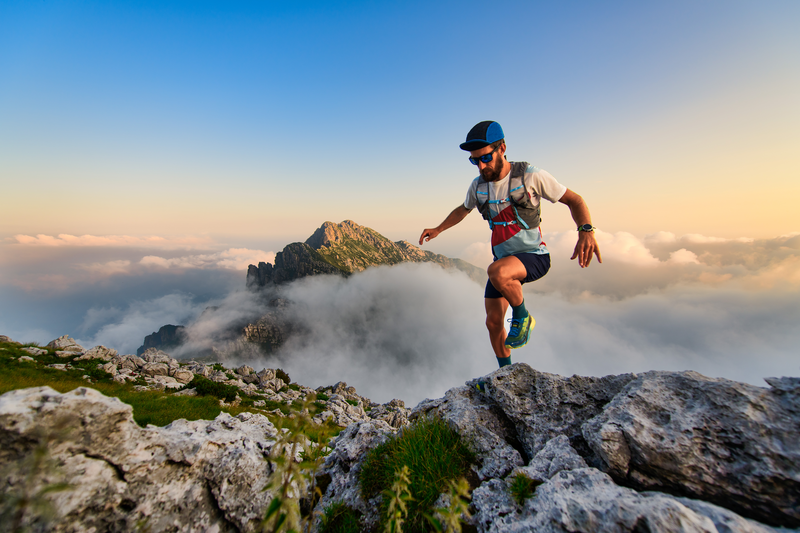
94% of researchers rate our articles as excellent or good
Learn more about the work of our research integrity team to safeguard the quality of each article we publish.
Find out more
REVIEW article
Front. Endocrinol. , 12 July 2019
Sec. Neuroendocrine Science
Volume 10 - 2019 | https://doi.org/10.3389/fendo.2019.00471
This article is part of the Research Topic GPCR in Inflammatory and Cancer Diseases View all 7 articles
It is well-established that the chemokine C-X-C motif ligand 13 (CXCL13) and its receptor, the G-protein coupled receptor (GPCR) CXCR5, play fundamental roles in inflammatory, infectious and immune responses. Originally identified as a B-cell chemoattractant, CXCL13 exerts important functions in lymphoid neogenesis, and has been widely implicated in the pathogenesis of a number of autoimmune diseases and inflammatory conditions, as well as in lymphoproliferative disorders. Current evidence also indicates that the CXCL13:CXCR5 axis orchestrates cell-cell interactions that regulate lymphocyte infiltration within the tumor microenvironment, thereby determining responsiveness to cytotoxic and immune-targeted therapies. In this review, we provide a comprehensive perspective of the involvement of CXCL13 and its receptor in cancer progression. Studies in recent years postulated novel roles for this chemokine in controlling the cancer cell phenotype, and suggest important functions in the growth and metastatic dissemination of solid tumors. Carcinogens have been found to induce CXCL13 production, and production of this chemokine within the tumor milieu has been shown to impact the proliferation, migration, and invasive properties of cancer cells. Thus, the complex networks of cellular interactions involving tumoral CXCL13 and CXCR5 integrate to promote cancer cell autonomous and non-autonomous responses, highlighting the relevance of autocrine and paracrine interactions in dictating the cancer phenotype. Dissecting the molecular and signaling events regulated by CXCL13 and how this chemokine dynamically controls the interaction between the cancer cell and the tumor microenvironment is key to identify novel effectors and therapeutic targets for cancer treatment.
Chemokines are a family of small molecular weight proteins known for their ability to act as chemoattractants, thereby functioning to induce the migration of nearby responding cells. These secreted proteins, together with a host of other extracellular mediators, including growth factors and eicosanoids, are key modulators of inflammation by controlling complex interaction networks via autocrine and paracrine mechanisms. Multiple diseases have been associated with aberrant production of chemokines and cytokines, including infectious diseases, chronic inflammation, and autoimmune disorders (1–4).
As part of the large family of cytokines, chemokines act on specific membrane receptors to activate signaling cascades that impact gene expression, thus inducing the production of other factors that contribute to the intricacy of cell-cell interactions. A distinctive characteristic of chemokines is the nature of receptors to which they bind. Unlike cytokines, such as IL-1 that binds to type I transmembrane receptors or TNFα that binds to pre-assembled transmembrane trimers, chemokines act through the activation of G protein-coupled receptors (GPCRs). Binding of chemokines to their cognate seven transmembrane GPCRs triggers structural rearrangements of the receptor that promotes its coupling with heterotrimeric G-proteins, leading to second messenger generation and the activation of intracellular signaling pathways (5–7).
To-date, more than 40 chemokines have been identified in humans, which are in all cases mostly basic, structurally related proteins of 8–14 kDa. Chemokines can be grouped into four classes based on the positioning of their N-terminal cysteine residues: CC, CXC, XC, and CX3C (8–11). The different chemokines play fundamental roles in development, homeostasis, and function of the immune system. Aside from chemotaxis, chemokines are known to have additional functions, including roles in cell proliferation, angiogenesis, and T-cell differentiation. Chemokines are also key players in inflammation, and their levels could be significantly elevated in tissues and plasma of patients with inflammatory conditions. Examples include well-established association between chemokines and diseases such as rheumatoid arthritis, asthma, and psoriasis (12–14).
The causal association between inflammation and cancer has been recognized for decades. Extensive data link chronic inflammatory processes, tissue injury, or infections and the development of cancer. In addition to cancer cells, solid tumors comprise a variety of stromal cells, such as fibroblasts and endothelial cells, as well as inflammatory cells that include lymphocytes, macrophages and neutrophils. Inflammatory cells in the tumor microenvironment functionally interact with cancer cells and other cells within that milieu to impact cancer cell growth and survival, invasion, and metastatic dissemination. In the tumor microenvironment, cancer cells, tumor-associated leukocytes, and stromal cells synergize for the local production of chemokines, among other factors. Tumor-derived chemokines also determine the inflow of leukocytes into the tumor. In the context of tumorigenesis, certain chemokines favor tumor growth and progression, while others boost anti-tumor immunity. The opposite functions of chemokines on tumor development involve attracting cells with pro- or anti-tumoral features (15–19). In this article, we comprehensively review the involvement of the chemokine C-X-C motif ligand 13 (CXCL13) in cancer progression, focusing primarily in novel aspects of CXCL13 biology in solid tumors.
Interest in chemokines grew tremendously in the early 1980's after the identification of soluble factors responsible for leukocyte and lymphocyte migration and activation. Whereas, early studies identified chemoattractants for monocytes, eosinophils, neutrophils, and T lymphocytes (20–23), B-cell specific chemokines remained enigmatic until the late 1990s, several years after discovery of the receptor for CXCL13, CXCR5 (originally called BLR1) (24).
First identified by comparing gene expression in malignant vs. benign B-cells, BLR1 (Burkitt's Lymphoma Receptor 1) mRNA was detected in Burkitt's lymphoma cell lines and lymphatic tissue but not in undifferentiated B-lymphocytes or hematopoietic cells of myeloid, monocytic, erythroid, or T-lymphocytic origin (24). This finding suggested a role for BLR1 in B-cell development and trafficking into lymphoid tissues, which was later demonstrated in mouse models (25). Because BLR1 mRNA encoded for a protein with a predicted structure containing seven hydrophobic transmembrane segments with a high similarity to the IL-8 receptor, BLR1 was thought to be the first GPCR identified in B-lymphocytes. The physiological importance of this BLR1 receptor was revealed through a gene deletion approach. BLR1-deficient mice failed to develop inguinal lymph nodes and demonstrated severely compromised primary follicle and germinal center formation in the spleen and Peyer's patches (26). This phenotype was explained in experiments assessing the migration pattern of BLR1-deficient B-cells when transferred into wild-type mice, which showed that BLR1−/− B lymphocytes entered T-cell areas of lymphoid tissues but not areas that foster B-cell development that are normally populated by B-cells. Altogether, these studies provided strong evidence that BLR1 was the receptor for a yet-unidentified factor and that its activity facilitated B-cell homing and development in lymphoid tissue.
By searching EST databases for putative CXC chemokines, two simultaneous studies reported the identification of the ligand for BLR1 (27, 28). The novel recombinant chemokine induced B-lymphocyte chemotaxis and it did so only in B-cells expressing BLR1 but not other known chemokine receptors. This was the first identification of a B-cell specific chemokine, and it was termed B cell-Attracting Chemokine 1 (BCA-1). The BCA-1 transcript hybridized most strongly to B-cell-rich follicles in the spleen, germinal centers in Peyer's patches, and lymph node follicles. The BCA-1 protein sequence contained four cysteine residues in a typical CXC chemokine pattern and was located on chromosome 4q21, in close proximity to most other known CXC chemokine genes (29). This chemokine was later renamed CXCL13.
The receptor for BCA-1/CXCL13, later renamed CXCR5, has ~40% amino acid homology to CXCR1, the receptor for IL-8 (30). Building on this sequence homology and the well-characterized structure and function of CXCR1, CXCR5-CXCR1 chimeras were used to dissect the function of the intercellular domains of CXCR5. Similar to CXCR1, CXCR5 activation was found to stimulate intracellular Ca2+ influx, ERK/MAPK signaling, and induce cellular chemotaxis (31), an effect that was mediated primarily by the second intracellular domain of CXCR5, called IC2. The CXCR5 IC2 shares 52% amino acid homology with CXCR1, including a DRY motif followed by a conserved YLXIV motif that is common to most chemokine receptors at the junction of the third transmembrane domain and IC2, which facilitates binding to intracellular heterotrimeric G proteins (32). Although the signaling pathways activated downstream of the GPCR CXCR5 have not been fully analyzed, it is known that this receptor couples to the PI3K/Akt, MEK/ERK, and Rac pathways to induce multiple cellular responses, not only in immune cells but also in cancer cells, as it will be discussed in other sections of this review. These pathways are depicted in Figure 1.
Figure 1. Activation of signaling pathways by CXCL13. The chemokine CXCL13 binds specifically to the GPCR CXCR5. Upon activation, CXCR5 couples to the activation of pathways implicated in cell survival, proliferation, and migration, therefore impacting on the tumorigenic and metastatic activity of cancer cells.
Ectopic germinal center-like lymphoid structures have been recognized within the affected tissues in numerous autoimmune diseases, including myasthenia gravis (33) and rheumatoid arthritis (34), long before the cloning of CXCL13 and CXCR5. After the studies outlined above identified the roles of CXCL13 and CXCR5 in the development of differentiated B-cells and their secondary lymphoid structures, it was natural to hypothesize that aberrant activation of this signaling axis contributes to autoimmune conditions. Abnormal lymphocyte aggregates similar to germinal centers were known to form within the synovium of affected joints in rheumatoid arthritis. Indeed, strong overexpression of CXCL13 mRNA and protein was found in the synovia of patients with rheumatoid arthritis, particularly in the regions of B-cell aggregation (35). Larger-scale analysis of patient synovia demonstrated an extremely high correlation between synovial B-cell rich germinal centers and CXCL13 overexpression, strongly suggesting that CXCL13 signaling contributes to the pathogenesis of these aberrant lymphoid structures (36). Similar contributions of CXCL13 to the formation of ectopic lymphoid structures was later identified in Sjögren's syndrome (37, 38), autoimmune thyroiditis (39), myasthenia gravis (40), systemic lupus erythematosis (SLE) (41), and multiple sclerosis (42). CXCL13 expression has been correlated with disease exacerbation and unfavorable prognosis in multiple sclerosis, Sjögren's (43), myasthenia gravis, and SLE, and CXCL13 has been proposed as a biomarker for diagnosis and progression in these conditions (44).
Whereas, the CXCL13:CXCR5 axis has been best characterized in these autoimmune disorders through the aberrant activity and differentiation of B-cells, numerous other autoimmune conditions appear to be driven by T follicular helper cells TFH cells (45, 46). TFH cells develop from naïve CD4+ T-cells within the interfollicular T cell zone area of lymphoid tissue in response to signals from dendritic cells that cause upregulation of CXCR5 and down-regulation of the T-cell homing marker CCR7 (47). This facilitates relocation of CD4+/CXCR5+/CCR7– TFH cells from T-cell zones to germinal centers within B-cell follicles, where they function primarily to support B-cell expansion and differentiation. Dysregulation of this process in autoimmune disease was first described in SLE (48), wherein an aberrant overabundance of TFH cells can cause spontaneous germinal center formation and autoimmunity (49). Indeed, circulating CXCR5+/CD4+ T-cells that resemble TFH cells have been identified in SLE patients, where they facilitate pathologic B cell differentiation and correlate with disease progression. Pathogenic roles for TFH cells have also been reported for many other autoimmune entities such as Sjögren's syndrome (50), primary biliary cholangitis (51), vitiligo (52), ankylosing spondylitis (53), and pemphigus vulgaris (54). Overall, dysregulation of the CXCL13:CXCR5 axis affecting both B- and TFH cell function is major player in autoimmune disorders, and potentially serves as a biomarker for disease progression and therapeutic response.
Based on the crucial roles the CXCL13:CXCR5 axis plays in both physiologic and pathologic immunity, it is not surprising that this axis has been implicated in the pathogenesis of a number of infectious diseases. Before CXCL13 had been cloned, CXCR5 was identified as a co-receptor for HIV-2 that renders THF cells susceptible to viral infection. Expression of CXCR5 and CXCL13 was shown to be dysregulated in HIV infection, such that the number of CXCR5+ B cells decreases with progression of HIV infection, together with an increase in plasma levels of CXCL13 (55). Later studies confirmed elevations of serum CXCL13 during chronic HIV infection and showed that CXCL13 levels correlated both with disease progression and viral load. CXCL13 levels decrease after highly active antiretroviral treatment (HAART) (56).
Mechanistically, a loss of CXCR5+ B-cells in HIV viremia was shown to drive expansion of CXCR5+ TFH cells within lymph nodes. These TFH cells secrete cytokines, such as IL-21, that induce plasma cell differentiation and immunoglobulin secretion, namely IgG1, explaining the B cell dysfunction and hypergammaglobulinemia observed in chronic HIV infection (57). Later studies confirmed expansion of CXCR5+ THF cells within lymph nodes during HIV infection and demonstrated that TFH cells serve as the major in vivo reservoir for HIV infection, replication, and production (58). Whereas, TFH cells within lymphoid tissues are expanded, the frequency and functionality of peripheral CXCR5+ TFH cells has been shown to decline during chronic HIV-1 infection. A subset of CXCR5+ THF cells that also express programmed death-1 (PD-1) have been shown to facilitate the development of a strong B-cell response in early HIV infection (59), and preservation of peripheral CXCR5+ TFH cells correlate with long-term control of infection. Finally, by demonstrating temporal correlation of CXCL13 plasma levels with progression of HIV infection, CXCL13 was proposed as a biomarker of systemic immune activation during HIV infection that may serve to predict AIDS-defining events (60).
It is clear that the CXCL13:CXCR5 axis is intimately involved in the initial and chronic phases of HIV infection, and, considering the central role this axis plays in humoral immunity, it is not surprising that CXCL13 has been implicated in the pathogenesis of several other infectious diseases. Of these, perhaps the best studied are the stages of Lyme disease and syphilis affecting the central nervous system (CNS) (Lyme neuroborreliosis and neurosyphilis). CXCL13 is overexpressed within the muscles of monkeys chronically infected with Borrelia burgdorferi (the etiological agent of Lyme disease), and CXCL13 was later shown to contribute to the formation of ectopic germinal centers within the central nervous system. Interestingly, whereas infection with B. burgdorferi appears to have no impact on plasma CXCL13 levels, once the bacteria establishes CNS infection, it leads to constitutively elevated CXCL13 levels in cerebrospinal fluid (CSF), which could be often more than several 100-fold greater than in the plasma (61). CXCL13 appears to recruit B-cells within the CNS and facilitate their differentiation to plasma cells that produce a burgdorferi-targeted humoral response. Indeed, CSF CXCL13 level has been proposed as a diagnostic biomarker for neuroborreliosis, and a recent meta-review of 18 studies calculated a pooled sensitivity and specificity of 89 and 96%, respectively, for CNS CXCL13 as a biomarker of disease (62).
Similar to neuroborreliosis, CXCL13 has been implicated in the pathogenesis of neurosyphilis, a serious complication of untreated syphilis. Neurosyphilis is typically a late manifestation of prolonged infection but can also occur in early disease, and it generally manifests as chronic meningitis, stroke-like symptoms, or neurological symptoms (dementia, tabes dorsalis, and paresis). Notably, CSF levels of CXCL13 were found to be 100-fold higher in patients infected with Treponema pallidum (the etiological agent of syphilis) than in uninfected individuals, although approximately four times lower than individuals with neuroborreliosis. Mechanistically, enrichment and activation of B-cells have been observed within the CNS in neurosyphilis, as well as ectopic germinal centers, suggesting that CNS T. pallidum infection leads to CXCL13 overexpression and a positive feedback loop that recruits and activates a strong humoral response within the CNS that contributes to destruction of neurological tissue (63). Similar to neuroborreliosis, CSF levels of CXCL13 have been proposed as a biomarker for neurosyphilis with a sensitivity and specificity of 85 and 89%, respectively (64), with the highest diagnostic value being in HIV-infected patients (65).
As outlined in the previous section, CXCL13 is strongly expressed by dendritic cells in the follicles within the spleen, lymph nodes, and Peyer's patches, where it binds to CXCR5 on mature B cells and THF cells to facilitate the development of these B cell-rich structures and B-cell differentiation. Imbalances in the CXCL13:CXCR5 axis may contribute to pathologies involving B-cells and THF cells. Early studies revealed that CXCL13 and CXCR5 are highly expressed in primary and secondary follicles within gastric lymphomas (66). Malignant cells in follicular lymphoma, which mimics the architectural and cellular structures of normal secondary lymphoid follicles in ectopic neoplastic foci, were shown to express CXCR5, secrete CXCL13, and migrate in response to CXCL13, suggesting that CXCL13 recruits malignant B-cells to ectopic germinal centers and contributes to their development (67). Shortly thereafter, overexpression of CXCL13 or CXCR5 was demonstrated in primary central nervous system B-cell lymphoma (68), cutaneous B and T-cell lymphoproliferative disorders, intraocular lymphomas, and chronic lymphocytic leukemia (69).
Just as CXCL13 has demonstrated potential clinical utility as a biomarker in infectious diseases involving the CNS, this chemokine has been also proposed as a marker of certain lymphomas. One of the strongest correlations has been demonstrated for angioimmunoblastic T-cell lymphoma (AITL), an aggressive nodal T cell lymphoma that accounts for approximately 1.5% of all non-Hodgkin lymphoma and 20% of peripheral T cell lymphomas. Because AITL is derived from TFH cells, CXCL13 was first suggested as a biomarker for this disease (70), and the 2016 WHO classification of lymphomas included CXCL13 in the diagnostic criteria for AITL (71). Elevated CXCL13 levels in the CSF have been demonstrated for CNS lymphomas (72), and when elevated together with IL-10 in the CSF, CXCL13 has a >99% specificity for primary and secondary lymphomas, leading to similar proposals that it serves as a biomarker for non-Hodgkin lymphoma involving the CNS (73).
Very recently, CXCL13 was identified as the most up-regulated cytokine in plasma from patients with idiopathic multicentric Castleman Disease, a poorly understood syndrome involving a “cytokine storm” driving polyclonal lymphoproliferation. Elevated CXCL13 plasma levels has been demonstrated in lymph nodes from these patients, likely in follicular dendritic cells and TFH cells (74). A schematic representation of the major physiological and pathological functions of CXCL13 is depicted in Figure 2.
Figure 2. Major functions of CXCL13 in immune responses, inflammation, and lymphoproliferative diseases. The chemokine CXCL13 has been identified as a major regulator of immune responses and plays key roles in the pathophysiology of inflammatory, infectious, and lymphoproliferative diseases. In addition, emerging evidence identified CXCL13 as a biomarker for cancer progression and response to therapy.
Naive CD4+ T helper precursor cells can differentiate into a variety of different T helper subsets, including T helper type-1 (exerting antitumoral responses), T helper type-2 (displaying inhibition of antitumoral responses), T helper 17 and regulatory T-cells. Noteworthy, T helper type 1-cells produce a particular set of cytokines such as IL-2, IFNγ, and TNFα, while T helper type-2 cells produce IL-4, IL-5, IL-6, IL-10, and IL-13. It has been reported that a shift from an immunological pattern with a T helper type-1 orientation to a T helper type-2 pattern mediated by cytokines is a key event in carcinogenesis (75, 76) (Figure 3A).
Figure 3. CXCL13 and the formation of tertiary lymphoid structures. (A) Cytokines play key roles in the immunomodulation of both T helper type-1 (cellular immunity) or T helper type-2 responses (humoral immunity). The shift from an immunological pattern with a T helper type-1 orientation to a T helper type-2 pattern mediated by cytokines is a key event in tumorigenesis. (B) CXCL13 produced by T-cells plays a fundamental role in the formation of tumor-associated tertiary lymphoid structures and germinal center orchestration: tumor infiltrating T-cells are recruited into the tumor site by transendothelial migration via high endothelial venules mediated by chemokine/chemokine receptor interactions. Intra-tumoral infiltration of TFH cells is a key step in the formation of tertiary lymphoid structures, thus generating and promoting B-cell responses in the germinal center (i.e., local memory B cell differentiation, as well as with the expansion of a subpopulation of T regulatory cells).
Tertiary lymphoid structures present in the tumor microenvironment of solid tumors are basically characterized by mature dendritic cells in a T-cell zone adjacent to B-cell follicle including a germinal center representing sites of lymphoid neogenesis (77–79) (Figure 3B). These tertiary lymphoid structures are essential sites for the initiation and/or maintenance of the local and systemic T- and B-cell responses against tumors and associate with a favorable clinical outcome for cancer patients. Hence, they can be considered novel biomarkers to stratify the overall survival risk of untreated cancer patients and as markers of efficient immunotherapies (80). Going deeper into the analysis of tertiary lymphoid structure neogenesis, several studies revealed an important participation of the CXCL13:CXCR5 axis in this process. For instance, the presence of functional tertiary lymphoid structures has been associated with long-term survival in lung cancer patients, and signaling by CXCL13, among other chemoattractants, has been shown to mediate T-cell recruitment to tertiary lymphoid structures (81). Regarding the orchestration of the T-cell migration mechanisms, it was demonstrated that T-cells express CXCR5. A new gateway mechanism proposed for T-cell migration into the tumor involves their recruitment via high endothelial venules mediated by chemokine/chemokine receptor interactions, thus reinforcing the concept that recruitment of tumor specific T-cells to intratumoral tertiary lymphoid structures is mediated by the CXCL13:CXCR5 axis (82).
Infiltration of TFH cells (83) is important for tertiary lymphoid structure formation and to generate germinal center B cell responses at the tumor site by the production of CXCL13. These tumor-infiltrating CXCL13-producing TFH cells were linked with promoting local memory B cell differentiation, as well as with the expansion of a subpopulation of T regulatory cells. Moreover, these cells were also related with the de novo activation of adaptive antitumor humoral responses in the chronic inflammatory breast cancer microenvironment. CXCL13:CXCR5 axis is involved in many biological responses in immune cells as well as in cancer cells (Figure 4), as described in subsequent sections.
Figure 4. The CXCL13:CXCR5 axis is a key element in B-cell and tumor cell responses. The chemokine CXCL13, acting on the GPCR CXCR5, promotes chemotaxis, germinal center formation, and the differentiation to plasma cells and B-memory lymphocytes. CXCL13 also target cancer cells to promote proliferation, migration, and invasion. These effects vary depending on the cancer type, which may express different levels of CXCR5.
Lung cancer is the leading cause of cancer-related deaths worldwide. The immune system exerts a central role in the lung cancer biology given, at least in part, by its pro-inflammatory milieu. While several studies provided solid evidence supporting the starring role of the CXCL13:CXCR5 axis in the promotion of lung tumor progression, studies focused on CXCL13 produced by immune cells also highlight its high predictive potential for response to immunotherapy.
CXCL13 was initially identified as part of an invasive network module in lung adenocarcinomas (84–86). A comparative analysis of an inflammatory signature in non-small cell lung cancer (NSCLC) and chronic obstructive pulmonary disease (COPD) patients revealed that CXCL13 was included among the chemokines elevated in NSCLC patients (86, 87). An inflammation score based on CXCL13 and three additional markers (CRP, MDC/CCL22, and IL-1RA) provided good separation in 10 year cumulative risks of lung cancer. CXCL13 was also identified as a predictive factor for risk of early stage lung adenocarcinoma (88). A specific gene expression signature associated with T-cell presence in tertiary lymphoid structures was identified in human lung cancer, which includes CXCL13 and other chemokines (89).
One interesting recent study focused on the prognostic relevance of tertiary lymphoid structures in lung squamous cell carcinoma patients treated with corticosteroid together with chemotherapy (90). This study identified a perivascular CXCL13 positive niche that supports tertiary lymphoid structure development, which is associated with improved patient survival. Steroid treatment impaired the formation of these lymphoid structures when compared with those untreated patients, who showed high tertiary lymphoid structures intratumoral density, as well as the expression of adaptive-immune response genes. This led to the hypothesis that corticosteroid treatment as neoadjuvant therapy abrogates germinal center formation with the subsequent loss of the tertiary lymphoid structures (90). Recent evidence also showed that CD8+ lymphocyte populations with high PD-1 expression from NSCLC patients express and secrete very high levels of CXCL13. Secretion of CXCL13 by the tumor infiltrating lymphocytes expressing high PD-1 levels serves to attract other immune cell subsets to the tumor microenvironment, including TFH cells and B-cells, and, strikingly, it strongly predicts response to anti-PD-1 therapy that correlates with increased overall survival and durable responses. Thus, CXCL13 and the immune cells that produced this chemokine in the NSCLC tumor microenvironment may represent novel biomarkers for response to targeted PD1/PD-L1 therapy (91).
Compelling data for the involvement of CXCL13 in lung cancer development was provided by Wang et al. (92), who studied the relationship between lung cancer development and environmental pollution in Xuanwei, a city in the Yunnan Province with one of the highest lung cancer incidences in China. This has been attributed to smoky coal combustion-generated polycyclic aromatic hydrocarbons (PAHs) pollution. A screening analysis of abnormally high inflammatory factors in NSCLC patients from this region revealed that 90% of the tumor tissues have elevated CXCL13 levels. CXCR5 could be also detected in human lung tumors, however its levels were not significantly different between cancer and normal tissue. The causal link between carcinogens and CXCL13 production was confirmed using a normal human lung epithelial exposed to the PAH benzo(a)pyrene (Ba[a]P), which caused elevated transcriptional activity of the CXCL13 gene via the aryl hydrocarbon receptor (AhR), a ligand-activated transcription factor that binds to a xenobiotic-responsive element (XRE) in this gene located 1.7 kb downstream from the transcription start site. Lung tumors from mice treated with Ba[a]P display elevated CXCL13 mRNA and protein levels. The relevance of CXCL13 in Ba[a]P-induced lung cancer was further confirmed using CXCL13 and CXCR5 knockout mice, which have impaired tumor formation in response to the carcinogen. CXCL13 within the tumors was produced by CD68+ macrophages. CXCL13 markedly increase the production of the macrophage-secreted cytokine SPP1 (secreted phosphoprotein-1 or osteopontin). The potential involvement of SPP1 was confirmed by silencing its expression, which led to impaired migration of lung cancer cells in co-culture models. CXCL13 and SPP1 serum concentrations were elevated in B[a]P-treated mice, and tumors show elevated SPP1 staining. Notably, the CXCL13-CXCR5-SPP1 signal induced an EMT phenotype in lung tumors from B[a]P-treated mice, which was characterized by E-cadherin down-regulation and up-regulation of mesenchymal markers N-cadherin, vimentin, Slug and Snail. SPP1 overexpression was also linked to increased levels of nuclear β-catenin.
Another study in NSCLC patients revealed significantly higher expression of CXCR5 in carcinomas relative to non-neoplastic lung tissue (93). Further, nuclear and membrane CXCR5 intensities were higher in NSCLC relative to non-neoplastic tissues. The relevance of the nuclear CXCR5 staining is unknown. Interestingly, this study also revealed higher levels of CXCL13 in serum of NSCLC patients compared to healthy controls. The functional significance of the high CXCR5 expression was analyzed using migration assays, which revealed a pro-migratory phenotype in NSCLC cells subject to CXCL13 stimulation. These results suggest that CXCL13 in the tumor microenvironment may act upon the cancer cell, possibly contributing to tumorigenic and metastatic phenotypes. It would be of great interest to determine whether lung cancer cells respond to CXCL13 by activating signaling pathways related to proliferation and survival, as this may reveal important direct effects of the chemokine resulting from paracrine or autocrine mechanisms.
Breast cancer exhibits one of the strongest relationships between CXCL13:CXCR5 axis and tumor progression. Using a microarray analysis, a study found significant CXCL13 overexpression in breast cancer specimens (94). Moreover, CXCL13 was identified as the most strongly overexpressed chemokine in breast cancer tissue compared with normal breast tissue. A positive correlation was found between the expressions of CXCL13 and CXCR5 in breast tumors. Analysis in breast cancer cell lines also revealed high levels of CXCL13 and expression of CXCR5. Strikingly, both ligand and receptor could be only detected intracellularly in cell lines growing in culture, with no measurable levels of the CXCL13 in the culture medium (by ELISA) or plasma membrane CXCR5 (by flow cytometry). Nonetheless, elevated serum concentrations of CXCL13 could be detected in patients with metastatic disease, possibly reflecting differences between 2-D cultures and tumors. Biswas et al. reported high co-expression of CXCL13 and CXCR5 within primary breast tumors, as determined by immunohistochemistry (95). Chemokine and receptor up-regulation are driven by different mechanisms involving transcriptional control via RelA and Nrf2 in the case of the CXCL13 gene, and epigenetic regulation (lack of CpG island methylation) in the case of the CXCR5 promoter (95). Along the same line, a study revealed high CXCL13 expression in primary tumors from Chinese young breast cancer patients (≤45 yo) but not in those from older women (≥65 yo). Moreover, CXCL13 expression was associated with grade 2/3, lymph node positive and ER negative status (96). Despite the differential CXCL13 expression found in breast cancer, a study failed to show elevated CXCL13 plasma levels in breast cancers patients relative to healthy controls (97). CXCL13 was also included in a set of 14 prognostic gene predictors of longer metastasis-free survival in early stage hormone receptor-negative and triple-negative breast cancer (TNBC) (98).
The analysis of a T-cell signature in breast cancer that includes CXCL13 revealed additional levels of complexity. In univariate analysis, mRNA expression of CXCL13 and other T-cell markers associate with longer metastasis free-survival, with a stronger prognostic effect in HER2 positive breast cancer (99). In a recent study, a four-gene signature that includes CXCL13 and predicts the extent of lymphocytic infiltration after neoadjuvant therapy in TNBC has been developed. This signature associates with good outcome, adding novel prognostic information for this aggressive breast cancer subtype (100). Likewise, the recent association between high CXCL13 and distant disease-free survival in early-stage breast cancer patients provided evidence that humoral immunity influences the survival outcomes in these patients, particularly those with TNBC (101).
In addition to the immune-related effects of CXCL13 in breast cancer, this chemokine exerts direct effects on breast cancer cells. In an early study using CXCR5-expressing breast cancer cells, CXCL13 induced changes in the expression of markers consistent with epithelial-to-mesenchymal transition (EMT). Indeed, CXCL13 up-regulates mesenchymal markers vimentin, Snail, Slug, N-cadherin, MMP9 and RANKL while down-regulating E-cadherin expression. In addition, CXCL13-treated breast cancer cells acquire a more elongated shape and a highly migratory phenotype characteristic of mesenchymal cells. From a signaling perspective, these effects are sensitive to p110α PI3K and Src inhibition (95). Interestingly, a recent study showed that an anti-CXCL13 antibody reduces MDA-MB-231 breast cancer cell viability by promoting apoptosis. CXCL13 inhibition reduced active ERK and cyclin D1 levels, and enhanced caspase-9 cleavage (47). Extra backing proof of the concept that CXCL13 plays a pivotal role in breast cancer growth and lymph node metastasis was gained in tumorigenesis experiments using 4T1 breast cancer cells. Treatment of mice with an anti-CXCL13 antibody impaired 4T1 tumor growth and ERK activation, thus affording a theoretical frame for clinical trials targeting CXCL13 (102).
An inverse correlation between the expression of CXCR5 and the p53 tumor suppressor was reported in the MCF-7 human breast cancer cell line. Silencing of p53 in these cells not only up-regulates CXCR5, but also potentiates CXCL13-mediated chemotaxis. Therefore, CXCR5 up-regulation may contribute to the anomalous phenotypes of p53-deficient cells. A CXCR5 promoter analysis revealed that p53 acts indirectly by repressing the activity of NF-κB transcription factors (103). Similar mechanisms for CXCR5 gene regulation may operate upon loss of related tumor suppressors p63 and p73 (104). These studies suggest a potential relationship between genotoxic stress and CXCR5 responses that could have significant prognostic and therapeutic implications in the context of chemotherapy.
Colorectal carcinoma is one of the most common malignancies worldwide. Extensive analysis of circulatory inflammatory factors at the time of colorectal cancer surgery, including cytokines, chemokines and interleukins, revealed CXCL13 as one of the factors associated with increased mortality (105). In agreement with this information, immunohistochemical analysis of advanced colorectal cancer specimens revealed significant elevation of CXCL13 and CXCR5 in tumors relative to normal tissue, which correlated with lymph node metastasis and neural invasion. Furthermore, patients with positive CXCR5 and CXCL13 expression have poor prognosis, both in terms of 5 year overall survival and 5 year relapse-free survival. Interestingly, CXCR5 staining localized primarily in the epithelial cells, whereas CXCL13 staining was mainly found in the mesenchymal cells (106). These results suggest a role of CXCR5 and CXCL13 as prognostic markers for colorectal carcinoma progression.
From a functional standpoint, CXCL13 induces proliferation and migration in CXCR5-expressing colon cancer cells (107). Interestingly, CXCR5 expression in mouse CT26 colon carcinoma was low in vitro, up-regulated in vivo, and rapidly lost when cells were explanted in vitro. In the liver, after intrasplenic injection, these CXCR5 transfectants initially grew faster than controls; however, the growth rate of control tumors accelerated later to become similar to the transfectants, likely due to CXCR5 up-regulation. These results suggest that expression of CXCR5 in tumor cells promotes their growth in the liver and, at least for CT26 cells, the receptor is required for outgrowth to large liver tumors. CXCL13 also promotes growth, migration, and invasion in human SW620 colon carcinoma cells. From a signaling standpoint, CXCL13 activates, and the migratory/invasive phenotype is sensitive to PI3K inhibition (108). This has significant clinical implications since abnormal PI3K signaling is a hallmark of colon cancer (109). Studies using the Nirp12 KO mouse model revealed that elevated CXCL13 expression occurs as a consequence of high non-canonical NF-κB activation (85). As in other cancers, CXCL13 and its receptor also play roles within the immune landscape of colon cancers (110).
In gastric cancer, CXCL13 expression is predictive of shorter overall survival. Within the T2–T4 stage patient group, low CXCL13 expression is associated with longer survival, particularly in patients who received adjuvant chemotherapy. High CXCL13 levels in gastric tumors are associated with larger tumor diameters (111). Notably, a transcriptome analysis of biopsies from gastric cancer patients revealed significant up-regulation of CXCL13, which is mainly expressed in isolated lymphoid follicles and small lymphoid aggregates (112). In gastric biopsies from H. Pylori infected patients, who have increased risk of developing gastric carcinoma, there is also significant CXCL13 overexpression (113). This finding fits with an early analysis in H. Pylori gastritis showing that CXCL13 expression was mainly confined to lymphocyte aggregates as well as to primary and secondary follicles. Analysis of CXCL13 expression in mucosa-associated lymphoid tissue (MALT) lymphomas shows that the transformed blasts seem to be the major source of the chemokine (66). Consistent with observations in other cancers, serum levels of CXCL13 were higher in patients with gastric cancer compared to healthy individuals, and this correlates with a high number of circulating TFH cells. Notably, CXCL13 concentrations were higher in patients with lymph node metastasis and high-grade disease (114).
CXCL13 also seems to play a prominent role in the development of pancreatic adenocarcinoma, one of the most aggressive and incurables forms of cancer. Human pancreatic carcinoma cell lines express CXCR5, and this GPCR can be detected in a significant proportion of human pancreatic tumors (107). Several pancreatic ductal adenocarcinoma cell lines display significant elevation of non-canonical NF-κB target genes, including CXCL13. Constitutive activation of the non-canonical NF-κB pathway requires stabilization of the kinase NIK and IKKα-dependent processing of NF-κB2/p100 to p52, which via heterodimerization with RelB heterodimers regulates the expression of genes encoding lymphoid-specific chemokines and cytokines (115). Constitutive activation of the non-canonical NF-κB pathway as observed in pancreatic cancer models may contribute to a positive pro-migratory autocrine loop via CXCR5 activation, as observed in prostate cancer models (see below). More recently, the Simon laboratory identified a pathway in KRas-driven pancreatic tumors that involves CXCL13 and the master regulator of the hypoxic transcriptional response HIF1α. Deletion of the Hif1a gene in mice surprisingly accelerates pancreatic carcinogenesis, which was accompanied by increased infiltration of B lymphocytes. Interestingly, one of the chemokines up-regulated by HIF1α was CXCL13. Depletion of B cells by administration of a CD20-specific monoclonal antibody reduced the number of lesions (116). Taking into consideration that CXCL13 staining can be detected in the stroma surrounding human and mouse PanIN lesions, most likely in the stromal fibroblast population, and that CXCL13 neutralization with a blocking antibody reduces pancreatic B cell infiltration and the growth KRas-driven tumors (117), it is logical to speculate that CXCL13 has a significant contribution to pancreatic tumorigenesis.
Hepatocellular carcinoma (HCC), the primary malignant tumor of the liver, has been highly associated with chronic inflammation related to alcohol intake and viral hepatitis. Many cytokines and chemokines have been linked to chronic liver disease and HCC, including CXCL13. Elevated CXCL13 and CXCR5 expression has been reported in human HCC, with a higher percentage of CXCR5+ or CXCL13+ cells in poorly differentiated tumors compared with well-differentiated tumors (118). Moreover, studies documented elevated CXCL13 serum levels in HCC patients, which correlate with tumor size, metastatic disease, advanced stages, and Alanine Transaminase/Aspartate Aminotransferase serum levels (118, 119). Nonetheless, in hepatitis B-related HCC, elevated serum CXCL13 did not correlate with overall survival and rather correlated with recurrence-free survival (118). Analysis of signaling networks revealed a mutual positive interaction between CXCL13 and the Wnt/β-catenin pathway in promoting liver cancer (119). These studies emphasize the potential roles of the CXCL13:CXCR5 axis as a biomarker in HCC and its potential prognostic relevance.
Prostate cancer is the most commonly diagnosed malignancy and the second leading cause of cancer death in men. Metastasis, particularly to the bone, occurs in most patients when disease becomes androgen-independent (“castration-resistant prostate cancer” or CRPC). Within the last decade, chemokines emerged as key players in prostate cancer progression. In this context, CXCL13 and CXCR5 appear to be highly relevant in prostate cancer cell proliferation, migration, and invasion, ultimately impacting disease progression and metastatic dissemination.
Early studies showed that CXCR5 is expressed in primary prostate cancer tissues at higher levels than normal tissue. In normal tissue and benign prostate hyperplasia samples, CXCR5 displays a predominant membrane and/or cytoplasmic distribution while in advanced prostate cancers it shows high nuclear expression (120). CXCL13 is elevated in serum of prostate cancer patients and was found to be a better predictor of prostate cancer than prostate-specific antigen (PSA). Also, CXCL13 is highly expressed in human bone marrow endothelial cells and osteoblasts, but not in osteoclasts, in response to IL-6 treatment. Furthermore, CXCL13 produced by bone marrow endothelial cells in response to IL-6 was able to induce prostate cancer cell invasion in a CXCR5-dependent manner (120).
Signaling studies revealed that DOCK2 (Dedicator of cytokinesis 2), ERK1/2, JNK and Akt signals mediate CXCL13 invasive and proliferative responses in prostate cancer cells. CXCL13 promotes proliferation in androgen-responsive LNCaP cells in a JNK-dependent, DOCK2-independent manner, whereas this effect is dependent on DOCK2 in androgen-independent PC3 cells. CXCL13-mediated invasion in prostate cancer cells depends on PI3K/Akt, Src, ERK, and FAK, but it is independent of DOCK2 (121, 122). Interestingly, androgen stimulates CXCL13 production in prostate cancer cells, and CXCL13 was found to be an androgen-responsive gene that contains a canonical androgen-responsive element in its promoter. From a functional standpoint, CXCL13 plays an important role in androgen receptor-induced cellular migration and invasion in LNCaP cells (123).
Our laboratory has recently implicated the oncogenic kinase PKCε in prostate cancer progression (124–127). This kinase is overexpressed in prostate cancer and cooperates with loss of the tumor suppressor Pten, a common alteration in prostate cancer, for the development of prostatic adenocarcinoma (128, 129). Gene profiling of PKCε-overexpressing/Pten-deleted prostate epithelial cells revealed CXCL13 as a top deregulated gene. PKCε overexpression and Pten loss (which leads to PI3K activation) up-regulates CXCL13 production and release, contributing to CXCR5 signal amplification, and ultimately resulting in a cell autonomous pro-migratory and tumorigenic autocrine loop. Indeed, silencing CXCR5 from prostate cancer cells reduces their proliferative, tumorigenic and motile capacities. CXCL13 up-regulation in prostate cancer cells is driven by the non-canonical NF-κB pathway. A responsive element for the non-canonical NF-κB pathway has been identified in the CXCL13 gene promoter, and CXCL13 promoter transcriptional activity is sensitive to pharmacological inhibition of PKC, PI3K, and NF-κB. Furthermore, silencing IKKα and NIK, key elements in the non-canonical NF-κB pathway, down-regulates CXCL13 mRNA levels and CXCL13 gene transcriptional activity (128) (Figure 5).
Figure 5. CXCL13 in prostate cancer. In prostate tumors, CXCL13 can be produced both by cancer cells as well as by cells in the tumor microenvironment, such as myofibroblasts. In prostate cancer cells, up-regulation of the kinase PKCε and loss of the tumor suppressor Pten (which leads to elevated PI3K activity) lead to the activation of the non-canonical NF-κB pathway, and transcriptionally activate the CXCL13 gene. CXCL13 produced in this autocrine manner, together with CXCL13 generated by stromal cells, may significantly impact on the tumorigenic and metastatic phenotypes of androgen-independent prostate cancer cells.
Using a Myc-CaP mouse model, Karin and coworkers studied the involvement of CXCL13 in CRPC. After castration, there was elevated expression of CXCL13 in myofibroblasts within the tumor remnants. Immunoablation of FAP (fibroblast activation protein)-expressing cells led to the disappearance of myofibroblasts that express CXCL13 in the tumor stroma of androgen-deprived Myc-CaP tumors, reduced the infiltration of T cells, B cells and dendritic cells into the tumor remnants, and retarded the evolution of CRPC. Mechanistically, FAP ablation prevented IKKα nuclear translocation in cancer cells. Blockade of TGF-β signaling abrogated not only B and T cell infiltration but also the induction of CXCL13-expressing myofibroblasts. Interestingly, cultured fibroblasts isolated from Myc-CaP tumors from non-castrated mice responded to hypoxic conditions by converting into myofibroblasts that produced CXCL13, an effect that is sensitive to phosphodiesterase 5 (PDE5) inhibition with sildenafil. Sildenafil significantly delayed CRPC in castrated Myc-CaP tumor-bearing mice. Myofibroblast activation, immune infiltration, and induction of TGF-β and CXCL13 could be also observed upon castration of TRAMP mice, a model that develops metastatic tumors with neuroendocrine differentiation. This study also identified higher expression of CXCL13 and nuclear HIF-1α in malignant prostate tissue compared with normal tissue or benign prostatic hyperplasia. Furthermore, B cells in malignant tissues were located next to CXCL13-expressing cells. These findings suggest that B lymphocytes recruited into androgen-deprived tumors by CXCL13 play an important role in malignant progression and metastatic dissemination of prostate cancer (130).
Altogether, evidence indicates that a complex network of cellular interactions involving CXCL13 and CXCR5 integrate to promote prostate cancer cell autonomous and non-autonomous pathways. These findings merit further translation into preclinical and clinical arenas, since targeting the CXCL13:CXCR5 axis may be a promising approach for the treatment of CRPC. Recent discoveries linking up-regulation of the CXCL13:CXCR5 axis to the dissemination of prostate cancer stem-like cells to lymph nodes and bone marrow (131) further support this concept.
A very recent study showed significant CXCL13 up-regulation in clear renal cell carcinoma (ccRCC) that correlates with advanced disease stage and poor prognosis, together with elevated CXCL13 serum levels in ccRCC patients. Receiver Operating Characteristic (ROC) curves showed that tissue and serum CXCL13 expression may represent a useful diagnostic biomarker for ccRCC. Notably, patients in the high CXCL13/high CXCR5 expression group have a worse prognosis. CXCL13 promotes the proliferation and migration of ccRCC cells and activates the PI3K/Akt/mTOR signaling pathway. Thus, the CXCL13:CXCR5 axis plays a significant role in ccRCC and could be a valuable therapeutic target and prognostic biomarker (132).
The CXCL13:CXCR5 axis has also been implicated in the initiation and progression of other solid tumors, such as ovarian cancer, melanoma, oral squamous cell carcinoma, osteosarcoma, thyroid cancer, and neuroblastoma (133–143). One interesting study showed that specific chemokine signatures may contribute to overall survival in wild-type and mutant p53 ovarian cancers, and CXCL13 was specifically associated with better overall survival (137). Also, ascites in obese mice have higher levels of macrophages and chemokines including CXCL13, suggesting that obesity may accelerate the peritoneal dissemination of ovarian cancer through higher production of pro-inflammatory chemokines and macrophages recruitment (138). CXCL13 has been also implicated in oral squamous cell carcinoma tumor progression and osteolysis. The tumor necrosis factor family member RANKL (Receptor Activator of NF-κB ligand) plays an important role in cancer invasion of bone/osteolysis. High CXCL13 expression levels have been reported in primary human oral squamous cell carcinoma tumors. c-Myc activation through the CXCL13:CXCR5 signaling axis stimulates RANKL expression in stromal/preosteoblast cells, therefore implicating CXCL13 as a potential therapeutic target to prevent oral squamous cell carcinoma invasion of bone/osteolysis (140).
Of note, immune dysregulation plays a key role in the development of osteosarcoma. Peripheral blood CD4+CXCR5+ T-cells induce B-cell activation and produce a number of cytokines that play critical roles in tumorigenesis. Patients with high tumor grade have an elevated percentage of CD4+CXCR5+ T-cells compared to those with low tumor grade. Moreover, Th1 and Th17 subtypes contribute to the upregulation of peripheral CD4+CXCR5+ T-cells in patients with metastasis or high tumor grade. These results argue for the involvement of peripheral CD4+CXCR5+ T-cells and the CXCL13 pathway in the pathogenesis and progression of osteosarcoma (136).
To summarize, CXCL13 and its receptor CXCR5 have emerged as key players of cancer initiation and progression. The identification of autocrine and paracrine interactions between the tumor microenvironment and cancer cells mediated by CXCL13 highlights how autonomous and non-autonomous mechanisms contribute to the development of the cancer phenotype and the dissemination of cancer cells to metastatic sites. From our perspective, the involvement of CXCL13:CXCR5 axis in solid tumors deserves to be fully investigated. Many efforts are still required to grasp a better and more conclusive understanding of the fundamentals of this pathway. Due to the great complexities and the wide spectrum of immunological and tumoral responses in different cellular contexts, particularly considering the anti-tumorigenic vs. pro-tumorigenic actions of this pathway and the lack of specific targeting agents, it is not yet feasible to use it for therapeutic intervention in cancer patients. The elucidation of CXCR5 signaling effectors and target genes would help profiling molecular scenarios controlling tumor development and its response to targeted therapies. New discoveries in the CXCL13:CXCR5 field would also aid clinical decision-making for cancer patients, bringing us closer to the promise of translational precision medicine.
MK, MD, and MC participated in the design, writing, and editing of the manuscript, and approved it for publication. MC generated the figures for the article.
This work was supported by grants R01-CA189765 and R01-ES026023 from NIH (MK) and 5T32CA009140 from NCI (MD).
The authors declare that the research was conducted in the absence of any commercial or financial relationships that could be construed as a potential conflict of interest.
1. Murdoch C, Finn A. Chemokine receptors and their role in inflammation and infectious diseases. Blood. (2000) 95:3032–43.
2. Charo IF, Ransohoff RM. The many roles of chemokines and chemokine receptors in inflammation. N Engl J Med. (2006) 354:610–21. doi: 10.1056/NEJMra052723
3. von Hundelshausen P, Agten SM, Eckardt V, Blanchet X, Schmitt MM, Ippel H, et al. Chemokine interactome mapping enables tailored intervention in acute and chronic inflammation. Sci Transl Med. (2017) 9:eaah6650. doi: 10.1126/scitranslmed.aah6650
4. Proost P, Struyf S, Van Damme J, Fiten P, Ugarte-Berzal E, Opdenakker G. Chemokine isoforms and processing in inflammation and immunity. J Autoimmun. (2017) 85:45–57. doi: 10.1016/j.jaut.2017.06.009
5. Stone MJ. Regulation of chemokine-receptor interactions and functions. Int J Mol Sci. (2017) 18:2415. doi: 10.3390/ijms18112415
6. Stone MJ, Hayward JA, Huang C, E Huma Z, Sanchez J. Mechanisms of regulation of the chemokine-receptor network. Int J Mol Sci. (2017) 18:342. doi: 10.3390/ijms18020342
7. Ziarek JJ, Kleist AB, London N, Raveh B, Montpas N, Bonneterre J, et al. Structural basis for chemokine recognition by a G protein-coupled receptor and implications for receptor activation. Sci Signal. (2017) 10:eaah5756. doi: 10.1126/scisignal.aah5756
8. Zlotnik A, Yoshie O. Chemokines: a new classification system and their role in immunity. Immunity. (2000) 12:121–7. doi: 10.1016/S1074-7613(00)80165-X
10. Laing KJ, Secombes CJ. Chemokines. Dev Comp Immunol. (2004) 28:443–60. doi: 10.1016/j.dci.2003.09.006
11. Laing KJ, Secombes CJ. Trout CC chemokines: comparison of their sequences and expression patterns. Mol Immunol. (2004) 41:793–808. doi: 10.1016/j.molimm.2004.03.038
12. Szekanecz Z, Koch AE. Successes and failures of chemokine-pathway targeting in rheumatoid arthritis. Nat Rev Rheumatol. (2016) 12:5–13. doi: 10.1038/nrrheum.2015.157
13. Teran LM. CCL chemokines and asthma. Immunol Today. (2000) 21:235–42. doi: 10.1016/S0167-5699(00)01634-0
14. Lima XT, Oliveira RT, Braga FG, Magalhães RF, Mamoni RL, Blotta MH. Circulating levels of chemokines in psoriasis. Autoimmunity. (2015) 48:57–60. doi: 10.3109/08916934.2014.947476
15. Balkwill FR. The chemokine system and cancer. J Pathol. (2012) 226:148–157. doi: 10.1002/path.3029
16. Balkwill F. Cancer and the chemokine network. Nat Rev Cancer. (2004) 4:540–50. doi: 10.1038/nrc1388
17. Nagarsheth N, Wicha MS, Zou W. Chemokines in the cancer microenvironment and their relevance in cancer immunotherapy. Nat Rev Immunol. (2017) 17:559–72. doi: 10.1038/nri.2017.49
18. Karin N. Chemokines and cancer: new immune checkpoints for cancer therapy. Curr Opin Immunol. (2018) 51:140–5. doi: 10.1016/j.coi.2018.03.004
19. Lazennec G, Richmond A. Chemokines and chemokine receptors: new insights into cancer-related inflammation. Trends Mol Med. (2010) 16:133–44. doi: 10.1016/j.molmed.2010.01.003
20. Altman LC, Snyderman R, Oppenheim JJ, Mergenhagen SE. A human mononuclear leukocyte chemotactic factor: characterization, specificity and kinetics of production by homologous leukocytes. J Immunol. (1973) 110:801–10.
21. Goetzl EJ, Austen KF. Purification and synthesis of eosinophilotactic tetrapeptides of human lung tissue: identification as eosinophil chemotactic factor of anaphylaxis. Proc Natl Acad Sci USA. (1975) 72:4123–7. doi: 10.1073/pnas.72.10.4123
22. Yoshimura T, Matsushima K, Oppenheim JJ, Leonard EJ. Neutrophil chemotactic factor produced by lipopolysaccharide (LPS)-stimulated human blood mononuclear leukocytes: partial characterization and separation from interleukin 1 (IL 1). J Immunol. (1987) 139:788–93.
23. Schall TJ, Bacon K, Toy KJ, Goeddel DV. Selective attraction of monocytes and T lymphocytes of the memory phenotype by cytokine RANTES. Nature. (1990) 347:669–71. doi: 10.1038/347669a0
24. Dobner T, Wolf I, Emrich T, Lipp M. Differentiation-specific expression of a novel G protein-coupled receptor from Burkitt's lymphoma. Eur J Immunol. (1992) 22:2795–9. doi: 10.1002/eji.1830221107
25. Kaiser E, Förster R, Wolf I, Ebensperger C, Kuehl WM, Lipp M. The G protein-coupled receptor BLR1 is involved in murine B cell differentiation and is also expressed in neuronal tissues. Eur J Immunol. (1993) 23:2532–9. doi: 10.1002/eji.1830231023
26. Förster R, Mattis AE, Kremmer E, Wolf E, Brem G, Lipp M. A putative chemokine receptor, BLR1, directs B cell migration to defined lymphoid organs and specific anatomic compartments of the spleen. Cell. (1996) 87:1037–47. doi: 10.1016/S0092-8674(00)81798-5
27. Legler DF, Loetscher M, Roos RS, Clark-Lewis I, Baggiolini M, Moser B. B cell-attracting chemokine. 1, a human CXC chemokine expressed in lymphoid tissues, selectively attracts B lymphocytes via BLR1/CXCR5. J Exp Med. (1998) 187:655–60. doi: 10.1084/jem.187.4.655
28. Gunn MD, Ngo VN, Ansel KM, Ekland EH, Cyster JG, Williams LT. A B-cell-homing chemokine made in lymphoid follicles activates Burkitt's lymphoma receptor-1. Nature. (1998) 391:799–803. doi: 10.1038/35876
29. Bièche I, Chavey C, Andrieu C, Busson M, Vacher S, Le Corre L, et al. CXC chemokines located in the 4q21 region are up-regulated in breast cancer. Endocr Relat Cancer. (2007) 14:1039–52. doi: 10.1677/erc.1.01301
30. Barella L, Loetscher M, Tobler A, Baggiolini M, Moser B. Sequence variation of a novel heptahelical leucocyte receptor through alternative transcript formation. Biochem J. (1995) 309:773–9. doi: 10.1042/bj3090773
31. Müller G, Lipp M. Signal transduction by the chemokine receptor CXCR5: structural requirements for G protein activation analyzed by chimeric CXCR1/CXCR5 molecules. Biol Chem. (2001) 382:1387–97. doi: 10.1515/BC.2001.171
32. Damaj BB, McColl SR, Mahana W, Crouch MF, Naccache PH. Physical association of Gi2alpha with interleukin-8 receptors. J Biol Chem. (1996) 271:12783–9. doi: 10.1074/jbc.271.22.12783
33. Guigou V, Emilie D, Berrih-Aknin S, Fumoux F, Fougereau M, Schiff C. Individual germinal centres of myasthenia gravis human thymuses contain polyclonal activated B cells that express all the Vh and Vk families. Clin Exp Immunol. (1991) 83:262–6. doi: 10.1111/j.1365-2249.1991.tb05625.x
34. Schröder AE, Greiner A, Seyfert C, Berek C. Differentiation of B cells in the nonlymphoid tissue of the synovial membrane of patients with rheumatoid arthritis. Proc Natl Acad Sci USA. (1996) 93:221–5. doi: 10.1073/pnas.93.1.221
35. Shi K, Hayashida K, Kaneko M, Hashimoto J, Tomita T, Lipsky PE, et al. Lymphoid chemokine B cell-attracting chemokine-1 (CXCL13) is expressed in germinal center of ectopic lymphoid follicles within the synovium of chronic arthritis patients. J Immunol. (2001) 166:650–5. doi: 10.4049/jimmunol.166.1.650
36. Takemura S, Klimiuk PA, Braun A, Goronzy JJ, Weyand CM. T cell activation in rheumatoid synovium is B cell dependent. J Immunol. (2001) 167:4710–8. doi: 10.4049/jimmunol.167.8.4710
37. Amft N, Curnow SJ, Scheel-Toellner D, Devadas A, Oates J, Crocker J, et al. Ectopic expression of the B cell-attracting chemokine BCA-1 (CXCL13) on endothelial cells and within lymphoid follicles contributes to the establishment of germinal center-like structures in Sjogren's syndrome. Arthritis Rheum. (2001) 44:2633–41. doi: 10.1002/1529-0131(200111)44:11<2633::AID-ART443>3.0.CO;2-9
38. Salomonsson S, Larsson P, Tengnér P, Mellquist E, Hjelmström P, Wahren-Herlenius M. Expression of the B cell-attracting chemokine CXCL13 in the target organ and autoantibody production in ectopic lymphoid tissue in the chronic inflammatory disease Sjogren's syndrome. Scand J Immunol. (2002) 55:336–42. doi: 10.1046/j.1365-3083.2002.01058.x
39. Aust G, Sittig D, Becherer L, Anderegg U, Schütz A, Lamesch P, et al. The role of CXCR5 and its ligand CXCL13 in the compartmentalization of lymphocytes in thyroids affected by autoimmune thyroid diseases. Eur J Endocrinol. (2004) 150:225–34. doi: 10.1530/eje.0.1500225
40. Meraouna A, Cizeron-Clairac G, Panse RL, Bismuth J, Truffault F, Tallaksen C, et al. The chemokine CXCL13 is a key molecule in autoimmune myasthenia gravis. Blood. (2006) 108:432–40. doi: 10.1182/blood-2005-06-2383
41. Lee HT, Shiao YM, Wu TH, Chen WS, Hsu YH, Tsai SF, et al. Serum BLC/CXCL13 concentrations and renal expression of CXCL13/CXCR5 in patients with systemic lupus erythematosus and lupus nephritis. J Rheumatol. (2010) 37:45–52. doi: 10.3899/jrheum.090450
42. Corcione A, Casazza S, Ferretti E, Giunti D, Zappia E, Pistorio A, et al. Recapitulation of B cell differentiation in the central nervous system of patients with multiple sclerosis. Proc Natl Acad Sci USA. (2004) 101:11064–9. doi: 10.1073/pnas.0402455101
43. Lee KE, Kang JH, Yim YR, Kim JE, Lee JW, Wen L, et al. Predictive significance of CCL21 and CXCL13 levels in the minor salivary glands of patients with Sjogren's syndrome. Clin Exp Rheumatol. (2017) 35:234–40.
44. Kramer JM, Klimatcheva E, Rothstein TL. CXCL13 is elevated in Sjogren's syndrome in mice and humans and is implicated in disease pathogenesis. J Leukoc Biol. (2013) 94:1079–89. doi: 10.1189/jlb.0113036
45. Moser B. CXCR5, the Defining Marker for Follicular B Helper T (TFH) Cells. Front Immunol. (2015) 6:296. doi: 10.3389/fimmu.2015.00296
46. Crotty S. brief history of T cell help to B cells. Nat Rev Immunol. (2015) 15:185–9. doi: 10.1038/nri3803
47. Ma JJ, Jiang L, Tong DY, Ren YN, Sheng MF, Liu HC. CXCL13 inhibition induce the apoptosis of MDA-MB-231 breast cancer cells through blocking CXCR5/ERK signaling pathway. Eur Rev Med Pharmacol Sci. (2018) 22:8755–62. doi: 10.26355/eurrev_201812_16641
48. Subramanian S, Tus K, Li QZ, Wang A, Tian XH, Zhou J, et al. A Tlr7 translocation accelerates systemic autoimmunity in murine lupus. Proc Natl Acad Sci USA. (2006) 103:9970–5. doi: 10.1073/pnas.0603912103
49. Linterman MA, Rigby RJ, Wong RK, Yu D, Brink R, Cannons JL, et al. Follicular helper T cells are required for systemic autoimmunity. J Exp Med. (2009) 206:561–76. doi: 10.1084/jem.20081886
50. Szabó K, Papp G, Szántó A, Tarr T, Zeher M. A comprehensive investigation on the distribution of circulating follicular T helper cells and B cell subsets in primary Sjogren's syndrome and systemic lupus erythematosus. Clin Exp Immunol. (2016) 183:76–89. doi: 10.1111/cei.12703
51. Adam L, Zoldan K, Hofmann M, Schultheiss M, Bettinger D, Neumann-Haefelin C, et al. Follicular T helper cell signatures in primary biliary cholangitis and primary sclerosing cholangitis. Hepatol Commun. (2018) 2:1051–63. doi: 10.1002/hep4.1226
52. Zhao S, Fang F, Tang X, Dou J, Wang W, Zheng X, et al. An in-depth analysis identifies two new independent signals in. (2017) 11q23.3 associated with vitiligo in the Chinese Han population. J Dermatol Sci 88:103–9. doi: 10.1016/j.jdermsci.2017.05.001
53. Long S, Ma L, Wang D, Shang X. High frequency of circulating follicular helper T cells is correlated with B cell subtypes in patients with ankylosing spondylitis. Exp Ther Med. (2018) 15:4578–86. doi: 10.3892/etm.2018.5991
54. Yuan H, Zhou S, Liu Z, Cong W, Fei X, Zeng W, et al. Pivotal role of lesional and perilesional T/B lymphocytes in pemphigus pathogenesis. J Invest Dermatol. (2017) 137:2362–70. doi: 10.1016/j.jid.2017.05.032
55. Cagigi A, Mowafi F, Phuong Dang LV, Tenner-Racz K, Atlas A, Grutzmeier S, et al. Altered expression of the receptor-ligand pair CXCR5/CXCL13 in B cells during chronic HIV-1 infection. Blood. (2008) 112:4401–10. doi: 10.1182/blood-2008-02-140426
56. Widney DP, Breen EC, Boscardin WJ, Kitchen SG, Alcantar JM, Smith JB, et al. Serum levels of the homeostatic B cell chemokine, CXCL13, are elevated during HIV infection. J Interferon Cytokine Res. (2005) 25:702–6. doi: 10.1089/jir.2005.25.702
57. Lindqvist M, van Lunzen J, Soghoian DZ, Kuhl BD, Ranasinghe S, Kranias G, et al. Expansion of HIV-specific T follicular helper cells in chronic HIV infection. J Clin Invest. (2012) 122:3271–80. doi: 10.1172/JCI64314
58. Perreau M, Savoye AL, De Crignis E, Corpataux JM, Cubas R, Haddad EK, et al. Follicular helper T cells serve as the major CD4 T cell compartment for HIV-1 infection, replication, and production. J Exp Med. (2013) 210:143–56. doi: 10.1084/jem.20121932
59. Cohen K, Altfeld M, Alter G, Stamatatos L. Early preservation of CXCR5+ PD-1+ helper T cells and B cell activation predict the breadth of neutralizing antibody responses in chronic HIV-1 infection. J Virol. (2014) 88:13310–21. doi: 10.1128/JVI.02186-14
60. Mehraj V, Ramendra R, Isnard S, Dupuy FP, Lebouché B, Costiniuk C, et al. CXCL13 as a biomarker of immune activation during early and chronic HIV infection. Front Immunol. (2019) 10:289. doi: 10.3389/fimmu.2019.00289
61. Schmidt C, Plate A, Angele B, Pfister HW, Wick M, Koedel U, et al. A prospective study on the role of CXCL13 in Lyme neuroborreliosis. Neurology. (2011) 76:1051–8. doi: 10.1212/WNL.0b013e318211c39a
62. Rupprecht TA, Manz KM, Fingerle V, Lechner C, Klein M, Pfirrmann M, et al. Diagnostic value of cerebrospinal fluid CXCL13 for acute Lyme neuroborreliosis. A systematic review and meta-analysis. Clin Microbiol Infect. (2018) 24:1234–40. doi: 10.1016/j.cmi.2018.04.007
63. Yu Q, Cheng Y, Wang Y, Wang C, Lu H, Guan Z, et al. Aberrant humoral immune responses in neurosyphilis: CXCL13/CXCR5 play a pivotal role for B-cell recruitment to the cerebrospinal fluid. J Infect Dis. (2017) 216:534–44. doi: 10.1093/infdis/jix233
64. Wang C, Wu K, Yu Q, Zhang S, Gao Z, Liu Y, et al. CXCL13, CXCL10 and CXCL8 as potential biomarkers for the diagnosis of neurosyphilis patients. Sci Rep. (2016) 6:33569. doi: 10.1038/srep33569
65. Mothapo KM, Verbeek MM, van der Velden LB, Ang CW, Koopmans PP, van der Ven A, et al. Has CXCL13 an added value in diagnosis of neurosyphilis? J Clin Microbiol. (2015) 53:1693–6. doi: 10.1128/JCM.02917-14
66. Mazzucchelli L, Blaser A, Kappeler A, Schärli P, Laissue JA, Baggiolini M, et al. BCA-1 is highly expressed in Helicobacter pylori-induced mucosa-associated lymphoid tissue and gastric lymphoma. J Clin Invest. (1999) 104:R49–54. doi: 10.1172/JCI7830
67. Husson H, Freedman AS, Cardoso AA, Schultze J, Munoz O, Strola G, et al. CXCL13 (BCA-1) is produced by follicular lymphoma cells: role in the accumulation of malignant B cells. Br J Haematol. (2002) 119:492–5. doi: 10.1046/j.1365-2141.2002.03832.x
68. Smith JR, Braziel RM, Paoletti S, Lipp M, Uguccioni M, Rosenbaum JT. Expression of B-cell-attracting chemokine 1 (CXCL13) by malignant lymphocytes and vascular endothelium in primary central nervous system lymphoma. Blood. (2003) 101:815–21. doi: 10.1182/blood-2002-05-1576
69. Bürkle A, Niedermeier M, Schmitt-Gräff A, Wierda WG, Keating MJ, Burger JA. Overexpression of the CXCR5 chemokine receptor, and its ligand, CXCL13 in B-cell chronic lymphocytic leukemia. Blood. (2007) 110:3316–25. doi: 10.1182/blood-2007-05-089409
70. Dupuis J, Boye K, Martin N, Copie-Bergman C, Plonquet A, Fabiani B, et al. Expression of CXCL13 by neoplastic cells in angioimmunoblastic T-cell lymphoma (AITL): a new diagnostic marker providing evidence that AITL derives from follicular helper T cells. Am J Surg Pathol. (2006) 30:490–4. doi: 10.1097/00000478-200604000-00009
71. Swerdlow SH, Campo E, Pileri SA, Harris NL, Stein H, Siebert R, et al. The 2016 revision of the World Health Organization classification of lymphoid neoplasms. Blood. (2016) 127:2375–90. doi: 10.1182/blood-2016-01-643569
72. Fischer L, Korfel A, Pfeiffer S, Kiewe P, Volk HD, Cakiroglu H, et al. CXCL13 and CXCL12 in central nervous system lymphoma patients. Clin Cancer Res. (2009) 15:5968–73. doi: 10.1158/1078-0432.CCR-09-0108
73. Rubenstein JL, Wong VS, Kadoch C, Gao HX, Barajas R, Chen L, et al. CXCL13 plus interleukin 10 is highly specific for the diagnosis of CNS lymphoma. Blood. (2013) 121:4740–8. doi: 10.1182/blood-2013-01-476333
74. Pierson SK, Stonestrom AJ, Shilling D, Ruth J, Nabel CS, Singh A, et al. Plasma proteomics identifies a 'chemokine storm' in idiopathic multicentric Castleman disease. Am J Hematol. (2018) 93:902–12. doi: 10.1002/ajh.25123
75. Jones GW, Hill DG, Jones SA. Understanding immune cells in tertiary lymphoid organ development: it is all starting to come together. Front Immunol. (2016) 7:401. doi: 10.3389/fimmu.2016.00401
76. Jones GW, Jones SA. Ectopic lymphoid follicles: inducible centres for generating antigen-specific immune responses within tissues. Immunology. (2016) 147:141–51. doi: 10.1111/imm.12554
77. Germain C, Gnjatic S, Tamzalit F, Knockaert S, Remark R, Goc J, et al. Presence of B cells in tertiary lymphoid structures is associated with a protective immunity in patients with lung cancer. Am J Respir Crit Care Med. (2014) 189:832–44. doi: 10.1164/rccm.201309-1611OC
78. Dieu-Nosjean MC, Goc J, Giraldo NA, Sautès-Fridman C, Fridman WH. Tertiary lymphoid structures in cancer and beyond. Trends Immunol. (2014) 35:571–80. doi: 10.1016/j.it.2014.09.006
79. Fridman WH, Pagès F, Sautès-Fridman C, Galon J. The immune contexture in human tumours: impact on clinical outcome. Nat Rev Cancer. (2012) 12:298–306. doi: 10.1038/nrc3245
80. Pitzalis C, Jones GW, Bombardieri M, Jones SA. Ectopic lymphoid-like structures in infection, cancer and autoimmunity. Nat Rev Immunol. (2014) 14:447–62. doi: 10.1038/nri3700
81. Dieu-Nosjean MC, Giraldo NA, Kaplon H, Germain C, Fridman WH, Sautès-Fridman C. Tertiary lymphoid structures, drivers of the anti-tumor responses in human cancers. Immunol Rev. (2016) 271:260–75. doi: 10.1111/imr.12405
82. Tokunaga R, Naseem M, Lo JH, Battaglin F, Soni S, Puccini A, et al. B cell and B cell-related pathways for novel cancer treatments. Cancer Treat Rev. (2019) 73:10–9. doi: 10.1016/j.ctrv.2018.12.001
83. Crotty S. T follicular helper cell biology: a decade of discovery and diseases. Immunity. (2019) 50:1132–48. doi: 10.1016/j.immuni.2019.04.011
84. Sun Y, Wang L, Jiang M, Huang J, Liu Z, Wolfl S. Secreted phosphoprotein 1 upstream invasive network construction and analysis of lung adenocarcinoma compared with human normal adjacent tissues by integrative biocomputation. Cell Biochem Biophys. (2010) 56:59–71. doi: 10.1007/s12013-009-9071-6
85. Allen IC, Wilson JE, Schneider M, Lich JD, Roberts RA, Arthur JC, et al. NLRP12 suppresses colon inflammation and tumorigenesis through the negative regulation of noncanonical NF-kappaB signaling. Immunity. (2012) 36:742–54. doi: 10.1016/j.immuni.2012.03.012
86. Shiels MS, Pfeiffer RM, Hildesheim A, Engels EA, Kemp TJ, Park JH, et al. Circulating inflammation markers and prospective risk for lung cancer. J Natl Cancer Inst. (2013) 105:1871–80. doi: 10.1093/jnci/djt309
87. Eide HA, Halvorsen AR, Sandhu V, Fåne A, Berg J, Haakensen VD, et al. Non-small cell lung cancer is characterised by a distinct inflammatory signature in serum compared with chronic obstructive pulmonary disease. Clin Transl Immunol. (2016) 5:e109. doi: 10.1038/cti.2016.65
88. Zhang Y, Yu K, Hu S, Lou Y, Liu C, Xu J, et al. MDC and BLC are independently associated with the significant risk of early stage lung adenocarcinoma. Oncotarget. (2016) 7:83051–9. doi: 10.18632/oncotarget.13031
89. de Chaisemartin L, Goc J, Damotte D, Validire P, Magdeleinat P, Alifano M, et al. Characterization of chemokines and adhesion molecules associated with T cell presence in tertiary lymphoid structures in human lung cancer. Cancer Res. (2011) 71:6391–9. doi: 10.1158/0008-5472.CAN-11-0952
90. Silina K, Soltermann A, Attar FM, Casanova R, Uckeley ZM, Thut H, et al. Germinal centers determine the prognostic relevance of tertiary lymphoid structures and are impaired by corticosteroids in lung squamous cell carcinoma. Cancer Res. (2018) 78:1308–20. doi: 10.1158/0008-5472.CAN-17-1987
91. Thommen DS, Koelzer VH, Herzig P, Roller A, Trefny M, Dimeloe S, et al. A transcriptionally and functionally distinct PD-1(+) CD8(+) T cell pool with predictive potential in non-small-cell lung cancer treated with PD-1 blockade. Nat Med. (2018) 24:994–1004. doi: 10.1038/s41591-018-0057-z
92. Wang GZ, Cheng X, Zhou B, Wen ZS, Huang YC, Chen HB, et al. The chemokine CXCL13 in lung cancers associated with environmental polycyclic aromatic hydrocarbons pollution. Elife. (2015) 4:e09419. doi: 10.7554/eLife.09419
93. Singh R, Gupta P, Kloecker GH, Singh S, Lillard JW. Expression and clinical significance of CXCR5/CXCL13 in human nonsmall cell lung carcinoma. Int J Oncol. (2014) 45:2232–40. doi: 10.3892/ijo.2014.2688
94. Panse J, Friedrichs K, Marx A, Hildebrandt Y, Luetkens T, Barrels K, et al. Chemokine CXCL13 is overexpressed in the tumour tissue and in the peripheral blood of breast cancer patients. Br J Cancer. (2008) 99:930–8. doi: 10.1038/sj.bjc.6604621
95. Biswas S, Roy Chowdhury S, Mandal G, Purohit S, Gupta A, Bhattacharyya A. RelA driven co-expression of CXCL13 and CXCR5 is governed by a multifaceted transcriptional program regulating breast cancer progression. Biochim Biophys Acta Mol Basis Dis. (2019) 1865:502–11. doi: 10.1016/j.bbadis.2018.12.002
96. Chen L, Huang Z, Yao G, Lyu X, Li J, Hu X, et al. The expression of CXCL13 and its relation to unfavorable clinical characteristics in young breast cancer. J Transl Med. (2015) 13:168. doi: 10.1186/s12967-015-0521-1
97. Narita D, Seclaman E, Anghel A, Ilina R, Cireap N, Negru S, et al. Altered levels of plasma chemokines in breast cancer and their association with clinical and pathological characteristics. Neoplasma. (2016) 63:141–9. doi: 10.4149/neo_2016_017
98. Yau C, Esserman L, Moore DH, Waldman F, Sninsky J, Benz CC. A multigene predictor of metastatic outcome in early stage hormone receptor-negative and triple-negative breast cancer. Breast Cancer Res. (2010) 12:R85. doi: 10.1186/bcr2753
99. Heimes AS, Madjar K, Edlund K, Battista MJ, Almstedt K, Elger T, et al. Subtype-specific prognostic impact of different immune signatures in node-negative breast cancer. Breast Cancer Res Treat. (2017) 165:293–300. doi: 10.1007/s10549-017-4327-0
100. Criscitiello C, Bayar MA, Curigliano G, Symmans FW, Desmedt C, Bonnefoi H, et al. A gene signature to predict high tumor-infiltrating lymphocytes after neoadjuvant chemotherapy and outcome in patients with triple-negative breast cancer. Ann Oncol. (2018) 29:162–9. doi: 10.1093/annonc/mdx691
101. Schmidt M, Weyer-Elberich V, Hengstler JG, Heimes AS, Almstedt K, Gerhold-Ay A, et al. Prognostic impact of CD4-positive T cell subsets in early breast cancer: a study based on the FinHer trial patient population. Breast Cancer Res. (2018) 20:15. doi: 10.1186/s13058-018-0942-x
102. Xu L, Liang Z, Li S, Ma J. Signaling via the CXCR5/ERK pathway is mediated by CXCL13 in mice with breast cancer. Oncol Lett. (2018) 15:9293–8. doi: 10.3892/ol.2018.8510
103. Mitkin NA, Hook CD, Schwartz AM, Biswas S, Kochetkov DV, Muratova AM, et al. p53-dependent expression of CXCR5 chemokine receptor in MCF-7 breast cancer cells. Sci Rep. (2015) 5:9330. doi: 10.1038/srep09330
104. Mitkin NA, Muratova AM, Sharonov GV, Korneev KV, Sviriaeva EN, Mazurov D, et al. p63 and p73 repress CXCR5 chemokine receptor gene expression in p53-deficient MCF-7 breast cancer cells during genotoxic stress. Biochim Biophys Acta Gene Regul Mech. (2017) 1860:1169–78. doi: 10.1016/j.bbagrm.2017.10.003
105. Olsen RS, Nijm J, Andersson RE, Dimberg J, Wågsäter D. Circulating inflammatory factors associated with worse long-term prognosis in colorectal cancer. World J Gastroenterol. (2017) 23:6212–9. doi: 10.3748/wjg.v23.i34.6212
106. Qi XW, Xia SH, Yin Y, Jin LF, Pu Y, Hua D, et al. Expression features of CXCR5 and its ligand, CXCL13 associated with poor prognosis of advanced colorectal cancer. Eur Rev Med Pharmacol Sci. (2014) 18:1916–24.
107. Meijer J, Zeelenberg IS, Sipos B, Roos E. The CXCR5 chemokine receptor is expressed by carcinoma cells and promotes growth of colon carcinoma in the liver. Cancer Res. (2006) 66:9576–82. doi: 10.1158/0008-5472.CAN-06-1507
108. Zhu Z, Zhang X, Guo H, Fu L, Pan G, Sun Y. CXCL13-CXCR5 axis promotes the growth and invasion of colon cancer cells via PI3K/AKT pathway. Mol Cell Biochem. (2015) 400:287–95. doi: 10.1007/s11010-014-2285-y
109. Sun Y, Tian H, Wang L. Effects of PTEN on the proliferation and apoptosis of colorectal cancer cells via the phosphoinositol-3-kinase/Akt pathway. Oncol Rep. (2015) 33:1828–36. doi: 10.3892/or.2015.3804
110. Bindea G, Mlecnik B, Tosolini M, Kirilovsky A, Waldner M, Obenauf AC, et al. Spatiotemporal dynamics of intratumoral immune cells reveal the immune landscape in human cancer. Immunity. (2013) 39:782–95. doi: 10.1016/j.immuni.2013.10.003
111. Wei Y, Lin C, Li H, Xu Z, Wang J, Li R, et al. CXCL13 expression is prognostic and predictive for postoperative adjuvant chemotherapy benefit in patients with gastric cancer. Cancer Immunol Immunother. (2018) 67:261–9. doi: 10.1007/s00262-017-2083-y
112. Hofman VJ, Moreilhon C, Brest PD, Lassalle S, Le Brigand K, Sicard D, et al. Gene expression profiling in human gastric mucosa infected with Helicobacter pylori. Mod Pathol. (2007) 20:974–89. doi: 10.1038/modpathol.3800930
113. Galamb O, Gyõrffy B, Sipos F, Dinya E, Krenács T, Berczi L, et al. Helicobacter pylori and antrum erosion-specific gene expression patterns: the discriminative role of CXCL13 and VCAM1 transcripts. Helicobacter. (2008) 13:112–26. doi: 10.1111/j.1523-5378.2008.00584.x
114. Meng X, Yu X, Dong Q, Xu X, Li J, Xu Q, et al. Distribution of circulating follicular helper T cells and expression of interleukin-21 and chemokine C-X-C ligand 13 in gastric cancer. Oncol Lett. (2018) 16:3917–22. doi: 10.3892/ol.2018.9112
115. Wharry CE, Haines KM, Carroll RG, May MJ. Constitutive non-canonical NFkappaB signaling in pancreatic cancer cells. Cancer Biol Ther. (2009) 8:1567–1576. doi: 10.4161/cbt.8.16.8961
116. Lee KE, Spata M, Bayne LJ, Buza EL, Durham AC, Allman D, et al. Hif1a deletion reveals pro-neoplastic function of B cells in pancreatic neoplasia. Cancer Discov. (2016) 6:256–69. doi: 10.1158/2159-8290.CD-15-0822
117. Pylayeva-Gupta Y, Das S, Handler JS, Hajdu CH, Coffre M, Koralov SB, et al. IL35-producing B cells promote the development of pancreatic neoplasia. Cancer Discov. (2016) 6:247–55. doi: 10.1158/2159-8290.CD-15-0843
118. Duan Z, Gao J, Zhang L, Liang H, Huang X, Xu Q, et al. Phenotype and function of CXCR5+CD45RA-CD4+ T cells were altered in HBV-related hepatocellular carcinoma and elevated serum CXCL13 predicted better prognosis. Oncotarget. (2015) 6:44239–53. doi: 10.18632/oncotarget.6235
119. Li C, Kang D, Sun X, Liu Y, Wang J, Gao P. The effect of C-X-C motif chemokine 13 on hepatocellular carcinoma associates with Wnt signaling. Biomed Res Int. (2015) 2015:345413. doi: 10.1155/2015/345413
120. Singh S, Singh R, Sharma PK, Singh UP, Rai SN, Chung LW, et al. Serum CXCL13 positively correlates with prostatic disease, prostate-specific antigen and mediates prostate cancer cell invasion, integrin clustering and cell adhesion. Cancer Lett. (2009) 283:29–35. doi: 10.1016/j.canlet.2009.03.022
121. El Haibi CP, Sharma PK, Singh R, Johnson PR, Suttles J, Singh S, et al.El PI3Kp110-, Src-, FAK-dependent and DOCK2-independent migration and invasion of CXCL13-stimulated prostate cancer cells. Mol Cancer. (2010) 9:85. doi: 10.1186/1476-4598-9-85
122. El-Haibi CP, Singh R, Sharma PK, Singh S, Lillard JW. CXCL13 mediates prostate cancer cell proliferation through JNK signalling and invasion through ERK activation. Cell Prolif. (2011) 44:311–9. doi: 10.1111/j.1365-2184.2011.00757.x
123. Fan L, Zhu Q, Liu L, Zhu C, Huang H, Lu S, et al. CXCL13 is androgen-responsive and involved in androgen induced prostate cancer cell migration and invasion. Oncotarget. (2017) 8:53244–61. doi: 10.18632/oncotarget.18387
124. Meshki J, Caino MC, von Burstin VA, Griner E, Kazanietz MG. Regulation of prostate cancer cell survival by protein kinase Cepsilon involves bad phosphorylation and modulation of the TNFalpha/JNK pathway. J Biol Chem. (2010) 285:26033–40. doi: 10.1074/jbc.M110.128371
125. Benavides F, Blando J, Perez CJ, Garg R, Conti CJ, DiGiovanni J, et al. Transgenic overexpression of PKCepsilon in the mouse prostate induces preneoplastic lesions. Cell Cycle. (2011) 10:268–77. doi: 10.4161/cc.10.2.14469
126. Garg R, Blando J, Perez CJ, Wang H, Benavides FJ, Kazanietz MG. Activation of nuclear factor kappaB (NF-kappaB) in prostate cancer is mediated by protein kinase C epsilon (PKCepsilon). J Biol Chem. (2012) 287:37570–82. doi: 10.1074/jbc.M112.398925
127. Gutierrez-Uzquiza A, Lopez-Haber C, Jernigan DL, Fatatis A, Kazanietz MG. PKCepsilon Is an Essential Mediator of Prostate Cancer Bone Metastasis. Mol Cancer Res. (2015) 13:1336–46. doi: 10.1158/1541-7786.MCR-15-0111
128. Garg R, Blando JM, Perez CJ, Abba MC, Benavides F, Kazanietz MG. Protein kinase C epsilon cooperates with PTEN loss for prostate tumorigenesis through the CXCL13-CXCR5 pathway. Cell Rep. (2017) 19:375–88. doi: 10.1016/j.celrep.2017.03.042
129. Garg R, Blando JM, Perez CJ, Lal P, Feldman MD, Smyth EM, et al. COX-2 mediates pro-tumorigenic effects of PKCepsilon in prostate cancer. Oncogene. (2018) 37:4735–49. doi: 10.1038/s41388-018-0318-9
130. Ammirante M, Shalapour S, Kang Y, Jamieson CA, Karin M. Tissue injury and hypoxia promote malignant progression of prostate cancer by inducing CXCL13 expression in tumor myofibroblasts. Proc Natl Acad Sci USA. (2014) 111:14776–81. doi: 10.1073/pnas.1416498111
131. Sorrentino C, Ciummo SL, Cipollone G, Caputo S, Bellone M, Di Carlo E. Interleukin-30/IL27p28 shapes prostate cancer stem-like cell behavior and is critical for tumor onset and metastasization. Cancer Res. (2018) 78:2654–68. doi: 10.1158/0008-5472.CAN-17-3117
132. Zheng Z, Cai Y, Chen H, Chen Z, Zhu D, Zhong Q, et al. CXCL13/CXCR5 axis predicts poor prognosis and promotes progression through PI3K/AKT/mTOR Pathway in Clear Cell Renal Cell Carcinoma. Front Oncol. (2018) 8:682. doi: 10.3389/fonc.2018.00682
133. Yuvaraj S, Griffin AC, Sundaram K, Kirkwood KL, Norris JS, Reddy SV. A novel function of CXCL13 to stimulate RANK ligand expression in oral squamous cell carcinoma cells. Mol Cancer Res. (2009) 7:1399–407. doi: 10.1158/1541-7786.MCR-08-0589
134. Airoldi I, Cocco C, Morandi F, Prigione I, Pistoia V. CXCR5 may be involved in the attraction of human metastatic neuroblastoma cells to the bone marrow. Cancer Immunol Immunother. (2008) 57:541–8. doi: 10.1007/s00262-007-0392-2
135. Zhou Y, Guo L, Sun H, Xu J, Ba T. CXCR5(+) CD8 T cells displayed higher activation potential despite high PD-1 expression, in tumor-involved lymph nodes from patients with thyroid cancer. Int Immunopharmacol. (2018) 62:114–9. doi: 10.1016/j.intimp.2018.07.002
136. Xiao H, Luo G, Son H, Zhou Y, Zheng W. Upregulation of peripheral CD4+CXCR5+ T cells in osteosarcoma. Tumour Biol. (2014) 35:5273–9. doi: 10.1007/s13277-014-1686-6
137. Ignacio RMC, Lee ES, Wilson AJ, Beeghly-Fadiel A, Whalen MM, Son D-S. Chemokine network and overall survival in TP53 wild-type and mutant ovarian cancer. Immune Netw. (2018) 18:e29. doi: 10.4110/in.2018.18.e29
138. Ignacio RMC, Lee ES, Wilson AJ, Beeghly-Fadiel A, Whalen MM, Son DS. Obesity-induced peritoneal dissemination of ovarian cancer and dominant recruitment of macrophages in ascites. Immune Netw. (2018) 18:e47. doi: 10.4110/in.2018.18.e47
139. Sun W, Qiu Z, Huang W, Cao M. Gene expression profiles and proteinprotein interaction networks during tongue carcinogenesis in the tumor microenvironment. Mol Med Rep. (2018) 17:165–71. doi: 10.3892/mmr.2017.7843
140. Sambandam Y, Sundaram K, Liu A, Kirkwood KL, Ries WL, Reddy SV. CXCL13 activation of c-Myc induces RANK ligand expression in stromal/preosteoblast cells in the oral squamous cell carcinoma tumor-bone microenvironment. Oncogene. (2013) 32:97–105. doi: 10.1038/onc.2012.24
141. Pandruvada SN, Yuvaraj S, Liu X, Sundaram K, Shanmugarajan S, Ries WL, et al. Role of CXC chemokine ligand. 13 in oral squamous cell carcinoma associated osteolysis in athymic mice. Int J Cancer. (2010) 126:2319–29. doi: 10.1002/ijc.24920
142. Liu W, Peng Y, Tobin DJ. A new 12-gene diagnostic biomarker signature of melanoma revealed by integrated microarray analysis. Peer J. (2013) 1:e49. doi: 10.7717/peerj.49
Keywords: CXCL13, CXCR5, inflammation, immune responses, cancer progression
Citation: Kazanietz MG, Durando M and Cooke M (2019) CXCL13 and Its Receptor CXCR5 in Cancer: Inflammation, Immune Response, and Beyond. Front. Endocrinol. 10:471. doi: 10.3389/fendo.2019.00471
Received: 03 June 2019; Accepted: 28 June 2019;
Published: 12 July 2019.
Edited by:
Rosa P. Gomariz, Complutense University of Madrid, SpainReviewed by:
Catherine Sautes-Fridman, INSERM U1138 Centre de Recherche des Cordeliers, FranceCopyright © 2019 Kazanietz, Durando and Cooke. This is an open-access article distributed under the terms of the Creative Commons Attribution License (CC BY). The use, distribution or reproduction in other forums is permitted, provided the original author(s) and the copyright owner(s) are credited and that the original publication in this journal is cited, in accordance with accepted academic practice. No use, distribution or reproduction is permitted which does not comply with these terms.
*Correspondence: Mariana Cooke, bWFyY29va2VAcGVubm1lZGljaW5lLnVwZW5uLmVkdQ==
Disclaimer: All claims expressed in this article are solely those of the authors and do not necessarily represent those of their affiliated organizations, or those of the publisher, the editors and the reviewers. Any product that may be evaluated in this article or claim that may be made by its manufacturer is not guaranteed or endorsed by the publisher.
Research integrity at Frontiers
Learn more about the work of our research integrity team to safeguard the quality of each article we publish.