and expression of chicken delta-crystallin gene in medaka embryos. Cell Differ. (1986) 19:237–44. doi: 10.1016/0045-6039(86)90100-4
4. Ansai S, Kinoshita M. Targeted mutagenesis using CRISPR/Cas system in medaka. Biol Open. (2014) 3:362–71. doi: 10.1242/bio.20148177
5. Sawamura R, Osafune N, Murakami T, Furukawa F, Kitano T. Generation of biallelic F0 mutants in medaka using the CRISPR/Cas9 system. Gene Cell. (2017) 22:756–63. doi: 10.1111/gtc.12511
6. Matsuda M, Nagahama Y, Shinomiya A, Sato T, Matsuda C, Kobayashi T, et al. DMY is a Y-specific DM-domain gene required for male development in the medaka fish. Nature. (2002) 417:559–563. doi: 10.1038/nature751
7. Matsuda M, Shinomiya A, Kinoshita M, Suzuki A, Kobayashi T, Paul-Prasanth B, et al. DMY gene induces male development in genetically female (XX) medaka fish. Proc Natl Acad Sci USA. (2007) 104:3865–70. doi: 10.1073/pnas.0611707104
8. Nanda I, Kondo M, Hornung U, Asakawa S, Winkler C, Shimizu A, et al. A duplicated copy of DMRT1 in the sex-determining region of the Y chromosome of the medaka, Oryzias latipes. Proc Natl Acad Sci USA. (2002) 99:11778–83. doi: 10.1073/pnas.182314699
9. Sato T, Endo T, Yamahira K, Hamaguchi S, Sakaizumi M. Induction of female-to-male sex reversal by high temperature treatment in Medaka, Oryzias latipes. Zool Sci. (2005) 22:985–8. doi: 10.2108/zsj.22.985
10. Hattori RS, Gould RJ, Fujioka T, Saito T, Kurita J, Strussmann CA, et al. Temperature-dependent sex determination in Hd-rR medaka Oryzias latipes: gender sensitivity, thermal threshold, critical period, and DMRT1 expression profile. Sex Dev. (2007) 1:138–46. doi: 10.1159/000100035
11. Hayashi Y, Kobira H, Yamaguchi T, Shiraishi E, Yazawa T, Hirai T, et al. High temperature causes masculinization of genetically female medaka by elevation of cortisol. Mol Reprod Dev. (2010) 77:679–86. doi: 10.1002/mrd.21203
12. Kitano T, Hayashi Y, Shiraishi E, Kamei Y. Estrogen rescues masculinization of genetically female medaka by exposure to cortisol or high temperature. Mol Reprod Dev. (2012) 79:719–26. doi: 10.1002/mrd.22080
13. Wendelaar Bonga SE. The stress response in fish. Physiol Rev. (1997) 77:591–625. doi: 10.1152/physrev.1997.77.3.591
14. Papadimitriou A, Priftis KN. Regulation of the hypothalamic-pituitary-adrenal axis. Neuroimmunomodulation. (2009) 16:265–71. doi: 10.1159/000216184
15. Grone BP, Maruska KP. Divergent evolution of two corticotropin-releasing hormone (CRH) genes in teleost fishes. Front Neurosci. (2015) 9:365. doi: 10.3389/fnins.2015.00365
16. Hosono K, Kikuchi Y, Miyanishi H, Hiraki-Kajiyama T, Takeuchi A, Nakasone K, et al. Teleocortin: a novel member of the CRH family in teleost fish. Endocrinology. (2015) 156:2949–57. doi: 10.1210/en.2015-1042
17. Castañeda Cortés DC, Arias Padilla LF, Langlois VS, Somoza GM, Fernandino JI. The central nervous system acts as a transducer of stress-induced masculinization through corticotropin-releasing hormone B. Development. (2019) 146:dev172866. doi: 10.1242/dev.172866
18. Pignatelli D, Ferreira J, Soares P, Costa MJ, Magalhães MC. Immunohistochemical study of heat shock proteins 27, 60, and 70 in the normal human adrenal and in adrenal tumors with suppressed ACTH production. Microsc Res Tech. (2003) 61:315–23. doi: 10.1002/jemt.10341
19. Hunt C, Morimoto RI. Conserved features of eukaryotic hsp70 genes revealed by comparison with the nucleotide sequence of human hsp70. Proc Natl Acad Sci USA. (1985) 82:6455–9. doi: 10.1073/pnas.82.19.6455
20. Wakamatsu Y, Inoue C, Hayashi H, Mishima N, Sakaizumi M, Ozato K. Establishment of new medaka (Oryzias latipes) stocks carrying genotypic sex markers. Environ Sci. (2003) 10:291–302.
21. Wang X, Chu LT, He J, Emelyanov A, Korzh V, Gong Z. A novel zebrafish bHLH gene, neurogenin3, is expressed in the hypothalamus. Gene. (2001) 275:47–55. doi: 10.1016/S0378-1119(01)00648-5
22. Murozumi N, Nakashima R, Hirai T, Kamei Y, Ishikawa-Fujiwara T, Todo T, et al. Loss of follicle-stimulating hormone receptor function causes masculinization and suppression of ovarian development in genetically female medaka. Endocrinology. (2014) 155:3136–45. doi: 10.1210/en.2013-2060
23. Oda S, Mikami S, Urushihara Y, Murata Y, Kamei Y, Deguchi T, et al. Identification of a functional Medaka heat shock promoter and characterization of its ability to induce in vitro and in vivo. Zool Sci. (2010) 27: 410–5. doi: 10.2108/zsj.27.410
24. Iwamatsu T. Stages of normal development in the medaka Oryzias latipes. Mech Dev. (2004) 121:605–18. doi: 10.1016/j.mod.2004.03.012
25. Yamaguchi T, Yoshinaga N, Yazawa T, Gen K, Kitano T. Cortisol is involved in temperature-dependent sex determination in the Japanese flounder. Endocrinology. (2010) 151:3900–8. doi: 10.1210/en.2010-0228
26. Shiraishi E, Hosseini H, Kang DK, Kitano T, Akiyama H. Nanosecond pulsed electric field suppresses development of eyes and germ cells through blocking synthesis of retinoic acid in Medaka (Oryzias latipes). PLoS ONE (2013) 8:e70670. doi: 10.1371/journal.pone.0070670
27. Kageyama K, Hanada K, Iwasaki Y, Sakihara S, Nigawara T, Kasckow J, et al. Pituitary adenylate cyclase-activating polypeptide stimulates corticotropin-releasing factor, vasopressin and interleukin-6 gene transcription in hypothalamic 4B cells. J Endocrinol. (2007)195:199–211. doi: 10.1677/JOE-07-0125
28. Yokota S, Kitahara M, Nagata K. Benzylidene lactam compound, KNK437, a novel inhibitor of acquisition of thermotolerance and heat shock protein induction in human colon carcinoma cells. Cancer Res. (2000) 60:2942–8.
29. Voyer J, Heikkila JJ. Comparison of the effect of heat shock factor inhibitor, KNK437, on heat shock- and chemical stress-induced hsp30 gene expression in Xenopus laevis A6 cells. Comp Biochem Physiol A Mol Integr Physiol. (2008) 151:253–61 doi: 10.1016/j.cbpa.2008.07.004
30. Leu JI, Pimkina J, Frank A, Murphy ME, George DL. A small molecule inhibitor of inducible heat shock protein 70. Mol Cell. (2009) 36:15–27. doi: 10.1016/j.molcel.2009.09.023
31. Kitano T, Takamune K, Kobayashi T, Nagahama Y, Abe SI. Suppression of P450 aromatase gene expression in sex-reversed males produced by rearing genetically female larvae at a high water temperature during a period of sex differentiation in the Japanese flounder (Paralichthys olivaceus). J Mol Endocrinol. (1999) 23:167–76. doi: 10.1677/jme.0.0230167
32. Furukawa F, Hamasaki S, Hara S, Uchimura T, Shiraishi E, Osafune N, et al. Heat shock factor 1 protects germ cell proliferation during early ovarian differentiation in medaka. Sci Rep. (2019) 9:6927. doi: 10.1038/s41598-019-43472-4
33. King BR, Smith R, Nicholson RC. Novel glucocorticoid and cAMP interactions on the CRH gene promoter. Mol Cell Endocrinol. (2002) 194:19–28. doi: 10.1016/S0303-7207(02)00218-6
34. Vamvakopoulos NC, Karl M, Mayol V, Gomez T, Stratakis CA, Margioris A, et al. Structural analysis of the regulatory region of the human corticotropin releasing hormone gene. FEBS Lett. (1990) 267:1–5. doi: 10.1016/0014-5793(90)80272-K
35. Gao T, Newton AC. The turn motif is a phosphorylation switch that regulates the binding of Hsp70 to protein kinase C. J Biol Chem. (2002) 277:31585–92. doi: 10.1074/jbc.M204335200
36. Blake MJ, Udelsman R, Feulner GJ, Norton DD, Holbrook NJ. Stress-induced heat shock protein 70 expression in adrenal cortex: an adrenocorticotropic hormone-sensitive, age-dependent response. Proc Natl Acad Sci USA. (1991) 88:9873–7, doi: 10.1073/pnas.88.21.9873
37. Udelsman R, Blake MJ, Stagg CA, Holbrook NJ. Endocrine control of stress-induced heat shock protein 70 expression in vivo. Surgery. (1994) 115:611–616.
Keywords: corticotropin-releasing hormone, cortisol, heat shock protein, hypothalamus, medaka, sex determination
Citation: Uchimura T, Hara S, Yazawa T, Kamei Y and Kitano T (2019) Involvement of Heat Shock Proteins on the Transcriptional Regulation of Corticotropin-Releasing Hormone in Medaka. Front. Endocrinol. 10:529. doi: 10.3389/fendo.2019.00529
Received: 21 May 2019; Accepted: 17 July 2019;
Published: 02 August 2019.
Edited by:
Honoo Satake, Suntory Foundation for Life Sciences, JapanReviewed by:
Yuji Oshima, Kyushu University, JapanJuan Ignacio Fernandino, CONICET Institute of Biotechnological Research (IIB-INTECH), Argentina
Copyright © 2019 Uchimura, Hara, Yazawa, Kamei and Kitano. This is an open-access article distributed under the terms of the Creative Commons Attribution License (CC BY). The use, distribution or reproduction in other forums is permitted, provided the original author(s) and the copyright owner(s) are credited and that the original publication in this journal is cited, in accordance with accepted academic practice. No use, distribution or reproduction is permitted which does not comply with these terms.
*Correspondence: Takeshi Kitano, tkitano@kumamoto-u.ac.jp