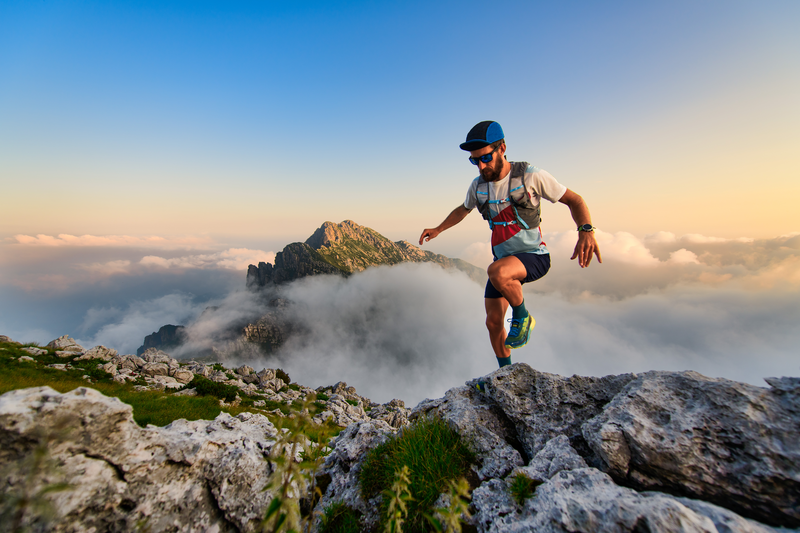
95% of researchers rate our articles as excellent or good
Learn more about the work of our research integrity team to safeguard the quality of each article we publish.
Find out more
PERSPECTIVE article
Front. Endocrinol. , 05 February 2019
Sec. Neuroendocrine Science
Volume 10 - 2019 | https://doi.org/10.3389/fendo.2019.00037
Identification of the biological features of autism is essential for designing an efficient treatment and for prevention of the disorder. Though the subject of extensive research, the neurophysiological features of autism remain unclear. One of the proposed biological causes of autism is malfunction of the pineal gland and deficiency of its principal hormone, melatonin. The main function of melatonin is to link and synchronize the body's homeostasis processes to the circadian and seasonal rhythms, and to regulate the sleep-wake cycle. Therefore, pineal dysfunction has been implicated based on the common observation of low melatonin levels and sleep disorders associated with autism. In this perspective, we highlight several recent findings that support the hypothesis of pineal gland/melatonin involvement in autism. Another common symptom of autism is abnormal neuroplasticity, such as cortical overgrowth and dendritic spine dysgenesis. Here, we synthesize recent information and speculate on the possibility that this abnormal neuroplasticity is caused by hyperactivity of endogenous N,N-dimethyltryptamine (DMT). The pineal gland was proposed as the source of DMT in the brain and therefore, our assumption is that besides melatonin deficiency, pineal dysfunction might also play a part in the development of autism through abnormal metabolism of DMT. We hope that this manuscript will encourage future research of the DMT hypothesis and reexamination of several observations that were previously attributed to other factors, to see if they could be related to pineal gland/melatonin malfunction. Such research could contribute to the development of autism treatment by exogenous melatonin and monitored light exposure.
Decades of intensive research have revealed a wide-range of physiological features (1, 2), genetic (3), and environmental effectors (4), which have been suggested to be the cause, trigger, or to enhance the risk of autism spectrum disorder (ASD). Yet, none of the hundreds of gene mutations or environmental conditions have been found to be consistently present across the wide spectrum of the heterogenic autistic population. One of the proposed biological causes of autism is malfunction of the pineal gland (5, 6).
The pineal gland is a minute neuroendocrine gland, located deep in the center of the brain, projecting from the posterior wall of the third ventricle. Its position on top of the third ventricle allows direct secretion to the cerebrospinal fluid (CSF), in addition to the main route of hormone secretion to the blood. Interestingly, an average excess of CSF volume in the subarachnoid space was detected in autistic infants (7). Increase in CSF volume could have an influence on the concentration and effectiveness of the components it delivers to the brain. Melatonin (N-acetyl-5-methoxytryptamine) is considered as the principal hormone produced by the pineal gland and its well-documented function is to link and synchronize the body's homeostasis processes to the circadian and seasonal rhythms, and regulate the sleep-wake cycle. Melatonin synthesis and secretion are mainly controlled by photosensory information that arrives from the suprachiasmatic nuclei (SCN). The SCN control of the pineal gland creates a strong circadian rhythm with high levels of melatonin secretion in the dark at night, and negligible levels during the day.
Melatonin functions through several receptors and mechanisms [for review, see, (8, 9)]. In short, the most well-characterized pathways, which are assumed to be the main targets of melatonin, are the membrane specific G protein-coupled melatonin receptors MT1 and MT2. These two principal receptors are expressed throughout the brain (9, 10). In addition, due to its lipophilic trait, melatonin is permeable to biological membranes and binds to intracellular proteins such as calmodulin, and directly and indirectly effects nuclear receptors like the receptor family RZR/ROR, which act as transcriptional activators and induce immunomodulatory effect. Moreover, recent studies proposed a putative binding site to quinone reductase II (MT3), a detoxification enzyme with metabolic and antioxidative effects (11).
Melatonin takes effect at early stages of embryo-fetal developmental. Actually, even before embryo development, melatonin affects oocyte quality, and possibly induces epigenetic modulation inside the oocyte (12, 13). During pregnancy the embryo depends on maternal melatonin since the pineal gland is activated only after birth. Maternal concentrations of melatonin increase after 24 weeks of gestation, with significantly higher levels after 32 weeks (14, 15). Melatonin receptors are widespread in the embryo and fetus from early stages, both in the nervous system, and in peripheral organs. Melatonin influences the internal rhythms of the offspring and has a direct developmental and neuroprotective effect on the fetal brain (13, 16). Besides the maternal source, melatonin is synthesized by the placenta which also express MT1 and MT2 receptors that mediate direct effects on placental maintenance (17). Vanecek (18) showed that in rat, the melatonin receptors in the pituitary gland decrease drastically postnatal, but maintain the same quantity in the hypothalamus. This finding implies different roles for melatonin during pregnancy and postnatal, and displays the dynamic distribution of melatonin receptors; as been found in the placenta during pregnancy (17) and for day/night expression in the brain (19). From the above it is clear that impairment of the melatonin pathway during pregnancy might increase risk of neurodevelopmental disorders, such as ASD (20).
The link between autism and pineal gland/melatonin malfunction was first recognized after observations revealing low concentrations of melatonin in individuals with autism (21). About 65% of ASD patients have less than half the average values of melatonin (22). Moreover, sleep disorder is one of the most common symptoms in ASD patients, with prevalence of 50–80%, compared to 9–50% in normal children (22, 23). Also, exogenous treatment with melatonin was found to be very beneficial in reducing the time until onset of sleep, and increasing sleep duration (6).
Genetically, autism-associated mutations have been found in genes encoding the key enzymes of melatonin synthesis, Aralkylamine N-acetyltransferase (AANAT), and Acetylserotonin O-methyltransferase (ASMT) (24, 25), as well as a reduced expression of the gene encoding AANAT in ASD (26). Ggene abnormalities have also been found in gene encoding the melatonin receptors MT1 and MT2. Though, a systematic review by Rossignol and Frye (6) concluded that the contribution of these genetic variations to melatonin deficiency are presented in only a small percentage of individuals with ASD.
Here, we highlight recent information that we suggest further supports the pineal gland/melatonin hypothesis. In addition to the link between melatonin and ASD, we propose new insights in light of the recent work by Calvin Ly et al. (27), speculating on the possibility that abnormal metabolism of endogenous N,N-dimethyltryptamine (DMT), possibly from the pineal gland (28), might cause the aberrant neuroplasticity and neural connectivity abnormalities observed in autistic patients (29, 30).
Braam et al. (31 included a Supplementary Table in their recent work that summarizes several observations that could be associated with melatonin deficiency in the autistic child or mother. Here we take some of their notes and expand the concept with our insights.
In addition to the chronobiological function, melatonin has two other putative roles. First, melatonin is considered to be one of the most efficient and vital endogenous antioxidants found in the brain and body tissues (32). Therefore, melatonin deficiency might cause neuronal damage induced by oxidative stress (33). Secondly, there is accumulating data indicating that melatonin is an immunomodulatory compound with immunostimulant or anti-inflammatory properties (34). Immune system abnormalities have been reported in children with autism (35) which might demonstrate an addition link between the pineal gland and ASD.
During embryonic and infant development, the pineal is the first gland to develop in the fetus, reaching its mature size at 2 years (36). However, melatonin production is not significant during the first 3 months of life. From the fourth month, the levels of nocturnal melatonin secretions steadily increase–reaching a peak at age three to five. This time scale has some degree of correlation with the age of autism diagnosis–after the first year and mainly between the ages of two to four, when the symptoms clearly present. Tauman et al. (37) reported an association between low melatonin excretion during the first prenatal weeks to delayed psychomotor development that first presented at 6 months of age; which correlates with the earliest time that ASD related symptoms might be evident (30). Aside from the above, reexamination of other observations from ASD could reveal novel correlations. First, melatonin synthesis is controlled by light absorbed by intrinsically photosensitive retinal ganglion cells (ipRGCs); these are specific light-sensitive cells that are separated from the principal visual photoreceptors. Malfunction in the ipRGCs area of the retina might provide an explanation for the association found between congenital visual impairment and the higher risk of developing autism (38, 39). This risk factor may the result of abnormal melatonin rhythms (40) and not due to melatonin deficiency since the average melatonin level is supposedly higher in blind subjects. Additionally, adults with ASD appear to be at significant risk for developing type 2 diabetes, coronary heart disease, and cancer (41, 42). These diseases and autism may share risk factors that are not directly linked to melatonin, such as obesity and genetic mutations. However, it has been suggested that melatonin is a protective factor against cancer (43) and coronary heart disease (44). There is also general agreement that melatonin metabolism has a link to type 2 diabetes (45), although the mechanism is still unclear (46).
Lastly, a set of recent studies have revealed that the prevalence rates of ASD are lowest in countries near the equator and the rate increases with latitude (47). In addition, there is accumulated data which indicates the enhanced risk of neurodevelopmental disabilities including ASD in children of immigrants to Europe and North America, mainly from Sub-Saharan Africa, East Africa, and Southeast Asia (48). Moreover, recent studies have revealed a link between month of conception and prevalence of ASD, with the highest rate among children conceived in the winter (49). Aside from maternal stress in the case of refugee immigrants, and seasonal pathogens, these observations were mainly attributed to maternal vitamin D deficiency (50). For example, autistic offspring of dark-skinned mothers who moved to higher latitudes and/or urban areas, resulting in lower level of maternal vitamin D due to decrease in sunlight exposure. Like vitamin D, melatonin production is highly related to sunlight exposure and varies seasonally. Therefore, we would like to propose that low or unbalanced levels of melatonin in the mother (20) or/and pineal/melatonin deficiency in the child, could provide an alternative or additional biological cause of vitamin D hypostasis (50, 51). Reexamination of the above observations focusing on the melatonin level may reveal new insights. For example, it may be that the seasonal conception effect (49) is due to the maternal melatonin level during gestation; not necessarily during the first trimester (15, 31), but rather in third trimester when maternal melatonin reaches its peak [summer, if conception took place in the winter (13)]. On the other hand, it could be that the effect is related to the date of birth. If this is the case, it can be assumed that the risk for developing ASD increases if a child has pineal dysfunction in addition to naturally low melatonin production over the summer months during a critical development period in the first years of life (52, 53). Additionally, breastfeeding was also examined, and proposed as a putative protective factor for ASD (54). Breastfeeding is the main source of melatonin during the first months of life (55), and therefore the seasonal effect on the infant might be the determining factor i.e., daylight hours. Though vitamin D and melatonin production are influenced by sunlight there are two significant parameters to be considered for melatonin alone. First, melatonin production significantly fluctuates both over the course of a day and seasonally (56). Second, melatonin synthesis is relatively sensitive to even dim afternoon light and is strongly influenced by artificial lighting, mainly in the blue light range. Russel et al. (57) proposed that night exposure to artificial light might be a risk factor for autism development. High levels of artificial lighting are common in the modern urban environment. Therefore, this factor could be related to the migration effect (48) when the frequency and intensity of night exposure to artificial lighting and hours of daylight change dramatically (56, 58). It is reasonable to assume that such a change will be most dramatic in populations where the biological clock and pineal function are inherently adjusted to very different illumination regimes. This could be comparable to studies on beta cell function in immigrants to Israel from Yemen; their insulin production was found to be insufficient for the new diet and lifestyle, causing high risk for type 2 diabetes (59). Moreover, the effect seems to peak when migration occurred around pregnancy and declined over time after immigration (60), which could indicate physiological adaptation of melatonin metabolism. For further possible effects of melatonin dysfunction on cognitive and behavioral aspects of ASD see the comprehensive review by Tordjman et al. (53).
Lastly, recent studies on Alzheimer's disease and general learning and memory research suggest that melatonin may also play an important role in synaptic stability, synaptic plasticity, and hippocampal neurogenesis through MT1 and MT2 receptors although, the mechanism is rather obscure (9, 61). As discussed in the next section, a defect in this function could also be linked to ASD symptoms.
It has previously been suggested that abnormal neural plasticity such as cortical overgrowth (30) and dendritic spine dysgenesis (29) is a common neurological symptom in ASD. Abnormal activation of the mammalian target of rapamycin)mTOR) has been proposed as the cause for these abnormal developments in ASD (62, 63). In addition, it was suggested that disruption of brain-derived neurotrophic factor (BDNF) activity through tyrosine kinase B (TrkB) signaling might play a role in autism initiation and propagation (64). Interestingly, disruption of melatonin synthesis in ASD was associated with an increase of the melatonin precursors, serotonin and N-acetylserotonin (NAS). NAS was found to be an agonist of the TrkB receptor and therefore in conjunction with serotonin might contribute to the abnormal neural plasticity in ASD [Figure 1; (25, 65)].
Figure 1. Schematic diagram illustrating how pineal gland dysfunction and/or MAO-A malfunction metabolism might result in hyperactivity of DMT, causing abnormal neuronal development and some of the behavioral/Physiological symptomns associated with ASD. On the upper right side is an overview of the way in which melatonin deficiency may also lead to abnormal neuronal plasticity through the melatonin precursor, NAS. In addition, relevant autism studies are specified. mTOR, mammalian target of rapamycin; BDNF, brain-derived neurotrophic factor; TrKB, tyrosine kinase B; INMT, Indolethylamine-N-mathyltransfersae; MAO-A, monoamine oxidase A; DMT, N,N-dimethyltryptamine; NAS, N-acetylserotonin; AANAT, Aralkylamine N-acetyltransferase; ASMT, Acetylserotonin O-methyltransferase; SERT, serotonin uptake transporter. *(?) The interaction between 5-HT2A receptors and BDNF is still not entirely understood.
A recent study by Ly et al. (27) showed that treatment of Drosophila and rats with DMT increases neuritogenesis, spinogenesis, and synaptogenesis through 5-HT2A receptor (5-HT2AR), TrkB, and mTOR signaling. The work of Ly et al. (27) is added to recent accumulated data on the effect of psychedelics (5-HT2AR agonists) like LSD and DMT structure analogs (66) on neuroplasticity, through cellular mechanisms such as the BDNF pathway, and even long term influence on gene expression in the brain (67, 68). The significant finding within the work of Ly et al. is that they show these effects for DMT–which was found to be endogenous in the brain. Based on the above, we propose that ASD may be affected by abnormal metabolism of endogenous DMT (Figure 1).
DMT is widely found in plants and animals, and although there have been indications for endogenous DMT in mammals since the 60 s, only in the last decade was it indisputably proven that DMT is present in humans (69). The source of endogenous DMT is unknown. One of the molecules being traced in order to identify the source of DMT is Indolethylamine-N-methyltransferase (INMT). INMT is thought to be the pivotal enzyme in the DMT production from the biogenic amine tryptamine, and therefore can indicate the sources of DMT. However, INMT methylates other substrates, and therefore its presence can only imply DMT production. INMT has been detected in many tissues in the body, primarily in the lungs, thyroid, and adrenal gland (70). Due to either popular trends or based on pure scientific reason, the pineal gland is the most studied area in the brain regarding DMT. The pineal gland was first proposed as the source of DMT in the brain since INMT had been found in human pineal, though its existence in other parts of the brain has been suggested as well (70–72). In 2013, Barker et al. (28, 73) provided the first strong indication for the existence of three endogenous forms of DMTs (DMT, 5-hydroxy-DMT and 5-methoxy-DMT) in the rat pineal. Still, the physiological function of DMT is unclear. Exogenous DMT has a clear and robust psychedelic effect, mainly through the activation of 5-HT2AR, and therefore it has been proposed that endogenous DMT might have a cognitive function. Yet, the role of DMT as an endogenous “psychedelic” remains controversial (74, 75). The main reason for the uncertainty is that only minute traces were detected (μg/kg), which were about 3 orders below the dose found to have psychedelic effects when delivered exogenously (mg/kg) (75, 76). It has even been considered a negligible non-functional concentration of byproduct from the tryptophan derivative indoleamines metabolism. However, there are reports (77) that link tumors in the pineal gland and peduncular hallucinosis (78) and florid psychotic symptoms (79). In connection to ASD, some autistic behavior such as synesthesia (80), sensory sensitivity, hyper attention to visual details (81), attraction to geometric shape (82), hallucinations (83), and extended pupils (84, 85) [note that this suppresses melatonin synthesis (86)] might be explained as a mild DMT psychedelic effect. Though, some of these symptoms such as synesthesia, might be the result of hyperconnectivity due to abnormal maturity of the brain (87), which as shown by Ly et al. (27) could also be a possible effect of DMT. Importantly, the DMT analog, 5-HO-DMT (Bufotenine) which is also a hallucinogen derived from tryptophan has been found in elevated urine levels in ASD patients (88).
From the above, it is clear that any attempt to propose a biophysiological effect for DMT should first address the concentration issue. Barker (76) pointed out several critical factors that were not taken into account in the previous works, and might explain the tiny concentration of non-physiological endogenous DMT found in the brain. Three of these factors are relevant to pineal production of DMT, which as in the case of melatonin, might vary largely over the course of the day, seasonally and according to age (89). First, none of the works checked for circadian changes in DMT level. Second, the age of the examined subjects was not taken into consideration. Third, for obvious reasons, most data were collected from blood and urine samples and only a few tested DMT levels in the CSF, where the pineal gland directly deposits the compounds produced (in addition to the blood system). DMT concentration in the brain might also alter significantly according to the mental state of the subject, for example varying under stress conditions (89). Furthermore, although not directly related to the pineal gland, and as yet unproven, it was suggested that DMT might be accumulated in vesicles in the brain, building a reservoir–ready to release in effective concentrations (70). Another speculation is that DMT releases in a local and specific manner which allows it to reach an effective concentration. The small amount of detected concentration of DMT could be partly attributed to the efficient enzyme monoamine oxidase A (MAO-A), which readily catalyzes DMT (as well as other monoamines, such as serotonin, dopamine, and norepinephrine). However, there is evidence of decreased activity of MAO-A in the brain of children with ASD (90). Similar to other mutations associated with ASD, the MAO-A is an x-linked recessive gene and therefore correlates with the 4:1 male-to-female ratio. MAO-A dysfunction could also be caused by endogenous MAO-A inhibitors such as pinoline that is thought to be produced by the pineal gland (91). Moreover, Kałuzna-Czaplinska et al. (92) argued that their preliminary results indicate the existence of endogenous MAO-A inhibitor in the urine of ASD children, and that this abnormality is possibly related to pineal gland malfunction. Moreover, Davis et al. found that polymorphism of the MAO-A gene is associated with brain structure volumes in children with autism (93).
As for the work of Ly et al. (27) they tested concentrations of DMT that produced a psychedelic effect (10 mg/kg). However, they did not check lower concentrations, and therefore their finding could not rule out the possibility that neuroplasticity could be affected, over time, by the trace concentration. Interestingly, they did find the same effect on neural activity with 10 mg/kg (hallucinogenic dose) and 1 mg/kg (sub hallucinogenic dose). Alongside their finding regarding 5-HT2AR, it has been proposed that DMT and/or closely related compounds such as bufotenine, have an effect and possible physiological function in neural plasticity and immune system mechanisms through the activation of other receptors such as the sigma-1 (94) or other serotonin receptors (76). Yet, most of the known DMT receptors including the sigma-1 receptor, have lower affinity compared to 5-HT2AR (95).
Finally, It has been suggested that in ASD there is a decrease in serotonin binding to 5-HT2AR while hypersensitivity to agonist drugs (96), and hypo or hyper-function of the serotonin uptake transporter (SERT). DMT has been shown to be taken up into neuronal cells via SERT (76). Therefore, it is possible that hypo-function of SERT and/or hypersensitivity of 5-HT2AR could result in an elevated effect of DMT.
Drawing from all these recent studies, we postulate that pineal gland dysfunction and/or MAO-A malfunction metabolism, results in hyperactivity of DMT causing the abnormal neuronal development associated with ASD, possibly through the mechanism proposed by Ly et al. [Figure 1; (27)].
In conclusion, a wide range of recent studies provide new support for the hypothesis that pineal gland dysfunction and abnormal metabolism of its related products might be a significant, still unrevealed cause of ASD. Elevated levels of the endogenous psychotomimetic molecule bufotenine has been reported in urine of ASD patients (88), but as far as we know, no assessment of DMT in ASD patients has been performed. We hope that this perspective will encourage future research to test the hypothesis that DMT metabolism is associated with ASD development, and reexamination of the results attributed to vitamin D dysfunction; only this time, based on the pineal gland/melatonin malfunction premise. The results of such studies have the potential to lead to an effective and relatively simple treatment and maybe prevention procedure for ASD, using exogenous melatonin (97, 98) even for infants (99). In addition, treatment could include monitoring of light exposure in infants, toddlers, pregnant women, breastfeeding mothers, and providing pumped breastmilk to the infants according to the time it was expressed (day or night) in order to assure the proper levels of melatonin.
TS and NN conceived and drafted the manuscript.
The authors declare that the research was conducted in the absence of any commercial or financial relationships that could be construed as a potential conflict of interest.
We thank Roxanne Halper for editorial assistance and Eyal Kapulnik for helpful discussion.
1. Samsam M, Ahangari R, Naser SA. Pathophysiology of autism spectrum disorders: revisiting gastrointestinal involvement and immune imbalance. World J Gastroenterol. (2014) 20:9942–51. doi: 10.3748/wjg.v20.i29.9942
2. Yenkoyan K, Grigoryan A, Fereshetyan K, Yepremyan D. Advances in understanding the pathophysiology of autism spectrum disorders. Behav Brain Res. (2017) 331:92–101. doi: 10.1016/j.bbr.2017.04.038
3. Ramaswami G, Geschwind DH. Genetics of autism spectrum disorder. Handb Clin Neurol. (2018) 147:321–9. doi: 10.1016/B978-0-444-63233-3.00021-X
4. Modabbernia A, Velthorst E, Reichenberg A. Environmental risk factors for autism: an evidence-based review of systematic reviews and meta-analyses. Mol Autism (2017) 8:13. doi: 10.1186/s13229-017-0121-4
5. Ratajczak HV. Theoretical aspects of autism: biomarkers–a review. J Immunotoxicol. (2011) 8:80–94. doi: 10.3109/1547691X.2010.538749
6. Rossignol DA, Frye RE. Melatonin in autism spectrum disorders: a systematic review and meta-analysis. Dev Med Child Neurol. (2011) 53:783–92. doi: 10.1111/j.1469-8749.2011.03980.x
7. Shen MD, Kim SH, McKinstry RC, Gu H, Hazlett HC, Nordahl CW, et al. Increased extra-axial cerebrospinal fluid in high-risk infants who later develop autism. Biol Psychiatry (2017) 82, 186–193. doi: 10.1016/j.biopsych.2017.02.1095
8. Emet M, Ozcan H, Ozel L, Yayla M, Halici Z, Hacimuftuoglu A. A review of melatonin, its receptors and drugs. Eurasian J Med. (2016) 48:135–41. doi: 10.5152/eurasianjmed.2015.0267
9. Ng KY, Leong MK, Liang H, Paxinos G. Melatonin receptors: distribution in mammalian brain and their respective putative functions. Brain Struct Funct. (2017) 222:2921–39. doi: 10.1007/s00429-017-1439-6
10. Slominski RM, Reiter RJ, Schlabritz-Loutsevitch N, Ostrom RS, Slominski AT. Melatonin membrane receptors in peripheral tissues: distribution and functions. Mol Cell Endocrinol. (2012) 351:152–66. doi: 10.1016/j.mce.2012.01.004
11. Boutin JA, Ferry G. Is there sufficient evidence that the melatonin binding site. J Pharmacol Exp Ther. (2019) 368:59–65. doi: 10.1124/jpet.118.253260
12. Irmak MK, Topal T, Oter S. Melatonin seems to be a mediator that transfers the environmental stimuli to oocytes for inheritance of adaptive changes through epigenetic inheritance system. Med Hypotheses (2005) 64:1138–43. doi: 10.1016/j.mehy.2004.12.014
13. Voiculescu SE, Zygouropoulos N, Zahiu CD, Zagrean AM. Role of melatonin in embryo fetal development. J Med Life (2014) 7:488–92.
14. Nakamura Y, Tamura H, Kashida S, Takayama H, Yamagata Y, Karube A, et al. Changes of serum melatonin level and its relationship to feto-placental unit during pregnancy. J Pineal Res. (2001) 30:29–33. doi: 10.1034/j.1600-079X.2001.300104.x
15. Sagrillo-Fagundes L, Assunção Salustiano EM, Yen PW, Soliman A, Vaillancourt C. Melatonin in pregnancy: effects on brain development and CNS programming disorders. Curr Pharm Des. (2016) 22:978–86. doi: 10.2174/1381612822666151214104624
16. Carlomagno G, Minini M, Tilotta M, Unfer V. From implantation to birth: insight into molecular melatonin functions. Int J Mol Sci. (2018) 19:E2802. doi: 10.3390/ijms19092802
17. Soliman A, Lacasse AA, Lanoix D, Sagrillo-Fagundes L, Boulard V, Vaillancourt C. Placental melatonin system is present throughout pregnancy and regulates villous trophoblast differentiation. J Pineal Res. (2015) 59:38–46. doi: 10.1111/jpi.12236
18. Vanecek J. The melatonin receptors in rat ontogenesis. Neuroendocrinology (1988) 48:201–3. doi: 10.1159/000125008
19. Pinato L, Ramos D, Hataka A, Rossignoli PS, Granado MD, Mazzetto MC, et al. Day/night expression of MT. J Chem Neuroanat. (2017) 81:10–7. doi: 10.1016/j.jchemneu.2017.01.005
20. Jin Y, Choi J, Won J, Hong Y. The relationship between autism spectrum disorder and melatonin during fetal development. Molecules (2018) 23:E198. doi: 10.3390/molecules23010198
21. Axt A. Autism viewed as a consequence of pineal gland malfunction. Farmakoterapia w Psychiatrii I Neurol. (1998) 98:112–34.
22. Geoffray MM, Nicolas A, Speranza M, Georgieff N. Are circadian rhythms new pathways to understand Autism Spectrum Disorder? J Physiol Paris (2016) 110(4 Pt B):434–8. doi: 10.1016/j.jphysparis.2017.06.002
23. Cohen S, Conduit R, Lockley SW, Rajaratnam SM, Cornish KM. The relationship between sleep and behavior in autism spectrum disorder (ASD): a review. J Neurodev Disord. (2014) 6:44. doi: 10.1186/1866-1955-6-44
24. Melke J, Goubran Botros H, Chaste P, Betancur C, Nygren G, Anckarsäter H, et al. Abnormal melatonin synthesis in autism spectrum disorders. Mol Psychiatry (2008) 13:90–8. doi: 10.1038/sj.mp.4002016
25. Pagan C, Goubran-Botros H, Delorme R, Benabou M, Lemière N, Murray K, et al. Disruption of melatonin synthesis is associated with impaired 14-3-3 and miR-451 levels in patients with autism spectrum disorders. Sci Rep. (2017) 7:2096. doi: 10.1038/s41598-017-02152-x
26. Hu VW, Sarachana T, Kim KS, Nguyen A, Kulkarni S, Steinberg ME, et al. Gene expression profiling differentiates autism case-controls and phenotypic variants of autism spectrum disorders: evidence for circadian rhythm dysfunction in severe autism. Autism Res. (2009) 2:78–97. doi: 10.1002/aur.73
27. Ly C, Greb AC, Cameron LP, Wong JM, Barragan EV, Wilson PC, et al. Psychedelics promote structural and functional neural plasticity. Cell Rep. (2018) 23:3170–82. doi: 10.1016/j.celrep.2018.05.022
28. Barker SA, Borjigin J, Lomnicka I, Strassman R. LC/MS/MS analysis of the endogenous dimethyltryptamine hallucinogens, their precursors, and major metabolites in rat pineal gland microdialysate. Biomed Chromatogr. (2013) 27:1690–700. doi: 10.1002/bmc.2981
29. Phillips M, Pozzo-Miller L. Dendritic spine dysgenesis in autism related disorders. Neurosci Lett. (2015) 601:30–40. doi: 10.1016/j.neulet.2015.01.011
30. Piven J, Elison JT, Zylka MJ. Toward a conceptual framework for early brain and behavior development in autism. Mol Psychiatry (2017) 22:1385–94. doi: 10.1038/mp.2017.131
31. Braam W, Ehrhart F, Maas APHM, Smits MG, Curfs L. Low maternal melatonin level increases autism spectrum disorder risk in children. Res Dev Disabil. (2018) 82:79–89. doi: 10.1016/j.ridd.2018.02.017
32. Reiter RJ, Mayo JC, Tan DX, Sainz RM, Alatorre-Jimenez M, Qin L. Melatonin as an antioxidant: under promises but over delivers. J Pineal Res. (2016) 61:253–78. doi: 10.1111/jpi.12360
33. Chauhan A, Chauhan V. Oxidative stress in autism. Pathophysiology (2006) 13:171–81. doi: 10.1016/j.pathophys.2006.05.007
34. Carrillo-Vico A, Lardone PJ, Alvarez-Sánchez N, Rodríguez-Rodríguez A, Guerrero JM. Melatonin: buffering the immune system. Int J Mol Sci. (2013) 14:8638–83. doi: 10.3390/ijms14048638
35. Ashwood P, Van de Water J. Is autism an autoimmune disease? Autoimmun Rev. (2004) 3:557–62. doi: 10.1016/j.autrev.2004.07.036
36. Sumida M, Barkovich AJ, Newton TH. Development of the pineal gland: measurement with MR. AJNR Am J Neuroradiol. (1996) 17:233–6.
37. Tauman R, Zisapel N, Laudon M, Nehama H, Sivan Y. Melatonin production in infants. Pediatr Neurol. (2002) 26:379–82. doi: 10.1016/S0887-8994(01)00417-9
38. Do B, Lynch P, Macris EM, Smyth B, Stavrinakis S, Quinn S, et al. Systematic review and meta-analysis of the association of Autism Spectrum Disorder in visually or hearing impaired children. Ophthalmic Physiol Opt. (2017) 37:212–24. doi: 10.1111/opo.12350
39. Mukaddes NM, Kilincaslan A, Kucukyazici G, Sevketoglu T, Tuncer S. Autism in visually impaired individuals. Psychiatry Clin Neurosci. (2007) 61:39–44. doi: 10.1111/j.1440-1819.2007.01608.x
40. Hull JT, Czeisler CA, Lockley SW. Suppression of melatonin secretion in totally visually blind people by ocular exposure to white light: clinical characteristics. Ophthalmology (2018) 125:1160–71. doi: 10.1016/j.ophtha.2018.01.036
41. Tyler CV, Schramm SC, Karafa M, Tang AS, Jain AK. Chronic disease risks in young adults with autism spectrum disorder: forewarned is forearmed. Am J Intellect Dev Disabil. (2011) 116:371–80. doi: 10.1352/1944-7558-116.5.371
42. Wen Y, Herbert MR. Connecting the dots: overlaps between autism and cancer suggest possible common mechanisms regarding signaling pathways related to metabolic alterations. Med Hypotheses (2017) 103:118–23. doi: 10.1016/j.mehy.2017.05.004
43. Reiter RJ, Rosales-Corral SA, Tan DX, Acuna-Castroviejo D, Qin L, Yang SF, et al. Melatonin, a full service anti-cancer agent: inhibition of initiation, progression and metastasis. Int J Mol Sci. (2017) 18:E843. doi: 10.3390/ijms18040843
44. Sánchez-Barceló EJ, Mediavilla MD, Tan DX, Reiter RJ. Clinical uses of melatonin: evaluation of human trials. Curr Med Chem. (2010) 17:2070–95.
45. Garfinkel D, Zorin M, Wainstein J, Matas Z, Laudon M, Zisapel N. Efficacy and safety of prolonged-release melatonin in insomnia patients with diabetes: a randomized, double-blind, crossover study. Diabetes Metab Syndr Obes. (2011) 4:307–13. doi: 10.2147/DMSO.S23904
46. Mulder H. Melatonin signalling and type 2 diabetes risk: too little, too much or just right? Diabetologia (2017) 60:826–9. doi: 10.1007/s00125-017-4249-8
47. Syed S, Moore KA, March E. A review of prevalence studies of Autism Spectrum Disorder by latitude and solar irradiance impact. Med Hypotheses (2017) 109:19–24. doi: 10.1016/j.mehy.2017.09.012
48. Abdullahi I, Leonard H, Cherian S, Mutch R, Glasson EJ, de Klerk N, et al. The Risk of neurodevelopmental disabilities in children of immigrant and refugee parents: current knowledge and directions for future research. Rev J Autism Dev Dis. (2018) 5:29–42. doi: 10.1007/s40489-017-0121-5
49. Mackay DF, Smith GC, Cooper SA, Wood R, King A, Clark DN, et al. Month of conception and learning disabilities: a record-linkage study of 801,592 children. Am J Epidemiol. (2016) 184:485–93. doi: 10.1093/aje/kww096
50. Cannell JJ. Vitamin D and autism, what's new? Rev Endocr Metab Disord. (2017) 18:183–93. doi: 10.1007/s11154-017-9409-0
51. Patrick RP, Ames BN. Vitamin D hormone regulates serotonin synthesis. Part 1: relevance for autism. FASEB J. (2014) 28:2398–413. doi: 10.1096/fj.13-246546
52. Gardener H, Spiegelman D, Buka SL. Perinatal and neonatal risk factors for autism: a comprehensive meta-analysis. Pediatrics (2011) 128:344–55. doi: 10.1542/peds.2010-1036
53. Tordjman S, Najjar I, Bellissant E, Anderson GM, Barburoth M, Cohen D, et al. Advances in the research of melatonin in autism spectrum disorders: literature review and new perspectives. Int J Mol Sci. (2013) 14:20508–42. doi: 10.3390/ijms141020508
54. Tseng PT, Chen YW, Stubbs B, Carvalho AF, Whiteley P, Tang CH, et al. Maternal breastfeeding and autism spectrum disorder in children: A systematic review and meta-analysis. Nutr Neurosci. 18:1–9. doi: 10.1080/1028415X.2017.1388598
55. Cohen Engler A, Hadash A, Shehadeh N, Pillar G. Breastfeeding may improve nocturnal sleep and reduce infantile colic: potential role of breast milk melatonin. Eur J Pediatr. (2012) 171:729–32. doi: 10.1007/s00431-011-1659-3
56. Adamsson M, Laike T, Morita T. Annual variation in daily light exposure and circadian change of melatonin and cortisol concentrations at a northern latitude with large seasonal differences in photoperiod length. J Physiol Anthropol. (2016) 36:6. doi: 10.1186/s40101-016-0103-9
57. Reiter RJ, Tamura H, Tan DX, Xu XY. Melatonin and the circadian system: contributions to successful female reproduction. Fertil Steril. (2014) 102:321–8. doi: 10.1016/j.fertnstert.2014.06.014
58. Hébert M, Martin SK, Lee C, Eastman CI. The effects of prior light history on the suppression of melatonin by light in humans. J Pineal Res. (2002) 33:198–203.
59. Blaychfeld-Magnazi M, Zornitzki T, Ulman M, Madar Z, Knobler H. Early beta-cell dysfunction characterizes males with type 2 diabetes of Yemenite origin. Acta Diabetol. (2016) 53:567–74. doi: 10.1007/s00592-016-0838-0
60. Magnusson C, Rai D, Goodman A, Lundberg M, Idring S, Svensson A, et al. Migration and autism spectrum disorder: population-based study. Br J Psychiatry (2012) 201:109–15. doi: 10.1192/bjp.bp.111.095125
61. Shi Y, Fang YY, Wei YP, Jiang Q, Zeng P, Tang N, et al. Melatonin in synaptic impairments of Alzheimer's Disease. J Alzheimers Dis. (2018) 63:911–26. doi: 10.3233/JAD-171178
62. Tang G, Gudsnuk K, Kuo SH, Cotrina ML, Rosoklija G, Sosunov A, et al. Loss of mTOR-dependent macroautophagy causes autistic-like synaptic pruning deficits. Neuron (2014) 83:1131–43. doi: 10.1016/j.neuron.2014.07.040
63. Winden KD, Ebrahimi-Fakhari D, Sahin M. Abnormal mTOR Activation in Autism. Annu Rev Neurosci. (2018) 41:1–23. doi: 10.1146/annurev-neuro-080317-061747
64. Saghazadeh A, Rezaei N. Brain-Derived neurotrophic factor levels in autism: a systematic review and meta-analysis. J Autism Dev Disord. (2017) 47:1018–29. doi: 10.1007/s10803-016-3024-x
65. Jang SW, Liu X, Pradoldej S, Tosini G, Chang Q, Iuvone PM, et al. N-acetylserotonin activates TrkB receptor in a circadian rhythm. Proc Natl Acad Sci USA. (2010) 107:3876–81. doi: 10.1073/pnas.0912531107
66. Dakic V, Minardi Nascimento J, Costa Sartore R, Maciel RM, de Araujo DB, Ribeiro S, et al. Short term changes in the proteome of human cerebral organoids induced by 5-MeO-DMT. Sci Rep. (2017) 7:12863. doi: 10.1038/s41598-017-12779-5
67. Martin DA, Marona-Lewicka D, Nichols DE, Nichols CD. Chronic LSD alters gene expression profiles in the mPFC relevant to schizophrenia. Neuropharmacology (2014) 83:1–8. doi: 10.1016/j.neuropharm.2014.03.013
69. Barker SA, McIlhenny EH, Strassman R. A critical review of reports of endogenous psychedelic N, N-dimethyltryptamines in humans: 1955–2010. Drug Test Anal. (2012) 4:617–35. doi: 10.1002/dta.422
70. Carbonaro TM, Gatch MB. Neuropharmacology of N,N-dimethyltryptamine. Brain Res Bull. (2016) 126(Pt 1):74–88. doi: 10.1016/j.brainresbull.2016.04.016
71. Mandell AJ, Morgan M. Indole(ethyl)amine N-methyltransferase in human brain. Nat New Biol. (1971) 230:85–7.
72. Saavedra JM, Coyle JT, Axelrod J. The distribution and properties of the nonspecific N-methyltransferase in brain. J Neurochem. (1973) 20:743–52.
73. Dean JG. Indolethylamine-N-methyltransferase polymorphisms: genetic and biochemical approaches for study of endogenous N,N,-dimethyltryptamine. Front Neurosci. (2018) 12:232. doi: 10.3389/fnins.2018.00232
74. Barker SA. N,N-dimethyltryptamine facts and myths. J Psychopharmacol. (2018a) 32:820–1. doi: 10.1177/0269881118767648
75. Nichols DE. N,N-dimethyltryptamine and the pineal gland: separating fact from myth. J Psychopharmacol. (2018) 32:30–6. doi: 10.1177/0269881117736919
76. Barker SA. N, N-Dimethyltryptamine (DMT), an endogenous hallucinogen: past, present, and future research to determine its role and function. Front Neurosci. (2018b) 12:536. doi: 10.3389/fnins.2018.00536
77. Madhusoodanan S, Ting MB, Farah T, Ugur U. Psychiatric aspects of brain tumors: a review. World J Psychiatry (2015) 5:273–85. doi: 10.5498/wjp.v5.i3.273
78. Miyazawa T, Fukui S, Otani N, Tsuzuki N, Katoh H, Ishihara S, et al. Peduncular hallucinosis due to a pineal meningioma. Case Rep J Neurosurg. (2001) 95:500–2. doi: 10.3171/jns.2001.95.3.0500
79. Craven C. Pineal germinoma and psychosis. J Am Acad Child Adolesc Psychiatry (2001) 40:6. doi: 10.1097/00004583-200101000-00008
80. Baron-Cohen S, Johnson D, Asher J, Wheelwright S, Fisher SE, Gregersen PK, et al. Is synaesthesia more common in autism? Mol Autism (2013) 4:40. doi: 10.1186/2040-2392-4-40
81. Baron-Cohen S, Ashwin E, Ashwin C, Tavassoli T, Chakrabarti B. Talent in autism: hyper-systemizing, hyper-attention to detail and sensory hypersensitivity. Philos Trans R Soc Lond B Biol Sci. (2009) 364:1377–83. doi: 10.1098/rstb.2008.0337
82. Shi L, Zhou Y, Ou J, Gong J, Wang S, Cui X, et al. Different visual preference patterns in response to simple and complex dynamic social stimuli in preschool-aged children with autism spectrum disorders. PLoS ONE (2015) 10:e0122280. doi: 10.1371/journal.pone.0122280
83. Milne E, Dickinson A, Smith R. Adults with autism spectrum conditions experience increased levels of anomalous perception. PLoS ONE (2017) 12:e0177804. doi: 10.1371/journal.pone.0177804
84. Anderson CJ, Colombo J. Larger tonic pupil size in young children with autism spectrum disorder. Dev Psychobiol. (2009) 51:207–11. doi: 10.1002/dev.20352
85. DiCriscio AS, Troiani V. Pupil adaptation corresponds to quantitative measures of autism traits in children. Sci Rep. (2017) 7:6476. doi: 10.1038/s41598-017-06829-1
86. Higuchi S, Ishibashi K, Aritake S, Enomoto M, Hida A, Tamura M, et al. Inter-individual difference in pupil size correlates to suppression of melatonin by exposure to light. Neurosci Lett. (2008) 440:23–6. doi: 10.1016/j.neulet.2008.05.037
87. Julia S, Edward H, Daphne M, Laura CG, Ferrinne S. Oxford handbook of synesthesia. In: Synesthesia in Infants and Very Young Children. Oxford, UK: Oxford University Press (2013) doi: 10.1093/oxfordhb/9780199603329.013.0003
88. Emanuele E, Colombo R, Martinelli V, Brondino N, Marini M, Boso M, et al. Elevated urine levels of bufotenine in patients with autistic spectrum disorders and schizophrenia. Neuro Endocrinol Lett. (2010) 31:117–21.
89. Beaton JM, Morris PE. Ontogeny of N,N-dimethyltryptamine and related indolealkylamine levels in neonatal rats. Mech Ageing Dev. (1984) 25:343–7.
90. Gu F, Chauhan V, Chauhan A. Monoamine oxidase-A and B activities in the cerebellum and frontal cortex of children and young adults with autism. J Neurosci Res. (2017) 95:1965–72. doi: 10.1002/jnr.24027
91. de la Fuente Revenga M, Pérez C, Morales-García JA, Alonso-Gil S, Pérez-Castillo A, Caignard DH, et al. Neurogenic potential assessment and pharmacological characterization of 6-Methoxy-1,2,3,4-tetrahydro-β-carboline (Pinoline) and melatonin-pinoline hybrids. ACS Chem Neurosci. (2015) 6:800–10. doi: 10.1021/acschemneuro.5b00041
92. Kałuzna-Czaplinska J, Józwik-Pruska J, Axt A. Chromatographic determination of harmalans in the urine of autistic children. Biomed Chromatogr. (2017) 31:e3951. doi: 10.1002/bmc.3951
93. Davis LK, Hazlett HC, Librant AL, Nopoulos P, Sheffield VC, Piven J, et al. Cortical enlargement in autism is associated with a functional VNTR in the monoamine oxidase A gene. Am J Med Genet B Neuropsychiatr Genet. (2008) 147B, 1145–51. doi: 10.1002/ajmg.b.30738
94. Fontanilla D, Johannessen M, Hajipour AR, Cozzi NV, Jackson MB, Ruoho AE. The hallucinogen N,N-dimethyltryptamine (DMT) is an endogenous sigma-1 receptor regulator. Science (2009) 323:934–7. doi: 10.1126/science.1166127
95. Rickli A, Moning OD, Hoener MC, Liechti ME. Receptor interaction profiles of novel psychoactive tryptamines compared with classic hallucinogens. Eur Neuropsychopharmacol. (2016) 26:1327–37. doi: 10.1016/j.euroneuro.2016.05.001
96. Muller CL, Anacker AMJ, Veenstra-VanderWeele J. The serotonin system in autism spectrum disorder: from biomarker to animal models. Neuroscience (2016) 321:24–41. doi: 10.1016/j.neuroscience.2015.11.010
97. Bruni O, Alonso-Alconada D, Besag F, Biran V, Braam W, Cortese S, et al. Current role of melatonin in pediatric neurology: clinical recommendations. Eur J Paediatr Neurol. (2015) 19:122–33. doi: 10.1016/j.ejpn.2014.12.007
98. Gagnon K, Godbout R. Melatonin and comorbidities in children with Autism Spectrum Disorder. Curr Dev Disord Rep. (2018) 5:197–206. doi: 10.1007/s40474-018-0147-0
Keywords: autism, pineal gland, N, N-dimethyltryptamine (DMT), melatonin, neural plasticity
Citation: Shomrat T and Nesher N (2019) Updated View on the Relation of the Pineal Gland to Autism Spectrum Disorders. Front. Endocrinol. 10:37. doi: 10.3389/fendo.2019.00037
Received: 16 October 2018; Accepted: 16 January 2019;
Published: 05 February 2019.
Edited by:
Heather K. Caldwell, Kent State University, United StatesReviewed by:
Ben Nephew, Worcester Polytechnic Institute, United StatesCopyright © 2019 Shomrat and Nesher. This is an open-access article distributed under the terms of the Creative Commons Attribution License (CC BY). The use, distribution or reproduction in other forums is permitted, provided the original author(s) and the copyright owner(s) are credited and that the original publication in this journal is cited, in accordance with accepted academic practice. No use, distribution or reproduction is permitted which does not comply with these terms.
*Correspondence: Tal Shomrat, dGFsc2hAcnVwcGluLmFjLmls
Disclaimer: All claims expressed in this article are solely those of the authors and do not necessarily represent those of their affiliated organizations, or those of the publisher, the editors and the reviewers. Any product that may be evaluated in this article or claim that may be made by its manufacturer is not guaranteed or endorsed by the publisher.
Research integrity at Frontiers
Learn more about the work of our research integrity team to safeguard the quality of each article we publish.