- 1Department of Clinical and Experimental Medicine, University of Pisa, Pisa, Italy
- 2Sant'Anna School of Advanced Studies, Institute of Life Sciences, Pisa, Italy
Pharmacological and dietary interventions targeting postprandial glycemia have proved effective in reducing the risk for type 2 diabetes and its cardiovascular complications. Besides meal composition and size, the timing of macronutrient consumption during a meal has been recently recognized as a key regulator of postprandial glycemia. Emerging evidence suggests that premeal consumption of non-carbohydrate macronutrients (i.e., protein and fat “preloads”) can markedly reduce postprandial glycemia by delaying gastric emptying, enhancing glucose-stimulated insulin release, and decreasing insulin clearance. The same improvement in glucose tolerance is achievable by optimal timing of carbohydrate ingestion during a meal (i.e., carbohydrate-last meal patterns), which minimizes the risk of body weight gain when compared with nutrient preloads. The magnitude of the glucose-lowering effect of preload-based nutritional strategies is greater in type 2 diabetes than healthy subjects, being comparable and additive to current glucose-lowering drugs, and appears sustained over time. This dietary approach has also shown promising results in pathological conditions characterized by postprandial hyperglycemia in which available pharmacological options are limited or not cost-effective, such as type 1 diabetes, gestational diabetes, and impaired glucose tolerance. Therefore, preload-based nutritional strategies, either alone or in combination with pharmacological treatments, may offer a simple, effective, safe, and inexpensive tool for the prevention and management of postprandial hyperglycemia. Here, we survey these novel physiological insights and their therapeutic implications for patients with diabetes mellitus and altered glucose tolerance.
Conceptual Framework
Type 2 diabetes (T2D) affects more than 400 million people worldwide and its prevalence is constantly increasing (1). The first metabolic alteration detectable in the progression of the disease is typically a loss of postprandial glucose control (2, 3), which is an independent risk factor for T2D (4, 5) and its complications (5–11). Targeting postprandial glycemia has proved effective for reducing the incidence of T2D (12–14). However, pharmacological control of postprandial glucose in the prediabetic stage rises ethical and economic concerns, and novel cost-effective treatments are required.
Medical nutrition therapy is recommended as first line treatment for prediabetes and T2D (15, 16) and may be a useful tool for improving glucose tolerance. In fact, meal composition and size have a profound impact on the physiological processes that regulate postprandial glycemia, such as gastric emptying and intestinal glucose absorption, pancreatic, and gut hormone release, hepatic insulin extraction, glucose uptake by insulin-sensitive tissues, and endogenous glucose production (17). Adherence to lifelong nutritional interventions involving energy restriction is often poor, and therefore alternative dietary strategies focusing on eating patterns are gaining growing interest.
One emerging approach is premeal ingestion of non-carbohydrate macronutrients (namely protein and fat), which has been shown to reduce postprandial hyperglycemia in both T2D and at-risk individuals (Figure 1A) (20). It has long been known that non-carbohydrate components of the meal can markedly influence postprandial glycemia (18, 21–25). More recently, the magnitude of the glucose-lowering effect of protein and fat was found to be even greater when these macronutrients are consumed before carbohydrates than mixed with them (22, 23). Based on these observations, “preloading” each meal with protein and fat or tailoring the sequence of macronutrient ingestion (i.e., consuming protein- and fat-rich food before carbohydrate) has been proposed as a novel strategy for the prevention and management of postprandial hyperglycemia.
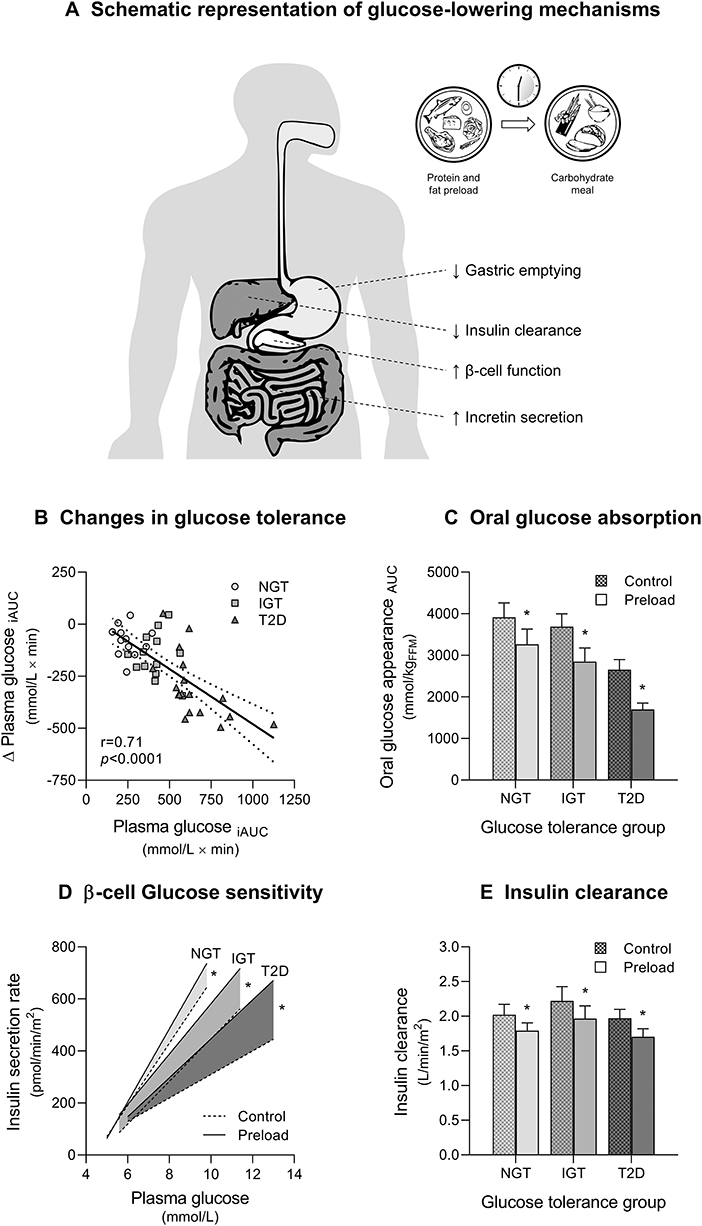
Figure 1. Glucose-lowering effects of mixed nutrient preloads. (A) Schematic representation of postprandial glucose-lowering mechanisms activated by nutrient preloads. (B) Reduction of postload glucose excursions (Δ Plasma glucose iAUC) during a 75 g oral glucose tolerance test (OGTT) after a mixed nutrient preload is proportional to the degree of glucose tolerance (Plasma glucose iAUC) in subjects with normal glucose tolerance (NGT), impaired glucose tolerance (IGT), and type 2 diabetes (T2D). The physiological mechanisms responsible for the improvement in glucose tolerance during a 75 g OGTT preceded by a mixed nutrient preload (Preload) compared with a control OGTT (Control) are: (C) decreased rate of appearance of oral glucose for delayed gastric emptying; (D) enhanced glucose-stimulated insulin secretion (β-cell glucose sensitivity); and (E) reduced insulin clearance. Data are pooled from Trico et al. (18) and Trico et al. (19), for a total of 43 subjects examined (12 NGT, 13 IGT, and 18 T2D, except for B where T2D = 10). *p < 0.05 using paired Wilcoxon signed-rank test for within-group difference between Preload and Control.
The number of experimental studies in support to the clinical application of this promising nutritional approach is rapidly growing. However, gathering all available evidence is challenging given the different keywords used by different groups to define similar dietary strategies [e.g., protein/fat/nutrient premeal consumption (26, 27) or preload (19, 23, 28–30), food/meal/nutrient sequence (31, 32) or order (33)]. A further degree of complexity in the interpretation and comparison of different findings is produced by the heterogeneity of study designs. In fact, the effect of preload-based nutritional interventions on postprandial glycemia appears largely dependent upon different variables, such as preload composition, size, and timing of ingestion, test meal stimulus, and individual glucose tolerance status (20) (Figure 1B).
Herein, we review the available evidence on the acute and chronic effect of protein and fat preloads on postprandial glycemia throughout the whole spectrum of glucose tolerance, from diabetic patients to prediabetic and healthy individuals (Table 1), the underpinning physiological mechanisms, and the potential therapeutic relevance in different clinical settings.
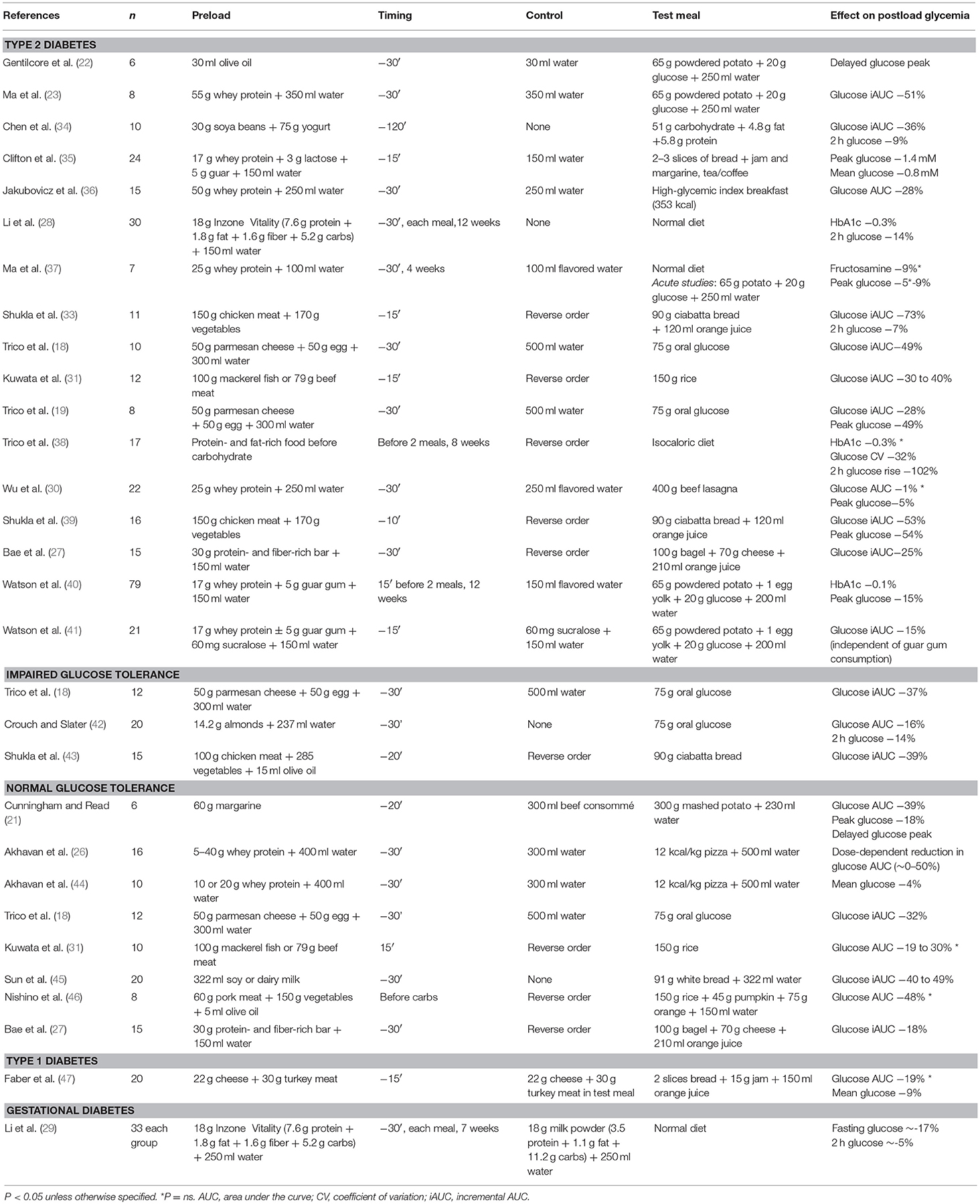
Table 1. Available studies examining the glucose-lowering effects of preload-based nutritional interventions.
Impact of Macronutrient Preloads on Postprandial Glycemia
Type 2 Diabetes
In subjects with type 2 diabetes (T2D), premeal consumption of protein and fat—either alone or in combination—has proved effective in decreasing or even normalizing postprandial hyperglycemia (Table 1). In 2006, Gentilcore et al. (22) demonstrated that 30 ml olive oil ingested 30 min before a carbohydrate meal was able to reduce and delay the postprandial glucose excursion in 6 diet-controlled T2D subjects. In 2009, the same group observed that a 55 g whey protein preload led to an even greater reduction in postprandial hyperglycemia in 8 diet-controlled T2D subjects (23). Thereafter, the ingestion of food rich in protein or fat before carbohydrate has been consistently associated with reduced postload glycemic excursions in T2D patients when compared with a carbohydrate-first meal pattern (18, 19, 27, 30, 31, 33–36, 39). On average, a ~40% reduction in glucose peak and a ~50–70% reduction in glucose excursion has been observed when protein and vegetables were consumed before carbohydrate, rather than mixed together or consumed after carbohydrate (33, 39). In our studies (18, 19), a small mixed protein and fat preload (50 g parmesan cheese and 50 g egg) was associated with a 30–50% reduction in glucose peak and overall excursion during an oral glucose tolerance test (OGTT) in well-controlled T2D patients. Similarly, Jakubowicz et al. (36) showed a ~30% reduction in postload glucose levels when 50 g whey protein were consumed before a high-glycemic index meal. Of note, the effect of macronutrient preloads on postprandial hyperglycemia in T2D appears comparable or even greater than that of current pharmacological therapy. In fact, Wu et al. (30) demonstrated that the glucose-lowering effect of a 25 g whey protein preload is similar to that of a dipeptidyl peptidase-4 (DPP-4) inhibitor (50 mg vildagliptin). Interestingly, combining the protein preload with vildagliptin was more effective for reducing postprandial glycemia compared with either treatment alone, thereby suggesting an additive effect. Further studies are needed to examine the interaction between nutrient preloads and oral hypoglycemic agents. In fact, preloading with saturated fat may lead to a deterioration in the glucose-lowering effect of DDP-4 inhibitors over time (48).
Prediabetic Subjects
In individuals with impaired glucose tolerance (IGT), a mixed nutrient preload ingested 30 min before an OGTT was able to decrease postload glucose concentrations by 37% when compared with a water preload (18) (Table 1). In agreement with this finding, Shukla et al. (43) observed a similar reduction (−39%) in postprandial glycemia in IGT subjects who consumed protein and vegetables before carbohydrate, compared with the same foods consumed in the reverse order (i.e., carbohydrate before protein and vegetables). In 20 subjects with IGT and/or isolated 1-h glucose ≥160 mg/dl, a small (14 g) almond preload reduced postprandial glycemia by 15% (42). Interestingly, the effect was greater in individuals with higher 2-h glucose concentrations, suggesting an inverse correlation between the individual degree of glucose tolerance and the magnitude of the glucose-lowering effect achievable with nutrient preloads (42).
Healthy Subjects
Nutrient preloads have been shown to reduce postprandial glucose concentrations even in subjects with normal glucose tolerance (NGT) (Table 1). Premeal consumption of either single amino acids (49, 50), whey protein (26, 44), a protein-enriched bar (27), dairy or soy milk (45), or margarine (21) before a carbohydrate-rich meal decreased postprandial glycemia in a dose-dependent manner in NGT subjects. Consistently, a mixed protein and fat preload reduced plasma glucose excursions after an OGTT by 32% in healthy young adults (18). Furthermore, the ingestion of either meat, fish, or vegetables before rice was able to decrease the postmeal glucose peak by ~50% and to delay it by 30–60 min when compared with eating the same food in the reverse order (i.e., rice first) (31, 46).
Type 1 Diabetes
Despite recent improvements in insulin therapy, a tight control of postprandial hyperglycemia remains difficult to achieve in type 1 diabetes, and is frequently associated with an increased risk of insulin-induced hypoglycemia. In this setting, a recent study by Faber et al. (47) has shown that protein and fat consumed 15 min prior to carbohydrates reduced by ~10% mean glucose levels in 20 type 1 diabetic children and adolescents. Remarkably, the nutrient preload was not associated with an increased risk of hypoglycemic episodes (47).
Gestational Diabetes
Glucose intolerance in pregnancy increases the risk of complications during delivery and the incidence of metabolic diseases later in life. In women with gestational diabetes (29), a treatment with low-carbohydrate preloads resulted in a significant reduction in both fasting and postprandial plasma glucose when compared with a dietary strategy implementing high-carbohydrate preloads. While low-carbohydrate preloads show promise, further studies are needed to determine efficacy and superiority of this approach.
Long-Term Efficacy, Safety, and Feasibility
Despite numerous experimental studies demonstrated the acute beneficial effect of protein and fat consumption before carbohydrate on postprandial glycemia, only a few studies evaluated the long-term efficacy, feasibility, and safety of preload-based dietary strategies. In T2D subjects, a 25 g whey protein preload consumed 30 min before each meal for 4 weeks showed a sustained effect on postprandial glucose, with a nearly significant reduction in fructosamine levels (p = 0.06) (37). Furthermore, a 12-week intervention with mixed nutrient preloads was associated with decreased postprandial glucose and glycated hemoglobin levels in T2D subjects (28, 40). Finally, a recent study found a reduction in both fasting and postprandial plasma glucose in women with gestational diabetes consuming low-carbohydrate preloads for ~9 ± 1 weeks (29).
Dietary strategies that require nutritional supplements (either food or artificial formulas) might be expensive and poorly accepted. Moreover, although previous studies did not show weight gain after chronic preload consumption (28, 37) possibly due to the compensatory satiating effect of protein (26, 28, 51–55), adding nutrient preloads to each meal may increase the total daily caloric intake, leading to an increase in body weight and diet-related metabolic alterations (56, 57).
To limit the risk of weight gain and to increase the feasibility and cost-effectiveness of dietary interventions exploiting the same glucose-lowering effects of nutrient preloads, other strategies have been proposed. Low-calorie fiber-rich preloads (e.g., guar gum, vegetables), alone or in combination with protein, have been shown to improve glucose tolerance in both healthy and diabetic subjects with negligible effects on body weight (40, 41, 58–61). Furthermore, our group (20, 38) and others (31–33, 39, 43, 46, 60) have recently proposed a nutritional approach that simply consists in manipulating the sequence of macronutrient ingestion during each meal. In a proof-of-concept study, postprandial glucose control significantly improved in T2D subjects instructed to consume protein- and fat-rich food before carbohydrate-rich food for 8 weeks under free-living conditions, with no differences in body weight, serum lipid profile and other metabolic markers (38). These data support carbohydrate-last meal patterns as an effective and safe behavioral strategy to reduce postprandial glucose excursions.
Physiological Mechanisms
Gastric Emptying
The effect of non-carbohydrate nutrients on glucose tolerance is largely dependent on their ability to delay gastric emptying (18, 19, 22, 23, 31, 37, 45). Gastric emptying modulates the rate of oral glucose delivery and absorption in the small intestine, and it can account for about one third of the variance in the early glucose excursion during an OGTT (62–65). Fat is the most potent macronutrient in slowing gastric emptying (21, 62, 66–68). In 1989, Cunningham and Read (21) showed that the effect of fat on gastric emptying is greater when fat is consumed prior to carbohydrate rather than mixed with it, suggesting that this effect is dependent on the digestion of fat to fatty acids (22, 69, 70). In 2009, a protein preload was also found to be effective in slowing gastric emptying (23), as later confirmed by other groups (44). The effect of protein preloads on gastric emptying appears to be smaller compared to fat (23, 44, 71) and substantially unchanged after a 4-week consumption (37). Fat and protein may exhibit an additive effect on gastric emptying. In fact, a mixed protein and fat preload can markedly reduce oral glucose absorption across different classes of glucose tolerance (from −16% in NGT to−42% in T2D) (18) (Figure 1C). Consistently, Kuwata et al. (31) observed that both meat and fish consumed before rice are able to delay gastric emptying, particularly in T2D (31).
Insulin Secretion and β Cell Function
The effect of nutrient preloads on postprandial glycemia largely depends on their insulinotropic action (18, 22, 23, 31, 33, 36, 50, 72). Among non-carbohydrate macronutrients, protein is the most effective in enhancing glucose-stimulated insulin secretion. Ma et al. (23) showed that a 55 g whey protein preload increases glucose-stimulated insulin release by 2- to 3-fold in T2D, and these results have been confirmed in both non-diabetic and T2D subjects (27, 36, 71). The insulinotropic effect of protein is dose-dependent (26) and likely mediated by both direct and incretin-mediated interactions of protein and amino acids with β cells (72–76). Although fat can enhance glucose-stimulated insulin secretion through direct and receptor-depended mechanisms (76–82), whether preloading with fat alone can affect insulin secretion is unclear. Indeed, the marked reduction in glucose responses due to the delay in gastric emptying after fat consumption usually leads to lower—rather than higher—absolute insulin levels in the early postprandial phase (21, 22). However, the insulin peak following a fat preload seems only delayed, but not reduced, even in the context of lower glucose levels (22). This observation suggests a positive—though small—effect of fat preloads on β cell function.
The effect of protein and fat on insulin secretion may be enforced by a synergistic interaction between the two classes of nutrients (18, 19, 83, 84). Our group showed that a mixed protein and fat preload increased plasma insulin levels during the first hour of an OGTT across the whole spectrum of glucose tolerance, despite lower glucose concentrations (18). The mixed preload increased the β cell responsiveness to plasma glucose (β cell glucose sensitivity) by 20% in NGT and prediabetic subjects, and almost doubled it in T2D subjects (Figure 1D). The greater enhancement of glucose-induced insulin secretion in T2D may be explained by a higher gradient of plasma amino acids after protein digestion and absorption in those subjects compared with healthy individuals (72, 85). Some studies with mixed preloads have reported different results (31, 39, 45, 46), likely due to a less rigorous estimation of β cell function (i.e., insulin and C-peptide levels were not adjusted for glucose concentrations) or to a stronger inhibition of gastric emptying by different preloads tested, which would minimize their impact on glucose-stimulated insulin secretion.
Insulin Clearance and Insulin Sensitivity
Besides a direct stimulation of insulin release by pancreatic β cells, macronutrient preloads may increase insulin bioavailability by reducing insulin degradation (“insulin clearance”), which mostly occurs in the liver. In fact, we reported an average ~10% reduction in insulin clearance during a 2-h OGTT after a mixed nutrient preload, without significant differences between NGT, IGT and T2D subjects (18) (Figure 1E). A subsequent experiment showed a 52% increase in plasma insulin levels in T2D subjects during a 5-h OGTT, which was due to the combination of a 28% lower insulin clearance and a 22% higher insulin secretion after the nutrient preload (19).
Nutrient preloads may also impact on postprandial glucose homeostasis by affecting peripheral and hepatic insulin action (“insulin sensitivity”). However, no evidence so far has shown a significant influence of nutrient preloads on insulin sensitivity (18, 19).
Incretin Hormones
Macronutrient preloads may exert their glucose-lowering effects by stimulating the release of gut hormones, such as the glucagon like peptide-1 (GLP-1) and the glucose-dependent insulinotropic polypeptide (GIP) (23, 44, 71, 86–91). GLP-1 and GIP are usually referred to as “incretin hormones” to underscore their stimulatory effect on pancreatic β cells, which is glucose-dependent, dose-dependent, and—only for GLP-1—largely preserved in T2D (86, 92). Furthermore, incretin hormones exhibit pleiotropic actions that include the inhibition of gastric emptying and appetite [by GLP-1 (86, 92)] and the reduction of hepatic insulin clearance [by GIP (90, 91)]. Preloading with either protein or fat alone enhanced GLP-1 concentrations in both T2D and healthy subjects, while only protein increased GIP levels in T2D (22, 23, 27, 36, 44, 71). When protein and fat were consumed together as a mixed preload, we observed an almost doubled GIP response, alongside with a modest but significant increase in plasma GLP-1 (18). These effects were comparable in individuals with different glucose tolerance, with a tendency to be more pronounced in IGT and T2D subjects (18, 19). Similarly, consuming meat or fish before carbohydrate resulted in higher GLP-1 and GIP concentrations in both T2D and healthy individuals, and these effects were greater in T2D (31).
Additional Mechanisms
Several additional mechanisms have been proposed to explain the effect of non-carbohydrate nutrients on postprandial glucose control. Along with GLP-1 and GIP, protein (23, 44) and fat (93, 94) can stimulate the release of other gut hormones, such as cholecystokinin (CCK) and peptide YY (PYY), which inhibit gastric emptying and appetite (95–98) and stimulate insulin secretion (99–101).
The sight, smell and taste of nutrients may trigger neural signals leading to anticipatory insulin release, which may partly explain the insulinotropic effect of nutrient preloads (102). However, the contribution of the so-called “cephalic phase” of insulin secretion on glucose tolerance is little (~1% of postprandial insulin release), transient (8–10 min from sensory stimulation) (102), and not supported by experimental evidence (18).
Furthermore, it should be noticed that the glucose-lowering effect of nutrient preloads occurs despite an increase in plasma glucagon levels (18, 19, 31, 71), which is expected to worsen glucose tolerance by promoting gluconeogenesis and glycogenolysis. However, endogenous glucose production was not affected by premeal consumption of protein and fat (18, 19), and the relevance of increased glucagon concentrations in this setting remains controversial.
Finally, the reduction of appetite and calorie intake following protein consumption, which is possibly mediated by the stimulation of GLP1 secretion, might contribute to weight loss after long-term consumption of protein preloads (51–55).
Conclusive Remarks and Future Perspectives
The experimental evidence discussed above indicates that premeal consumption of protein and fat can markedly reduce postprandial glycemia across the whole spectrum of glucose tolerance. The mechanisms underlying this effect include a delay in gastric emptying as well as an enhancement of glucose-stimulated insulin release and a decrease in hepatic insulin clearance, resulting, respectively, in slower glucose absorption and hyperinsulinemia (22, 23, 72). From the clinical perspective, the glucose-lowering effect of nutrient preloads is comparable in magnitude to that of current antihyperglycemic drugs (30), is proportionally greater in T2D than prediabetic and non-diabetic subjects (20), and appears to be sustained over time (37, 38). Preload-based dietary strategies can be useful in the management of T2D, either alone or in combination with pharmacological treatments, due to their additive effects (30). Furthermore, preload-based diets are of particular interest in clinical settings in which available pharmacological options are limited, including type 1 diabetes (47) and gestational diabetes (29), or not cost-effective, such as in the large number of individuals at high risk to develop T2D (18, 43). Remarkably, the same improvement in postprandial glycemia after nutrient preload consumption appears to be achievable by optimal timing of carbohydrate ingestion during a meal (i.e., carbohydrate-last meal pattern) (20, 31–33, 38, 39, 43, 46). This promising approach would avoid additional energy intake when compared with nutrient preloads, thereby minimizing the risk of body weight gain and diet-related metabolic alterations. Further refinement is required to determine the optimum timing and quantity of macronutrient consumption during a meal, as well as to standardize nutritional recommendations for targeting postprandial glycemia in different clinical settings. Larger studies are also needed to confirm the encouraging preliminary data on long-term efficacy, feasibility, and safety of these dietary approaches.
In summary, consistent experimental evidence suggests that preload-based nutritional strategies may offer a novel simple, effective, safe, and inexpensive therapeutic approach for the prevention and management of postprandial hyperglycemia and T2D.
Author Contributions
LN and AM: data collection and analysis, interpretation of results, and manuscript writing; DT: funding, study design, data collection and analysis, interpretation of results, manuscript writing, and final editing. All authors read and approved the final submitted version of the manuscript.
Funding
DT is funded by the EFSD Mentorship Programme.
Conflict of Interest Statement
The authors declare that the research was conducted in the absence of any commercial or financial relationships that could be construed as a potential conflict of interest.
Acknowledgments
The authors are grateful to Prof. Andrea Natali (Department of Clinical and Experimental Medicine, University of Pisa) for the insightful comments and suggestions.
References
1. Ingelfinger JR, Jarcho JA. Increase in the incidence of diabetes and its implications. N Engl J Med. (2017) 376:1473–4. doi: 10.1056/NEJMe1616575
2. Lebovitz HE. Postprandial hyperglycaemic state: importance and consequences. Diabetes Res Clin Pract. (1998) 40(Suppl.):S27–8.
3. Monnier L, Lapinski H, Colette C. Contributions of fasting and postprandial plasma glucose increments to the overall diurnal hyperglycemia of type 2 diabetic patients: variations with increasing levels of HbA(1c). Diabetes Care. (2003) 26:881–5. doi: 10.2337/diacare.26.3.881
4. Abdul-Ghani MA, Abdul-Ghani T, Ali N, Defronzo RA. One-hour plasma glucose concentration and the metabolic syndrome identify subjects at high risk for future type 2 diabetes. Diabetes Care. (2008) 31:1650–5. doi: 10.2337/dc08-0225
5. Gerstein HC, Santaguida P, Raina P, Morrison KM, Balion C, Hunt D, et al. Annual incidence and relative risk of diabetes in people with various categories of dysglycemia: a systematic overview and meta-analysis of prospective studies. Diabetes Res Clin Pract. (2007) 78:305–12. doi: 10.1016/j.diabres.2007.05.004
6. Kawasaki R, Wang JJ, Wong TY, Kayama T, Yamashita H, et al. Impaired glucose tolerance, but not impaired fasting glucose, is associated with retinopathy in Japanese population: the funagata study. Diabetes Obes Metab. (2008) 10:514–5. doi: 10.1111/j.1463-1326.2007.00824.x
7. Tominaga M, Eguchi H, Manaka H, Igarashi K, Kato T, Sekikawa A. Impaired glucose tolerance is a risk factor for cardiovascular disease, but not impaired fasting glucose. the funagata diabetes study. Diabetes Care. (1999) 22:920–4.
8. Leiter LA, Ceriello A, Davidson JA, Hanefeld M, Monnier L, Owens DR, et al. Postprandial glucose regulation: new data and new implications. Clin Ther. (2005) 27(Suppl. B):S42–56. doi: 10.1016/j.clinthera.2005.11.020
9. Glucose tolerance and mortality: comparison of WHO and American Diabetes Association diagnostic criteria. The DECODE study group. European Diabetes Epidemiology Group. Diabetes Epidemiology: Collaborative analysis Of Diagnostic criteria in Europe. Lancet. (1999) 354:617–21.
10. Cavalot F, Pagliarino A, Valle M, Di Martino L, Bonomo K, Massucco P, et al. Postprandial blood glucose predicts cardiovascular events and all-cause mortality in type 2 diabetes in a 14-year follow-up: lessons from the San Luigi Gonzaga Diabetes Study. Diabetes Care. (2011) 34:2237–43. doi: 10.2337/dc10-2414
11. Ceriello A, Hanefeld M, Leiter L, Monnier L, Moses A, Owens D, et al. Postprandial glucose regulation and diabetic complications. Arch Intern Med. (2004) 164:2090–5. doi: 10.1001/archinte.164.19.2090
12. Chiasson JL, Josse RG, Gomis R, Hanefeld M, Karasik A, Laakso M, et al. Acarbose treatment and the risk of cardiovascular disease and hypertension in patients with impaired glucose tolerance: the STOP-NIDDM trial. J Am Med Assoc. (2003) 290:486–94. doi: 10.1001/jama.290.4.486
13. Raz I, Ceriello A, Wilson PW, Battioui C, Su EW, Kerr L, et al. Post hoc subgroup analysis of the HEART2D trial demonstrates lower cardiovascular risk in older patients targeting postprandial versus fasting/premeal glycemia. Diabetes Care. (2011) 34:1511–3. doi: 10.2337/dc10-2375
14. Potti A, Mukherjee S, Petersen R, Dressman HK, Bild A, Koontz J, et al. Pioglitazone for diabetes prevention in impaired glucose tolerance. N Engl J Med. (2011) 364:1104–15. doi: 10.1056/NEJMoa1010949
15. Evert AB, Boucher JL, Cypress M, Dunbar SA, Franz MJ, Mayer-Davis EJ, et al. Nutrition therapy recommendations for the management of adults with diabetes. Diabetes Care. (2013) 36:3821–42. doi: 10.2337/dc13-2042
16. Davies MJ, D'Alessio DA, Fradkin J, Kernan WN, Mathieu C, Mingrone G, et al. Management of hyperglycemia in type 2 diabetes, 2018. a consensus report by the American Diabetes Association (ADA) and the European Association for the Study of Diabetes (EASD). Diabetes Care. (2018) 41:2669–701. doi: 10.2337/dci18-0033
17. Gerich J. Pathogenesis and management of postprandial hyperglycemia: role of incretin-based therapies. Int J Gen Med. (2013) 6:877–95. doi: 10.2147/IJGM.S51665
18. Tricò D, Baldi S, Tulipani A, Frascerra S, Macedo MP, Mari A, et al. Mechanisms through which a small protein and lipid preload improves glucose tolerance. Diabetologia. (2015) 58:2503–12. doi: 10.1007/s00125-015-3710-9
19. Tricò D, Filice E, Baldi S, Frascerra S, Mari A, Natali A. Sustained effects of a protein and lipid preload on glucose tolerance in type 2 diabetes patients. Diabetes Metab. (2016) 42:242–8. doi: 10.1016/j.diabet.2016.03.004
20. Tricò D, Natali A. Modulation of postprandial glycemic responses by noncarbohydrate nutrients provides novel approaches to the prevention and treatment of type 2 diabetes. Am J Clin Nutr. (2017) 106:701–2. doi: 10.3945/ajcn.117.157255
21. Cunningham KM, Read NW. The effect of incorporating fat into different components of a meal on gastric emptying and postprandial blood glucose and insulin responses. Br J Nutr. (1989) 61:285–90. doi: 10.1079/BJN19890116
22. Gentilcore D, Chaikomin R, Jones KL, Russo A, Feinle-Bisset C, Wishart JM, et al. Effects of fat on gastric emptying of and the glycemic, insulin, and incretin responses to a carbohydrate meal in type 2 diabetes. J Clin Endocrinol Metab. (2006) 91:2062–7. doi: 10.1210/jc.2005-2644
23. Ma J, Stevens JE, Cukier K, Maddox AF, Wishart JM, Jones KL, et al. Effects of a protein preload on gastric emptying, glycemia, and gut hormones after a carbohydrate meal in diet-controlled type 2 diabetes. Diabetes Care. (2009) 32:1600–2. doi: 10.2337/dc09-0723
24. Meng H, Matthan NR, Ausman LM, Lichtenstein AH. Effect of macronutrients and fiber on postprandial glycemic responses and meal glycemic index and glycemic load value determinations. Am J Clin Nutr. (2017) 105:842–53. doi: 10.3945/ajcn.116.144162
25. Wolever TM, Nuttall FQ, Lee R, Wong GS, Josse RG, Csima A, et al. Prediction of the relative blood glucose response of mixed meals using the white bread glycemic index. Diabetes Care. (1985) 8:418–28. doi: 10.2337/diacare.8.5.418
26. Akhavan T, Luhovyy BL, Brown PH, Cho CE, Anderson GH. Effect of premeal consumption of whey protein and its hydrolysate on food intake and postmeal glycemia and insulin responses in young adults. Am J Clin Nutr. (2010) 91:966–75. doi: 10.3945/ajcn.2009.28406
27. Bae JH, Kim LK, Min SH, Ahn CH, Cho YM. Postprandial glucose-lowering effect of premeal consumption of protein-enriched, dietary fiber-fortified bar in individuals with type 2 diabetes mellitus or normal glucose tolerance. J Diabetes Invest. (2018) 9:1110–8. doi: 10.1111/jdi.12831
28. Li CJ, Norstedt G, Hu ZG, Yu P, Li DQ, Li J, et al. Effects of a macro-nutrient preload on type 2 diabetic patients. Front Endocrinol. (2015) 6:139. doi: 10.3389/fendo.2015.00139
29. Li L, Xu J, Zhu W, Fan R, Bai Q, Huang C, et al. Effect of a macronutrient preload on blood glucose level and pregnancy outcome in gestational diabetes. J Clin Transl Endocrinol. (2016) 5:36–41. doi: 10.1016/j.jcte.2016.04.001
30. Wu T, Little TJ, Bound MJ, Borg M, Zhang X, Deacon CF, et al. A protein preload enhances the glucose-lowering efficacy of vildagliptin in Type 2 diabetes. Diabetes Care. (2016) 39:511–7. doi: 10.2337/dc15-2298
31. Kuwata H, Iwasaki M, Shimizu S, Minami K, Maeda H, Seino S, et al. Meal sequence and glucose excursion, gastric emptying and incretin secretion in type 2 diabetes: a randomised, controlled crossover, exploratory trial. Diabetologia. (2016) 59:453–61. doi: 10.1007/s00125-015-3841-z
32. Monnier L, Bonnet F, Colette C. Tailoring nutrient sequence and content to improve glucose tolerance: why and how to do it. Diabetes Metab. (2016) 42:211–4. doi: 10.1016/j.diabet.2016.04.003
33. Shukla AP, Iliescu RG, Thomas CE, Aronne LJ. Food order has a significant impact on postprandial glucose and insulin levels. Diabetes Care. (2015) 38:e98–9. doi: 10.2337/dc15-0429
34. Chen MJ, Jovanovic A, Taylor R. Utilizing the second-meal effect in type 2 diabetes: practical use of a soya-yogurt snack. Diabetes Care. (2010) 33:2552–4. doi: 10.2337/dc10-0552
35. Clifton PM, Galbraith C, Coles L. Effect of a low dose whey/guar preload on glycemic control in people with type 2 diabetes–a randomised controlled trial. Nutr J. (2014) 13:103. doi: 10.1186/1475-2891-13-103
36. Jakubowicz D, Froy O, Ahrén B, Boaz M, Landau Z, Bar-Dayan Y, et al. Incretin, insulinotropic and glucose-lowering effects of whey protein pre-load in type 2 diabetes: a randomised clinical trial. Diabetologia. (2014) 57:1807–11. doi: 10.1007/s00125-014-3305-x
37. Ma J, Jesudason DR, Stevens JE, Keogh JB, Jones KL, Clifton PM, et al. Sustained effects of a protein “preload” on glycaemia and gastric emptying over 4 weeks in patients with type 2 diabetes: a randomized clinical trial. Diabetes Res Clin Pract. (2015) 108:e31-4. doi: 10.1016/j.diabres.2015.02.019
38. Tricò D, Filice E, Trifirò S, Natali A. Manipulating the sequence of food ingestion improves glycemic control in type 2 diabetic patients under free-living conditions. Nutr Diab. (2016) 6:e226. doi: 10.1038/nutd.2016.33
39. Shukla AP, Andono J, Touhamy SH, Casper A, Iliescu RG, Mauer E, et al. Carbohydrate-last meal pattern lowers postprandial glucose and insulin excursions in type 2 diabetes. BMJ Open Diabetes Res Care. (2017) 5:e000440. doi: 10.1136/bmjdrc-2017-000440
40. Watson LE, Phillips LK, Wu T, Bound MJ, Checklin HL, Grivell J, et al. A whey/guar “preload” improves postprandial glycaemia and glycated haemoglobin levels in type 2 diabetes: A 12-week, single-blind, randomized, placebo-controlled trial. Diabetes Obes Metab. (2018). [Epub ahead of print]. doi: 10.1111/dom.13604
41. Watson LE, Phillips LK, Wu T, Bound MJ, Checklin H, Grivell J, et al. Differentiating the effects of whey protein and guar gum preloads on postprandial glycemia in type 2 diabetes. Clin Nutr. (2018). [Epub ahead of print]. doi: 10.1016/j.clnu.2018.12.014.
42. Crouch MA, Slater RT. Almond “Appetizer” effect on glucose tolerance test (GTT) results. J Am Board Fam Med. (2016) 29:759–66. doi: 10.3122/jabfm.2016.06.160128
43. Shukla AP, Dickison M, Coughlin N, Karan A, Mauer E, Truong W, et al. The impact of food order on postprandial glycaemic excursions in prediabetes. Diabetes Obes Metab. (2018) 21(2):377–81. doi: 10.1111/dom.13503
44. Akhavan T, Luhovyy BL, Panahi S, Kubant R, Brown PH, Anderson GH. Mechanism of action of pre-meal consumption of whey protein on glycemic control in young adults. J Nutr Biochem. (2014) 25:36–43. doi: 10.1016/j.jnutbio.2013.08.012
45. Sun L, Tan KWJ, Han CMS, Leow MK, Henry CJ. Impact of preloading either dairy or soy milk on postprandial glycemia, insulinemia and gastric emptying in healthy adults. Eur J Nutr. (2017) 56:77–87. doi: 10.1007/s00394-015-1059-y
46. Nishino K, Sakurai M, Takeshita Y, Takamura T. Consuming carbohydrates after meat or vegetables lowers postprandial excursions of glucose and insulin in nondiabetic subjects. J Nutr Sci Vitaminol. (2018) 64:316–20. doi: 10.3177/jnsv.64.316
47. Faber EM, van Kampen PM, Clement-de Boers A, Houdijk ECAM, van der Kaay DCM. The influence of food order on postprandial glucose levels in children with type 1 diabetes. Pediatr Diab. (2018) 19:809–15. doi: 10.1111/pedi.12640
48. Kuwata H, Okamoto S, Seino Y, Murotani K, Tatsuoka H, Usui R, et al. Relationship between deterioration of glycated hemoglobin-lowering effects in dipeptidyl peptidase-4 inhibitor monotherapy and dietary habits: Retrospective analysis of Japanese individuals with type 2 diabetes. J Diabetes Invest. (2018) 9:1153–8. doi: 10.1111/jdi.12779
49. Ullrich SS, Fitzgerald PC, Nkamba I, Steinert RE, Horowitz M, Feinle-Bisset C. Intragastric lysine lowers the circulating glucose and insulin responses to a mixed-nutrient drink without slowing gastric emptying in healthy adults. J Nutr. (2017) 147:1275–81. doi: 10.3945/jn.117.252213
50. Ullrich SS, Fitzgerald PC, Schober G, Steinert RE, Horowitz M, Feinle-Bisset C. Intragastric administration of leucine or isoleucine lowers the blood glucose response to a mixed-nutrient drink by different mechanisms in healthy, lean volunteers. Am J Clin Nutr. (2016) 104:1274–84. doi: 10.3945/ajcn.116.140640
51. Tey SL, Salleh N, Henry CJ, Forde CG. Effects of consuming preloads with different energy density and taste quality on energy intake and postprandial blood glucose. Nutrients. (2018) 10:E161. doi: 10.3390/nu10020161
52. Abou-Samra R, Keersmaekers L, Brienza D, Mukherjee R, Macé K. Effect of different protein sources on satiation and short-term satiety when consumed as a starter. Nutr J. (2011) 10:139. doi: 10.1186/1475-2891-10-139
53. Astbury NM, Stevenson EJ, Morris P, Taylor MA, Macdonald IA. Dose-response effect of a whey protein preload on within-day energy intake in lean subjects. Br J Nutr. (2010) 104:1858–67. doi: 10.1017/S000711451000293X
54. Giezenaar C, Trahair LG, Rigda R, Hutchison AT, Feinle-Bisset C, Luscombe-Marsh ND, et al. Lesser suppression of energy intake by orally ingested whey protein in healthy older men compared with young controls. Am J Physiol Regul Integr Comp Physiol. (2015) 309:R845–54. doi: 10.1152/ajpregu.00213.2015
55. Yabe D, Kuwata H, Fujiwara Y, Murotani K, Ito S, Asano H, et al. Effects of dietary instructions including meal-sequence for prediabetes subjects—comparison with conventional approach. Diabetes. (2018) 67 (Suppl 1). doi: 10.2337/db18-53-LB
56. Morgantini C, Trifirò S, Tricò D, Meriwether D, Baldi S, Mengozzi A., et al. A short-term increase in dietary cholesterol and fat intake affects high-density lipoprotein composition in healthy subjects. Nutr Metab Cardiovasc Dis. (2018) 28:575–81. doi: 10.1016/j.numecd.2018.03.005
57. Tricò D, Trifirò S, Mengozzi A, Morgantini C, Baldi S, Mari A, et al. Reducing cholesterol and fat intake improves glucose tolerance by enhancing beta cell function in nondiabetic subjects. J Clin Endocrinol Metab. (2018) 103:622–31. doi: 10.1210/jc.2017-02089
58. Groop PH, Aro A, Stenman S, Groop L. Long-term effects of guar gum in subjects with non-insulin-dependent diabetes mellitus. Am J Clin Nutr. (1993) 58:513–8. doi: 10.1093/ajcn/58.4.513
59. Sierra M, Garcia JJ, Fernández N, Diez MJ, Calle AP, Sahagún AM, et al. Effects of ispaghula husk and guar gum on postprandial glucose and insulin concentrations in healthy subjects. Eur J Clin Nutr. (2001) 55:235–43. doi: 10.1038/sj.ejcn.1601147
60. Imai S, Matsuda M, Hasegawa G, Fukui M, Obayashi H, Ozasa N, et al. A simple meal plan of 'eating vegetables before carbohydrate' was more effective for achieving glycemic control than an exchange-based meal plan in Japanese patients with type 2 diabetes. Asia Pac J Clin Nutr. (2011) 20:161–8.
61. Steinert RE, Raederstorff D, Wolever TM. Effect of consuming oat bran mixed in water before a meal on glycemic responses in healthy humans-a pilot study. Nutrients. (2016) 8:E524. doi: 10.3390/nu8090524
62. Chaikomin R, Rayner CK, Jones KL, Horowitz M. Upper gastrointestinal function and glycemic control in diabetes mellitus. World J Gastroenterol. (2006) 12:5611–21. doi: 10.3748/wjg.v12.i35.5611
63. Horowitz M, Edelbroek MA, Wishart JM, Straathof JW. Relationship between oral glucose tolerance and gastric emptying in normal healthy subjects. Diabetologia. (1993) 36:857–62. doi: 10.1007/BF00400362
64. Tricò D, Mengozzi A, Frascerra S, Scozzaro MT, Mari A, Natali A. Intestinal glucose absorption is a key determinant of 1-hour post-load plasma glucose levels in non-diabetic subjects. J Clin Endocrinol Metab. (2018). [Epub ahead of print]. doi: 10.1210/jc.2018-02166.
65. Jones KL, Horowitz M, Carney BI, Wishart JM, Guha S, Green L. Gastric emptying in early noninsulin-dependent diabetes mellitus. J Nucl Med. (1996) 37:1643–8.
66. Lin HC, Zhao XT, Wang L. Fat absorption is not complete by midgut but is dependent on load of fat. Am J Physiol. (1996) 271(1 Pt. 1):G62-7. doi: 10.1152/ajpgi.1996.271.1.G62
67. Collier G, O'Dea K. The effect of coingestion of fat on the glucose, insulin, and gastric inhibitory polypeptide responses to carbohydrate and protein. Am J Clin Nutr. (1983) 37:941–4. doi: 10.1093/ajcn/37.6.941
68. Houghton LA, Mangnall YF, Read NW. Effect of incorporating fat into a liquid test meal on the relation between intragastric distribution and gastric emptying in human volunteers. Gut. (1990) 31:1226–9. doi: 10.1136/gut.31.11.1226
69. Chaikomin R, Russo A, Rayner CK, Feinle-Bisset C, O'Donovan DG, Horowitz M, et al. Effects of lipase inhibition on gastric emptying and alcohol absorption in healthy subjects. Br J Nutr. (2006) 96:883–7. doi: 10.1017/BJN20061922
70. O'Donovan D, Horowitz M, Russo A, Feinle-Bisset C, Murolo N, Gentilcore D, et al. Effects of lipase inhibition on gastric emptying of, and on the glycaemic, insulin and cardiovascular responses to, a high-fat/carbohydrate meal in type 2 diabetes. Diabetologia. (2004) 47:2208–14. doi: 10.1007/s00125-004-1591-4
71. Bjørnshave A, Holst JJ, Hermansen K. Pre-meal effect of whey proteins on metabolic parameters in subjects with and without type 2 diabetes: a randomized, crossover trial. Nutrients. (2018) 10:E122. doi: 10.3390/nu10020122
72. Tricò D, Frascerra S, Baldi S, Mengozzi A, Nesti L, Mari A, et al. The insulinotropic effect of a high-protein nutrient preload is mediated by the increase of plasma amino acids in type 2 diabetes. Eur J Nutr. (2018). [Epub ahead of print]. doi: 10.1007/s00394-018-1778-y
73. Floyd JC, Fajans SS, Conn JW, Knopf RF, Rull J. Stimulation of insulin secretion by amino acids. J Clin Invest. (1966) 45:1487–502. doi: 10.1172/JCI105456
74. van Loon LJ, Saris WH, Verhagen H, Wagenmakers AJ. Plasma insulin responses after ingestion of different amino acid or protein mixtures with carbohydrate. Am J Clin Nutr. (2000) 72:96–105. doi: 10.1093/ajcn/72.1.96
75. Floyd JC, Fajans SS, Pek S, Thiffault CA, Knopf RF, Conn JW. Synergistic effect of certain amino acid pairs upon insulin secretion in man. Diabetes, 1970. 19:102–8. doi: 10.2337/diab.19.2.102
76. Newsholme P, Cruzat V, Arfuso F, Keane K. Nutrient regulation of insulin secretion and action. J Endocrinol. (2014) 221:R105–20. doi: 10.1530/JOE-13-0616
77. Nolan CJ, Madiraju MS, Delghingaro-Augusto V, Peyot ML, Prentki M. Fatty acid signaling in the beta-cell and insulin secretion. Diabetes. (2006) 55(Suppl. 2):S16–23. doi: 10.2337/db06-S003
78. Crespin SR, Greenough WB, Steinberg D. Stimulation of insulin secretion by long-chain free fatty acids. A direct pancreatic effect. J Clin Invest. (1973) 52:1979–84. doi: 10.1172/JCI107382
79. Rebelos E, Seghieri M, Natali A, Balkau B, Golay A, Piatti PM, et al. Influence of endogenous NEFA on beta cell function in humans. Diabetologia. (2015) 58:2344–51. doi: 10.1007/s00125-015-3685-6
80. Natali A, Baldi S, Bonnet F, Petrie J, Trifirò S, Tricò D, et al. Plasma HDL-cholesterol and triglycerides, but not LDL-cholesterol, are associated with insulin secretion in non-diabetic subjects. Metabolism. (2017) 69:33–42. doi: 10.1016/j.metabol.2017.01.001
81. Tricò D, Natali A, Mari A, Ferrannini E, Santoro N, Caprio S. Triglyceride-rich very low-density lipoproteins (VLDL) are independently associated with insulin secretion in a multiethnic cohort of adolescents. Diabetes Obes Metab. (2018) 20:2905–10. doi: 10.1111/dom.13467
82. Seghieri M, Tricò D, Natali A. The impact of triglycerides on glucose tolerance: Lipotoxicity revisited. Diabetes Metab. (2017) 43:314–22. doi: 10.1016/j.diabet.2017.04.010
83. Alsalim W, Tura A, Pacini G, Omar B, Bizzotto R, Mari A, et al. Mixed meal ingestion diminishes glucose excursion in comparison with glucose ingestion via several adaptive mechanisms in people with and without type 2 diabetes. Diabetes Obes Metab. (2016) 18:24–33. doi: 10.1111/dom.12570
84. Lan-Pidhainy X, Wolever TM. The hypoglycemic effect of fat and protein is not attenuated by insulin resistance. Am J Clin Nutr. (2010) 91:98–105. doi: 10.3945/ajcn.2009.28125
85. Muscelli E, Frascerra S, Casolaro A, Baldi S, Mari A, Gall W, et al. The amino acid response to a mixed meal in patients with type 2 diabetes: effect of sitagliptin treatment. Diabetes Obes Metab, 2014. 16:1140–7. doi: 10.1111/dom.12350
86. Holst JJ. The physiology of glucagon-like peptide 1. Physiol Rev. (2007) 87:1409–39. doi: 10.1152/physrev.00034.2006
87. Seino Y, Fukushima M, Yabe D. GIP and GLP-1, the two incretin hormones: Similarities and differences. J Diabetes Invest. (2010) 1:8–23. doi: 10.1111/j.2040-1124.2010.00022.x
88. Karamanlis A, Chaikomin R, Doran S, Bellon M, Bartholomeusz FD, Wishart JM, et al. Effects of protein on glycemic and incretin responses and gastric emptying after oral glucose in healthy subjects. Am J Clin Nutr. (2007) 86:1364–8. doi: 10.1093/ajcn/86.5.1364
89. Pupovac J, Anderson GH. Dietary peptides induce satiety via cholecystokinin-A and peripheral opioid receptors in rats. J Nutr. (2002) 132:2775–80. doi: 10.1093/jn/132.9.2775
90. Shuster LT, Go VL, Rizza RA, O'Brien PC, Service FJ. Incretin effect due to increased secretion and decreased clearance of insulin in normal humans. Diabetes. (1988) 37:200–3. doi: 10.2337/diab.37.2.200
91. Rudovich NN, Rochlitz HJ, Pfeiffer AF. Reduced hepatic insulin extraction in response to gastric inhibitory polypeptide compensates for reduced insulin secretion in normal-weight and normal glucose tolerant first-degree relatives of type 2 diabetic patients. Diabetes. (2004) 53:2359–65. doi: 10.2337/diabetes.53.9.2359
92. Campbell JE, Drucker DJ. Pharmacology, physiology, and mechanisms of incretin hormone action. Cell Metab. (2013) 17:819–37. doi: 10.1016/j.cmet.2013.04.008
93. Liddle RA. Regulation of cholecystokinin secretion in humans. J Gastroenterol. (2000) 35:181–7. doi: 10.1007/s005350050328
94. Vincent RP, le Roux CW. The satiety hormone peptide YY as a regulator of appetite. J Clin Pathol. (2008) 61:548–52. doi: 10.1136/jcp.2007.048488
95. Yamagishi T, Debas HT. Cholecystokinin inhibits gastric emptying by acting on both proximal stomach and pylorus. Am J Physiol. (1978) 234:E375–8. doi: 10.1152/ajpendo.1978.234.4.E375
96. Rayner CK, Park HS, Doran SM, Chapman IM, Horowitz M. Effects of cholecystokinin on appetite and pyloric motility during physiological hyperglycemia. Am J Physiol Gastrointest Liver Physiol. (2000) 278:G98–G104. doi: 10.1152/ajpgi.2000.278.1.G98
97. Borovicka J, Kreiss C, Asal K, Remy B, Mettraux C, Wells A, et al. Role of cholecystokinin as a regulator of solid and liquid gastric emptying in humans. Am J Physiol. (1996) 271(3 Pt. 1):G448–53. doi: 10.1152/ajpgi.1996.271.3.G448
98. Allen JM, Fitzpatrick ML, Yeats JC, Darcy K, Adrian TE, Bloom SR. Effects of peptide YY and neuropeptide Y on gastric emptying in man. Digestion. (1984) 30:255–62. doi: 10.1159/000199117
99. Lo CM, Obici S, Dong HH, Haas M, Lou D, Kim DH, et al. Impaired insulin secretion and enhanced insulin sensitivity in cholecystokinin-deficient mice. Diabetes. (2011) 60:2000–7. doi: 10.2337/db10-0789
100. Rehfeld JF. Cholecystokinin-from local gut hormone to ubiquitous messenge. Front Endocrinol. (2017) 8:47. doi: 10.3389/fendo.2017.00047
101. Batterham RL, Cowley MA, Small CJ, Herzog H, Cohen MA, Dakin CL, et al. Gut hormone PYY(3-36) physiologically inhibits food intake. Nature. (2002) 418:650–4. doi: 10.1038/nature00887
Keywords: macronutrient preloads, food order, gastric emptying, glucose tolerance, insulin secretion, postprandial glycemia, medical nutrition therapy, type 2 diabetes
Citation: Nesti L, Mengozzi A and Tricò D (2019) Impact of Nutrient Type and Sequence on Glucose Tolerance: Physiological Insights and Therapeutic Implications. Front. Endocrinol. 10:144. doi: 10.3389/fendo.2019.00144
Received: 07 December 2018; Accepted: 18 February 2019;
Published: 08 March 2019.
Edited by:
Tongzhi Wu, University of Adelaide, AustraliaReviewed by:
Tanya J. Little, University of Adelaide, AustraliaDaisuke Yabe, Gifu University, Japan
Copyright © 2019 Nesti, Mengozzi and Tricò. This is an open-access article distributed under the terms of the Creative Commons Attribution License (CC BY). The use, distribution or reproduction in other forums is permitted, provided the original author(s) and the copyright owner(s) are credited and that the original publication in this journal is cited, in accordance with accepted academic practice. No use, distribution or reproduction is permitted which does not comply with these terms.
*Correspondence: Domenico Tricò, domenico.trico@for.unipi.it
†These authors have contributed equally to this work