- 1Department of Animal Biotechnology, Faculty of Agriculture and Veterinary, University of Prishtina, Pristina, Kosovo
- 2Animal Physiology and Immunology Weihenstephan, Technical University of Munich, Munich, Germany
- 3Department of Veterinary Sciences, Ludwig Maximilian University of Munich, Munich, Germany
The aim of this study was to characterize the regulation pattern of prostaglandin family members namely prostaglandin F2alpha (PTGF), prostaglandin E2 (PTGE), their receptors (PTGFR, PTGER2, PTGER4), cyclooxygenase 2 (COX-2), PTGF synthase (PTGFS), and PTGE synthase (PTGES) in the bovine follicles during preovulatory period and early corpus luteum (CL). Ovaries containing preovulatory follicles or CL were collected by transvaginal ovariectomy (n = 5 cows/group), and the follicles were classified: (I) before GnRH treatment; (II) 4 h after GnRH; (III) 10 h after GnRH; (IV) 20 h after GnRH; (V) 25 h after GnRH, and (VI) 60 h after GnRH (early CL). In these samples, the concentrations of progesterone (P4), estradiol (E2), PTGF and PTGE were investigated in the follicular fluid (FF) by validated EIA. Relative mRNA abundance of genes encoding for prostaglandin receptors (PTGFR, PTGER2, PTGER4), COX-2, PTGFS and PTGES were quantified by RT-qPCR. The localization of COX-2 and PTGES were investigated by established immunohistochemistry in fixed follicular and CL tissue samples. The high E2 concentration in the FF of the follicle group before GnRH treatment (495.8 ng/ml) and during luteinizing hormone (LH) surge (4 h after GnRH, 574.36 ng/ml), is followed by a significant (P<0.05) downregulation afterwards with the lowest level during ovulation (25 h after GnRH, 53.11 ng/ml). In contrast the concentration of P4 was very low before LH surge (50.64 mg/ml) followed by a significant upregulation (P < 0.05) during ovulation (537.18 ng/ml). The mRNA expression of COX-2 increased significantely (P < 0.05) 4 h after GnRH and again 20 h after GnRH, followed by a significant decrease (P < 0.05) after ovulation (early CL). The mRNA of PTGFS in follicles before GnRH was high followed by a continuous and significant downregulation (P < 0.05) afterwards. In contrast, PTGES mRNA abundance increased significantely (P < 0.05) in follicles 20 h after GnRH treatment and remained high afterwards. The mRNA abundance of PTGFR, PTGER2, and PTGER4 in follicles before GnRH was high, followed by a continuous and significant down regulation afterwards and significant increase (P < 0.05) only after ovulation (early CL). The low concentration of PTGF (0.04 ng/ml) and PTGE (0.15 ng/ml) in FF before GnRH, increased continuously in follicle groups before ovulation and displayed a further significant and dramatic increase (P < 0.05) around ovulation (101.01 ng/ml, respectively, 484.21 ng/ml). Immunohistochemically, the granulosa cells showed an intensive signal for COX-2 and PTGES in follicles during preovulation and in granulosa-luteal cells of the early CL. In conclusion, our results indicate that the examined bovine prostaglandin family members are involved in the local mechanisms regulating final follicle maturation and ovulation during the folliculo-luteal transition and CL formation.
Introduction
The ovarian cycle in bovine is characterized by regularly repeating patterns of cellular proliferation, differentiation and transformation that accompanies follicular maturation and ovulation during the folliculo-luteal transition and corpus lutem (CL) formation and function (1–5). It is well-known that the ruminant reproductive function and especially the ovarian cycle is regulated through endocrine, as well as intraluteal (autocrine/paracrine) actions (6–9). The LH surge triggers a biochemical cascade that leads to the ovulation, resulting in development of the CL (10–12). Ovulation occurs as a result of a dynamic interaction between the luteinising hormone (LH) surge and local follicular factors including steroid hormones, extracellular matrix (ECM) proteases, prostaglandins, vasoactive peptides and growth factors in a time-dependent manner (1, 13–15). During these developments in the bovine ovary, steroid hormones and prostaglandins seem to be highly important regulatory mediators playing a central role in the regulation of the estrous cycle (16–22). Progesterone (P4) and estradiol (E2) steroid production of ovulatory follicles change dramatically during the preovulatory period, suggesting them to have an important role during ovulation (19, 23–25). The later stage of follicular development, ovulation and CL formation depends upon growth of new blood vessels (angiogenesis) and the establishment of a functional blood supply (12, 26, 27).
A recent finding demonstrates that steroid hormones and prostaglandins in addition to different angiogenic factors are required for angiogenesis and folliculo-luteal transition (28–33). The prostaglandins are of particular interest because of their endocrine as well as local effects within the ovarian tissue during different physiological stages (20, 34, 35). Intraluteal prostaglandin production is regulated by a variety of endocrine and autocrine/paracrine factors secreted by different immune cells, namely, macrophages, eosinophils, lymphocytes and monocytes (5, 8, 36). The production of prostaglandins from arachidonic acid is primarily governed by the rate-limiting enzymes cyclooxygenase (COX)-1 and COX-2 (16, 34). The downstream enzymes, PTGF synthase (PTGFS) and PTGE synthase (PTGES), catalyze the conversion of prostaglandin H2 precursors to prostaglandin F2alpha (PTGF) and prostaglandin E2 (PTGE) respectively, (14, 20). PTGF has the highest affinity for the specific receptor (PTGFR), and PTGE may interact with at least four receptor subtypes (PTGER1—PTGER4) and initiate biological signaling pathways (21, 37–39).
The steroid hormones and prostaglandins were shown to regulate ovarian cycle in cattle, but the examination of these factors during final follicle regulation, ovulation and CL formation, has not been thoroughly elucidated to date. Therefore, we tested the hypothesis if the preovulatory LH surge may affect COX-2, prostaglandin synathases (PTGFS and PTGES), prostaglandin ligands (PTGF and PTGE) and their receptors (PTGFR, PTGER2, and PTGER4), which may have further effects to the folliculo-luteal transition and CL formation in the cow. With the present study, we aim to evaluate the expression pattern and localization of earlier mentioned prostaglandin family members in time-defined follicle classes before (control) and after the application of GnRH and after ovulation (early CL) in the cow.
Materials and Methods
Animals, Procedure of Superovulation and Collection of Ovaries
The animal trail was approved by the animal ethics committee located at the government of Upper Bavaria (reference number 211-2531.3-33/96). The study was conducted on 30 German Fleckvieh cows and the superovulation procedure was conducted as described by Berisha et al. (40). For confirmation of LH surge, blood samples were collected from the jugular vein at −24 h, −12 h, −1 h, and 0 h before and 3 h and 12 h after GnRH application (27). The bovine ovaries (containing preovulatory follicles or early CL) were collected at (I) 0 h, (II) 4 h, (III) 10 h, (IV) 20 h, (V) 25 h (for follicle collection) and (VI) 60 h (for early CL collection) relative to injection of GnRH (n = 5 cow/ group) as described by Berisha et al. (27). The schematic time schedule of the superovulatory treatment and ovary collection is shown in Figure 1.
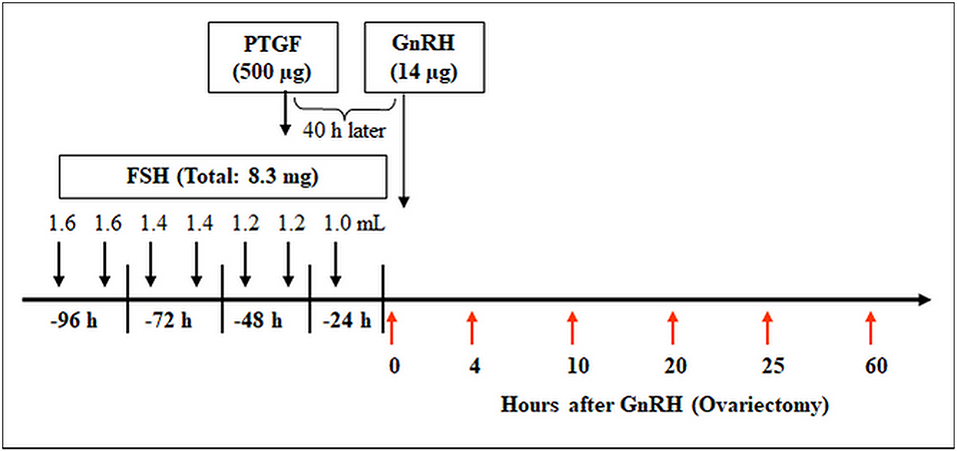
Figure 1. Time schedule of the treatment for multiple ovulation and ovary collection in cows. Ovaries containing preovulatory follicles or new CL were collected at (I) 0 h, (II) 4 h, (III) 10 h, (IV) 20 h, (V) 25 h (follicles), and (VI) 60 h (early CL, Day 2–3) relative to injection of GnRH to induce an luteinizing hormone (LH) surge (n = 5 cows/group).
Collection, Classification and Preparation of Preovulatory Follicles and Early CL
Only follicles which appeared healthy (i.e., well-vascularised and having transparent follicular wall and fluid) and whose diameter were >10 mm were collected. The number of follicles per ovary varied between 8 and 20. Follicular fluid (FF) was aspirated from single follicles, and then the follicles tissue (theca interna and granulose cells) aliquots were stored at −80°C until extraction for RNA. The FF (1.0–1.5 ml) was stored from single follicles at −20°C until determination of P4, E2, PTGF, and PTGE (40). The follicles, after aspiration of FF and injection of fixative (27) and pieces of CL tissue were fixed for immunohistochemical analysis of COX-2 and PTGES (22).
Hormone Determinations
The concentrations of P4, E2, PTGF, and PTGE were determined in the FF with an enzyme immunoassay (EIA) using the second antibody technique as described by our lab and reviewed by Berisha et al. (40). The concentration of progesterone in blood plasma was measured using EIA technique as described by Berisha et al. (41).
Total RNA Extraction and Quality Determination
Total RNA from 200 mg follicles and CL (deep frozen by −80°C) were extracted with peqGOLD TriFAst (PeqLab, Erlangen, Germany) according to the manufacturer's instructions and described by Berisha et al. (40). For DNA digestion the DNA-free kit (Ambion, Austin, USA) was used. Total RNA was dissolved in RNAse-free water and spectroscopically quantified at 260 nm. The purity of isolated RNA was verified by optical density (OD) absorption ratio OD260 nm/OD280 nm between 1.8 and 2.0.
The RNA integrity was measured with the Agilent 2100 bioanalyzer (Agilent Technologies, Deutschland Gmbh, Waldbronn, Germany) in conjunction with the RNA 6000 Nano Assay according to the manufacturer's instructions. The Bioanalyzer 2100 enables the standardization of total RNA quality control for quantitative downstream applications (42). The automatically calculated RNA Integrity Number (RIN) allows classification of total RNA based on a numbering system from 1 to 10, with 1 being the most degraded profile and 10 being the most intact (43). Herein integer total RNA with RIN values of 7–8 were achieved over all tissue extractions.
RNA Reverse Transcription and Real-Time PCR
Constant amounts of 1 μg of total RNA were reverse-transcribed to cDNA using the following master mix: 26 μl RNAse-free water, 12 μl 5 × Buffer (Promega, Mannheim, Germany), 3 μl Random Primers (50 μM) (Invitrogen, Carlsbad, Germany), 3 μl dNTPs (10 mM) (Fermentas, St. Leon-Rot, Germany) and 200 U of M-MLV Reverse Transcriptase (Promega, Mannheim, Germany) according to the manufacturer's instructions. A master mix of the reaction components was prepared according to Berisha et al. (22). The following Real-Time PCR protocol was employed for all investigated factors: denaturation for 10 min at 95°C, 40 cycles of a three segmented amplification and quantification program (denaturation for 10 s at 95°C, annealing for 10 s at 60°C, elongation for 15 s at 72°C), a melting step by slow heating from 60 to 99°C with a rate of 0.5°C/s and continuous fluorescence measurement, and a final cooling down to 40°C. Data were analyzed using Rotor-Gene 3000 software (Corbett Research version 5.03). The relative mRNA abundance of each target gene were calculated using the “comparative quantification” method (Corbett Reasearch). The changes in mRNA expression of examined target genes were assayed by normalization to the stable expressed and internal UBQ control gene. In order to obtain the CT (cycle threshold) difference the data were analyzed using the well-established ΔΔCT method described by Livak and Schmittgen (44). Thereby ΔCT was not subtracted from a non-treated control group, which does not exist in this study, but from the constant number 40, so that a high “40-ΔCT” value indicated a high-gene expression level and vice versa. This results in directly comparable relative expression values between the examined follicle classes before and after the application of GnRH and after ovulation (early CL) in the cow.
Immunohistochemistry of COX-2 and PTGES
Paraffin-embedded mature follicles and CL tissue (fixed in Bouin's fluid for 24 h) were cut into 5-μm serial sections and collected on amino-propyltriethoxysilane coated slides (SupraFrost Ultra Plus; Menzel-Gläser, Braunschweig, Germany). Paraffin sections were dewaxed and then washed three times for 5 min with PBS at pH 7.4. The sections were incubated with polyclonal primary antibodies to PTGES (ab62050; diluted 1:300, host rabbit; Abcam, Cambridge, UK; secondary antibody: pig anti-rabbit IgG (F(ab′)2), diluted 1:300) and with polyclonal primary antibodies to COX-2 (ab2367; diluted 1:400, host goat; Abcam; secondary antibody: rabbit-anti-goat IgG (F(ab′)2), diluted 1:300) at 6°C overnight. Endogenous peroxidase activity was blocked with 7.5% H2O2 (diluted in distilled water) at room temperature for 10 min.
Nonspecific antibody binding was blocked with Dako protein block serum-free (Dako Deutschland GmbH; Hamburg, Germany) for 10 min. The sections were incubated with the primary antibodies at 6°C overnight. Localization of the antigen was achieved using the avidin–biotin-complex technique. The appropriate biotinylated secondary antibodies were incubated with the sections for 16 h at room temperature. Subsequently, treatment with Strept-ABComplex-HRP (Dako Deutschland GmbH) was performed for 30 min at room temperature, and treatment with 1 mg/ml 3,3-diaminobenzidinetetrahydrochloride (BIOTREND Chemikalien GmbH; Cologne, Germany) was performed for 5 min. All incubations were performed in a humidified chamber.
Sections were slightly counterstained with haematoxylin (20 s), dehydrated and mounted with the Eukitt quick hardening mounting medium for microscopy (Fluka Analytical; Sigma-Aldrich Laborchemikalien GmbH, Seelze, Germany). Negative controls were performed by incubating with the 3,3-diaminobenzidine reagent alone to exclude the possibility of detecting the non-suppressed endogenous peroxidase activity. A lack of detectable staining in the negative controls demonstrated that the reactions were specific. The images were captured with a Leica Labo-Lux microscope equipped with a Zeiss Axiocam camera (Zeiss, Munich, Germany). As positive controls, ovarian tissues from quails (Coturnix japonica) and cats (Felis silvestris) of proven immunoreactivity were used (45). The reaction intensities were marked as weak (+), distinct (++), and strong (+ + +).
Statistical Analysis
The statistical significance of differences in hormone concentration in FF and mRNA expressions in follicle and CL tissue of the examined factors was assessed by one way ANOVA followed by the Holm Sidak as a multiple comparison test. Data, which failed the normality or equal variance test, were tested by one way ANOVA on ranks followed by the Kruskal-Wallis test (Sigma Stat 3.0). All experimental data are shown as means±SEM (n = 8–12). The differences were considered significant if P < 0.05.
Results
Concentration of E2, P4 and PTGE and PTGF in FF During Preovulation
We analyzed the concentration of E2, P4, PTGF, and PTGE in FF for a better characterization of follicle groups before and after GnRH application and during ovulation (Table 1).
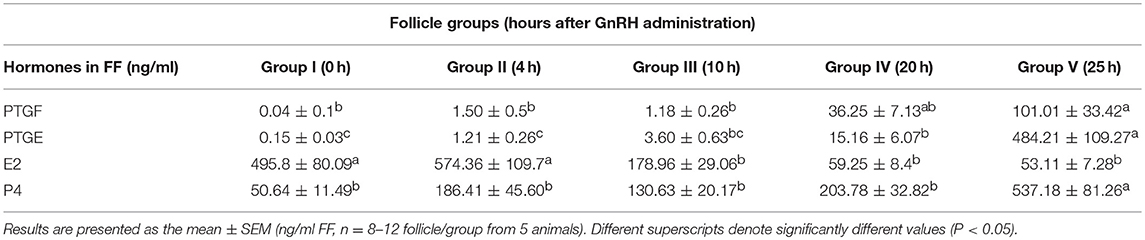
Table 1. Concentration of Prostaglandin F2alpha (PTGF), Prostaglandin E2 (PTGE), Estradiol (E2), and Progesterone (P4) in follicular fluid (FF) of preovulatory follicles collected at (I) 0 h, (II) 4 h, (III) 10 h, (IV) 20 h, and (V) 25 h relative to injection of GnRH to induce an LH surge.
The high E2 concentration in the FF of the follicle group before GnRH treatment (495.8 ng/ml) and during luteinizing hormone (LH) surge (4 h after GnRH, 574.36 ng/ml), is followed by a significant (P < 0.05) downregulation afterwards with the lowest level during ovulation (25 h after GnRH, 53.11 ng/ml). In contrast the concentration of P4 was very low before LH surge (50.64 mg/ml) followed by a significant upregulation (P < 0.05) during ovulation (537.18 ng/ml).
The low concentration of PTGF (0.04 ng/ml) and PTGE (0.15 ng/ml) in FF before GnRH, increased continuously in follicle groups before ovulation and displayed a further significant and dramatic increase (P <0.05) around ovulation (101.01 ng/ml respectively 484.21 ng/ml).
Confirmation of Primer Specificity and Sequence Analysis
The mRNA expression was quantified by the reverse transcription quantitative polymerase chain reaction (RT-qPCR), as described in detail in our previous study (46). Amplified RT-qPCR products were separated on agarose gel electrophoresis for length verification, and for sequence confirmation additionally sequenced by a commercial provider (TopLab, Munich, Germany). All amplified RT-qPCR products showed 100% homology to the known bovine gene sequence published in NCBI GenBank (complete sequences are not shown herein). The primer sequences and expected PCR product length are shown in Table 2.
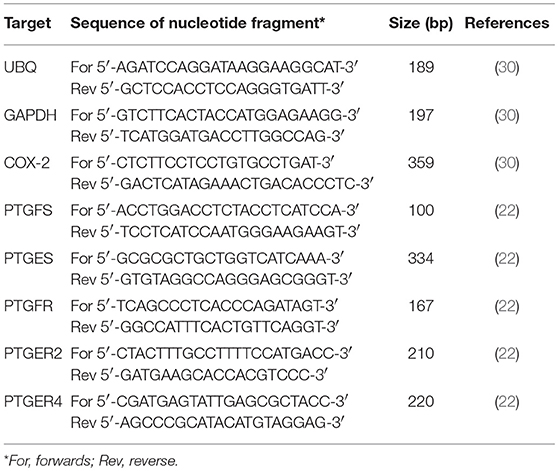
Table 2. Primer sequences for investigates genes, respective RT-qPCR product length, and appropriate reference.
Relative mRNA Abundance
To evaluate equal quantity and quality of the preceding RT reaction in each sample, the housekeeping genes ubiquitin (UBQ) and glyceraldehyde-3-phosphate dehydrogenase (GAPDH) were examined in all samples. As both housekeeping genes were constantly expressed in all samples we choose UBQ as normalizer. The results of mRNA expression of examined factors (Figures 2, 3) are presented as changes (40-ΔCT ± SEM from 6 follicles or CL per group) in the target gene expression, normalized to UBQ as described by Berisha et al. (22).
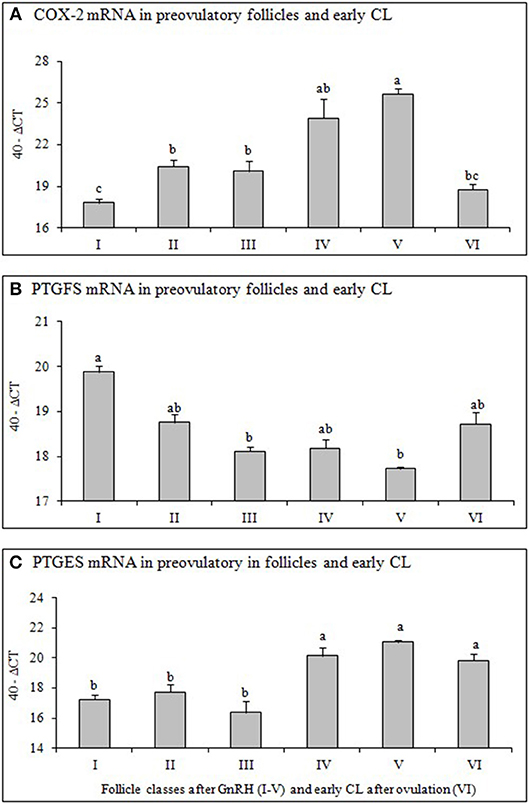
Figure 2. Changes of mRNA for (A) Cyclooxygenase 2 (COX-2), (B) Prostaglandin F2alpha synthase (PTGFS) and (C) Prostaglandin E2 synthase (PTGFS) in preovulatory follicles collected at (I) 0 h, (II) 4 h, (III) 10 h, (IV) 20 h, (V) 25 h (follicles), and (VI) 60 h (new CL, Day 2–3) relative to injection of GnRH to induce an LH surge (5 follicles or CL per group from 5 animals). Different superscripts denote statistically different values (P < 0.05).
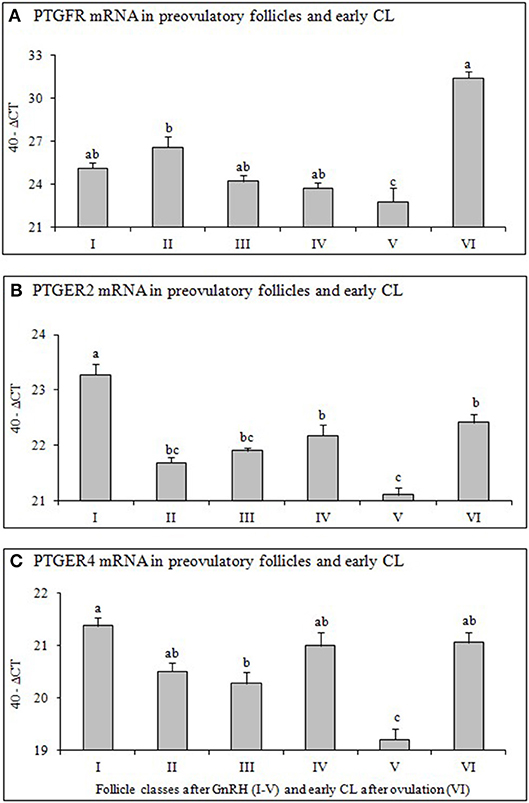
Figure 3. Expression of mRNA for (A) Prostaglandin F2alpha receptor (PTGFR), (B) Prostaglandin E2 receptor 2 (PTGER2), and (C) Prostaglandin E2 receptor 4 (PTGER4) in preovulatory follicles collected at (I) 0 h, (II) 4 h, (III) 10 h, (IV) 20 h, (V) 25 h (follicles), and (VI) 60 h (new CL, Day 2–3) relative to injection of GnRH to induce an LH surge (5 follicles or CL/group from 5 animals). Different superscripts denote statistically different values (P < 0.05).
Relative mRNA Expression of COX-2, PTGES and PTGFS in Preovulatory Follicles and Early CL
The mRNA expression of COX-2 (Figure 2A) increased significantely (P < 0.05) 4 h after GnRH and again 20 h after GnRH, followed by a significant decrease (P < 0.05) after ovulation (early CL). The mRNA of PTGFS (Figure 2B) in follicles before GnRH was high followed by a continuous and significant downregulation (P < 0.05) afterwards. In contrast, PTGES mRNA abundance increased significantely (P < 0.05) in follicles 20 h after GnRH treatment and remained high afterwards (Figure 2C).
Relative mRNA Expression of PTGFR, PTGER2 and PTGER4 in Preovulatory Follicles and Early CL
The mRNA abundance of PTGFR (Figure 3A), PTGER2 (Figure 3B), and PTGER4 (Figure 3C) in follicles before GnRH was high, followed by a continuous and significant down regulation afterwards and significant (P < 0.05) increase only after ovulation (early CL).
Immunohistochemical Localization of COX-2 in Preovulatory Follicles and Early CL
The multilayered epithelium of follicles at the time of ovulation (follicle diameter 18- 25 mm) displayed a clear signal for COX-2 (Figure 4A). COX-2 was expressed in the cytoplasm of more than 90% of the high prismatic basal cells located at the top of the basal membrane (BM). A slight staining was also seen in the theca interna surrounding the follicular epithelium. On days 1–2 after ovulation a strong signal for COX-2 could be noted in a subpopulation of cells located in the apical half of the folded membrana granulosa around the former antrum (Figure 4C). They were surrounded by other granulosa cells (GC), which showed only a weak signal. On days 3–4 scattered and distinctly COX-2-positive progesterone producing granulosa-luteal cells (LC) occurred in the developing corpus luteum (Figure 4E).
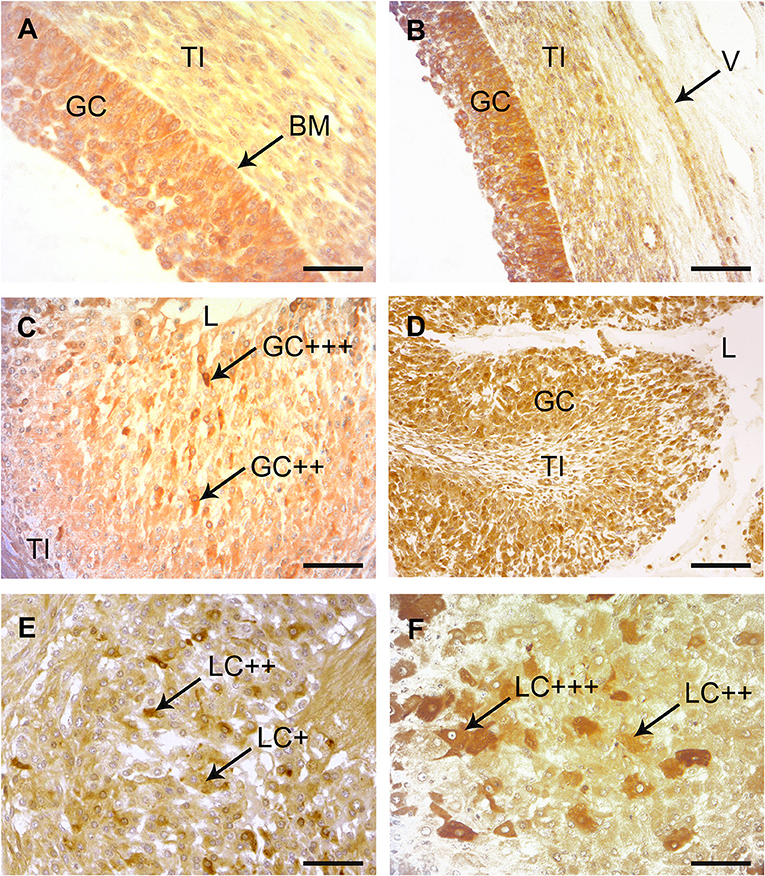
Figure 4. Immunohistochemical localization of COX-2 and PTGES in bovine preovulatory follicles and early corpus lutem (CL). (A) Immunohistochemical localization of COX-2 in a mature preovulatory follicle. A distinct to strong immunostaining was found throughout the granulosa cells (GC) with increaded intensity adjacent to the basement membrane (BM). The theca interna (TI) was also weakly stained. SB = 75 μm. (B) Immunohistochemical localization of PTGES in a mature preovulatory follicle. A distinct to strong immunostaining is found in the cytoplasma of the GC. Similar to the result with COX-2, the basal cells show stronger reactions than the luminal cells. The TI and the endothelium of this longitudinal cut vessel (V) show slight positive reactions. SB = 150 μm. (C) Immunohistochemical localization of COX-2 in the CL at days 1–2. A subpopulation of the folded membrana granulosa (GC) surrounding the remaining lumen (L) of the former antrum of the ovulated follicle display distinct (++) to strong (+ + +) positive immunostaining with the COX-2 antibody. TI = theca interna; SB = 100 μm. (D) Immunohistochemical localization of PTGES in the CL at days 1–2. Many of the former GC display distinct immunostaining. L = lumen, TI = theca interna; SB = 200 μm. (E) Immunohistochemical localization of COX-2 in the CL at days 3–4. At this magnification many granulosa-luteal cells (LC) with distinct (++) immunostaining alternate with negative or only weakly stained cells (+). SB = 150 μm. (F) Immunohistochemical localization of PTGES in the CL at days 3–4. Several of the granulosa-luteal cells (LC) show strong immunostaining (+ + +) with PTGES antibodies, whereas the rest of this cell population display only distinct to moderate immunopositivity (++). SB = 75 μm.
Immunohistochemical Localization of PTGES in Preovulatory Follicles and Early CL
A distinct to strong immunoreactivity for PTGES was found throughout the follicular epithelium before ovulation (Figure 4B). Most cells of the theca interna (TI) displayed only weak immunoreactivity for this enzyme and the stromal cells of the theca were almost negative. On days 1–2 after ovulation, granulosa cells (GC) of the developing CL showed distinct signal with the PTGES antibody (±). The signal intensity of the granulosa-luteal cells (LC) increased in the CL at days 3–4 (Figure 4F).
Discussion
Recent studies have demonstrated the important role of steroid hormones and prostaglandins during follicle development, ovulation and CL formation in different species and various study models (8, 22, 31, 47, 48). Our present study demonstrates the expression pattern of steroid hormones (E2 and P4) and prostaglandin family members (COX-2, PTGFS, PTGES, PTGF, PTGE, and their receptors) in different timely defined follicle classes before and after GnRH application and after ovulation (early CL) in the cow. We have shown in our previous studies (40, 49) that superovulated follicles after GnRH application are comparable to natural ovulation in the cow. We demonstrated in addition that also the time interval between the LH surge and ovulation is quite comparable between induced ovulation (40) and spontaneous ovulation in cows (49).
The LH surge initiates a series of biochemical events in ovary, such as upregulation of steroids, prostaglandins, ECM proteases and many locally produced growth and angiogenic factors to complement gonadotropins action in process of ovulation and CL formation (8, 13, 27, 50–52).
It is well-known that P4 and E2 steroid production of preovulatory follicles change dramatically during the periovulatory period, suggesting them to play an important role during final follicle development and ovulation (11, 14, 23–25, 53, 54). In addition our previous studies demonstrated clear evidence for steroids as local regulators of follicular and luteal activity (4, 19). In vivo and in vitro studies demonstrated production and localization of P4 and E2 and their specific receptors in both granulosa and theca cells of perovulatory follicles (11, 14, 23–25). The E2 concentration in the FF of our study (Table 2) was high in the follicle group before and during LH surge, followed by a significant downregulation afterwards. In contrast the concentration of P4 was very low before LH surge followed by a significant upregulation during LH surge, with maximal level during ovulation (Table 2). Our present data agree with results of Fortune et al. (11) suggesting that increase in follicular P4 production and associated decreases in E2 concentration in FF prior ovulation, reflect transition from a follicular to a luteal steroidogenic profile of cells.
The results of our present study clearly demonstrate the expression pattern of examined prostaglandin family members, which depends on the developmental stage of the follicles before and after LH surge and after ovulation (early CL) in the bovine ovary. The multilayered epithelium of mature follicles shows a distinct to strong signal for COX-2 and PTGES, whereas the theca interna is only weakly positive (Figure 4). At day 1–2 after ovulation, a subpopulation of GC of the former follicle epithelium is distinctly immunopositive for COX-2 and PTGES. According to Fortune et al. (11), the major source of prostaglandins is believed to be GC of the preovulatory follicle. At day 3–4 large (granulosa luteal cells) and small (theca luteal cells) can be discerned. A subpopulation of the large luteal cells is distinctly immunopositive for both enzymes. As COX-2 and PTGES are key enzymes for the synthesis of prostaglandins, their similar expression pattern makes sense.
It is well-known that LH surge and ovulation process influence production of COX, prostaglandin synthases (PTGFS and PTGES), prostaglandin ligands (PTGF and PTGE) but also regulates expression of prostaglandin receptors (PTGFR, PTGER2, and PTGE4). In our present study the concentration of PTGF and PTGE in FF (Table 2) was low prior to LH surge (before GnRH application), increased continuously and significantly 20h after GnRH and further increase around ovulation (25 h after GnRH). The rapid increase of PTGF and especially PTGE in preovulatory follicles close to ovulation is in accordance with previous studies in bovine and other mammals (55–57). The data of Fortune et al. (11) supported the hypothesis that prostaglandins, especially PTGE, can stimulate P4 secretion by both follicular cell types and suggest a positive feedback relationship between P4 and the prostaglandins. In addition, Bridges and Fortune (21) demonstrated that both theca and GC of preovulatory follicles are targets for both the PTGF and the PTGE produced by the GC. The results of some earlier studies showed clearly that ovulation process is dependent from follicular prostaglandin production also in cows (23, 47, 53–59). LH surge has been reported to induce enzymes modulating prostaglandin production and specific prostaglandin receptors in the preovulatory follicles (21, 33, 39, 60).
The upregulation of COX-2 and PTGES expression in our study was followed by a dramatic increase of PTGF and PTGE in the FF starting 20 h after GnRH application (Table 2). The preovulatory increases of prostaglandin concentration in FF have shown to be necessary component of the ovulation in several species (11, 33, 57, 59–61). Some earliest studies in different species suggested both PTGF and PTGE to be important to the ovulatory process (59, 62). However, the recent studies in different mammalian species clearly demonstrated that PTGE is more essential for ovulation and CL formation (14, 39, 47). Based on these findings, it is widely accepted that PGE2 is an essential paracrine mediator of the LH surge in mammalian (39, 57). The concentration of PTGE during ovulation in our study (Table 2) was much higher (484.21 ng/ml) than PTGF concentration (101.01 ng/ml). The marked increase of PTGF and especially PTGE in response to LH surge agrees with observation of other authors made in cow (23, 33, 59, 63).
The final follicle growth and ovulation process involves intense interactions between endothelial, steroidogenic and migrating immune cells, leading to the folliculo-luteal cell transition, ECM remodeling and further angiogenesis in the developing CL (9). Previous studies demonstrated the effects of LH surge in remodeling of ECM by regulation of diverse proteases of the plasminogen activators (PA) and the matrix metalloproteinase (MMP) enzyme systems in follicles during ovulation process and CL formation in cow (64–69). In addition Fortune et al. (11) demonstrated the effect of LH, P4 and prostaglandins in regulation of ADAMTS proteases (A Disintegrin And Metalloproteinase with Thrombo Spondin motifs), suggesting an important role of these factors in remodeling the preovulatory follicle during ovulation and angiogenesis. This study as well as others demonstrated that prostaglandins act via multiple receptors to regulate follicle-luteal transition and CL formation (22, 37–39, 57).
Not just the upregulation, but also the shift in the localization of different factors after LH surge suggests them to have an important role during the follicle-luteal transition. In our previous study in the same samples we have shown a distinct change in localization of FGF2 in the bovine follicles from the theca cell compartments (cytoplasm of endothelial cells) to the GC initiated by the LH surge. We suggested that nuclear FGF2 localization may be important for GC survival until ovulation and for a transition of GC to luteal cells in early CL (40). Examples of spatial differences are obvious for FGF2 (28) and, in particular, FGF7, for which the ligand is localized in the theca tissue and its receptor is localized mainly in GC (70). However, the aim of the present study was to analyze the regulation of the factors examined in whole follicle tissue (without GC and TI separation) in order to compare the expression between whole follicles tissue (GC and TI) and CL during follicle–luteal transition.
The significant upregulation of all prostaglandin receptors just after ovulation (early CL) in our study (Figure 3) demonstrates the important role of prostaglandins they play in the ovulation process, luteinisation and CL formation. Our previous study (22) demonstrated that PTGF and PTGE are involved in the local (autocrine/paracrine) mechanisms regulating CL formation and function. In early CL, the PTGE is known as a luteotropic factor, as it stimulates in vitro P4 production by bovine luteal steroidogenic cells (71, 72). In addition, PTGF stimulates P4 production, as well as PTGE, by cultured luteal cells (16, 73–75). Furthermore, PTGF and PTGE can suppress the apoptosis of steroidogenic and endothelial cells in bovine CL (72). In addition, our previous in vivo studies demonstrated that PTGF stimulates itself and PTGE in bovine CL (76, 77).
Moreover, in the early CL, prostaglandins regulate the expression of angiogenic factors in a luteal stage-dependent manner (9, 12, 35, 78). High expression and tissue levels of angiogenic factors during the early luteal stage (PTGF-refractory days) suggest a survival function for both endothelial cells of the capillaries and steroidogenic cells of the CL (12, 79). In our recent study we assume that LH modulates prostaglandin production in the bovine CL by stimulating the expressions of COX-2, prostaglandin synthases and angiogenic factors and that these actions help to maintain CL function during the early luteal phase (22). Moreover, intraluteal prostaglandins in the early CL may have different other functions, such as cellular transition and differentiation, blood flow regulation and intercellular communication (34, 80).
In conclusion, our results indicate that the LH surge upregulated prostaglandin family members in the follicle before ovulation, support the potential role of these system components in GC and TI tissue remodeling during ovulation process. The further upregulation of prostaglandin family members after ovulation (early CL) suggests that they play an important role in follicle-luteal transition and CL formation in the cow.
Data Availability
All datasets generated for this study are included in the manuscript and/or the supplementary files.
Ethics Statement
The experimental protocol was approved by the institutional care and use committee (AZ 211-2531.3-33/96).
Author Contributions
BB and DS: designed and executed all the experiments. BB: wrote the manuscript. MP: qRT-PCR and data analysis. FS and DR: immunohistochemistry and data analysis. All the authors edited the manuscript.
Funding
This study was supported by the German Research Foundation (DFG BE 3189/2-2).
Conflict of Interest Statement
The authors declare that the research was conducted in the absence of any commercial or financial relationships that could be construed as a potential conflict of interest.
References
1. Fortune JE. Ovarian follicular growth and development in mammals. Biol Reprod. (1994) 50:225–32. doi: 10.1095/biolreprod50.2.225
2. Fraser HM, Lunn SF. Angiogenesis and its control in the female reproductive system. Br Med Bull. (2000) 56:787–97. doi: 10.1258/0007142001903364
3. Berisha B, Schams D, Kosmann M, Amselgruber W, Einspanier R. Expression and tissue concentration of vascular endothelial growth factor, its receptors, and localization in the bovine corpus luteum during estrous cycle and pregnancy. Biol Reprod. (2000) 63:1106–14. doi: 10.1095/biolreprod63.4.1106
4. Berisha B, Schams D. Ovarian function in ruminants. Domest Anim Endocrinol. (2005) 29:305–17. doi: 10.1016/j.domaniend.2005.02.035
5. Meidan R, Levy N. The ovarian endothelin network: an evolving story. Trends Endocrinol Metab. (2007) 18:379–85. doi: 10.1016/j.tem.2007.09.002
6. Berisha B, Schams D, Miyamoto A. The expression of angiotensin and endothelin system members in bovine corpus luteum during estrous cycle and pregnancy. Endocrine. (2002) 19:305–12. doi: 10.1385/ENDO:19:3:305
7. Robinson RS, Nicklin LT, Hammond AJ, Schams D, Hunter MG, Mann GE. Fibroblast growth factor 2 is more dynamic than vascular endothelial growth factor A during niswenthe follicle-luteal transition in the cow. Biol Reprod. (2007) 77:28–36. doi: 10.1095/biolreprod.106.055434
8. Skarzynski DJ, Piotrowska-Tomala KK, Lukasik K, Galvão A, Farberov S, Zalman Y, et al. Growth and regression in bovine corpora lutea: regulation by local survival and death pathways. Reprod Domest Anim. (2013) 48(Suppl. 1):25–37. doi: 10.1111/rda.12203
9. Berisha B, Schams D, Rodler D, Pfaffl MW. Angiogenesis in The Ovary - the most important regulatory event for follicle and corpus luteum development and function in cow - an overview. Anat Histol Embryol. (2016) 45:124–30. doi: 10.1111/ahe.12180
10. Schams D, Berisha B. Regulation of corpus luteum function in cattle-an overview. Reprod Domest Anim. (2004) 39:241–51. doi: 10.1111/j.1439-0531.2004.00509.x
11. Fortune JE, Willis EL, Bridges PJ, Yang CS. The periovulatory period in cattle: progesterone, prostaglandins, oxytocin and ADAMTS proteases. Anim Reprod. (2009) 6:60–71.
12. Zalman Y, Klipper E, Farberov S, Mondal M, Wee G, Folger JK, et al. Regulation of angiogenesis-related prostaglandin f2alpha-induced genes in the bovine corpus luteum. Biol Reprod. (2012) 30:92. doi: 10.1095/biolreprod.111.095067
13. Tsafriri A, Lindner HR, Zor U, Lamprecht SA. Physiological role of prostaglandins in the induction of ovulation. Prostaglandins. (1972) 2:1–10. doi: 10.1016/0090-6980(72)90024-X
14. Bridges PJ, Komar CM, Fortune JE. Gonadotropin-induced expression of messenger ribonucleic acid for cyclooxygenase-2 and production of prostaglandins E and F2alpha in bovine preovulatory follicles are regulated by the progesterone receptor. Endocrinology. (2006) 147:4713–22. doi: 10.1210/en.2005-1575
15. Berisha B, Schams D, Rodler D, Sinowatz F, Pfaffl MW. Expression pattern of HIF1alpha and vasohibins during follicle maturation and corpus luteum function in the bovine ovary. Reprod Domest Anim. (2017) 52:130–9. doi: 10.1111/rda.12867
16. Milvae RA, Hansel W. Prostacyclin, prostaglandin F2α and progesterone production by bovine luteal cells during the estrous cycle. Biol Reprod. (1983) 29:1063–8. doi: 10.1095/biolreprod29.5.1063
17. Okuda K, Uenoyama Y, Berisha B, Lange IG, Taniguchi H, Kobayashi S, et al. Estradiol-17beta is produced in bovine corpus luteum. Biol Reprod. (2001) 65:1634–9. doi: 10.1095/biolreprod65.6.1634
18. Kobayashi S, Acosta TJ, Ozawa T, Hayashi K, Berisha B, Ohtani M, et al. Intraluteal release of angiotensin II and progesterone in vivo during corpora lutea development in the cow: effect of vasoactive peptides. Biol Reprod. (2002) 66:174–9. doi: 10.1095/biolreprod66.1.174
19. Schams D, Berisha B. Steroids as local regulators of ovarian activity in domestic animals. Domest Anim Endocrinol. (2002) 23:53–65. doi: 10.1016/S0739-7240(02)00145-5
20. Arosh JA, Banu SK, Chapdelaine P, Madore E, Sirois J, Fortier MA. Prostaglandin biosynthesis, transport, and signaling in corpus luteum: a basis for auto-regulation of luteal function. Endocrinology. (2004) 145:2551–60. doi: 10.1210/en.2003-1607
21. Bridges PJ, Fortune JE. Regulation, action and transport of prostaglandins during the periovulatory period in cattle. Mol Cell Endocrinol. (2007) 15:1–9. doi: 10.1016/j.mce.2006.08.002
22. Berisha B, Schams D, Rodler D, Sinowatz F, Pfaffl MW. Changes in the expression of prostaglandin family members in bovine corpus luteum during the estrous cycle and pregnancy. Mol Reprod Dev. (2018) 85:622–34. doi: 10.1002/mrd.22999
23. Wise T, Vernon MW, Maurer RR. Oxytocin, prostaglandins E and F, estradiol, progesterone, sodium and potassium in preovulatory bovine follicles, either developed normally or stimulated by FSH. Theriogenology. (1986) 26:757–78. doi: 10.1016/0093-691X(86)90006-3
24. Suchanek E, Simunic V, Macas E, Kopjar B, Grizelj V. Prostaglandin F2 alpha, progesterone and estradiol concentrations in human follicular fluid and their relation to success of in vitro fertilization. Eur J Obstet Gynecol Reprod Biol. (1988) 28:331–9. doi: 10.1016/0028-2243(88)90019-6
25. Komar CM, Berndtson AK, Evans AC, Fortune JE. Decline in circulating estradiol during the periovulatory period is correlated with decreases in estradiol and androgen, and in messenger RNA for p450 aromatase and p450 17alpha-hydroxylase, in bovine preovulatory follicles. Biol Reprod. (2001) 64:1797–805. doi: 10.1095/biolreprod64.6.1797
26. Hayashi KG, Berisha B, Matsui M, Schams D, Miyamoto A. Expression of mRNA for the angiopoietin-tie system in granulosa cells during follicular development in cows. J Reprod Dev. (2004) 50:477–80. doi: 10.1262/jrd.50.477
27. Berisha B, Steffl M, Welter H, Kliem H, Meyer HH, Schams D, et al. Effect of the luteinising hormone surge on regulation of vascular endothelial growth factor and extracellular matrix-degrading proteinases and their inhibitors in bovine follicles. Reprod Fertil Dev. (2008) 20:258–68. doi: 10.1071/RD07125
28. Berisha B, Schams D, Kosmann M, Amselgruber W, Einspanier R. Expression and localisation of vascular endothelial growth factor and basic fibroblast growth factor during the final growth of bovine ovarian follicles. J Endocrinol. (2000) 167:371–82. doi: 10.1677/joe.0.1670371
29. Schams D, Kosmann M, Berisha B, Amselgruber WM, Miyamoto A. Stimulatory and synergistic effects of luteinising hormone and insulin like growth factor 1 on the secretion of vascular endothelial growth factor and progesterone of cultured bovine granulosa cells. Exp Clin Endocrinol Diabetes. (2001) 109:155–62. doi: 10.1055/s-2001-14839
30. Hayashi KG, Acosta TJ, Tetsuka M, Berisha B, Matsui M, Schams D, et al. Involvement of angiopoietin-tie system in bovine follicular development and atresia: messenger RNA expression in theca interna and effect on steroid secretion. Biol Reprod. (2003) 69:2078–84. doi: 10.1095/biolreprod.103.017152
31. Trau HA, Brannstrom M, Curry TE Jr, Duffy DM. Prostaglandin E2 and vascular endothelial growth factor A mediate angiogenesis of human ovarian follicular endothelial cells. Hum Reprod. (2016) 31:436–44. doi: 10.1093/humrep/dev320
32. Bender HR, Trau HA, Duffy DM. Placental growth factor is required for ovulation, luteinization, and angiogenesis in primate ovulatory follicles. Endocrinology. (2018) 159:710–22. doi: 10.1210/en.2017-00739
33. Shrestha K, Meidan R. The cAMP-EPAC pathway mediates PGE2-induced FGF2 in bovine granulosa cells. Endocrinology. (2018) 159:3482–91. doi: 10.1210/en.2018-00527
34. Wiltbank MC, Ottobre JS. Regulation of intraluteal production of prostaglandins. Reprod Biol Endocrinol. (2003) 1:91. doi: 10.1186/1477-7827-1-91
35. Weems YS, Ma Y, Ford SP, Nett TM, Vann RC, Lewis AW, et al. Effects of intraluteal implants of prostaglandin E1 or E2 on angiogenic growth factors in luteal tissue of Angus and Brahman cows. Theriogenology. (2014) 82:1224–30. doi: 10.1016/j.theriogenology.2014.07.039
36. Pate JL, Toyokawa K, Walusimbi S, Brzezicka E. The interface of the immune and reproductive systems in the ovary: lessons learned from the corpus luteum of domestic animal models. Am J Reprod Immunol. (2010) 64:275–86. doi: 10.1111/j.1600-0897.2010.00906.x
37. Markosyan N, Duffy DM. Prostaglandin E2 acts via multiple receptors to regulate plasminogen-dependent proteolysis in the primate periovulatory follicle. Endocrinology. (2009) 150:435–44. doi: 10.1210/en.2008-0591
38. Harris SM, Aschenbach LC, Skinner SM, Dozier BL, Duffy DM. Prostaglandin E2 receptors are differentially expressed in subpopulations of granulosa cells from primate periovulatory follicles. Biol Reprod. (2011) 85:916–23. doi: 10.1095/biolreprod.111.091306
39. Kim SO, Duffy DM. Mapping PTGERs to the ovulatory follicle: regional responses to the ovulatory PGE2 Signal. Biol Reprod. (2016) 95:33. doi: 10.1095/biolreprod.116.140574
40. Berisha B, Steffl M, Amselgruber W, Schams D. Changes in fibroblast growth factor 2 and its receptors in bovine follicles before and after GnRH application and after ovulation. Reproduction. (2006) 131:319–29. doi: 10.1530/rep.1.00798
41. Kliem H, Welter H, Kraetzl WD, Steffl M, Meyer HH, Schams D, et al. Expression and localisation of extracellular matrix degrading proteases and their inhibitors during the oestrous cycle and after induced luteolysis in the bovine corpus luteum. Reproduction. (2007) 134:535–47. doi: 10.1530/REP-06-0172
42. Kirchner B, Paul V, Riedmaier I, Pfaffl MW. mRNA and microRNA purity and integrity: the key to success in expression profiling. Methods Mol Biol. (2014) 1160:43–53. doi: 10.1007/978-1-4939-0733-5_5
43. Mueller O, Lightfoot S, Schroeder A. RNA Integrity Number (RIN) – Standardization of RNA Quality Control. Agilent Application Notes Publication Number-5989-1165EN: 1-8. (2004). Available online at: https://www.agilent.com/cs/library/applications/5989-1165EN.pdf
44. Livak KJ, Schmittgen TD. Analysis of relative gene expression data using real-time quantitative PCR and the 2(-Delta Delta C(T)) Method. Methods. (2001) 25:402–8. doi: 10.1006/meth.2001.1262
45. Rodler D, Sinowatz F. Expression of prostaglandin-synthesizing enzymes (cyclooxygenase 1, cyclooxygenase 2) in the ovary of the quail (Coturnix japonica). Folia Biol. (2015) 61:125–33. doi: 10.1016/j.acthis.2014.11.005
46. Berisha B, Meyer HH, Schams D. Effect of prostaglandin F2 alpha on local luteotropic and angiogenic factors during induced functional luteolysis in the bovine corpus luteum. Biol Reprod. (2010) 82:940–7. doi: 10.1095/biolreprod.109.076752
47. Peters MW, Pursley JR, Smith GW. Inhibition of intrafollicular PGE2 synthesis and ovulation following ultrasound-mediated intrafollicular injection of the selective cyclooxygenase-2 inhibitor NS-398 in cattle. J Anim Sci. (2004) 82:1656–62. doi: 10.2527/2004.8261656x
48. Trau HA, Davis JS, Duffy DM. Angiogenesis in the primate ovulatory follicle is stimulated by luteinizing hormone via prostaglandin E2. Biol Reprod. (2015) 92:15. doi: 10.1095/biolreprod.114.123711
49. Schams D, Schallenberger E, Hoffmann B, Karg H. The oestrous cycle of the cow: hormonal parameters and time relationships concerning oestrus, ovulation, and electrical resistance of the vaginal mucus. Acta Endocrinol. (1977) 86:180–92. doi: 10.1530/acta.0.0860180
50. Curry TE Jr, Osteen KG. The matrix metalloproteinase system: changes, regulation, and impact throughout the ovarian and uterine reproductive cycle. Endocr Rev. (2003) 24:428–65. doi: 10.1210/er.2002-0005
51. Schams D, Steinberg V, Steffl M, Meyer HH, Berisha B. Expression and possible role of fibroblast growth factor family members in porcine antral follicles during final maturation. Reproduction. (2009) 138:141–9. doi: 10.1530/REP-09-0033
52. Schilffarth S, Antoni B, Schams D, Meyer HH, Berisha B. The expression of apelin and its receptor APJ during different physiological stages in the bovine ovary. Int J Biol Sci. (2009) 13:344–50. doi: 10.7150/ijbs.5.344
53. Shemesh M. Inhibitory action of follicular fluid on progesterone and prostaglandin synthesis in bovine follicles. J Endocrinol. (1979) 82:27–31. doi: 10.1677/joe.0.0820027
54. Murdoch WJ, Peterson TA, Van Kirk EA, Vincent DL, Inskeep EK. Interactive roles of progesterone, prostaglandins, and collagenase in the ovulatory mechanism of the ewe. Biol Reprod. (1986) 35:1187–94. doi: 10.1095/biolreprod35.5.1187
55. Armstrong DT. Prostaglandins and follicular functions. J Reprod Fertil. (1981) 62:283–91. doi: 10.1530/jrf.0.0620283
56. Murdoch WJ, Hansen TR, McPherson LA. A review: role of eicosanoids in vertebrate ovulation. Prostaglandins. (1993) 46:85–115. doi: 10.1016/0090-6980(93)90037-8
57. Markosyan N, Dozier BL, Lattanzio FA, Duffy DM. Primate granulosa cell response via prostaglandin E2 receptors increases late in the periovulatory interval. Biol Reprod. (2006) 75:868–76. doi: 10.1095/biolreprod.106.053769
58. De Silva M, Reeves JJ. Indomethacin inhibition of ovulation in the cow. J Reprod Fertil. (1985) 75:547–9. doi: 10.1530/jrf.0.0750547
59. Algire JE, Srikandakumar A, Guilbault LA, Downey BR. Preovulatory changes in follicular prostaglandins and their role in ovulation in cattle. Can J Vet Res. (1992) 56:67–9.
60. Sirois J. Induction of prostaglandin endoperoxide synthase-2 by human chorionic gonadotropin in bovine preovulatory follicles in vivo. Endocrinology. (1994) 135:841–8. doi: 10.1210/endo.135.3.8070377
61. Duffy DM, Dozier BL, Seachord CL. Prostaglandin dehydrogenase and prostaglandin levels in periovulatory follicles: implications for control of primate ovulation by prostaglandin E2. J Clin Endocrinol Metab. (2005) 90:1021–7. doi: 10.1210/jc.2004-1229
62. Duffy DM, Stouffer RL. The ovulatory gonadotrophin surge stimulates cyclooxygenase expression and prostaglandin production by the monkey follicle. Mol Hum Reprod. (2001) 7:731–9. doi: 10.1093/molehr/7.8.731
63. Shemesh M, Hansel W. Stimulation of prostaglandin synthesis in bovine ovarian tissue by arachidonic acid and luteinizing hormone. Biol Reprod. (1975) 13:448–52. doi: 10.1095/biolreprod13.4.448
64. Smith MF, McIntush EW, Ricke WA, Kojima FN, Smith GW. Regulation of ovarian extracellular matrix remodelling by metalloproteinases and their tissue inhibitors: effects on follicular development, ovulation and luteal function. J Reprod Fertil Suppl. (1999) 54:367–81.
65. Dow MP, Bakke LJ, Cassar CA, Peters MW, Pursley JR, Smith GW. Gonadotropin surge-induced upregulation of the plasminogen activators (tissue plasminogen activator and urokinase plasminogen activator) and the urokinase plasminogen activator receptor within bovine periovulatory follicular and luteal tissue. Biol Reprod. (2002) 66:1413–21. doi: 10.1095/biolreprod66.5.1413
66. Bakke LJ, Li Q, Cassar CA, Dow MP, Pursley JR, Smith GW. Gonadotropin surge-induced differential upregulation of collagenase-1 (MMP-1) and collagenase-3 (MMP-13) mRNA and protein in bovine preovulatory follicles. Biol Reprod. (2004) 71:605–12. doi: 10.1095/biolreprod.104.027185
67. Li Q, Bakke LJ, Pursley JR, Smith GW. Localization and temporal regulation of tissue inhibitors of metalloproteinases 3 and 4 in bovine preovulatory follicles. Reproduction. (2004) 128:555–64. doi: 10.1530/rep.1.00282
68. Curry TE Jr, Smith MF. Impact of extracellular matrix remodeling on ovulation and the folliculo-luteal transition. Semin Reprod Med. (2006) 24:228–41. doi: 10.1055/s-2006-948552
69. Duffy DM, Ko C, Jo M, Brannstrom M, Curry TE Jr. Ovulation: parallels with inflammatory processes. Endocr Rev. (2018). 40:369–416. doi: 10.1210/er.2018-00075
70. Berisha B, Welter H, Shimizu T, Miyamoto A, Meyer HH, Schams D. Expression of fibroblast growth factor 1 (FGF1) and FGF7 in mature follicles during the periovulatory period after GnRH in the cow. J Reprod Dev. (2006) 52:307–13. doi: 10.1262/jrd.17077
71. Kotwica J, Skarzynski D, Mlynarczuk J, Rekawiecki R. Role of prostaglandin E2 in basal and noradrenaline-induced progesterone secretion by the bovine corpus luteum. Prostaglandins Other Lipid Mediat. (2003) 70:351–9. doi: 10.1016/S0090-6980(02)00149-1
72. Bowolaksono A, Nishimura R, Hojo T, Sakumoto R, Acosta TJ, Okuda K. Anti-apoptotic roles of prostaglandin E2 and F2alpha in bovine luteal steroidogenic cells. Biol Reprod. (2008) 79:310–7. doi: 10.1095/biolreprod.107.066084
73. Miyamoto A, von Lützow H, Schams D. Acute actions of prostaglandin F2 alpha, E2, and I2 in microdialyzed bovine corpus luteum in vitro. Biol Reprod. (1993) 49:423–30. doi: 10.1095/biolreprod49.2.423
74. Okuda K, Uenoyama Y, Lee KW, Sakumoto R, Skarzynski DJ. Progesterone stimulation by prostaglandin F2alpha involves protein kinase C pathway in cultured bovine luteal cells. J Reprod Dev. (1998) 44:79–84. doi: 10.1262/jrd.44.79
75. Kawaguchi S, Bowolaksono A, Yoshioka S, Sakumoto R, Okuda K. Luteoprotective mechanisms of prostaglandin F2α stimulated by luteinizing hormone in the bovine corpus luteum. J Reprod Dev. (2013) 59:225–30. doi: 10.1262/jrd.2012-187
76. Kobayashi S, Miyamoto A, Berisha B, Schams D. Growth hormone, but not luteinizing hormone, acts with luteal peptides on prostaglandin F2alpha and progesterone secretion by bovine corpora lutea in vitro. Prostaglandins Other Lipid Mediat. (2001) 63:79–92. doi: 10.1016/S0090-6980(00)00099-X
77. Kobayashi S, Acosta TJ, Hayashi K, Berisha B, Ozawa T, Ohtani M, et al. Intraluteal release of prostaglandin F2α and E2 during corpora lutea development in the cow. J Reprod Dev. (2002) 48:583–90. doi: 10.1262/jrd.48.583
78. Kobayashi S, Berisha B, Amselgruber WM, Schams D, Miyamoto A. Production and localisation of angiotensin II in the bovine early corpus luteum: a possible interaction with luteal angiogenic factors and prostaglandin F2 alpha. J Endocrinol. (2001) 170:369–80. doi: 10.1677/joe.0.1700369
79. Gabler C, Plath-Gabler A, Killian GJ, Berisha B, Schams D. Expression pattern of fibroblast growth factor (FGF) and vascular endothelial growth factor (VEGF) system members in bovine corpus luteum endothelial cells during treatment with FGF-2, VEGF or oestradiol. Reprod Domest Anim. (2004) 39:321–7. doi: 10.1111/j.1439-0531.2004.00517.x
80. Grazul AT, Kirsch JD, Slanger WD, Marchello MJ, Redmer DA. Prostaglandin F2 alpha, oxytocin and progesterone secretion by bovine luteal cells at several stages of luteal development: effects of oxytocin, luteinizing hormone, prostaglandin F2 alpha and estradiol-17 beta. Prostaglandins. (1989) 38:307–18. doi: 10.1016/0090-6980(89)90135-4
Keywords: prostaglandins, steroids, gene regulation, ovarian function, follicle, ovulation, cow
Citation: Berisha B, Rodler D, Schams D, Sinowatz F and Pfaffl MW (2019) Prostaglandins in Superovulation Induced Bovine Follicles During the Preovulatory Period and Early Corpus Luteum. Front. Endocrinol. 10:467. doi: 10.3389/fendo.2019.00467
Received: 21 March 2019; Accepted: 27 June 2019;
Published: 10 July 2019.
Edited by:
Jens Vanselow, Leibniz Institute for Farm Animal Biology, GermanyReviewed by:
Dariusz Jan Skarzynski, Institute of Animal Reproduction and Food Research (PAN), PolandRubina Kumari Baithalu, National Dairy Research Institute (ICAR), India
Copyright © 2019 Berisha, Rodler, Schams, Sinowatz and Pfaffl. This is an open-access article distributed under the terms of the Creative Commons Attribution License (CC BY). The use, distribution or reproduction in other forums is permitted, provided the original author(s) and the copyright owner(s) are credited and that the original publication in this journal is cited, in accordance with accepted academic practice. No use, distribution or reproduction is permitted which does not comply with these terms.
*Correspondence: Bajram Berisha, YmFqcmFtLmJlcmlzaGEmI3gwMDA0MDt1bmktcHIuZWR1