- 1Laboratory of Animal Reproduction, Graduate School of Bioagricultural Sciences, Nagoya University, Nagoya, Japan
- 2Faculty of Veterinary Medicine, Okayama University of Science, Imabari, Japan
Pubertal onset is thought to be timed by an increase in pulsatile gonadotropin-releasing hormone (GnRH)/gonadotropin secretion in mammals. The underlying mechanism of pubertal onset in mammals is still an open question. Evidence accumulated in the last 15 years suggests that kisspeptin/neurokinin B/dynorphin A (KNDy) neurons in the hypothalamic arcuate nucleus play a key role in pubertal onset by triggering pulsatile GnRH/gonadotropin secretin in mammals. Specifically, KNDy neurons are now considered a part of GnRH pulse generator, in which neurokinin B facilitates and dynorphin A inhibits, the synchronized discharge of KNDy neurons in autocrine and/or paracrine manners. Kisspeptin serves as a potent secretagogue of GnRH secretion and thus its release is fundamental to pubertal increase in GnRH/gonadotropin secretion in mammals. Proposed mechanisms inhibiting Kiss1 (kisspeptin gene) expression during childhood to juvenile varies from species to species: we envisage that negative feedback action of estrogen plays a key role in the inhibition of Kiss1 expression in KNDy neurons in rodents and sheep, whereas estrogen-independent inhibition of kisspeptin secretion by γ-amino butyric acid or neuropeptide Y are suggested to be responsible for the pre-pubertal suppression of GnRH/gonadotropin secretion in primates. Taken together, the timing of pubertal onset is postulated to be controlled by upstream regulators for kisspeptin biosynthesis and secretion in mammals.
Introduction
The reproductive system is governed by the hypothalamo-pituitary-gonadal axis and has a unique functional quiescence during childhood in mammals. Pubertal onset is thought to be timed by an increase in gonadotropin-releasing hormone (GnRH)/gonadotropin secretion. The underlying mechanism controlling pubertal onset still remains elusive (1). Evidence accumulated in the last 15 years suggests that a neuropeptide kisspeptin plays a key role in pubertal onset via triggering GnRH/gonadotropin secretion in mammals. This article provides a brief historical background including the discoveries of gonadotropins, GnRH, and kisspeptin, and our current understanding of how the brain controls pubertal onset in mammals.
GnRH/Gonadotropin Secretion Triggers Pubertal Onset in Mammals
The concept that factor(s) synthesized and secreted by the pituitary gland controls pubertal onset in mammals dates back to the late 1920s, when physiologists examined effects of hypophysectomy or extract of the pituitary gland on gonadal activities and found the factor(s) that induced pubertal onset in immature rodents (2). First, they believed that the pituitary gland synthesizes and secretes a single gonadotropic factor. Later, increasing evidence suggested the existence of two gonadotropic factors in the pituitary gland and two gonadotropins, follicle-stimulating hormone (FSH) and luteinizing hormone (LH), were successfully purified from the pituitary gland in the early 1930s (3). In the late 1940s, Harris predicted that the secretion of pituitary gonadotropins would be controlled by factor(s) secreted from the hypothalamic neurons through the pituitary portal circulation (4). This prediction promoted studies on the discovery of such hypothalamic factor(s) in several mammals. Consequently, the mammalian form of GnRH, a decapeptide, which stimulates both FSH and LH secretion from the pituitary gland, was isolated and sequenced by two independent laboratories led by Schally and Guillemin in the early 1970s (5, 6).
Establishment of the radioimmunoassay by Yalow and Berson (7) in 1950s has facilitated studies on GnRH/gonadotropin secretion. By using frequent blood collection and this radioimmunoassay, Knobil et al. clearly demonstrated that two modes of gonadotropin secretion in female rhesus monkeys, as a model of humans (8–10): one is tonic gonadotropin secretion that controls follicular development and steroidogenesis in the ovary and the other is a large secretion of LH, namely a LH surge, that controls ovulation and corpus luteum formation. As for tonic secretion, Knobil et al. successfully showed the pulsatile nature of LH secretion (9). They assumed that the pulsatile nature of tonic gonadotropin secretion is most likely caused by pulsatile GnRH stimulation to the pituitary gland and clearly demonstrated the physiological significance of pulsatile GnRH stimulation on the pituitary gland to maintain circulating gonadotropin levels (11). Importantly, continuous GnRH stimulation to the pituitary gland suppressed gonadotropin secretion (11). These findings clearly indicate that the pulsatile nature of GnRH secretion is required to maintain the normal responsiveness of the pituitary gland to GnRH. The Knobil laboratory also demonstrated that this pulsatile GnRH stimulation successfully triggered pubertal onset in immature female monkeys (12). This finding suggests that an initiation of pulsatile GnRH secretion is the first step in pubertal onset in mammals. Accumulating evidence suggests that pubertal onset is triggered by an increase in pulsatile secretion of GnRH/gonadotropins in several mammalian species (13–16).
Pulsatile GnRH secretion in the pituitary portal circulation was first described in sheep in 1982 by Clarke and Cummins (17) with a pituitary portal cannulation, and then examined with the same skillful technique in more detail in 1992 by Moenter et al. (18). These studies clearly demonstrated that each GnRH pulse detected in the pituitary portal circulation corresponds to each LH pulse detected in the peripheral circulation. Pulsatile GnRH secretion is not easily detectable in the peripheral circulation due to a combination of the short half-life of GnRH and the dilution in the relatively large volume of peripheral circulation (18). Therefore, plasma LH profiles showing pulsatile LH secretion have been used as good surrogate for pulsatile GnRH secretion into the pituitary portal circulation in mammals.
Kisspeptin Controls GnRH Pulse Generation and Pubertal Onset
Pulsatile GnRH secretion from the nerve terminals of GnRH neurons at the median eminence into the pituitary portal circulation has been assumed to be driven by a so-called “GnRH pulse generator” (19, 20). The Knobil laboratory first established a method for the detection of GnRH pulse generator activity via an electrophysiological approach in rhesus monkeys (21): They clearly demonstrated that rhythmic increases in the multiple unit activity (MUA), correspond to LH pulses detected in the peripheral circulation, was successfully monitored from the recording electrodes implanted in the mediobasal hypothalamus. This electrophysiological approach was subsequently adapted to rats and goats (22–24) and showed that rhythmic increases in the MUA recorded in the mediobasal hypothalamus, or more specifically in the arcuate nucleus (ARC), also correspond to LH pulses in such species.
Increasing evidence suggests that hypothalamic kisspeptin [first named as metastin (25)] neurons play a key role in controlling pubertal onset via stimulation of GnRH/gonadotropin secretion in mammals. A novel peptide kisspeptin was discovered from the human placenta as an endogenous ligand of GPR54, an orphan G-protein coupled receptor, in 2001 (25, 26). Subsequently, clinical studies revealed that inactivating mutations of the GPR54 gene caused the impairment of pubertal maturation and reproductive functions with hyposecretion of gonadotropins, i.e., hypogonadotropic hypogonadism, in humans (27, 28). These findings suggest that kisspeptin serves as a gatekeeper of pubertal onset in the hypothalamo-pituitary cascade regulating reproductive system in humans. Indeed, kisspeptin profoundly stimulated gonadotropin secretion in several mammals including humans (29–35) and the stimulatory effect of kisspeptin on gonadotropin secretion was blocked by GnRH antagonists in rodent models (29–31). These studies clearly indicate a physiological role of kisspeptin as a potent secretagogue of GnRH in the hypothalamus. In 2012, the phenotypes of patients carrying GPR54 mutations, i.e., hypogonadotropic hypogonadism and lack of puberty, was recapitulated in patients carrying inactivating mutations of the KISS1 gene (coding kisspeptin) (36). Further, several rodent models lacking functional kisspeptin or its receptor by gene targeting, namely Kiss1 or Gpr54 knockout mice, showed pubertal failure and infertility (28, 34, 37–39). In addition, Kiss1 knockout rats never showed puberty and demonstrated a lack of both pulse and surge modes of gonadotropin secretion (40). These findings strongly suggest that kisspeptin-GPR54 signaling plays a key role in the mechanism controlling GnRH/gonadotropin secretion and pubertal onset in mammals. Indeed, Keen et al. (41) demonstrated that a pubertal increase in pulsatile kisspeptin secretion along with pulsatile GnRH secretion at the median eminence in rhesus monkeys. Kisspeptin administration successfully stimulated GnRH/LH secretion in pre-pubertal and pubertal mammals including rodents (42–44) and primates (45–47).
It is well-accepted that kisspeptin directly stimulates GnRH secretion via GPR54 expressed in GnRH neurons in rodents (30, 34). Indeed, the targeted deletion of Gpr54 in GnRH neurons of mice resulted in infertility, whereas the reintroduction of Gpr54 to GnRH neurons in Gpr54-null mice resulted in fertility (48), suggesting that GPR54 expression solely in GnRH neurons is sufficient for fertility. A previous study showed stable expression of GnRH mRNA in the hypothalamus during the pubertal transition in rats (49). Thus, kisspeptin has a role to trigger GnRH secretion rather than GnRH synthesis at the onset of puberty in mammals.
Two Populations of Kisspeptin Neurons Control Pulse and Surge-Mode of GnRH Secretion
There are two populations of hypothalamic kisspeptin neurons: one is localized in the ARC and the other population is localized in the anteroventral periventricular nucleus and periventricular nucleus continuum (AVPV-PeN) in rodents and the pre-optic area (POA) in other mammals including ruminants, primates, pigs, and musk shrews (50–60). Kisspeptin neurons localized in the AVPV-PeN of rodents are possibly equivalent to those in the POA of the other animals. Accumulating evidence suggests that the neurons in the ARC have a role in GnRH pulse generation described above, whereas the neurons in the AVPV-PeN have been recognized to play a pivotal role in mediating positive feedback action of estrogen to induce GnRH/LH surge in rodents (61–64).
The most plausible interpretation is that ARC kisspeptin neurons serve as a part of the GnRH pulse generator in mammals, because rhythmic increases in the MUA are successfully detected from recording electrodes that are placed in close proximately to ARC kisspeptin neurons in goats (53) but not in the lateral median eminence where GnRH nerve terminals are abundantly located. Further, a study with a GCaMP6 fiber photometry technique demonstrated in mice that ARC kisspeptin neurons exhibited rhythmic increases in intra-cellular Ca2+ corresponding to LH pulses and that optogenetic stimulation or inhibition of ARC kisspeptin neurons induced or suppressed pulsatile LH secretion, respectively (65). GnRH neuronal terminals in the median eminence seem to be an action site of kisspeptin for the generation of GnRH pulses (Figure 1), because immunoelectron microscopy revealed a close association of kisspeptin and GnRH fibers in the median eminence in rats and goats (66, 67). Further, few typical synaptic structures between kisspeptin and GnRH fibers were found in the median eminence, suggesting that kisspeptin acts on GnRH fibers in a non-synaptic manner, such as “volume transmission” (66, 67). GnRH cell bodies also seem to be an action site of ARC kisspeptin neurons, because a tract-tracing study revealed the projection of ARC kisspeptin neuron to the POA in mice (68) and confocal microscopy revealed contacts of kisspeptin fibers from the ARC population to GnRH neurons in ewes (69).
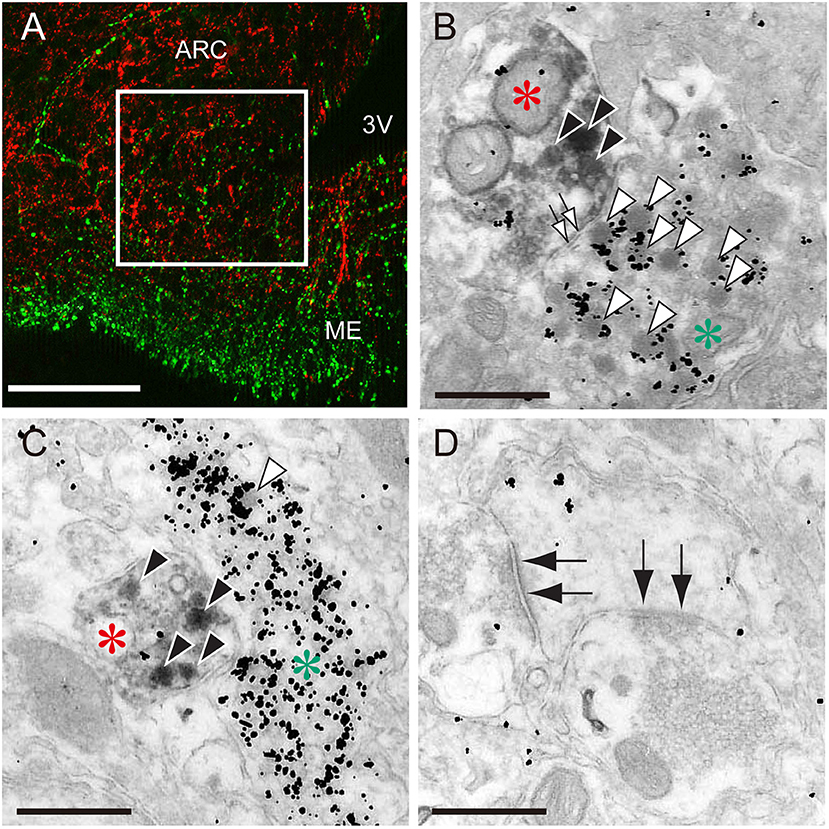
Figure 1. The close association of kisspeptin and gonadotropin-releasing hormone (GnRH) fibers in the median eminence of female rats. (A) An immunofluorescence photomicrograph showing kisspeptin (red)- and GnRH (green)-immunoreactivities in the arcuate nucleus (ARC)-median eminence (ME) region. Note that the square in the internal zone of the median eminence shows the area for electron microscopy. Scale bar, 50 μm. 3V, third ventricle. (B,C) Immunoelectron micrographs showing kisspeptin- (red asterisks) and GnRH-immunoreactive fibers (green asterisks) visualized by diaminobenzidine and silver enhancement of colloidal gold, respectively. Solid and open arrowheads show kisspeptin- and GnRH-immunoreactive secretory granules, respectively. Open arrows show non-synaptic contact between kisspeptin- and GnRH-neuronal elements. Scale bar, 500 nm. (D) Electron micrograph showing synaptic contacts (solid allows) in other neurons. Scale bar, 500 nm. Originally published in Uenoyama et al. (66) (permission was obtained from John Wiley and Sons).
Increasing evidence suggests that the anterior population of hypothalamic kisspeptin neurons serves as GnRH surge generator that evokes the GnRH/LH surge in response to the positive feedback action of estrogen derived from the pre-ovulatory follicles in mammals, because estrogen up-regulates Kiss1 expression and/or neuronal activation in this population (52, 54–57, 70–72). We recently reviewed the sexual dimorphism and role of AVPV-PeN kisspeptin neurons elsewhere (73).
In contrast to AVPV Kiss1 expression, ARC Kiss1 expression is down-regulated by estrogen. This suggests that ARC kisspeptin neurons mediate the negative feedback action of estrogen on pulsatile gonadotropin secretion in mammals (33, 50, 52). Recently, Treen et al. (74) have generated immortalized Kiss1-expressing cells from the murine hypothalamus and successfully replicated estrogenic regulation of Kiss1 expression in both AVPV- and ARC-derived immortalized Kiss1-expressing cells. Such immortalized AVPV and ARC Kiss1-expressing cells would serve as a promising platform to explore the molecular mechanism mediating estrogenic controls of Kiss1 expression in the two hypothalamic kisspeptin neurons.
Interestingly, the interaction between ARC and AVPV kisspeptin neurons was recently suggested through a tract-tracing study showed that ARC kisspeptin neurons project to the AVPV kisspeptin neurons in mice (75). Further, an optogenetic study indicated that the channelrhodopsin-2-mediated light stimulation of ARC kisspeptin neurons activated GnRH neurons via AVPV kisspeptin neurons and suggested that this pathway is likely involved in the pre-ovulatory GnRH/LH surge (76). This finding is consistent with our previous finding that ARC kisspeptin neurons are activated at the proestrous stage of estrus cycle in female rats (33). Interestingly, Kiss1 expression in both POA and caudal ARC increased just before GnRH/LH surges in ewes and monkeys (59, 60, 77). These findings are also consistent with previous findings that the mediobasal hypothalamus including the ARC is likely involved in GnRH/LH surge generation in those species (78–81).
Ours and other previous studies showed a pubertal increase in both ARC and AVPV Kiss1 expression in female rodents (42, 51, 82). As discussed below, we envision that the regulation of ARC Kiss1 expression is fundamental to control pubertal onset of tonic gonadotropin secretion, whereas AVPV Kiss1 expression is secondarily controlled by estrogen secreted from the ovary under the tonic stimulation of gonadotropin (82).
Cellular and Molecular Mechanism of GnRH Pulse Generation and Pubertal Onset
Clinical studies also demonstrated the involvement of neurokinin B, a member of tachykinin family (83), in regulation of GnRH/gonadotropin secretion and pubertal onset in humans: loss-of-function mutations of TAC3 gene (coding neurokinin B) or TACR3 gene (coding tachykinin NK3 receptor (NK3R), which preferably bind to neurokinin B) also caused hypogonadotropic hypogonadism and pubertal failure in humans (84–86). It is noteworthy that both neurokinin B and NK3R are co-localized in most of ARC kisspeptin neurons of several mammalian species (87–89). Further, dynorphin A, an endogenous opioid peptide, is also co-localized in ARC kisspeptin neurons (87–89). Thus, the kisspeptin/neurokinin B/dynorphin A (KNDy) co-expressing neurons in the ARC are referred to as KNDy neurons (90). Wakabayashi et al. (89) clearly demonstrated that a central administration of neurokinin B facilitated the frequency of the rhythmic increases in the MUA in goats. They also demonstrated that the frequency of the rhythmic increases in the MUA was suppressed by a central administration of dynorphin A and facilitated by antagonism of the kappa-opioid receptor (KOR, which preferably binds to dynorphin A) signaling in goats (89). Similarly, a central administration of neurokinin B or KOR antagonist facilitated pulsatile LH secretion in sheep (91, 92). Recently, Weems et al. demonstrated that KOR is expressed in both KNDy and GnRH neurons and proposed a current working model that dynorphin A acts on both KNDy and GnRH neurons to terminate each GnRH pulse in ewes (93–95). This is in agreement with our previous study that showed that chronic peripheral administration of NK3R agonist or KOR antagonist prior to puberty advanced pubertal onset in female rats via an increase in tonic gonadotropin secretion (96). Taken together, it is tempting to speculate that neurokinin B-NK3R and/or dynorphin A-KOR signaling may serve as a driving force for GnRH pulse generation to trigger the pubertal onset in an autocrine and/or paracrine manner in mammals. Previous studies have shown pubertal increase in ARC Tac3 as well as Kiss1 expression in mRNA or protein levels in rodents (82, 97, 98) and sheep (99).
Our recent in vitro study using a primary culture of green fluorescent protein (GFP)-tagged kisspeptin neurons obtained from transgenic Kiss1-GFP mice, proposed possible involvement of gap junctions between KNDy neurons as well as between KNDy neurons and glial cells in the generation of rhythmic activity of kisspeptin neurons under the neurokinin B-NK3R signaling (100). Briefly, neurokinin B secreted from KNDy neurons binds NK3R to open Ca2+ channels in an autocrine/paracrine manner (#1 in Figure 2), triggering Ca2+ influx into the NK3R-expressing KNDy neurons (#2 in Figure 2). The increased intracellular Ca2+ may propagate to neighboring KNDy neurons and glial cells via gap junctions, even if those neurons do not express NK3R (#3 in Figure 2). The gap junctions between KNDy neurons or between KNDy neurons and glial cells might be formed, at least in part, by connexins 26 or 37, because quantitative RT-PCR analysis showed Gjb2 (connexin 26 gene) and Gja4 (connexin 37 gene) expression in kisspeptin neurons of the Kiss1-GFP mice (100). The propagation of intracellular Ca2+ increase would result in synchronized discharge of ARC KNDy neurons and then causes pulsatile kisspeptin secretion at the median eminence (#4 in Figure 2). Finally, kisspeptin in turn induces pulsatile GnRH secretion into the pituitary portal circulation (#5 in Figure 2). Neurokinin B-NK3R signaling is supposed to be a major mechanism underlying the synchronized discharge of KNDy neurons, because ~80% KNDy neurons express NK3R (100, 101). The gap junctional communication would contribute to ensure synchronized discharge of KNDy neurons regardless of NK3R expression. Indeed, a previous study reported that patients with mutations of connexin-26 showed hypogonadotropic hypogonadism and a lack of pubertal onset (102).
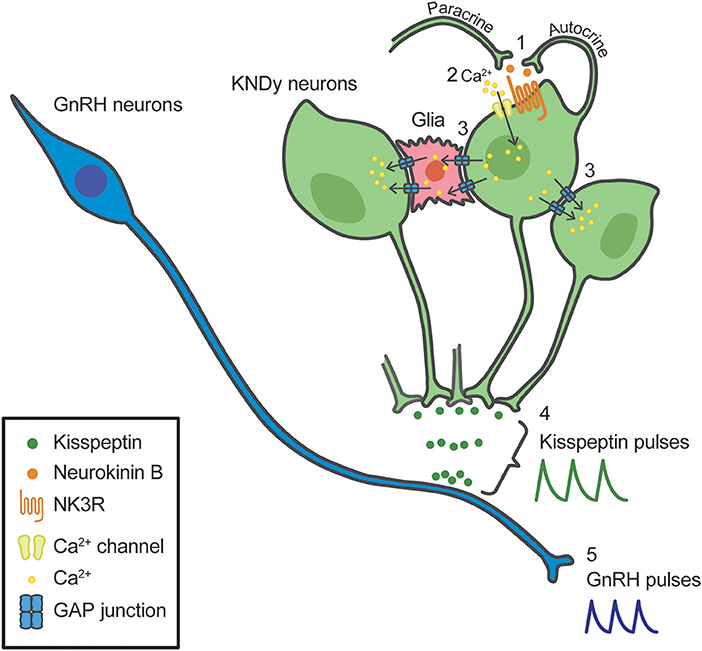
Figure 2. Schematic illustration of a model for the synchronized discharge of arcuate kisspeptin/neurokinin B/dynorphin A (KNDy) neurons and subsequent GnRH secretion. Neurokinin B secreted from KNDy neurons (green) binds tachykinin NK3 receptor (NK3R) in an autocrine/paracrine manner (1), triggering Ca2+ influx into the NK3R-expressing KNDy neurons (2). The increased intracellular Ca2+ may propagate to neighboring KNDy neurons and glial cells (red) via gap junctions, even if those neurons do not express NK3R (3). The propagation of intracellular Ca2+ increase would result in synchronized discharge of KNDy neurons and then causes pulsatile kisspeptin secretion (4). Resultant pulsatile kisspeptin secretion controls pulsatile GnRH secretion at the median eminence (5).
Species Difference in the Pre-Pubertal Restraint of GnRH/Gonadotropin Secretion
A current interpretation for brain mechanism controlling pubertal onset in mammals is presented schematically in Figures 3, 4. Several lines of evidence suggest that the key players in this mechanism are ARC KNDy neurons, which serve as the GnRH pulse generator, regulate pulsatile GnRH/gonadotropin secretion and hence pubertal onset in mammals including rodents, ruminants, and primates. GnRH pulse generation is postulated to be suspended by a lack of kisspeptin secretion in mammals during the pre-pubertal period. Indeed, previous studies demonstrated a pubertal increase in Kiss1 expression in rodents (42, 82) and a pubertal increase in kisspeptin secretion at the median eminence in primates (41).
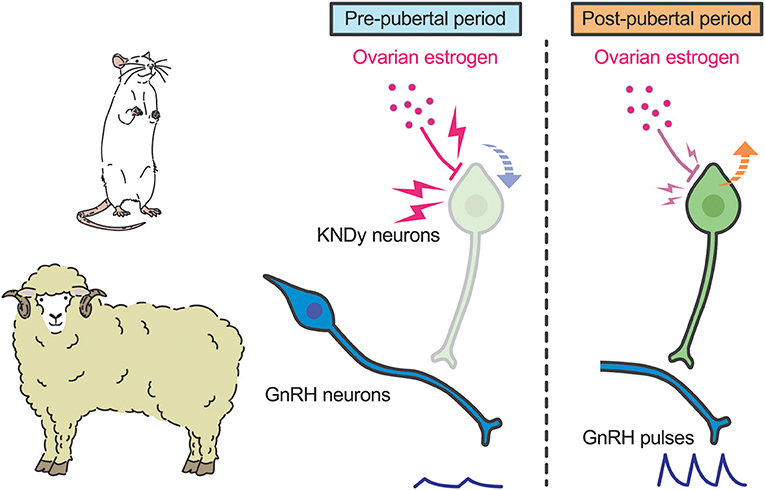
Figure 3. Schematic illustration showing a current interpretation for the brain mechanism controlling pubertal onset in rats and sheep. We envision that ovarian estrogen strongly suppresses ARC Kiss1 expression (down arrow). The inhibitory action of estrogen on ARC Kiss1 expression somehow decreases during the pubertal transition, resulting in an increase in Kiss1 expression (up arrow). Resultant pulsatile kisspeptin secretion triggers pubertal increase in pulsatile GnRH secretion.
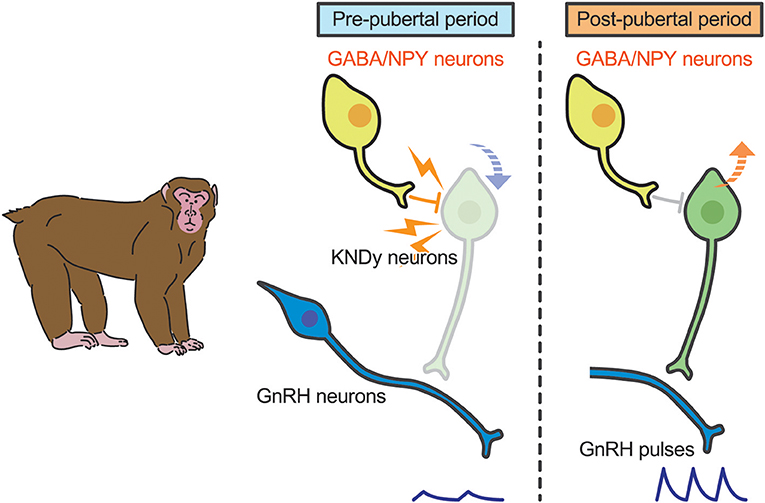
Figure 4. Schematic illustration showing a current interpretation for brain mechanism controlling pubertal onset in primates. We envision that γ-amino butyric acid (GABA) or neuropeptide Y (NPY) suppresses kisspeptin secretion (down arrow) in primates. Those inhibitory actions somehow disappear during the pubertal transition, resulting in an increase in Kiss1 expression (up arrow). Resultant kisspeptin secretion triggers pubertal increase in GnRH secretion.
Interestingly, the underlying mechanism of suppression of kisspeptin biosynthesis and secretion during pre-pubertal period differs from species to species. In rodents and sheep, ovariectomy acutely induced gonadotropin secretion even in pre-pubertal period, whereas estrogen replacement inhibited gonadotropin secretion until a normal pre-pubertal period expired (43, 82, 103). These findings suggest that estrogen plays a key role in the pre-pubertal restraint of GnRH/gonadotropin secretion and that the inhibitory action of estrogen seems to decrease during pubertal transition in those species (Figure 3). Indeed, estrogen strongly suppresses ARC Kiss1 expression in female rats during the pre-pubertal period, but estrogen moderately suppresses ARC Kiss1 expression in female rats during the post-pubertal period (82). Further, ovariectomy increased the number of ARC kisspeptin-immunoreactive cells in sheep in the pre-pubertal period, but not in the post-pubertal period (99). The regulation of ARC Kiss1 expression by estrogen during pubertal transition is consistent with the classical “gonadostat hypothesis” (104, 105) which states that a decrease in the sensitivity to the negative feedback action of estrogen would be associated with the pubertal increase in GnRH/gonadotropin secretion in rodents. We envisage that a small amount of estrogen derived from the immature ovary directly inhibits Kiss1 expression via estrogen receptor α (ERα) in kisspeptin neurons, because kisspeptin neuron-specific ERα knockout mice showed premature increases in ARC Kiss1 expression and gonadotropin secretion, which resulted in the precocious pubertal onset (106, 107). Indeed, estrogen implantation into the ARC suppressed LH pulses in ovariectomized rats at the pre-pubertal period (43). Interestingly, estrogen implantation into the POA also suppressed LH pulses in ovariectomized rats at the pre-pubertal period, suggesting that ARC kisspeptin neurons are not a solitary inhibitory action site of estrogen to suppress Kiss1 expression and hence GnRH/gonadotropin secretion in rats (43). Further studies are needed to clarify how sensitivity to estrogen decreases during pubertal transition in rats and sheep.
In primates, plasma gonadotropin profiles in gonadectomized animals were comparable to gonad-intact controls during juvenile and childhood: gonadotropin secretion was suppressed until just before puberty in both gonad-intact and gonadectomized animals (108, 109). Interestingly, estrogen-dependent suppression of gonadotropin secretion is manifested just before puberty onset in monkeys (110, 111). Thus, the central mechanism inhibiting GnRH/gonadotropin secretion in primates appears to differ from that in rodents and sheep (109, 112). A current interpretation for the brain mechanism controlling pubertal onset in primates was reported elsewhere (113). Briefly, two hypotheses have been suggested for the central suppression of GnRH/gonadotropin secretion during childhood to juvenile in primates (Figure 4). One is that tonic inhibition by γ-aminobutyric acid (GABA) neurotransmission would be responsible for the central suppression of GnRH/gonadotropin secretion in pre-pubertal monkeys (112); the other is that neuropeptide Y neurons could be responsible for the pre-pubertal suppression of GnRH/gonadotropin secretion (114). KNDy neurons may mediate the inhibitory role of GABA and/or NPY neurons in pre-pubertal restraint of GnRH neurons in primates. Further studies are needed to clarify how these inhibitory actions disappear at the pubertal onset in primates.
Metabolic Control of Pubertal Onset
It has been well-accepted that nutritional or metabolic cues are important determinants of the initiation of tonic GnRH/gonadotropin secretion during pubertal transition, as evidenced by suppression of gonadotropin secretion when growth is retarded by a food restriction in several mammalian species including sheep (115) and rats (116, 117). Interestingly, such animals exhibited pubertal onset when they reached “critical” body weights, at which normally grown animals with ad libitum feeding exhibited pubertal onset (115, 116). The “critical” body weight hypothesis for determining pubertal onset was initially proposed by Frisch and Revelle (118) as an explanation of findings that girls showed puberty onset when they reached a body weight of around 47 kg at 17 years old in 1840s, and at 13 years old in 1960s along with nutritional improvements (119, 120).
Nutritional or metabolic cues reportedly control hypothalamic Kiss1 expression in rodents. Castellano et al. (121) demonstrated that short-time fasting inhibited hypothalamic Kiss1 expression along with the suppression of LH secretion in peripubertal rats. Inhibition of Kiss1 expression and LH release by fasting has also been reported in adult rats and mice (122–126). Our recent tract-tracing study showed that hypothalamic kisspeptin neurons receive neuronal inputs from the hindbrain ependymocytes (127), which are suggested as a central energy sensor, in rats (128). The hindbrain ependymocytes, which express pancreatic glucokinase—a rate-limiting enzyme for glucose metabolism—and AMP-activated protein kinase, have been proposed to be able to sense, in particular, lowered glucose availability for controlling GnRH/LH secretion in rats (129–131). Thus, it is speculated that pre-pubertal suppression of hypothalamic Kiss1/kisspeptin expression might be, at least partly, mediated by this neuronal network.
Energy storage in the adipose tissue has been also considered to be involved in pubertal onset for a long time (132, 133). Leptin, the first hormone discovered to be secreted from the adipocytes, was then considered as a signal that relays the attainment of energy storage from the adipose tissue to the brain. Indeed, the leptin receptor is expressed in several hypothalamic and extra-hypothalamic nuclei including the ARC (134): KNDy neurons in the ARC express leptin receptors in mice (135). Importantly, leptin-null mice showed decreased ARC Kiss1 expression (135) and pubertal failure (136). Moreover, leptin administration restored ARC Kiss1 expression (135) and fertility in the leptin-null mice (136). Further, leptin administration induced pubertal onset in normal and growth-retarded rodents (137–140). These studies suggest that leptin is a prerequisite of normal pubertal onset. Interestingly, an increase in leptin secretion is not necessarily preceded with the pubertal onset in several mammals including primates and rodents (141–145). In humans, serum leptin concentration increased during pubertal development in girls, but remained constant in boys (141, 143). Circulating leptin remained constant during puberty in male monkeys (142, 144) and female rats (145). Donato et al. (146) showed that genetic deletion of the leptin receptor selectively from hypothalamic kisspeptin neurons had no critical effect on puberty and subsequent reproductive performance in mice, suggesting that leptin indirectly acts on kisspeptin neurons to control Kiss1 expression. Further, recent studies suggested two separate leptin signaling pathways from ARC proopiomelanocortin (POMC) neurons and pituitary adenylate cyclase activating polypeptide (PACAP) neurons to ARC kisspeptin neurons in mice (147, 148). Overall, leptin is now considered as one of the permissive metabolic factors that allow pubertal development to proceed (149).
Ghrelin, a hormone mainly secreted from the stomach (150, 151), has been suggested to cooperate with leptin for the metabolic control of puberty onset in mammals (152–154): ghrelin has an inhibitory effect, while leptin has a stimulatory effect on pubertal onset. Indeed, repeated administration of ghrelin during pubertal transition partly delayed pubertal onset along with a decrease in serum LH and testosterone levels in male rats (155, 156). This notion is consistent with previous studies suggesting that ghrelin has an orexigenic effect as a peripheral signal of energy insufficiency, because ghrelin was shown to increase food intake in humans and rodents and plasma ghrelin levels increased by fasting and decreased by food intake in humans (157, 158). Further studies are needed to clarify the mechanisms underlying metabolic control of pubertal onset in mammals.
Conclusions and Perspective
Studies during the twentieth century have increased our understanding of the mechanism controlling pubertal onset in mammals along with the discoveries of gonadotropins from the pituitary gland and GnRH from the hypothalamus. Most significantly, a breakthrough came in twenty-first century when kisspeptin and its receptor emerged. We now envisage that ARC kisspeptin neurons (also known as KNDy neurons), as a part of the GnRH pulse generator, are instrumental for pubertal onset in mammals via triggering GnRH/gonadotropin secretion. The timing of pubertal onset would be controlled by upstream regulators for kisspeptin biosynthesis and secretion. These are tightly controlled by steroid-dependent (rodents and sheep) or steroid-independent (primates) mechanisms as mentioned above. In addition, pubertal changes in kisspeptin biosynthesis and secretion may also be controlled by nutritional or metabolic cues. Further studies are needed to fully elucidate underlying mechanism of pubertal onset in mammals.
Author Contributions
YU collected the information and wrote the manuscript. SN collected the information and designed the pictures. NI and HT collected the information and critically revised the manuscript.
Funding
The present study was supported in part by JSPS KAKENHI Grant Numbers 16K07987 (to NI) and 18H03973 (to HT).
Conflict of Interest Statement
The authors declare that the research was conducted in the absence of any commercial or financial relationships that could be construed as a potential conflict of interest.
Acknowledgments
We respectfully acknowledge the contributions of the late Prof. Kei-ichro Maeda, University of Tokyo, who provided invaluable insights, support, and guidance for our original studies reviewed in this article. We would like to thank Dr. Nicola Skoulding for the editorial assistance.
References
2. Hamburger C. Historical introduction. In: Wolstenholme GEW, Knight J, editors. Gonadotropins: Physiochemical and Immunological Properties. London, WI: J. & A. Churchill Ltd. (1966). p. 2–10.
3. Fevold H, Hisaw F, Leonard S. The gonad stimulating and the luteinizing hormones of the anterior lobe of the hypophesis. Am J Physiol. (1931) 97:291–301. doi: 10.1152/ajplegacy.1931.97.2.291
4. Harris GW. Neural control of the pituitary gland. Physiol Rev. (1948) 28:139–79. doi: 10.1152/physrev.1948.28.2.139
5. Matsuo H, Baba Y, Nair RM, Arimura A, Schally AV. Structure of the porcine LH- and FSH-releasing hormone. I. The proposed amino acid sequence. Biochem Biophys Res Commun. (1971) 43:1334–9. doi: 10.1016/S0006-291X(71)80019-0
6. Amoss M, Burgus R, Blackwell R, Vale W, Fellows R, Guillemin R. Purification, amino acid composition and N-terminus of the hypothalamic luteinizing hormone releasing factor (LRF) of ovine origin. Biochem Biophys Res Commun. (1971) 44:205–10. doi: 10.1016/S0006-291X(71)80179-1
7. Yalow RS. Radioimmunoassay: a probe for the fine structure of biologic systems. Science. (1978) 200:1236–45. doi: 10.1126/science.208142
8. Neill JD, Johansson ED, Datta JK, Knobil E. Relationship between the plasma levels of luteinizing hormone and progesterone during the normal menstrual cycle. J Clin Endocrinol Metab. (1967) 27:1167–73. doi: 10.1210/jcem-27-8-1167
9. Dierschke DJ, Bhattacharya AN, Atkinson LE, Knobil E. Circhoral oscillations of plasma LH levels in the ovariectomized rhesus monkey. Endocrinology. (1970) 87:850–3. doi: 10.1210/endo-87-5-850
10. Monroe SE, Atkinson LE, Knobil E. Patterns of circulating luteinizing hormone and their relation to plasma progesterone levels during the menstrual cycle of the Rhesus monkey. Endocrinology. (1970) 87:453–5. doi: 10.1210/endo-87-3-453
11. Belchetz PE, Plant TM, Nakai Y, Keogh EJ, Knobil E. Hypophysial responses to continuous and intermittent delivery of hypopthalamic gonadotropin-releasing hormone. Science. (1978) 202:631–3. doi: 10.1126/science.100883
12. Wildt L, Marshall G, Knobil E. Experimental induction of puberty in the infantile female rhesus monkey. Science. (1980) 207:1373–5. doi: 10.1126/science.6986658
13. Andrews WW, Ojeda SR. A detailed analysis of the serum luteinizing hormone secretory profile in conscious, free-moving female rats during the time of puberty. Endocrinology. (1981) 109:2032–9. doi: 10.1210/endo-109-6-2032
14. Foster DL, Ebling FJP, Vannerson LA, Wood RI, Fenner DE. Regulation of puberty in the lamb: internal and external cues. in: Imura H, Shizume K, and Yoshida S, editors. Progress in Endocrinology. Amsterdam: Elsevier (1988). p. 861–6.
15. Watanabe G, Terasawa E. In vivo release of luteinizing hormone releasing hormone increases with puberty in the female rhesus monkey. Endocrinology. (1989) 125:92–9. doi: 10.1210/endo-125-1-92
16. Sisk CL, Richardson HN, Chappell PE, Levine JE. In vivo gonadotropin-releasing hormone secretion in female rats during peripubertal development and on proestrus. Endocrinology. (2001) 142:2929–36. doi: 10.1210/endo.142.7.8239
17. Clarke IJ, Cummins JT. The temporal relationship between gonadotropin releasing hormone (GnRH) and luteinizing hormone (LH) secretion in ovariectomized ewes. Endocrinology. (1982) 111:1737–9. doi: 10.1210/endo-111-5-1737
18. Moenter SM, Brand RM, Midgley AR, Karsch FJ. Dynamics of gonadotropin-releasing hormone release during a pulse. Endocrinology. (1992) 130:503–10. doi: 10.1210/endo.130.1.1727719
19. Lincoln DW, Fraser HM, Lincoln GA, Martin GB, McNeilly AS. Hypothalamic pulse generators. Recent Prog Horm Res. (1985) 41:369–419. doi: 10.1016/B978-0-12-571141-8.50013-5
20. Maeda K, Tsukamura H, Ohkura S, Kawakami S, Nagabukuro H, Yokoyama A. The LHRH pulse generator: a mediobasal hypothalamic location. Neurosci Biobehav Rev. (1995) 19:427–37. doi: 10.1016/0149-7634(94)00069-D
21. Wilson RC, Kesner JS, Kaufman JM, Uemura T, Akema T, Knobil E. Central electrophysiologic correlates of pulsatile luteinizing hormone secretion in the rhesus monkey. Neuroendocrinology. (1984) 39:256–60. doi: 10.1159/000123988
22. Kawakami M, Uemura T, Hayashi R. Electrophysiological correlates of pulsatile gonadotropin release in rats. Neuroendocrinology. (1982) 35:63–7. doi: 10.1159/000123356
23. Mori Y, Nishihara M, Tanaka T, Shimizu T, Yamaguchi M, Takeuchi Y, et al. Chronic recording of electrophysiological manifestation of the hypothalamic gonadotropin-releasing hormone pulse generator activity in the goat. Neuroendocrinology. (1991) 53:392–5. doi: 10.1159/000125746
24. Nishihara M, Takeuchi Y, Tanaka T, Mori Y. Electrophysiological correlates of pulsatile and surge gonadotrophin secretion. Rev Reprod. (1999) 4:110–6. doi: 10.1530/ror.0.0040110
25. Ohtaki T, Shintani Y, Honda S, Matsumoto H, Hori A, Kanehashi K, et al. Metastasis suppressor gene KiSS-1 encodes peptide ligand of a G-protein-coupled receptor. Nature. (2001) 411:613–7. doi: 10.1038/35079135
26. Kotani M, Detheux M, Vandenbogaerde A, Communi D, Vanderwinden JM, Le Poul E, et al. The metastasis suppressor gene KiSS-1 encodes kisspeptins, the natural ligands of the orphan G protein-coupled receptor GPR54. J Biol Chem. (2001) 276:34631–6. doi: 10.1074/jbc.M104847200
27. de Roux N, Genin E, Carel JC, Matsuda F, Chaussain JL, Milgrom E. Hypogonadotropic hypogonadism due to loss of function of the KiSS1-derived peptide receptor GPR54. Proc Natl Acad Sci USA. (2003) 100:10972–6. doi: 10.1073/pnas.1834399100
28. Seminara SB, Messager S, Chatzidaki EE, Thresher RR, Acierno JS Jr, Shagoury JK, et al. The GPR54 gene as a regulator of puberty. N Engl J Med. (2003) 349:1614–27. doi: 10.1056/NEJMoa035322
29. Gottsch ML, Cunningham MJ, Smith JT, Popa SM, Acohido BV, Crowley WF, et al. A role for kisspeptins in the regulation of gonadotropin secretion in the mouse. Endocrinology. (2004) 145:4073–7. doi: 10.1210/en.2004-0431
30. Irwig MS, Fraley GS, Smith JT, Acohido BV, Popa SM, Cunningham MJ, et al. Kisspeptin activation of gonadotropin releasing hormone neurons and regulation of KiSS-1 mRNA in the male rat. Neuroendocrinology. (2004) 80:264–72. doi: 10.1159/000083140
31. Matsui H, Takatsu Y, Kumano S, Matsumoto H, Ohtaki T. Peripheral administration of metastin induces marked gonadotropin release and ovulation in the rat. Biochem Biophys Res Commun. (2004) 320:383–8. doi: 10.1016/j.bbrc.2004.05.185
32. Dhillo WS, Chaudhri OB, Patterson M, Thompson EL, Murphy KG, Badman MK, et al. Kisspeptin-54 stimulates the hypothalamic-pituitary gonadal axis in human males. J Clin Endocrinol Metab. (2005) 90:6609–15. doi: 10.1210/jc.2005-1468
33. Kinoshita M, Tsukamura H, Adachi S, Matsui H, Uenoyama Y, Iwata K, et al. Involvement of central metastin in the regulation of preovulatory luteinizing hormone surge and estrous cyclicity in female rats. Endocrinology. (2005) 146:4431–6. doi: 10.1210/en.2005-0195
34. Messager S, Chatzidaki EE, Ma D, Hendrick AG, Zahn D, Dixon J, et al. Kisspeptin directly stimulates gonadotropin-releasing hormone release via G protein-coupled receptor 54. Proc Natl Acad Sci USA. (2005) 102:1761–6. doi: 10.1073/pnas.0409330102
35. Shahab M, Mastronardi C, Seminara SB, Crowley WF, Ojeda SR, Plant TM. Increased hypothalamic GPR54 signaling: a potential mechanism for initiation of puberty in primates. Proc Natl Acad Sci USA. (2005) 102:2129–34. doi: 10.1073/pnas.0409822102
36. Topaloglu AK, Tello JA, Kotan LD, Ozbek MN, Yilmaz MB, Erdogan S, et al. Inactivating KISS1 mutation and hypogonadotropic hypogonadism. N Engl J Med. (2012) 366:629–35. doi: 10.1097/OGX.0b013e31825bc1be
37. d'Anglemont de Tassigny X, Fagg LA, Dixon JP, Day K, Leitch HG, Hendrick AG, et al. Hypogonadotropic hypogonadism in mice lacking a functional Kiss1 gene. Proc Natl Acad Sci USA. (2007) 104:10714–9. doi: 10.1073/pnas.0704114104
38. Lapatto R, Pallais JC, Zhang D, Chan YM, Mahan A, Cerrato F, et al. Kiss1−/− mice exhibit more variable hypogonadism than Gpr54−/− mice. Endocrinology. (2007) 148:4927–36. doi: 10.1210/en.2007-0078
39. Chan YM, Broder-Fingert S, Wong KM, Seminara SB. Kisspeptin/Gpr54-independent gonadotrophin-releasing hormone activity in Kiss1 and Gpr54 mutant mice. J Neuroendocrinol. (2009) 21:1015–23. doi: 10.1111/j.1365-2826.2009.01926.x
40. Uenoyama Y, Nakamura S, Hayakawa Y, Ikegami K, Watanabe Y, Deura C, et al. Lack of pulse and surge modes and glutamatergic stimulation of LH release in Kiss1 knockout rats. J. Neuroendocrinol. (2015) 27:187–197. doi: 10.1111/jne.12257
41. Keen KL, Wegner FH, Bloom SR, Ghatei MA, Terasawa E. An increase in kisspeptin-54 release occurs with the pubertal increase in luteinizing hormone-releasing hormone-1 release in the stalk-median eminence of female rhesus monkeys in vivo. Endocrinology. (2008) 149:4151–7. doi: 10.1210/en.2008-0231
42. Navarro VM, Castellano JM, Fernandez-Fernandez R, Barreiro ML, Roa J, Sanchez-Criado JE, et al. Developmental and hormonally regulated messenger ribonucleic acid expression of KiSS-1 and its putative receptor, GPR54, in rat hypothalamus and potent luteinizing hormone-releasing activity of KiSS-1 peptide. Endocrinology. (2004) 145:4565–74. doi: 10.1210/en.2004-0413
43. Uenoyama Y, Tanaka A, Takase K, Yamada S, Pheng V, Inoue N, et al. Central estrogen action sites involved in prepubertal restraint of pulsatile luteinizing hormone release in female rats. J Reprod Dev. (2015) 61:351–9. doi: 10.1262/jrd.2014-143
44. Han SK, Gottsch ML, Lee KJ, Popa SM, Smith JT, Jakawich SK, et al. Activation of gonadotropin-releasing hormone neurons by kisspeptin as a neuroendocrine switch for the onset of puberty. J Neurosci. (2005) 25:11349–56. doi: 10.1523/JNEUROSCI.3328-05.2005
45. Guerriero KA, Keen KL, Millar RP, Terasawa E. Developmental changes in GnRH release in response to kisspeptin agonist and antagonist in female rhesus monkeys (Macaca mulatta): implication for the mechanism of puberty. Endocrinology. (2012) 153:825–36. doi: 10.1210/en.2011-1565
46. Garcia JP, Keen KL, Kenealy BP, Seminara SB, Terasawa E. Role of kisspeptin and neurokinin B signaling in male rhesus monkey puberty. Endocrinology. (2018) 159:3048–60. doi: 10.1210/en.2018-00443
47. Kalil B, Ramaswamy S, Plant TM. The distribution of substance P and kisspeptin in the mediobasal hypothalamus of the male rhesus monkey and a comparison of intravenous administration of these peptides to release GnRH as reflected by LH secretion. Neuroendocrinology. (2016) 103:711–23. doi: 10.1159/000442420
48. Kirilov M, Clarkson J, Liu X, Roa J, Campos P, Porteous R, et al. Dependence of fertility on kisspeptin-Gpr54 signaling at the GnRH neuron. Nat Commun. (2013) 4:2492. doi: 10.1038/ncomms3492
49. Roth C, Leonhardt S, Theiling K, Lakomek M, Jarry H, Wuttke W. Ontogeny of the GNRH-, glutaminase- and glutamate decarboxylase-gene expression in the hypothalamus of female rats. Brain Res Dev Brain Res. (1998) 110:105–14. doi: 10.1016/S0165-3806(98)00102-3
50. Smith JT, Cunningham MJ, Rissman EF, Clifton DK, Steiner RA. Regulation of Kiss1 gene expression in the brain of the female mouse. Endocrinology. (2005) 146:3686–92. doi: 10.1210/en.2005-0488
51. Clarkson J, Herbison AE. Postnatal development of kisspeptin neurons in mouse hypothalamus; sexual dimorphism and projections to gonadotropin-releasing hormone neurons. Endocrinology. (2006) 147:5817–25. doi: 10.1210/en.2006-0787
52. Adachi S, Yamada S, Takatsu Y, Matsui H, Kinoshita M, Takase K, et al. Involvement of anteroventral periventricular metastin/kisspeptin neurons in estrogen positive feedback action on luteinizing hormone release in female rats. J Reprod Dev. (2007) 53:367–78. doi: 10.1262/jrd.18146
53. Ohkura S, Takase K, Matsuyama S, Mogi K, Ichimaru T, Wakabayashi Y, et al. Gonadotrophin-releasing hormone pulse generator activity in the hypothalamus of the goat. J Neuroendocrinol. (2009) 21:813–21. doi: 10.1111/j.1365-2826.2009.01909.x
54. Tomikawa J, Homma T, Tajima S, Shibata T, Inamoto Y, Takase K, et al. Molecular characterization and estrogen regulation of hypothalamic KISS1 gene in the pig. Biol Reprod. (2010) 82:313–9. doi: 10.1095/biolreprod.109.079863
55. Inoue N, Sasagawa K, Ikai K, Sasaki Y, Tomikawa J, Oishi S, et al. Kisspeptin neurons mediate reflex ovulation in the musk shrew (Suncus murinus). Proc Natl Acad Sci USA. (2011) 108:17527–32. doi: 10.1073/pnas.1113035108
56. Matsuda F, Nakatsukasa K, Suetomi Y, Naniwa Y, Ito D, Inoue N, et al. The LH surge-generating system is functional in male goats as in females: involvement of kisspeptin neurones in the medial preoptic area. J Neuroendocrinol. (2014) 27:57–65. doi: 10.1111/jne.12235
57. Watanabe Y, Uenoyama Y, Suzuki J, Takase K, Suetomi Y, Ohkura S, et al. Oestrogen-induced activation of preoptic kisspeptin neurones may be involved in the luteinizing hormone surge in male and female Japanese monkeys. J Neuroendocrinol. (2014) 26:909–17. doi: 10.1111/jne.12227
58. Hassaneen ASA, Naniwa Y, Suetomi Y, Matsuyama S, Kimura K, Ieda N, et al. Immunohistochemical characterization of the arcuate kisspeptin/neurokinin B/dynorphin (KNDy) and preoptic kisspeptin neuronal populations in the hypothalamus during the estrous cycle in heifers. J Reprod Dev. (2016) 62:471–7. doi: 10.1262/jrd.2016-075
59. Smith JT, Shahab M, Pereira A, Pau KY, Clarke IJ. Hypothalamic expression of KISS1 and gonadotropin inhibitory hormone genes during the menstrual cycle of a non-human primate. Biol Reprod. (2010) 83:568–77. doi: 10.1095/biolreprod.110.085407
60. Smith JT, Li Q, Pereira A, Clarke IJ. Kisspeptin neurons in the ovine arcuate nucleus and preoptic area are involved in the preovulatory luteinizing hormone surge. Endocrinology. (2009) 150:5530–8. doi: 10.1210/en.2009-0712
61. Gu GB, Simerly RB. Projections of the sexually dimorphic anteroventral periventricular nucleus in the female rat. J Comp Neurol. (1997) 384:142–64.
62. Simerly RB. Organization and regulation of sexually dimorphic neuroendocrine pathways. Behav Brain Res. (1998) 92:195–203. doi: 10.1016/S0166-4328(97)00191-5
63. Wiegand SJ, Terasawa E. Discrete lesions reveal functional heterogeneity of suprachiasmatic structures in regulation of gonadotropin secretion in the female rat. Neuroendocrinology. (1982) 34:395–404. doi: 10.1159/000123335
64. Wiegand SJ, Terasawa E, Bridson WE, Goy RW. Effects of discrete lesions of preoptic and suprachiasmatic structures in the female rat. Alterations in the feedback regulation of gonadotropin secretion. Neuroendocrinology. (1980) 31:147–57. doi: 10.1159/000123066
65. Clarkson J, Han SY, Piet R, McLennan T, Kane GM, Ng J, et al. Definition of the hypothalamic GnRH pulse generator in mice. Proc Natl Acad Sci USA. (2017) 114:E10216–23. doi: 10.1073/pnas.1713897114
66. Uenoyama Y, Inoue N, Pheng V, Homma T, Takase K, Yamada S, et al. Ultrastructural evidence of kisspeptin-gonadotrophin-releasing hormone interaction in the median eminence of female rats: Implication of axo-axonal regulation of GnRH release. J Neuroendocrinol. (2011) 23:863–70. doi: 10.1111/j.1365-2826.2011.02199.x
67. Matsuyama S, Ohkura S, Mogi K, Wakabayashi Y, Mori Y, Tsukamura H, et al. Morphological evidence for direct interaction between kisspeptin and gonadotropin-releasing hormone neurons at the median eminence of the male goat: an immunoelectron microscopic study. Neuroendocrinology. (2011) 94:323–32. doi: 10.1159/000331576
68. Yeo SH, Herbison AE. Projections of arcuate nucleus and rostral periventricular kisspeptin neurons in the adult female mouse brain. Endocrinology. (2011) 152:2387–99. doi: 10.1210/en.2011-0164
69. Merkley CM, Coolen LM, Goodman RL, Lehman MN. Evidence for changes in numbers of synaptic inputs onto KNDy and GnRH neurones during the preovulatory LH Surge in the Ewe. J Neuroendocrinol. (2015) 27:624–35. doi: 10.1111/jne.12293
70. Clarkson J, d'Anglemont de Tassigny X, Moreno AS, Colledge WH, Herbison AE. Kisspeptin-GPR54 signaling is essential for preovulatory gonadotropin-releasing hormone neuron activation and the luteinizing hormone surge. J Neurosci. (2008) 28:8691–7. doi: 10.1523/JNEUROSCI.1775-08.2008
71. Homma T, Sakakibara M, Yamada S, Kinoshita M, Iwata K, Tomikawa J, et al. Significance of neonatal testicular sex steroids to defeminize anteroventral periventricular kisspeptin neurons and the GnRH/LH surge system in male rats. Biol Reprod. (2009) 81:1216–25. doi: 10.1095/biolreprod.109.078311
72. Tsukamura H, Homma T, Tomikawa J, Uenoyama Y, Maeda K. Sexual differentiation of kisspeptin neurons responsible for sex difference in gonadotropin release in rats. Ann N Y Acad Sci. (2010) 1200:95–103. doi: 10.1111/j.1749-6632.2010.05645.x
73. Tsukamura H, Maeda KI, Uenoyama Y. Fetal/perinatal programming causing sexual dimorphism of the kisspeptin–GnRH neuronal network. in: Herbison AE, and Plant TM, editors. The GnRH Neuron and Its Control. Hoboken, NJ: Wiley (2018). p. 43–60. doi: 10.1002/9781119233275.ch3
74. Treen AK, Luo V, Chalmers JA, Dalvi PS, Tran D, Ye W, et al. Divergent regulation of ER and Kiss genes by 17β-estradiol in hypothalamic ARC versus AVPV models. Mol Endocrinol. (2016) 30:217–33. doi: 10.1210/me.2015-1189
75. Yip SH, Boehm U, Herbison AE, Campbell RE. Conditional viral tract tracing delineates the projections of the distinct kisspeptin neuron populations to gonadotropin-releasing hormone (GnRH) neurons in the mouse. Endocrinology. (2015) 156:2582–94. doi: 10.1210/en.2015-1131
76. Qiu J, Nestor CC, Zhang C, Padilla SL, Palmiter RD, Kelly MJ, et al. High-frequency stimulation-induced peptide release synchronizes arcuate kisspeptin neurons and excites GnRH neurons. Elife. (2016) 5:e16246. doi: 10.7554/eLife.16246
77. Estrada KM, Clay CM, Pompolo S, Smith JT, Clarke IJ. Elevated KiSS-1 expression in the arcuate nucleus prior to the cyclic preovulatory gonadotrophin-releasing hormone/lutenising hormone surge in the ewe suggests a stimulatory role for kisspeptin in oestrogen-positive feedback. J Neuroendocrinol. (2006) 18:806–9. doi: 10.1111/j.1365-2826.2006.01485.x
78. Krey LC, Butler WR, Knobil E. Surgical disconnection of the medial basal hypothalamus and pituitary function in the rhesus monkey. I. Gonadotropin secretion. Endocrinology. (1975) 96:1073–87. doi: 10.1210/endo-96-5-1073
79. Plant TM. A comparison of the neuroendocrine mechanisms underlying the initiation of the preovulatory LH surge in the human, Old World monkey and rodent. Front Neuroendocrinol. (2012) 33:160–8. doi: 10.1016/j.yfrne.2012.02.002
80. Blache D, Fabre-Nys CJ, Venier G. Ventromedial hypothalamus as a target for oestradiol action on proceptivity, receptivity and luteinizing hormone surge of the ewe. Brain Res. (1991) 546:241–9. doi: 10.1016/0006-8993(91)91488-M
81. Caraty A, Fabre-Nys C, Delaleu B, Locatelli A, Bruneau G, Karsch FJ, et al. Evidence that the mediobasal hypothalamus is the primary site of action of estradiol in inducing the preovulatory gonadotropin releasing hormone surge in the ewe. Endocrinology. (1998) 139:1752–60. doi: 10.1210/endo.139.4.5904
82. Takase K, Uenoyama Y, Inoue N, Matsui H, Yamada S, Shimizu M, et al. Possible role of oestrogen in pubertal increase of Kiss1/kisspeptin expression in discrete hypothalamic areas of female rats. J Neuroendocrinol. (2009) 21:527–37. doi: 10.1111/j.1365-2826.2009.01868.x
83. Pennefather JN, Lecci A, Candenas ML, Patak E, Pinto FM, Maggi CA. Tachykinins and tachykinin receptors: a growing family. Life Sci. (2004) 74:1445–63. doi: 10.1016/j.lfs.2003.09.039
84. Guran T, Tolhurst G, Bereket A, Rocha N, Porter K, Turan S, et al. Hypogonadotropic hypogonadism due to a novel missense mutation in the first extracellular loop of the neurokinin B receptor. J Clin Endocrinol Metab. (2009) 94:3633–9. doi: 10.1210/jc.2009-0551
85. Topaloglu AK, Reimann F, Guclu M, Yalin AS, Kotan LD, Porter KM, et al. TAC3 and TACR3 mutations in familial hypogonadotropic hypogonadism reveal a key role for Neurokinin B in the central control of reproduction. Nat Genet. (2009) 41:354–8. doi: 10.1038/ng.306
86. Gianetti E, Tusset C, Noel SD, Au MG, Dwyer AA, Hughes VA, et al. TAC3/TACR3 mutations reveal preferential activation of gonadotropin-releasing hormone release by neurokinin B in neonatal life followed by reversal in adulthood. J Clin Endocrinol Metab. (2010) 95:2857–67. doi: 10.1210/jc.2009-2320
87. Goodman RL, Lehman MN, Smith JT, Coolen LM, de Oliveira CV, Jafarzadehshirazi MR, et al. Kisspeptin neurons in the arcuate nucleus of the ewe express both dynorphin A and neurokinin B. Endocrinology. (2007) 148:5752–60. doi: 10.1210/en.2007-0961
88. Navarro VM, Gottsch ML, Chavkin C, Okamura H, Clifton DK, Steiner RA. Regulation of gonadotropin-releasing hormone secretion by kisspeptin/dynorphin/neurokinin B neurons in the arcuate nucleus of the mouse. J Neurosci. (2009) 29:11859–66. doi: 10.1523/JNEUROSCI.1569-09.2009
89. Wakabayashi Y, Nakada T, Murata K, Ohkura S, Mogi K, Navarro VM, et al. Neurokinin B and dynorphin A in kisspeptin neurons of the arcuate nucleus participate in generation of periodic oscillation of neural activity driving pulsatile gonadotropin-releasing hormone secretion in the goat. J Neurosci. (2010) 30:3124–32. doi: 10.1523/JNEUROSCI.5848-09.2010
90. Lehman MN, Coolen LM, Goodman RL. Minireview: kisspeptin/neurokinin B/dynorphin (KNDy) cells of the arcuate nucleus: a central node in the control of gonadotropin-releasing hormone secretion. Endocrinology. (2010) 151:3479–89. doi: 10.1210/en.2010-0022
91. Goodman RL, Hileman SM, Nestor CC, Porter KL, Connors JM, Hardy SL, et al. Kisspeptin, neurokinin B, and dynorphin act in the arcuate nucleus to control activity of the GnRH pulse generator in ewes. Endocrinology. (2013) 154:4259–69. doi: 10.1210/en.2013-1331
92. Goodman RL, Coolen LM, Lehman MN. A role for neurokinin B in pulsatile GnRH secretion in the ewe. Neuroendocrinology. (2014) 99:18–32. doi: 10.1159/000355285
93. Weems PW, Witty CF, Amstalden M, Coolen LM, Goodman RL, Lehman MN. Kappa-opioid receptor is colocalized in GnRH and KNDy cells in the female ovine and rat brain. Endocrinology. (2016) 157:2367–79. doi: 10.1210/en.2015-1763
94. Weems PW, Coolen LM, Hileman SM, Hardy S, McCosh RB, Goodman RL, et al. Evidence that dynorphin acts upon KNDy and GnRH neurons during GnRH pulse termination in the ewe. Endocrinology. (2018) 159:3187–99. doi: 10.1210/en.2018-00435
95. Goodman RL, Ohkura S, Okamura H, Coolen LM, Lehman MN. KNDy hypothesis for generation of GnRH pulses: evidence from sheep and goats. in: Herbison AE, and Plant TM, editors. The GnRH Neuron and Its Control. Hoboken, NJ: Wiley (2018). p. 289–324. doi: 10.1002/9781119233275.ch12
96. Nakahara T, Uenoyama Y, Iwase A, Oishi S, Nakamura S, Minabe S, et al. Chronic peripheral administration of kappa-opioid receptor antagonist advances puberty onset associated with acceleration of pulsatile luteinizing hormone secretion in female rats. J Reprod Dev. (2013) 59:479–84. doi: 10.1262/jrd.2013-046
97. Semaan SJ, Kauffman AS. Daily successive changes in reproductive gene expression and neuronal activation in the brains of pubertal female mice. Mol Cell Endocrinol. (2015) 401:84–97. doi: 10.1016/j.mce.2014.11.025
98. Gill JC, Navarro VM, Kwong C, Noel SD, Martin C, Xu S, et al. Increased neurokinin B (Tac2) expression in the mouse arcuate nucleus is an early marker of pubertal onset with differential sensitivity to sex steroid-negative feedback than Kiss1. Endocrinology. (2012) 153:4883–93. doi: 10.1210/en.2012-1529
99. Nestor CC, Briscoe AM, Davis SM, Valent M, Goodman RL, Hileman SM. Evidence of a role for kisspeptin and neurokinin B in puberty of female sheep. Endocrinology. (2012) 153:2756–65. doi: 10.1210/en.2011-2009
100. Ikegami K, Minabe S, Ieda N, Goto T, Sugimoto A, Nakamura S, et al. Evidence of involvement of neurone-glia/neurone-neurone communications via gap junctions in synchronised activity of KNDy neurones. J Neuroendocrinol. (2017) 29:1–14. doi: 10.1111/jne.12480
101. Navarro VM, Gottsch ML, Wu M, Garcia-Galiano D, Hobbs SJ, Bosch MA, et al. Regulation of NKB pathways and their roles in the control of Kiss1 neurons in the arcuate nucleus of the male mouse. Endocrinology. (2011) 152:4265–75. doi: 10.1210/en.2011-1143
102. Houang M, Gourmelen M, Moatti L, Le BY, Garabedian EN, Denoyelle F. Hypogonadotrophic hypogonadism associated with prelingual deafness due to a connexin 26 gene mutation. J Pediatr Endocrinol Metab. (2002) 15:219–23. doi: 10.1515/JPEM.2002.15.2.219
103. Foster DL, Ryan KD. Endocrine mechanisms governing transition into adulthood: a marked decrease in inhibitory feedback action of estradiol on tonic secretion of luteinizing hormone in the lamb during puberty. Endocrinology. (1979) 105:896–904. doi: 10.1210/endo-105-4-896
104. Ramirez DV, McCann SM. Comparison of the regulation of luteinizing hormone (LH) secretion in immature and adult rats. Endocrinology. (1963) 72:452–64. doi: 10.1210/endo-72-3-452
105. Eldridge JC, McPherson JC III, Mahesh VB. Maturation of the negative feedback control of gonadotropin secretion in the female rat. Endocrinology. (1974) 94:1536–40. doi: 10.1210/endo-94-6-1536
106. Mayer C, Acosta-Martinez M, Dubois SL, Wolfe A, Radovick S, Boehm U, et al. Timing and completion of puberty in female mice depend on estrogen receptor alpha-signaling in kisspeptin neurons. Proc Natl Acad Sci USA. (2010) 107:22693–8. doi: 10.1073/pnas.1012406108
107. Dubois SL, Wolfe A, Radovick S, Boehm U, Levine JE. Estradiol restrains prepubertal gonadotropin secretion in female mice via activation of ERα in kisspeptin neurons. Endocrinology. (2016) 157:1546–54. doi: 10.1210/en.2015-1923
108. Plant TM. A study of the role of the postnatal testes in determining the ontogeny of gonadotropin secretion in the male rhesus monkey (Macaca mulatta). Endocrinology. (1985) 116:1341–50. doi: 10.1210/endo-116-4-1341
109. Plant TM, Barker-Gibb ML. Neurobiological mechanisms of puberty in higher primates. Hum Reprod Update. (2004) 10:67–77. doi: 10.1093/humupd/dmh001
110. Chongthammakun S, Claypool LE, Terasawa E. Ovariectomy increases in vivo luteinizing hormone-releasing hormone release in pubertal, but not prepubertal, female rhesus monkeys. J Neuroendocrinol. (1993) 5:41–50. doi: 10.1111/j.1365-2826.1993.tb00362.x
111. Chongthammakun S, Terasawa E. Negative feedback effects of estrogen on luteinizing hormone-releasing hormone release occur in pubertal, but not prepubertal, ovariectomized female rhesus monkeys. Endocrinology. (1993) 132:735–43. doi: 10.1210/endo.132.2.8425492
112. Terasawa E, Fernandez DL. Neurobiological mechanisms of the onset of puberty in primates. Endocr Rev. (2001) 22:111–51. doi: 10.1210/er.22.1.111
113. Terasawa E, Guerriero KA, Plant TM. Kisspeptin and puberty in mammals. Adv Exp Med Biol. (2013) 784:253–73. doi: 10.1007/978-1-4614-6199-9_12
114. El Majdoubi M, Sahu A, Ramaswamy S, Plant TM. Neuropeptide Y: a hypothalamic brake restraining the onset of puberty in primates. Proc Natl Acad Sci USA. (2000) 97:6179–84. doi: 10.1073/pnas.090099697
115. Foster DL, Olster DH. Effect of restricted nutrition on puberty in the lamb: patterns of tonic luteinizing hormone (LH) secretion and competency of the LH surge system. Endocrinology. (1985) 116:375–81. doi: 10.1210/endo-116-1-375
116. Bronson FH. Food-restricted, prepubertal, female rats: rapid recovery of luteinizing hormone pulsing with excess food, and full recovery of pubertal development with gonadotropin-releasing hormone. Endocrinology. (1986) 118:2483–7. doi: 10.1210/endo-118-6-2483
117. Sisk CL, Bronson FH. Effects of food restriction and restoration on gonadotropin and growth hormone secretion in immature male rats. Biol Reprod. (1986) 35:554–61. doi: 10.1095/biolreprod35.3.554
118. Frisch RE, Revelle R. Height and weight at menarche and a hypothesis of menarche. Arch Dis Childh. (1971) 46:695–701. doi: 10.1136/adc.46.249.695
119. Tanner JM. Earlier maturation in man. Sci Am. (1968) 218:21–7. doi: 10.1038/scientificamerican0168-21
121. Castellano JM, Navarro VM, Fernandez-Fernandez R, Nogueiras R, Tovar S, Roa J, et al. Changes in hypothalamic KiSS-1 system and restoration of pubertal activation of the reproductive axis by kisspeptin in undernutrition. Endocrinology. (2005) 146:3917–25. doi: 10.1210/en.2005-0337
122. Kalamatianos T, Grimshaw SE, Poorun R, Hahn JD, Coen CW. Fasting reduces KiSS-1 expression in the anteroventral periventricular nucleus (AVPV): effects of fasting on the expression of KiSS-1 and neuropeptide Y in the AVPV or arcuate nucleus of female rats. J Neuroendocrinol. (2008) 20:1089–97. doi: 10.1111/j.1365-2826.2008.01757.x
123. Matsuzaki T, Iwasa T, Kinouchi R, Yoshida S, Murakami M, Gereltsetseg G, et al. Fasting reduces the kiss1 mRNA levels in the caudal hypothalamus of gonadally intact adult female rats. Endocr J. (2011) 58:1003–12. doi: 10.1507/endocrj.K11E-131
124. Luque RM, Kineman RD, Tena-Sempere M. Regulation of hypothalamic expression of KiSS-1 and GPR54 genes by metabolic factors: analyses using mouse models and a cell line. Endocrinology. (2007) 148:4601–11. doi: 10.1210/en.2007-0500
125. Cagampang FR, Maeda K, Yokoyama A, Ota K. Effect of food deprivation on the pulsatile LH release in the cycling and ovariectomized female rat. Horm Metab Res. (1990) 22:269–72. doi: 10.1055/s-2007-1004900
126. Cagampang FR, Maeda KI, Tsukamura H, Ohkura S, Ota K. Involvement of ovarian steroids and endogenous opioids in the fasting-induced suppression of pulsatile LH release in ovariectomized rats. J Endocrinol. (1991) 129:321–8. doi: 10.1677/joe.0.1290321
127. Deura C, Minabe S, Ikegami K, Inoue N, Uenoyama Y, Maeda KI, et al. Morphological analysis for neuronal pathway from the hindbrain ependymocytes to the hypothalamic kisspeptin neurons. J Reprod Dev. (2019) 65:129–37. doi: 10.1262/jrd.2018-122
128. Kinoshita M, Moriyama R, Tsukamura H, Maeda KI. A rat model for the energetic regulation of gonadotropin secretion: role of the glucose-sensing mechanism in the brain. Domest Anim Endocrinol. (2003) 25:109–20. doi: 10.1016/S0739-7240(03)00050-X
129. Maekawa F, Toyoda Y, Torii N, Miwa I, Thompson RC, Foster DL, et al. Localization of glucokinase-like immunoreactivity in the rat lower brain stem: for possible location of brain glucose-sensing mechanisms. Endocrinology. (2000) 141:375–84. doi: 10.1210/endo.141.1.7234
130. Minabe S, Deura C, Ikegami K, Goto T, Sanbo M, Hirabayashi M, et al. Pharmacological and morphological evidence of AMPK-mediated energy sensing in the lower brain stem ependymocytes to control reproduction in female rodents. Endocrinology. (2015) 156:2278–87. doi: 10.1210/en.2014-2018
131. Moriyama R, Tsukamura H, Kinoshita M, Okazaki H, Kato Y, Maeda K. In vitro increase in intracellular calcium concentrations induced by low or high extracellular glucose levels in ependymocytes and serotonergic neurons of the rat lower brainstem. Endocrinology. (2004) 145:2507–15. doi: 10.1210/en.2003-1191
132. Chehab FF. Leptin as a regulator of adipose mass and reproduction. Trends Pharmacol Sci. (2000) 21:309–14. doi: 10.1016/S0165-6147(00)01514-5
133. Frisch RE. Body fat, puberty and fertility. Biol Rev Camb Philos Soc. (1984) 59:161–88. doi: 10.1111/j.1469-185X.1984.tb00406.x
134. Elmquist JK, Bjorbaek C, Ahima RS, Flier JS, Saper CB. Distributions of leptin receptor mRNA isoforms in the rat brain. J Comp Neurol. (1998) 395:535–47.
135. Smith JT, Acohido BV, Clifton DK, Steiner RA. KiSS-1 neurones are direct targets for leptin in the ob/ob mouse. J Neuroendocrinol. (2006) 18:298–303. doi: 10.1111/j.1365-2826.2006.01417.x
136. Chehab FF, Lim ME, Lu R. Correction of the sterility defect in homozygous obese female mice by treatment with the human recombinant leptin. Nat Genet. (1996) 12:318–20. doi: 10.1038/ng0396-318
137. Chehab FF, Mounzih K, Lu R, Lim ME. Early onset of reproductive function in normal female mice treated with leptin. Science. (1997) 275:88–90. doi: 10.1126/science.275.5296.88
138. Ahima RS, Dushay J, Flier SN, Prabakaran D, Flier JS. Leptin accelerates the onset of puberty in normal female mice. J Clin Invest. (1997) 99:391–5. doi: 10.1172/JCI119172
139. Cheung CC, Thornton JE, Kuijper JL, Weigle DS, Clifton DK, Steiner RA. Leptin is a metabolic gate for the onset of puberty in the female rat. Endocrinology. (1997) 138:855–8. doi: 10.1210/endo.138.2.5054
140. Gruaz NM, Lalaoui M, Pierroz DD, Englaro P, Sizonenko PC, Blum WF, et al. Chronic administration of leptin into the lateral ventricle induces sexual maturation in severely food-restricted female rats. J Neuroendocrinol. (1998) 10:627–33. doi: 10.1046/j.1365-2826.1998.00247.x
141. Carlsson B, Ankarberg C, Rosberg S, Norjavaara E, Albertsson-Wikland K, Carlsson LM. Serum leptin concentrations in relation to pubertal development. Arch Dis Childh. (1997) 77:396–400. doi: 10.1136/adc.77.5.396
142. Plant TM, Durrant AR. Circulating leptin does not appear to provide a signal for triggering the initiation of puberty in the male rhesus monkey (Macaca mulatta). Endocrinology. (1997) 138:4505–8. doi: 10.1210/endo.138.10.5574
143. Arslanian S, Suprasongsin C, Kalhan SC, Drash AL, Brna R, Janosky JE. Plasma leptin in children: relationship to puberty, gender, body composition, insulin sensitivity, and energy expenditure. Metabolism. (1998) 47:309–12. doi: 10.1016/S0026-0495(98)90262-1
144. Urbanski HF, Pau KY. A biphasic developmental pattern of circulating leptin in the male rhesus macaque (Macaca mulatta). Endocrinology. (1998) 139:2284–6. doi: 10.1210/endo.139.5.5962
145. Ahima RS, Prabakaran D, Flier JS. Postnatal leptin surge and regulation of circadian rhythm of leptin by feeding. Implications for energy homeostasis and neuroendocrine function. J Clin Invest. (1998) 101:1020–7. doi: 10.1172/JCI1176
146. Donato J Jr, Cravo RM, Frazao R, Gautron L, Scott MM, Lachey J, et al. Leptin's effect on puberty in mice is relayed by the ventral premammillary nucleus and does not require signaling in Kiss1 neurons. J Clin Invest. (2011) 121:355–68. doi: 10.1172/JCI45106
147. Manfredi-Lozano M, Roa J, Ruiz-Pino F, Piet R, Garcia-Galiano D, Pineda R, et al. Defining a novel leptin-melanocortin-kisspeptin pathway involved in the metabolic control of puberty. Mol Metab. (2016) 5:844–57. doi: 10.1016/j.molmet.2016.08.003
148. Ross RA, Leon S, Madara JC, Schafer D, Fergani C, Maguire CA, et al. PACAP neurons in the ventral premammillary nucleus regulate reproductive function in the female mouse. Elife. (2018) 7:e35960. doi: 10.7554/eLife.35960
149. Urbanski HF. Leptin and puberty. Trends Endocrinol Metab. (2001) 12:428–9. doi: 10.1016/S1043-2760(01)00505-7
150. Horvath TL, Diano S, Sotonyi P, Heiman M, Tschop M. Minireview: ghrelin and the regulation of energy balance–a hypothalamic perspective. Endocrinology. (2001) 142:4163–9. doi: 10.1210/endo.142.10.8490
151. Kojima M, Hosoda H, Date Y, Nakazato M, Matsuo H, Kangawa K. Ghrelin is a growth-hormone-releasing acylated peptide from stomach. Nature. (1999) 402:656–60. doi: 10.1038/45230
152. Tena-Sempere M. Ghrelin and reproduction: ghrelin as novel regulator of the gonadotropic axis. Vitam Horm. (2008) 77:285–300. doi: 10.1016/S0083-6729(06)77012-1
153. Garcia MC, Lopez M, Alvarez CV, Casanueva F, Tena-Sempere M, Dieguez C. Role of ghrelin in reproduction. Reproduction. (2007) 133:531–40. doi: 10.1530/REP-06-0249
154. Avendano MS, Vazquez MJ, Tena-Sempere M. Disentangling puberty: novel neuroendocrine pathways and mechanisms for the control of mammalian puberty. Hum Reprod Update. (2017) 23:737–63. doi: 10.1093/humupd/dmx025
155. Fernandez-Fernandez R, Navarro VM, Barreiro ML, Vigo EM, Tovar S, Sirotkin AV, et al. Effects of chronic hyperghrelinemia on puberty onset and pregnancy outcome in the rat. Endocrinology. (2005) 146:3018–25. doi: 10.1210/en.2004-1622
156. Martini AC, Fernandez-Fernandez R, Tovar S, Navarro VM, Vigo E, Vazquez MJ, et al. Comparative analysis of the effects of ghrelin and unacylated ghrelin on luteinizing hormone secretion in male rats. Endocrinology. (2006) 147:2374–82. doi: 10.1210/en.2005-1422
157. Wren AM, Seal LJ, Cohen MA, Brynes AE, Frost GS, Murphy KG, et al. Ghrelin enhances appetite and increases food intake in humans. J Clin Endocrinol Metab. (2001) 86:5992. doi: 10.1210/jcem.86.12.8111
Keywords: dynorphin A, gonadotropin-releasing hormone (GnRH), GnRH pulse generator, kisspeptin, KNDy, neurokinin B, puberty
Citation: Uenoyama Y, Inoue N, Nakamura S and Tsukamura H (2019) Central Mechanism Controlling Pubertal Onset in Mammals: A Triggering Role of Kisspeptin. Front. Endocrinol. 10:312. doi: 10.3389/fendo.2019.00312
Received: 17 December 2018; Accepted: 30 April 2019;
Published: 21 May 2019.
Edited by:
Takayoshi Ubuka, Waseda University, JapanReviewed by:
Chad D. Foradori, Auburn University, United StatesHitoshi Ozawa, Nippon Medical School, Japan
Aleisha Marie Moore, Kent State University, United States
Casey Nestor, North Carolina State University, United States
Copyright © 2019 Uenoyama, Inoue, Nakamura and Tsukamura. This is an open-access article distributed under the terms of the Creative Commons Attribution License (CC BY). The use, distribution or reproduction in other forums is permitted, provided the original author(s) and the copyright owner(s) are credited and that the original publication in this journal is cited, in accordance with accepted academic practice. No use, distribution or reproduction is permitted which does not comply with these terms.
*Correspondence: Yoshihisa Uenoyama, dWVub3lhbWEmI3gwMDA0MDtuYWdveWEtdS5qcA==