- 1Children's Diabetes Centre, Perth Children's Hospital, Perth, WA, Australia
- 2UWA Centre for Child Health Research, University of Western Australia, Perth, WA, Australia
- 3School of Human Sciences, University of Western Australia, Perth, WA, Australia
- 4Telethon Kids Institute, Perth Children's Hospital, Perth, WA, Australia
- 5Department of Pediatrics, The Hospital of Halland, Kungsbacka, Sweden
- 6Institute of Clinical Sciences, Sahlgrenska Academy at University of Gothenburg, Gothenburg, Sweden
Regular physical activity during childhood is important for optimal physical and psychological development. For individuals with Type 1 Diabetes (T1D), physical activity offers many health benefits including improved glycemic control, cardiovascular function, blood lipid profiles, and psychological well-being. Despite these benefits, many young people with T1D do not meet physical activity recommendations. Barriers to engaging in a physically active lifestyle include fear of hypoglycemia, as well as insufficient knowledge in managing diabetes around exercise in both individuals and health care professionals. Diabetes and exercise management is complex, and many factors can influence an individual's glycemic response to exercise including exercise related factors (such as type, intensity and duration of the activity) and person specific factors (amount of insulin on board, person's stress/anxiety and fitness levels). International guidelines provide recommendations for clinical practice, however a gap remains in how to apply these guidelines to a pediatric exercise consultation. Consequently, it can be challenging for health care practitioners to advise young people with T1D how to approach exercise management in a busy clinic setting. This review provides a structured approach to the child/adolescent exercise consultation, based on a framework of questions, to assist the health care professional in formulating person-specific exercise management plans for young people with T1D.
Introduction
Regular physical activity during childhood is essential to promote optimal physical (1, 2) and psychological (3, 4) development. Physical activity is a key part of childhood and is not limited to sport and other forms of structured exercise; it encompasses playing and being generally physically active. However, it is well-recognized that many families do not adopt the recommendations that children and adolescents should engage in 60 min or more of physical activity daily (5). Many factors contribute to the fact that a majority of young people do not achieve recommended time spent in activity, including perceived lack of time, motivation and resources. In addition to these factors, children and young people with T1D must overcome specific challenges related to managing diabetes and exercise. These challenges include maintaining stable blood glucose levels before, during and after exercise (6) and fear of hypoglycemia (7), especially on the nights after exercise (8). Health professionals can play a key role in assisting families to overcome these challenges as physical activity confers many benefits for individuals with T1D including; improved glycemic control (9, 10), cardiovascular function (11), blood lipid profiles (10) and psychological well-being (3).
Patterns of physical activity in young people differ from adults and therefore they merit a different management approach. In young children, physical activity is usually unplanned, based- around play and often varies from day to day (12). Thus, in this young age group it can be difficult to make planned adjustments of insulin or carbohydrate, and caregivers need to be equipped to problem solve as challenges arise. In contrast, older children and adolescents may be engaging in more structured exercise such as school sports and extra-curricular activities which may involve competition (13, 14). This planned exercise provides an opportunity for sequential review and refinement of exercise strategies. In addition to changes in patterns and types of exercise during childhood, physiological responses such as changes in insulin sensitivity with growth and pubertal development impact on glucose levels (15). Furthermore, responsibilities for diabetes management change over time, with a transfer of responsibility from parents and caregivers to the increasingly independent young person.
Exercise management for young people with T1D is complex and one approach does not fit all. Many factors influence an individual's glycemic response to exercise including the type, intensity and duration of the activity (16, 17), the amount of insulin on board (18) and the person's stress/anxiety levels (19). To further complicate management, even when all these factors are kept constant, an individual's response to exercise may (20, 21) or may not be predictable on repeated exercise occasions (22).
Diabetes should not prevent individuals from achieving their exercise goals whether these are occasional fun activities or at a more high-performance level. Indeed, many individuals with T1D have gone on to accomplish extraordinary sporting achievements (23). Due to the complexity of the many factors affecting blood glucose levels, individuals often embrace a trial and error approach to manage their blood glucose levels. This approach may be compounded by the fact that health care professionals can lack the knowledge and skills or simply don't allocate time to address these challenges in a busy clinic setting. However, challenges can be overcome with appropriate training. This has been recognized most recently through the work of the JDRF sponsored Performance in Exercise and Knowledge (PEAK) initiative (24), but this excellent source of information is not pediatric specific.
There are established international pediatric exercise guidelines for the pediatric population (25) and a comprehensive pediatric-specific review of exercise in both T1D and Type 2 diabetes by Pivovarov et al. provides current perspectives and a decision tree-based approach for blood glucose management in children with T1D (26). However, it should be noted that significant gaps in the literature exist and further research is required to address these gaps. Collaborating with patients and their families at all stages of the research process should be considered when designing studies as this approach may help uptake of findings into clinical practice (27).
Although existing pediatric and general based guidelines comprehensively review the literature around diabetes management and exercise, and make recommendations for clinical practice, a gap in the literature remains in facilitating the healthcare professional to apply these evidenced- based recommendations to an exercise consultation.
The aim of this paper is to provide a structured approach for the health care professional to use in a child/adolescent exercise consultation. The goal of this approach is to facilitate the formulation of a person-specific exercise management plan. The key to this approach is ensuring recommendations are individualized and dynamic- with the use of feedback plans that are revisited and refined. A brief review of physiology is followed by the strategies or “exercise tools” commonly used in clinical practice. A series of targeted questions to structure the exercise consultation are then presented to enable selection of the appropriate exercise tool/s for the individual young person with T1D.
Physiology
The success of the healthcare professional in assisting patients with effective exercise plans is likely to be improved by the understanding of some of the basic physiological principles of exercise and diabetes. The physiology of exercise in T1D has been reviewed in depth elsewhere (24, 28).
In an individual without diabetes, glucose provision for exercise originates predominately from the liver as a result of increased levels of glucagon and reduced circulating levels of insulin. However, during exercise in insulin-treated people with T1D, the insulin level cannot be rapidly changed, counter regulatory hormone responses can be either blunted at times or can surge as a consequence of high intensity exercise or competition (29). These hormonal imbalances can result in hypo, hyper or euglycemia (see Figure 1), which adds challenge to the management approach to exercise and diabetes (30, 31).
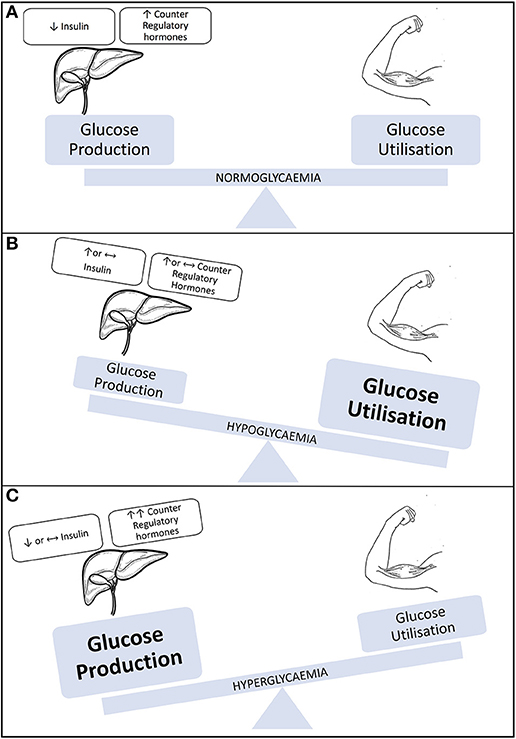
Figure 1. Illustration representing blood glucose levels during exercise. (A) Individual without type 1 diabetes/well-managed T1D. (B) Individual with T1D engaging in sustained aerobic activity. (C) Individual with T1D engaging in aerobic activity. Modified with permission from Chu et al. (31).
High individual variability exists in the blood glucose responses to different forms of exercise. In general, aerobic exercise decreases blood glucose levels, anaerobic exercise or high intensity aerobic exercise increases blood glucose levels when performed under near basal insulinemic conditions, and resistance activities are associated with relative glucose stability (32). In addition to being affected by the type, duration and intensity of the activity, individual responses are dependent on additional factors, including initial blood glucose concentrations (33), individual fitness (34), concentrations of insulin glucagon, and other counter-regulatory hormones in the circulation (35), and the nutritional status of the individual (36).
Most clinicians are familiar with the concept that insulin facilitates movement of glucose into muscle cells, but it is less commonly appreciated that muscle contraction per se is an insulin-independent mechanism that promotes glucose uptake into skeletal muscle with consequent additive increased risk of hypoglycemia (37). Increased glucose uptake into muscle can persist for hours after exercise and impact on post-exercise glycaemia (38).
Exercise Tools and Management Strategies
Creating a specific exercise management plan for a young person with T1D involves understanding their pattern of physical activity, then selecting the appropriate strategy. There are a limited number of strategies available to manage blood glucose levels before, during and after exercise. These tools include glucose monitoring, carbohydrate intake, insulin adjustment, exercise strategies, and technology based tools. The choice of strategy is made easier by understanding some basic physiology of exercise and diabetes as described above.
Glucose Monitoring
Monitoring blood glucose either by self-monitoring (SMBG) or increasingly commonly, real-time continuous glucose monitoring (rtCGM) and intermittent scanning glucose monitoring, is important for managing glycemia before, during and after exercise. Information gathered from glucose monitoring allows refinement of future exercise strategies and can inform how different factors and behaviors influence blood glucose levels. Blood glucose levels at the onset of exercise can be used to tailor glycemic management strategies.
Expert opinion suggests that although blood glucose target levels at the start of exercise should be individualized, 7–10 mmol/l is an acceptable starting range for adult patients doing aerobic exercise for up to 60-min duration (24). There are no existing guidelines for target blood glucose levels at the onset of exercise in children. However, in adults, under hyperinsulinemic conditions, the rate of fall in blood glucose levels may result in hypoglycemia if prolonged exercise is initiated within this starting range, without any adjustment to insulin dose or carbohydrate intake. Thus, in addition to starting blood glucose level, it is important to consider the rate of change of blood glucose levels to inform management decisions. Of note, although anecdotal evidence suggests a blood glucose level > 10 mmol/L may adversely affect exercise performance, studies to date have failed to demonstrate a difference in sports skill performance during acute hyperglycemia compared to normoglycemia (39, 40). Currently the evidence base is limited on whether there is an optimal blood glucose target range for exercise performance.
Continuous Glucose Monitoring (CGM) provides detailed information on not only glucose levels but trends before, during and after exercise. Information gathered from CGM can help individuals learn how different factors and behaviors influence their blood glucose levels and plan for future activities. Moreover, strategies regarding correction of hyperglycemia and prevention of hypoglycemia can be refined as information obtained from CGM could be regarded as more complete than SMBG.
What SMBG adds, as a complementary method, is an increased point accuracy as there is a lag time between blood glucose and interstitial glucose levels when glucose levels are changing rapidly, such as during exercise. This lag-time, may lead to a marked overestimation of blood glucose levels when levels are falling rapidly (41, 42). However, advancement in CGM technology has made these devices increasingly accurate (43, 44) and user-friendly. Some devices now offer the option of sharing real-time glucose levels with others or “followers”. This feature potentially allows parents/caregivers to alert their child to impending hypoglycemia during and after sports and physical activity. An observational study of 25 young people with T1D aged 8-17 years in a camp-setting showed that use of a carbohydrate intake algorithm in response to sensor glucose levels and trends prevents hypoglycemia during exercise (45).
Given that regular blood glucose monitoring provides a highly effective means to prevent hypoglycemia during and after exercise, clinicians should strongly encourage their patients not only to monitor their blood glucose levels prior to engaging in any physical activity, but also to exercise only if their glucose monitors and enough test strips are readily available or near reach (unless on CGM), particularly for situations where carrying these devices might be impractical (e.g., contact sports, swimming). Finally, clinicians should encourage their patients to measure their blood glucose levels during as well as early and for several hours after exercise, and remind them that CGM tends to overestimate blood glucose levels when they are rapidly falling.
Carbohydrate Intake
Carbohydrate consumption before, during and after exercise can be used to prevent and treat exercise -mediated hypoglycemia (46). Factors influencing the amount of carbohydrate intake required to prevent exercise-mediated hypoglycemia include body mass, circulating insulin levels and the type, intensity and duration of exercise. The blood glucose level and trend at the start of exercise are other factors to consider and recommendations based on these parameters should be individualized.
The carbohydrate requirement to prevent exercise-mediated hypoglycemia increases with plasma insulin levels (47), with the pattern of blood glucose response to exercise being highly unpredictable under hyperinsulinaemic compared to near basal insulinaemic conditions (22).
If exercise is occurring when circulating insulin levels are high, such as within 3 h of a meal-time insulin bolus, then up to 1.0–1.5 g of carbohydrate/kg ideal body weight/ hour of sustained activity may be required (48, 49). In contrast, when exercise is taking place several hours after a meal-time bolus, or when the insulin bolus dose has been lowered pre-exercise, then carbohydrate requirements are lower (~0.3–0.5 g/kg ideal body mass/h (47, 49). When insulin levels are close to basal levels, such as when exercise is performed before breakfast, the risk of hypoglycemia is minimal (50) and carbohydrate supplementation may not be required (51, 52). Given that categorizing carbohydrate requirements in a dichotomous manner (high vs. low insulin levels) is a simplified approach that does not take into account all the factors influencing glucose requirements, Francesco et al have developed an algorithm that estimates an individuals' glucose requirement during activity based on personalized situation specific information including insulin concentration (53).
In addition to being affected by insulin levels, the amount of carbohydrate to prevent exercise mediated hypoglycemia varies with the intensity and duration of exercise (18). A study in young people with T1D exercising under basal insulin levels reported that glucose requirements to maintain euglycemia during 40 min of exercise increase with intensity up to 50% and 65% VO2 peak, but with no glucose required at 80% VO2 peak (54). It remains to be established if this pattern is still observed when exercise is performed for a longer duration or under high circulating insulin levels.
The type and timing of carbohydrate ingestion should also be considered. Carbohydrates with a high glycemic Index (GI) such as glucose in liquid, tablet, and gel forms, are digested and absorbed more quickly, resulting in a rapid rise in blood glucose levels. In contrast, low GI foods, including fruits and wholemeal bread, are released more slowly causing a gradual and sustained rise in glycemia (55, 56).
A meal or snack containing low GI carbohydrate 1–4 h prior to exercise can increase hepatic glycogen stores and provide sustained carbohydrate release during exercise (57, 58). In contrast, high GI carbohydrates are preferable immediately prior to and during prolonged exercise (59). High GI snacks are also recommended in early recovery (1–2 h post exercise) to replenish glycogen stores (60) and to avoid hypoglycemia in this period of heightened insulin sensitivity. A bedtime snack containing carbohydrate, fat and protein may help reduce the risk of hypoglycemia on nights following exercise (61).
Clinical recommendations for carbohydrate intake will vary if the goal is hypoglycemia prevention, weight reduction, improving glycaemic control or optimal exercise performance. Since high carbohydrate intake is often recommended for healthy individuals without diabetes before and during prolonged exercise to optimize endurance performance (60), this strategy has recently been explored in adults with T1D (62), The authors report that increased carbohydrate supplementation, matched with increased insulin doses, is safe and allows the prevention of hypoglycemia during prolonged aerobic activity (62). This strategy has not been explored in the pediatric population. It is important to match insulin dose with extra CHO intake as excessive carbohydrate supplementation without matched insulin may result in hyperglycemia (63).
Insulin Adjustment
Insulin adjustment, along with balanced carbohydrate intake, is a key tool for managing blood glucose levels during and after exercise. The degree to which blood glucose levels fall during exercise is dependent on the amount of circulating insulin (47). Reduction in insulin doses to prevent exercise-mediated hypoglycemia is typically required for prolonged (>30 min) moderate intensity exercise, particularly if insulin is above basal levels (18, 64).
Options for insulin adjustment vary depending on insulin regimen. Insulin pumps allow greater flexibility in adjusting basal rates than injection regimens. Therefore, basal dose adjustments are most relevant to patients on insulin pumps, particularly for unplanned exercise, whereas bolus dose adjustment can be applied to most regimens. For those on Multiple Daily Injections (MDI), basal dose reduction can reduce the risk of nocturnal hypoglycemia on nights following afternoon exercise (25). However, this approach may result in hyperglycemia, and if this is the case, should be reserved for when participating in more activity than usual, such as sports camps.
Exercise-Based Strategies
Utilization of the glycemic effects of specific types of exercise can play a role in managing blood glucose levels. The blood glucose rising effect of sprinting in young people with T1D suggests that this may provide a strategy to reduce hypoglycemia risk during and after exercise (65). Indeed, a series of studies in young adults with T1D have shown that a maximal 10 s sprint performed before (66) or after (67) moderate intensity exercise can prevent blood glucose levels from falling early after exercise. Furthermore, frequent short sprints (4 s sprints every 2 min) during moderate intensity exercise reduce the decline in blood glucose levels compared to continuous moderate intensity exercise during and early after exercise (68, 69). These strategies have been trialed in clinic-based studies, but real-world data are lacking.
In contrast, exercise induced hyperglycemia can be managed with moderate intensity exercise. Anecdotal evidence in adults suggests that moderate intensity exercise can be used as a strategy to counteract exercise-induced hyperglycemia, a technique known as an “aerobic cool down” (70). The mechanisms underlying this technique remain to be well-understood, they may include increased glucose (71) and lactate oxidation. Lactate is a substrate for gluconeogenesis and would potentially otherwise be converted to glucose in the liver resulting in hyperglycemia (72, 73).
Advances in Diabetes Technology
Diabetes technology is rapidly evolving and provides further tools to aid glucose management and reduce the burden of diabetes during exercise (74). Technology based tools include smart phone applications, insulin pumps, CGM, sensor augmented pump therapy and closed loop technology. Smart phone applications can help individuals to record exercise management and aid exercise management decisions (75). Insulin pumps enable greater flexibility in insulin adjustment as basal rates can be reduced or stopped before during or after exercise to prevent exercise mediated hypoglycemia. Despite some issues with sensor accuracy at times of rapid glucose flux, CGM can provide comprehensive dynamic real-time glucose information around exercise to help inform diabetes management decisions.
Perhaps the biggest game changer in current diabetes management is the ability to link sensor glucose readings to insulin delivery in sensor augmented and closed loop pump therapy. Sensor augmented pump (SAP) therapy includes low glucose suspend and predictive low glucose suspend technology, where a mathematical algorithm suspends basal insulin delivery when sensor glucose levels fall below, or are predicted to fall below a pre-set threshold. Studies investigating the use of low glucose suspend and predictive low glucose suspend technology during exercise have shown a reduction in hypoglycemia (21, 76).
Closed loop systems expand on the concept of SAP therapy by using a control algorithm that continually increases or decreases hormone delivery in response to sensor glucose levels. Systems include dual hormone (insulin and glucagon) and single hormone (insulin only) pumps. Closed loop technology may be seen as the future of diabetes management, however physical activity remains one of the biggest challenges to fully automated systems (77). This is because physical activity in individuals with T1D induces rapid changes in glucose levels due to hormone imbalances that are dependent on the type, duration and intensity of the activity as well as individual factors such as fitness levels and the amount of circulating insulin on board. Furthermore, the glucose trends during physical activity may be variable within and between individuals. Further research is needed to guide exercise recommendations in patients using the current closed loop systems
For closed loop systems to be effective at this time of rapid and potentially unpredictable glucose fluctuations, several challenges must be overcome including sensor glucose lag-time (as previously discussed), pharmacokinetics of subcutaneous insulin administration and how to sense not only that physical activity is occurring but the intensity of the activity taking place (77).
In an individual without T1D, insulin levels rapidly decrease at the onset or exercise. This is difficult to replicate in T1D as insulin is administered subcutaneously and levels are not under endogenous control. Furthermore, absorption of insulin may be increased from stores of already administered subcutaneous insulin during exercise due to increased blood flow to the working muscle (78). Bihormonal pumps infusing both glucagon and insulin may help overcome this challenge (79).
Closed loop systems may be better able to manage blood glucose levels during exercise if the system could “sense” the intensity and duration of the physical activity. Future developments may include integration of physical activity monitoring such as heart rate and or accelerometery data into closed loop systems.
A Structured Series of Questions for the Exercise Consultation
A structured approach to exercise management in young people with Type 1 diabetes involving targeted and clinically relevant questions can provide the framework for an effective consultation and aid selection of the most appropriate tools as described above. We recommend starting from the center of Figure 2 and working outwards. Some health care professionals may find the use of a consistent template that addresses each of the 5 questions helpful. These questions can be re-visited in sequential consultations together with information from glucose monitoring to refine recommendations.
Q1. What Are the Individual's Exercise and Glycemic Goals?
The first step in formulating a patient's exercise management plan involves identifying the patient's exercise goal, as this will influence subsequent management decisions. Exercise goals may include: weight loss, exercise performance, socialization and fitness. Glycemic goals in addition to hypoglycemia prevention may include, maintaining blood glucose levels in target range, or achieving a specific blood glucose level to maximize exercise performance.
If the exercise goal is facilitating weight loss, it would be most appropriate to choose an insulin-reduction based strategy to manage blood glucose level during and after exercise so as to avoid excessive CHO intake particularly after exercise. If socialization and fun is the goal, then insulin dose reduction may be key to minimize time spent out of the activity to test blood glucose level and ingest CHO. In contrast, if the primary goal is exercise performance, then an individual may be aiming for increasing CHO intake before (e.g., CHO loading) and during exercise to maximize performance while managing their blood glucose levels so as to minimize or avoid any interruptions.
Strategies for exercise performance may focus on matching carbohydrate intake to the specific nutritional requirement of the activity (rather than hypoglycemia prevention) and insulin doses should be increased accordingly (62). If fitness is the goal, and the patient has a plan to incrementally increase physical activity over time, it will be important to anticipate a reduction in total daily insulin requirement to avoid hypoglycemia as insulin sensitivity increases in response to exercise training.
Q2. What Is the Exercise Type, Duration and Intensity?
The next step is to consider, the type, duration and intensity of the physical activity. This will allow anticipation of the expected effect on glucose levels (24). As mentioned above, continuous aerobic activity performed when insulin levels are high will result in a decrease in blood glucose levels and require a reduction in insulin and/or an increase in carbohydrate supplementation to avoid hypoglycemia. In contrast, short duration high intensity aerobic or anaerobic activity, a pattern typical of children's natural play (80), may maintain glucose levels during exercise and even result in early post-exercise hyperglycemia (16, 81) if performed while plasma insulin is close to basal level. Competition-induced anticipatory stress may also raise blood glucose levels as a result of elevated catecholamine levels.
Exercise-related hyperglycemia may require a conservative insulin correction dose (50% of usual dose) administered immediately after exercise (82). Alternatively, an aerobic cool-down session can be used to counteract post-exercise hyperglycemia (70). Since such a use of insulin or exercise as a means to oppose hyperglycemia post-exercise may increase the risk of hypoglycemia if the insulin dose or the duration of the cool-down session is excessive these strategies should be adopted only if accompanied by regular blood glucose monitoring.
Q3. Timing of Exercise Relative to the Last Insulin Bolus Dose?
Establishing the timing of exercise in relation to the last insulin bolus provides insight on relative insulin levels and will guide decisions on insulin adjustment, in particular if reductions should be made to basal or bolus insulin doses.
When exercise is planned to take place within 3 h of administration of an insulin bolus (high insulin levels), then a bolus dose reduction of 25-75% can be trialed (18, 83) It should be noted that the smaller the insulin dose administered, the shorter its duration of action (84). Recommendations for bolus reduction should be relative to the duration and intensity of the exercise (24). Alternatively, or in combination with insulin adjustment, pre-exercise carbohydrate intake to up to 1.0–1.5 g of carbohydrate/kg ideal body weight/ hour of sustained activity (85) may be required as discussed above.
Exercise performed when plasma insulin is close to basal levels (e.g., in the morning prior to breakfast) is less likely to result in hypoglycemia as circulating insulin levels are typically low. If hypoglycemia is occurring in this setting then management options include: carbohydrate consumption or reduction of basal insulin. Carbohydrate intake to prevent hypoglycemia when insulin levels are low is approximately 0.3-0.5 g/kg ideal body mass/hr; (47, 49). Reduction of basal insulin is more easily achieved for individuals on pump regimens and recommendations include a temporary basal rate reduction of 50-80% (64). To be effective, basal rates should ideally be reduced 60–90 min prior to the onset of activity. Other strategies to consider include use of automated basal insulin suspension functions during and after exercise, including: low glucose suspend (76) and predictive low glucose suspend technology (21).
Late onset post exercise hypoglycemia is the phenomenon of overnight hypoglycemia particularly occurring after late afternoon exercise (38, 86). A basal reduction; for those on insulin pumps a basal rate reduction of 20% for 6 h (87), or for those on MDI a 20% basal dose reduction in combination with a carbohydrate snack at bedtime (25), can reduce the incidence of nocturnal hypoglycemia in response to a prior bout of afternoon exercise.
Q4. Is the Exercise Planned or Spontaneous?
It is helpful to ascertain if the individual engages in mostly planned or spontaneous physical activity. If exercise is planned, then strategies based on insulin adjustment with or without carbohydrate supplementation can be implemented (see above). Furthermore, exploring if planned events involve the same type of activity at the same time of day will help establish if glycemic patterns are reproducible for that individual on different occasions when conditions are similar. If exercise is spontaneous and pre-exercise adjustment of bolus and basal insulin are not available in advance, then the options available are limited to basal insulin reduction, carbohydrate supplementation or use of glycemia-rising high intensity exercise (67, 81).
Q5. Have There Been any Episodes of Hypoglycemia and /or Exercise Prior to the Exercise Session?
When reviewing the glycemic response to a period of exercise it is helpful to find out if there have been any episodes of hypoglycemia or exercise prior to the exercise event. Both preceding exercise (88) and hypoglycemia (89) can attenuate the counter-regulatory response to subsequent exercise thereby increasing the risk of hypoglycemia.
The pattern and frequency of prior exercise is informative. Regular participation in aerobic activity increases insulin sensitivity and may consequently lower the total daily insulin requirement (90, 91). Therefore, if exercise is occurring on a day to day basis, further insulin dose reduction may be required.
Conclusion
It can be challenging for health care practitioners to advise young people with T1D how to approach exercise safely. Clinical guidelines and a recent comprehensive consensus statement on exercise and T1D provide thorough evidence-based recommendations. This paper aims to facilitate the healthcare professional in applying such evidence-based recommendations to clinical practice using a structured approach to the exercise consultation based on a framework of relevant questions. The tools or management strategies are provided as a starting base to help clinicians work with patients and families to achieve their exercise goals. This framework of questions together with information from blood glucose monitoring should be re-visited at sequential consultations to allow refinement of future exercise management strategies.
Author Contributions
TC was responsible for the manuscript preparation. VS, PF, PA, TJ, and ED reviewed and edited the manuscript. All authors approved the final version of this article.
Conflict of Interest Statement
The authors declare that the research was conducted in the absence of any commercial or financial relationships that could be construed as a potential conflict of interest.
Acknowledgments
We would like to thank the children and families attending the Children's Diabetes Centre in Perth, Western Australia.
Abbreviations
T1D, Type 1 Diabetes; SMBG, Self-monitoring blood glucose; CGM, Continuous glucose monitoring; rtCGM, Real-time continuous glucose monitoring; SAP, Sensor Augmented Pump.
References
1. Brouwer SI, Stolk RP, Liem ET, Lemmink KA, Corpeleijn E. The role of fitness in the association between fatness and cardiometabolic risk from childhood to adolescence. Pediatr Diab. (2013) 14:57–65. doi: 10.1111/j.1399-5448.2012.00893.x
2. Burrows M. Exercise and bone mineral accrual in children and adolescents. J Sports Sci Med. (2007) 6:305–12.
3. Dunton GF, Huh J, Leventhal AM, Riggs N, Hedeker D, Spruijt-Metz D, et al. Momentary assessment of affect, physical feeling states, and physical activity in children. Health Psychol. (2014) 33:255–63. doi: 10.1037/a0032640
4. Esteban-Cornejo I, Tejero-Gonzalez CM, Martinez-Gomez D, del-Campo J, Gonzalez-Galo A, Padilla-Moledo C, et al. Independent and combined influence of the components of physical fitness on academic performance in youth. J Pediatr. (2014) 165:306–12.e2. doi: 10.1016/j.jpeds.2014.04.044
5. Physical Activity Guidelines Advisory Committee report 2008. To the secretary of health and human services. Part A: executive summary. Nutr Rev. (2009) 67:114–20. doi: 10.1111/j.1753-4887.2008.00136.x
6. Lascar N, Kennedy A, Hancock B, Jenkins D, Andrews RC, Greenfield S, et al. Attitudes and barriers to exercise in adults with type 1 diabetes (T1DM) and how best to address them: a qualitative study. PLoS ONE. (2014) 9:e108019. doi: 10.1371/journal.pone.0108019
7. Brazeau AS, Mircescu H, Desjardins K, Dube MC, Weisnagel SJ, Lavoie C, et al. The barriers to physical activity in Type 1 diabetes (BAPAD-1) scale: predictive validity and reliability. Diab Metabol. (2012) 38:164–70. doi: 10.1016/j.diabet.2011.10.005
8. Van Name MA, Hilliard ME, Boyle CT, Miller KM, DeSalvo DJ, Anderson BJ, et al. Nighttime is the worst time: parental fear of hypoglycemia in young children with type 1 diabetes. Pediatr Diabet. (2017) 19:114–20 doi: 10.1111/pedi.12525
9. MacMillan F, Kirk A, Mutrie N, Matthews L, Robertson K, Saunders DH. A systematic review of physical activity and sedentary behavior intervention studies in youth with type 1 diabetes: study characteristics, intervention design, and efficacy. Pediatr Diab. (2014) 15:175–89. doi: 10.1111/pedi.12060
10. Salem MA, Aboelasrar MA, Elbarbary NS, Elhilaly RA, Refaat YM. Is exercise a therapeutic tool for improvement of cardiovascular risk factors in adolescents with type 1 diabetes mellitus? A randomised controlled trial. Diabetol Metabol Syndr. (2010) 2:47. doi: 10.1186/1758-5996-2-47
11. Seeger JP, Thijssen DH, Noordam K, Cranen ME, Hopman MT, Nijhuis-van der Sanden MW. Exercise training improves physical fitness and vascular function in children with type 1 diabetes. Diab Obes Metabol. (2011) 13:382–4. doi: 10.1111/j.1463-1326.2011.01361.x
12. Brasholt M, Chawes B, Kreiner-Moller E, Vahlkvist S, Sinding M, Bisgaard H. Objective assessment of levels and patterns of physical activity in preschool children. Pediatr Res. (2013) 74:333–8. doi: 10.1038/pr.2013.99
13. Sera F, Griffiths LJ, Dezateux C, Geraci M, Cortina-Borja M. Using functional data analysis to understand daily activity levels and patterns in primary school-aged children: cross-sectional analysis of a UK-wide study. PLoS ONE. (2017) 12:e0187677. doi: 10.1371/journal.pone.0187677
14. Sigmund E, De Ste Croix M, Miklankova L, Fromel K. Physical activity patterns of kindergarten children in comparison to teenagers and young adults. Eur J Publ Health. (2007) 17:646–51. doi: 10.1093/eurpub/ckm033
15. Amiel SA, Sherwin RS, Simonson DC, Lauritano AA, Tamborlane WV. Impaired insulin action in puberty. A contributing factor to poor glycemic control in adolescents with diabetes. N Engl J Med. (1986) 315:215–9. doi: 10.1056/NEJM198607243150402
16. Bally L, Zueger T, Buehler T, Dokumaci AS, Speck C, Pasi N, et al. Metabolic and hormonal response to intermittent high-intensity and continuous moderate intensity exercise in individuals with type 1 diabetes: a randomised crossover study. Diabetologia. (2016) 59:776–84. doi: 10.1007/s00125-015-3854-7
17. Tonoli C, Heyman E, Roelands B, Buyse L, Cheung SS, Berthoin S, et al. Effects of different types of acute and chronic (training) exercise on glycaemic control in type 1 diabetes mellitus: a meta-analysis. Sports Med. (2012) 42:1059–80. doi: 10.1007/BF03262312
18. Rabasa-Lhoret R, Bourque J, Ducros F, Chiasson JL. Guidelines for premeal insulin dose reduction for postprandial exercise of different intensities and durations in type 1 diabetic subjects treated intensively with a basal-bolus insulin regimen (ultralente-lispro). Diabetes Care. (2001) 24:625–30. doi: 10.2337/diacare.24.4.625
19. Kreisman SH, Halter JB, Vranic M, Marliss EB. Combined infusion of epinephrine and norepinephrine during moderate exercise reproduces the glucoregulatory response of intense exercise. Diabetes. (2003) 52:1347–54. doi: 10.2337/diabetes.52.6.1347
20. Temple MY, Bar-Or O, Riddell MC. The reliability and repeatability of the blood glucose response to prolonged exercise in adolescent boys with IDDM. Diabetes Care. (1995) 18:326–32. doi: 10.2337/diacare.18.3.326
21. Abraham MB, Davey R, O'Grady MJ, Ly TT, Paramalingam N, Fournier PA, et al. Effectiveness of a predictive algorithm in the prevention of exercise-induced hypoglycemia in type 1 diabetes. Diab Technol Therapeut. (2016) 18:543–50. doi: 10.1089/dia.2016.0141
22. Biankin SA, Jenkins AB, Campbell LV, Choi KL, Forrest QG, Chisholm DJ. Target-seeking behavior of plasma glucose with exercise in type 1 diabetes. Diabetes Care. (2003) 26:297–301. doi: 10.2337/diacare.26.2.297
23. Kordonouri O, Vazeou A, Scharf M, Wursig M, Battelino T. Striving for control: lessons learned from a successful international Type 1 Diabetes Youth Challenge. Acta Diabetol. (2017) 54:403–9. doi: 10.1007/s00592-017-0964-3
24. Riddell MC, Gallen IW, Smart CE, Taplin CE, Adolfsson P, Lumb AN, et al. Exercise management in type 1 diabetes: a consensus statement. Lancet Diab Endocrinol. (2017) 5:377-390 doi: 10.1016/S2213-8587(17)30014-1
25. Adolfsson P, Riddell MC, Taplin CE, Davis EA, Fournier PA, Annan F, et al. ISPAD Clinical Practice Consensus Guidelines 2018: exercise in children and adolescents with diabetes. Pediatr Diab. (2018) 19 (Suppl 27):205–26. doi: 10.1111/pedi.12755
26. Pivovarov JA, Taplin CE, Riddell MC. Current perspectives on physical activity and exercise for youth with diabetes. Pediatr Diab. (2015) 16:242–55. doi: 10.1111/pedi.12272
27. Klaprat N, MacIntosh A, McGavock JM. Gaps in knowledge and the need for patient-partners in research related to physical activity and type 1 diabetes: a narrative review. Front Endocrinol. (2019) 10:42. doi: 10.3389/fendo.2019.00042
28. Galassetti P, Riddell MC. Exercise and type 1 diabetes (T1DM). Compr Physiol. (2013) 3:1309–36. doi: 10.1002/cphy.c110040
29. Camacho RC, Galassetti P, Davis SN, Wasserman DH. Glucoregulation during and after exercise in health and insulin-dependent diabetes. Exerc Sport Sci Rev. (2005) 33:17–23.
30. Riddell MC, Iscoe KE. Physical activity, sport, and pediatric diabetes. Pediatr Diab. (2006) 7:60–70. doi: 10.1111/j.1399-543X.2006.00146.x
31. Chu L, Hamilton J, Riddell MC. Clinical management of the physically active patient with type 1 diabetes. Phys Sports Med. (2011) 39:64–77. doi: 10.3810/psm.2011.05.1896
32. Yardley JE, Sigal RJ, Perkins BA, Riddell MC, Kenny GP. Resistance exercise in type 1 diabetes. Can J Diab. (2013) 37:420–6. doi: 10.1016/j.jcjd.2013.07.020
33. Garcia-Garcia F, Kumareswaran K, Hovorka R, Hernando ME. Quantifying the acute changes in glucose with exercise in type 1 diabetes: a systematic review and meta-analysis. Sports Med. (2015) 45:587–99. doi: 10.1007/s40279-015-0302-2
34. Al Khalifah RA, Suppere C, Haidar A, Rabasa-Lhoret R, Ladouceur M, Legault L. Association of aerobic fitness level with exercise-induced hypoglycaemia in Type 1 diabetes. Diabetic Med. (2016) 33:1686–90. doi: 10.1111/dme.13070
35. Coker RH, Kjaer M. Glucoregulation during exercise : the role of the neuroendocrine system. Sports Med. (2005) 35:575–83. doi: 10.2165/00007256-200535070-00003
36. Burke LM, Hawley JA, Angus DJ, Cox GR, Clark SA, Cummings NK, et al. Adaptations to short-term high-fat diet persist during exercise despite high carbohydrate availability. Med Sci Sports Exer. (2002) 34:83–91. doi: 10.1097/00005768-200201000-00014
37. Jessen N, Goodyear LJ. Contraction signaling to glucose transport in skeletal muscle. J Appl Physiol. (2005) 99:330–7. doi: 10.1152/japplphysiol.00175.2005
38. McMahon SK, Ferreira LD, Ratnam N, Davey RJ, Youngs LM, Davis EA, et al. Glucose requirements to maintain euglycemia after moderate-intensity afternoon exercise in adolescents with type 1 diabetes are increased in a biphasic manner. J Clin Endocrinol Metabol. (2007) 92:963–8. doi: 10.1210/jc.2006-2263
39. Stettler C, Jenni S, Allemann S, Steiner R, Hoppeler H, Trepp R, et al. Exercise capacity in subjects with type 1 diabetes mellitus in eu- and hyperglycaemia. Diab Metabol Res Rev. (2006) 22:300–6. doi: 10.1002/dmrr.608
40. Kelly D, Hamilton JK, Riddell MC. Blood glucose levels and performance in a sports cAMP for adolescents with type 1 diabetes mellitus: a field study. Int J Pediatr. (2010) 2010:216167. doi: 10.1155/2010/216167
41. Taleb N, Emami A, Suppere C, Messier V, Legault L, Chiasson JL, et al. Comparison of two continuous glucose monitoring systems, dexcom G4 platinum and medtronic paradigm veo enlite system, at rest and during exercise. Diab Technol Therap. (2016) 18:561–7. doi: 10.1089/dia.2015.0394
42. Davey RJ, Low C, Jones TW, Fournier PA. Contribution of an intrinsic lag of continuous glucose monitoring systems to differences in measured and actual glucose concentrations changing at variable rates in vitro. J Diab Sci Technol. (2010) 4:1393-9. doi: 10.1177/193229681000400614
43. Yardley JE, Sigal RJ, Kenny GP, Riddell MC, Lovblom LE, Perkins BA. Point accuracy of interstitial continuous glucose monitoring during exercise in type 1 diabetes. Diab Technol Therapeut. (2013) 15:46-9. doi: 10.1089/dia.2012.0182
44. Bally L, Zueger T, Pasi N, Carlos C, Paganini D, Stettler C. Accuracy of continuous glucose monitoring during differing exercise conditions. Diab Res Clin Pract. (2016) 112:1–5. doi: 10.1016/j.diabres.2015.11.012
45. Riddell MC, Milliken J. Preventing exercise-induced hypoglycemia in type 1 diabetes using real-time continuous glucose monitoring and a new carbohydrate intake algorithm: an observational field study. Diab Technol Therapeut. (2011) 13:819-25. doi: 10.1089/dia.2011.0052
46. Dube MC, Lavoie C, Galibois I, Weisnagel SJ. Nutritional strategies to prevent hypoglycemia at exercise in diabetic adolescents. Med Sci Sports Exerc. (2012) 44:1427–32. doi: 10.1249/MSS.0b013e3182500a35
47. Francescato MP, Geat M, Fusi S, Stupar G, Noacco C, Cattin L. Carbohydrate requirement and insulin concentration during moderate exercise in type 1 diabetic patients. Metabolism. (2004) 53:1126–30. doi: 10.1016/j.metabol.2004.03.015
48. Riddell MC, Bar-Or O, Hollidge-Horvat M, Schwarcz HP, Heigenhauser GJ. Glucose ingestion and substrate utilization during exercise in boys with IDDM. J Appl Physiol. (2000) 88:1239–46. doi: 10.1152/jappl.2000.88.4.1239
49. Robertson K, Adolfsson P, Scheiner G, Hanas R, Riddell MC. Exercise in children and adolescents with diabetes. Pediatr Diab. (2009) 10 (Suppl 12):154–68. doi: 10.1111/j.1399-5448.2009.00567.x
50. Ruegemer JJ, Squires RW, Marsh HM, Haymond MW, Cryer PE, Rizza RA, et al. Differences between prebreakfast and late afternoon glycemic responses to exercise in IDDM patients. Diabetes Care. (1990) 13:104–10. doi: 10.2337/diacare.13.2.104
51. Soo K, Furler SM, Samaras K, Jenkins AB, Campbell LV, Chisholm DJ. Glycemic responses to exercise in IDDM after simple and complex carbohydrate supplementation. Diabetes Care. (1996) 19:575–9. doi: 10.2337/diacare.19.6.575
52. Nathan DM, Madnek SF, Delahanty L. Programming pre-exercise snacks to prevent post-exercise hypoglycemia in intensively treated insulin-dependent diabetics. Ann Int Med. (1985) 102:483–6. doi: 10.7326/0003-4819-102-4-483
53. Francescato MP, Ajcevic M, Accardo A. Carbohydrate requirement for exercise in Type 1 diabetes: effects of insulin concentration. J Diab Sci Technol. (2019) 2019:1932296819826962. doi: 10.1177/1932296819826962
54. Shetty VB, Fournier PA, Davey RJ, Retterath AJ, Paramalingam N, Roby HC, et al. Effect of exercise intensity on glucose requirements to maintain euglycemia during exercise in type 1 diabetes. J Clin Endocrinol Metabol. (2016) 101:972–80. doi: 10.1210/jc.2015-4026
55. Asp NG. Nutritional classification and analysis of food carbohydrates. Am J Clin Nutr. (1994) 59(3 Suppl):679s–81s. doi: 10.1093/ajcn/59.3.679Sa
56. Foster-Powell K, Holt SH, Brand-Miller JC. International table of glycemic index and glycemic load values: 2002. Am J Clin Nutr. (2002) 76:5–56. doi: 10.1093/ajcn/76.1.5
57. Bracken RM, Page R, Gray B, Kilduff LP, West DJ, Stephens JW, et al. Isomaltulose improves glycemia and maintains run performance in type 1 diabetes. Med Sci Sports Exer. (2012) 44:800–8. doi: 10.1249/MSS.0b013e31823f6557
58. West DJ, Morton RD, Stephens JW, Bain SC, Kilduff LP, Luzio S, et al. Isomaltulose improves postexercise glycemia by reducing CHO oxidation in T1DM. Med Sci Sports Exer. (2011) 43:204–10. doi: 10.1249/MSS.0b013e3181eb6147
59. Grimm JJ, Ybarra J, Berne C, Muchnick S, Golay A. A new table for prevention of hypoglycaemia during physical activity in type 1 diabetic patients. Diab Metabol. (2004) 30:465-70. doi: 10.1016/S1262-3636(07)70144-1
60. Thomas DT, Erdman KA, Burke LM. American college of sports medicine joint position statement. nutrition and athletic performance. Med Sci Sports Exer. (2016) 48:543–68. doi: 10.1249/MSS.0000000000000852
61. Kalergis M, Schiffrin A, Gougeon R, Jones PJ, Yale JF. Impact of bedtime snack composition on prevention of nocturnal hypoglycemia in adults with type 1 diabetes undergoing intensive insulin management using lispro insulin before meals: a randomized, placebo-controlled, crossover trial. Diabetes Care. (2003) 26:9–15. doi: 10.2337/diacare.26.1.9
62. Adolfsson P, Mattsson S, Jendle J. Evaluation of glucose control when a new strategy of increased carbohydrate supply is implemented during prolonged physical exercise in type 1 diabetes. Eur J Appl Physiol. (2015) 115:2599–607. doi: 10.1007/s00421-015-3251-4
63. Francescato MP, Stel G, Stenner E, Geat M. Prolonged exercise in type 1 diabetes: performance of a customizable algorithm to estimate the carbohydrate supplements to minimize glycemic imbalances. PLoS ONE. (2015) 10:e0125220. doi: 10.1371/journal.pone.0125220
64. Franc S, Daoudi A, Pochat A, Petit MH, Randazzo C, Petit C, et al. Insulin-based strategies to prevent hypoglycaemia during and after exercise in adult patients with type 1 diabetes on pump therapy: the DIABRASPORT randomized study. Diab Obes Metabol. (2015) 17:1150–7. doi: 10.1111/dom.12552
65. Yardley JE, Sigal RJ. Exercise strategies for hypoglycemia prevention in individuals with type 1 diabetes. DiabSpectr. (2015) 28:32–8. doi: 10.2337/diaspect.28.1.32
66. Bussau VA, Ferreira LD, Jones TW, Fournier PA. A 10-s sprint performed prior to moderate-intensity exercise prevents early post-exercise fall in glycaemia in individuals with type 1 diabetes. Diabetologia. (2007) 50:1815–8. doi: 10.1007/s00125-007-0727-8
67. Bussau VA, Ferreira LD, Jones TW, Fournier PA. The 10-s maximal sprint: a novel approach to counter an exercise-mediated fall in glycemia in individuals with type 1 diabetes. Diabetes Care. (2006) 29:601–6. doi: 10.2337/diacare.29.03.06.dc05-1764
68. Guelfi KJ, Jones TW, Fournier PA. The decline in blood glucose levels is less with intermittent high-intensity compared with moderate exercise in individuals with type 1 diabetes. Diabetes Care. (2005) 28:1289–94. doi: 10.2337/diacare.28.6.1289
69. Guelfi KJ, Jones TW, Fournier PA. Intermittent high-intensity exercise does not increase the risk of early postexercise hypoglycemia in individuals with type 1 diabetes. Diabetes Care. (2005) 28:416–8. doi: 10.2337/diacare.28.2.416
70. Colberg SR, Sigal RJ, Yardley JE, Riddell MC, Dunstan DW, Dempsey PC, et al. Physical activity/exercise and diabetes: a position statement of the american diabetes association. Diabetes Care. (2016) 39:2065–79. doi: 10.2337/dc16-1728
71. Jenni S, Oetliker C, Allemann S, Ith M, Tappy L, Wuerth S, et al. Fuel metabolism during exercise in euglycaemia and hyperglycaemia in patients with type 1 diabetes mellitus–a prospective single-blinded randomised crossover trial. Diabetologia. (2008) 51:1457–65. doi: 10.1007/s00125-008-1045-5
72. Adeva-Andany M, Lopez-Ojen M, Funcasta-Calderon R, Ameneiros-Rodriguez E, Donapetry-Garcia C, Vila-Altesor M, et al. Comprehensive review on lactate metabolism in human health. Mitochondrion. (2014) 17:76–100. doi: 10.1016/j.mito.2014.05.007
73. Miller BF, Fattor JA, Jacobs KA, Horning MA, Navazio F, Lindinger MI, et al. Lactate and glucose interactions during rest and exercise in men: effect of exogenous lactate infusion. J Physiol. (2002) 544(Pt 3):963–75. doi: 10.1113/jphysiol.2002.027128
74. Tagougui S, Taleb N, Rabasa-Lhoret R. The benefits and limits of technological advances in glucose management around physical activity in patients type 1 diabetes. Front Endocrinol. (2018) 9:818. doi: 10.3389/fendo.2018.00818
75. Groat D, Soni H, Grando MA, Thompson B, Kaufman D, Cook CB. Design and testing of a smartphone application for real-time self-tracking diabetes self-management behaviors. Appl Clin Informat. (2018) 9:440–9. doi: 10.1055/s-0038-1660438
76. Brazg RL, Bailey TS, Garg S, Buckingham BA, Slover RH, Klonoff DC, et al. The ASPIRE study: design and methods of an in-clinic crossover trial on the efficacy of automatic insulin pump suspension in exercise-induced hypoglycemia. J Diab Sci Technol. (2011) 5:1466–71. doi: 10.1177/193229681100500621
77. Riddell MC, Zaharieva DP, Yavelberg L, Cinar A, Jamnik VK. Exercise and the development of the artificial pancreas: one of the more difficult series of hurdles. J Diab Sci Technol. (2015) 9:1217–26. doi: 10.1177/1932296815609370
78. Koivisto VA, Felig P. Effects of leg exercise on insulin absorption in diabetic patients. N Engl J Med. (1978) 298:79–83. doi: 10.1056/NEJM197801122980205
79. Taleb N, Emami A, Suppere C, Messier V, Legault L, Ladouceur M, et al. Efficacy of single-hormone and dual-hormone artificial pancreas during continuous and interval exercise in adult patients with type 1 diabetes: randomised controlled crossover trial. Diabetologia. (2016) 59:2561–71. doi: 10.1007/s00125-016-4107-0
80. Bailey RC, Olson J, Pepper SL, Porszasz J, Barstow TJ, Cooper DM. The level and tempo of children's physical activities: an observational study. Med Sci Sports Exer. (1995) 27:1033–41. doi: 10.1249/00005768-199507000-00012
81. Marliss EB, Vranic M. Intense exercise has unique effects on both insulin release and its roles in glucoregulation: implications for diabetes. Diabetes. (2002) 51 (Suppl 1):S271–83. doi: 10.2337/diabetes.51.2007.S271
82. Turner D, Luzio S, Gray BJ, Bain SC, Hanley S, Richards A, et al. Algorithm that delivers an individualized rapid-acting insulin dose after morning resistance exercise counters post-exercise hyperglycaemia in people with Type 1 diabetes. Diabetic Med. (2016) 33:506–10. doi: 10.1111/dme.12870
83. Bracken RM, West DJ, Stephens JW, Kilduff LP, Luzio S, Bain SC. Impact of pre-exercise rapid-acting insulin reductions onketogenesis following running in Type 1 diabetes. Diabetic Med. (2011) 28:218–22. doi: 10.1111/j.1464-5491.2010.03162.x
84. Nosek L, Roggen K, Heinemann L, Gottschalk C, Kaiser M, Arnolds S, et al. Insulin aspart has a shorter duration of action than human insulin over a wide dose-range. Diab Obes Metabol. (2013) 15:77–83. doi: 10.1111/j.1463-1326.2012.01677.x
85. Robertson K, Riddell MC, Guinhouya BC, Adolfsson P, Hanas R. ISPAD Clinical Practice Consensus Guidelines 2014. Exercise in children and adolescents with diabetes. Pediatr Diab. (2014) 15 (Suppl 20):203–23. doi: 10.1111/pedi.12176
86. Tsalikian E, Mauras N, Beck RW, Tamborlane WV, Janz KF, Chase HP, et al. Impact of exercise on overnight glycemic control in children with type 1 diabetes mellitus. J Pediatr. (2005) 147:528–34. doi: 10.1016/j.jpeds.2005.04.065
87. Taplin CE, Cobry E, Messer L, McFann K, Chase HP, Fiallo-Scharer R. Preventing post-exercise nocturnal hypoglycemia in children with type 1 diabetes. J Pediatr. (2010) 157:784–8.e1. doi: 10.1016/j.jpeds.2010.06.004
88. Sandoval DA, Guy DL, Richardson MA, Ertl AC, Davis SN. Effects of low and moderate antecedent exercise on counterregulatory responses to subsequent hypoglycemia in type 1 diabetes. Diabetes. (2004) 53:1798–806. doi: 10.2337/diabetes.53.7.1798
89. Dagogo-Jack SE, Craft S, Cryer PE. Hypoglycemia-associated autonomic failure in insulin-dependent diabetes mellitus. Recent antecedent hypoglycemia reduces autonomic responses to, symptoms of, and defense against subsequent hypoglycemia. J Clin Invest. (1993) 91:819–28. doi: 10.1172/JCI116302
90. Landt KW, Campaigne BN, James FW, Sperling MA. Effects of exercise training on insulin sensitivity in adolescents with type I diabetes. Diabetes Care. (1985) 8:461–5. doi: 10.2337/diacare.8.5.461
Keywords: Type 1 diabetes, exercise, physical activity, hypoglycemia, blood glucose, child, adolescent, consultation
Citation: Chetty T, Shetty V, Fournier PA, Adolfsson P, Jones TW and Davis EA (2019) Exercise Management for Young People With Type 1 Diabetes: A Structured Approach to the Exercise Consultation. Front. Endocrinol. 10:326. doi: 10.3389/fendo.2019.00326
Received: 13 September 2018; Accepted: 07 May 2019;
Published: 14 June 2019.
Edited by:
Jan Polák, Charles University, CzechiaReviewed by:
Hidetaka Hamasaki, Hamasaki Clinic, JapanAngela Lombardi, Albert Einstein College of Medicine, United States
Copyright © 2019 Chetty, Shetty, Fournier, Adolfsson, Jones and Davis. This is an open-access article distributed under the terms of the Creative Commons Attribution License (CC BY). The use, distribution or reproduction in other forums is permitted, provided the original author(s) and the copyright owner(s) are credited and that the original publication in this journal is cited, in accordance with accepted academic practice. No use, distribution or reproduction is permitted which does not comply with these terms.
*Correspondence: Tarini Chetty, Tarinichetty@gmail.com