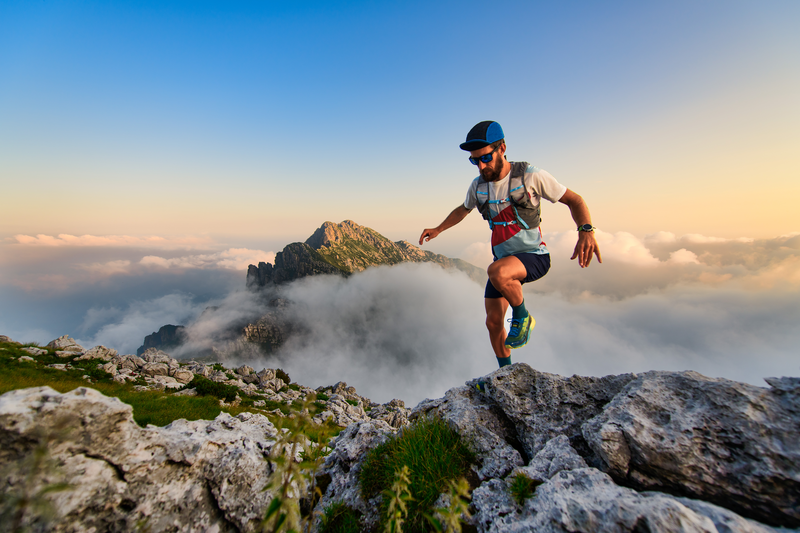
94% of researchers rate our articles as excellent or good
Learn more about the work of our research integrity team to safeguard the quality of each article we publish.
Find out more
REVIEW article
Front. Endocrinol. , 30 January 2019
Sec. Experimental Endocrinology
Volume 10 - 2019 | https://doi.org/10.3389/fendo.2019.00026
This article is part of the Research Topic Cross Talk Between The Immune System and Metabolism View all 14 articles
As a cytokine in interleukin-1(IL-1) family, interleukin-33(IL-33) usually exists in the cytoplasm and cell nucleus. When the cells are activated or damaged, IL-33 can be secreted into extracellular and regulate the functions of various immune cells through binding to its specific receptor suppression of tumorigenicity 2 (ST2). Except regulating the function of immune cells including T cells, B cells, dendritic cells (DCs), macrophages, mast cells, and innate lymphoid cells, IL-33 also plays an important role in metabolic diseases and has received an increasing attention. This review summarizes the regulation of IL-33 on different immune cells in lipid metabolism, which will help to understand the pathology of abnormal lipid metabolic diseases, such as atherosclerosis and type 2 diabetes.
IL-33, a new member of the IL-1 family, was discovered in 2005 (1) while its receptor ST2 containing intracellular domain Toll/IL-1R (TIR) was found in BALB/c-3t3 mouse fibroblasts in 1989 (1, 2). The receptor complex of IL-33 is composed of ST2 and interleukin-1 receptor accessory protein (IL-1RAcP). IL-33 mediates its biological effect through binding to its specific receptor ST2 (2, 3), whereas the expression of ST2 is restricted and determines the cellular responsiveness to IL-33 treatment (3). Two forms of ST2 have been demonstrated, a membrane-bound form (ST2L) and a soluble form (sST2), the latter which prevents its signaling as the decoy receptor for IL-33. IL-33 is mainly expressed in fibroblasts, epithelial cells and endothelial cells, and especially in high endothelial venules (HEV) (4). Indeed, as designated as an “alarmin,” IL-33 is usually released after cell injury to alert the immune system and initiate repair processes. In a recent study, islet mesenchymal-cell-derived IL-33 has been identified as an islet immunoregulatory feature (5). As the receptor of IL-33, ST2 is expressed in many immune cells. IL-33 is a dual-function cytokine. In the absence of inflammatory stimulation, IL-33 is located in the nucleus as a nuclear factor. Once the cell is damaged and/or necrotic, IL-33 can be released from the nucleus and then act as an endogenous “alarmin” (4). The activation signal produced by IL-33/ST2 pathway is transmitted to the cell and a series of signal transmissions activate nuclear factor kappa-light-chain-enhancer of activated B cells (NF-κB) and mitogen-activated protein kinase (MAPK) pathway to regulate immune response (1, 6). Under normal physiological condition, inflammation induced by a dysregulated lipid metabolism is benefit for the maintenance of homeostasis and is controlled to avoid excessive damage to the host. However, if not properly controlled, the inflammatory response will promote the excessive production of lipid metabolites, inflammatory cytokines and adhesion molecules, which lead to acute or chronic diseases (7), such as obesity, non-alcoholic steatohepatitis (NASH), atherosclerosis, and acute cardiovascular events. To date, an increasing body of evidence has demonstrated that IL-33 plays a critical role in the lipid metabolism. This review highlights the function of IL-33/ST2 axis on different immune cells in the metabolic disorders.
IL-33 and ST2 have been shown to be expressed in human and murine adipose tissue, and IL-33 expression is strongly correlated with leptin expression in human adipose tissue (8). In addition, administration of IL-33 increases browning of white adipose tissue and energy expenditure in mice (9). These observations show that a critical role of IL-33 played in the adipose tissues homeostasis.
Macrophages have functional plasticity in adipose tissue inflammation, which can exhibit pro-inflammatory or anti-inflammatory function. According to the phenotypes and secreted cytokines, macrophages can be divided into two categories named as classical activated macrophages (CAM, M1 type) and alternatively activated macrophages (AAM, M2 type), respectively. CAM are generated in response to helper T1 cells (Th1 cells)-related cytokines, such as interferon-γ (IFN-γ) and tumor necrosis factor-α (TNF-α), while AAM polarization is linked to the helper T2 cells (Th2 cells)-related cytokines (IL-4 and IL-13) (10). Previous studies showed that AAM could attenuate adipose tissue inflammation and obesity-induced insulin resistance (11–14). It has been showed that ST2 can be detected on the cell surface of macrophages. IL-33 can promote the expression of lipopolysaccharide (LPS) receptor components such as myeloid differentiation factor 2 (MD2), toll-like receptor (TLR) 4, soluble cluster of differentiation 14 (CD14) and myeloid differentiation primary response gene 88 (Myd88), which result in an enhanced inflammatory cytokine production (15). However, IL-33 administration improves glucose tolerance, which is associated with the accumulation of M2 macrophages in adipose tissue of ob/ob mice that are the mutant mice to construct the model of Type II diabetes (16). As the result of purine metabolism disorder, gout is a very common metabolic disease in human (17, 18). Hyperlipidaemia is common in gout patients including increased low-density lipoprotein (LDL) cholesterol and decreased high-density lipoprotein (HDL) cholesterol (19). The serum IL-33 expression is predominantly increased in gout patients compared to healthy controls and positively correlated with the expression of HDL, while negatively correlated with LDL expression (20). It has been reported that the elevated IL-33 level is considerably reduced in renal impairment when compared with normal renal function in gout patients (20–22). These data suggest that IL-33 may prevent the kidney injury through regulating the lipid metabolism, which may be resulted from the AAM polarization.
Although ST2 can be detected on the cell surface of macrophages, IL-33/ST2 signaling cannot directly promote AAM polarization. The involvement of IL-33/ST2 signaling in the differentiation and activation of AAM is associated with type II cytokines induction (23–25). A previous finding showed that a population of cells expressing ST2 in adipose was potential to produce large amounts of Th2 cytokines in response to IL-33 (26). Recent studies have named this population as group 2 innate lymphoid cells (ILC2s), characterized by expressing ST2 receptor, and secreting type 2 cytokines such as IL-5 and IL-13 in response to IL-33 (27–30). In addition, soluble ST2 can prevent ILC2s from IL-33 stimulation (31). Recent observation has shown that ILC2s activation favors macrophages toward a protective AAM, which lead to a reduced lipid storage and decrease gene expression of lipid metabolism and adiposeness (32). Furthermore, it has showed that IL-13Rα2 may act as a critical checkpoint in the protective effect of the IL-33/IL-13 axis in obesity (33). In addition, IL-33 promotes β cell function through islet-resident ILC2s that elicite retinoic acid (RA)-producing capacities in macrophages and dendritic cells via the secretion of IL-13 and colony-stimulating factor 2 (5). These data suggest that IL-33 plays a protective role in the adipose tissue inflammation through regulating macrophage function, which is closely associated with the activation of ILC2 to produce type 2 cytokine and IL-4Rα signaling.
As a subset of T cells, the regulatory T cells (Tregs) play a critical role in suppressing autoimmune reactivity and have gained an increasing attention in the autoimmune diseases (34). It is shown that an impaired Tregs function is investigated in ST2 gene knockout mice with streptozotocin-induced diabetes, where the glycaemia and β cell loss are severe (35). Indeed, the exogenous IL-33 treatment propagates Tregs expressing the ST2 on the cellular surface, which suggests that the Tregs expansion induced by IL-33 administration is likely to be the result of a direct effect of IL-33 on ST2L+ Tregs (36, 37). Besides, ST2+ DCs stimulated by IL-33 to secrete IL-2, which promotes the selective expansion of ST2+ Tregs vs. non-Tregs, are required for in vitro and in vivo Tregs expansion (37, 38). In the Th1/Th17-mediated allograft rejection, IL-33 treatment can prevent allograft rejection through increasing ST2 positive Tregs in mice (39). In the mouse model of trinitrobenzene sulfonic acid (TNBS)-induced colitis, dextran sulfate sodium (DSS)-induced colitis or T cell adoptive transfer induced colitis, IL-33 can increase the number of Foxp3+ Tregs (40–42).
The Tregs also play a immunosuppressive function in obesity-associated inflammation (43). Interestingly, studies have also demonstrated that IL-33 maintain homeostasis in adipose tissue. A high level of ST2 expression is observed on human adipose tissue Tregs. Furthermore, IL-33 treatment can induce vigorous population expansion of Tregs in obese mice, and the changes of metabolic parameters are significantly correlated with the increased Tregs (44, 45). IL-33 signaling through the IL-33 receptor ST2 and the myeloid differentiation factor MyD88 pathway is essential for the development and maintenance of Tregs in visceral adipose tissue (44). However, ILC2-intrinsic IL-33 activation is required for Tregs accumulation in vivo and is independent of ILC2 type 2 cytokines but partially dependent on direct co-stimulatory interactions via the inducible costimulator ligand (ICOSL)/ICOS pathway (46). Concordantly, the ST2+ Tregs population is with a higher expression of activated marker ICOS and CD44 (38). Thus, IL-33 plays a protective role in adipose tissue inflammation through directly and indirectly regulating Tregs function.
It has also been reported that increasing severity of insulin resistance and microalbuminuria is strongly correlated with the decreased level of IL-33 in patients with diabetic nephropathy, where an enhanced Th1 and suppressed Th2 response is observed (47). ST2 is selectively and stably expressed on the surface of Th2 cells, and IL-33 can effectively induce the immune response of Th2 cells and the expression of Th2 related cytokines IL-5 and IL-13 without increasing IFN-γ expression (48, 49). These studies suggest that the ST2/IL-33 axis is closely associated with the Th1/Th2 response imbalance in the development of diabetes. Atherosclerosis is characterized by the formation of fibrotic plaques in the major arteries and increased Th1 immune response, which leads to myocardinfal iarction and stroke (50, 51). It has been shown that Th1-to-Th2 shift can reduce the development of atherosclerosis (52, 53). Due to the effect of IL-33 on Th2-type immune response, IL-33 exhibits a protective role in the pathogenesis of atherosclerosis (54). Previous findings also showed that the reduced level of IL-33 might increase the risk of atherosclerosis development for certain individuals (55). These data suggest a crucial role of IL-33 in the lipid metabolism through regulating T cells differentiation.
Due to the vital role of IL-33 in the metabolic homeostasis, a sound understanding of the production, regulation, and function of IL-33 will facilitate the treatment of metabolic disorders. The potential mechanisms (Figure 1) of IL-33/ST2 axis in the metabolic disorders may include: (1) IL-33 promotes the AAM polarization; (2) IL-33 regulates Tregs and Th2 differentiation and function; and (3) IL-33 regulates the function of ILC2. Notably, the AAM polarization induced by IL-33 depends on Type 2 cytokines, which may be released from ILC2. However, most studies in this area were mainly carried out on animal models and there were limited clinical trials. To what extent IL-33 contributes to metabolic disorders in humans still requires further investigation.
Figure 1. The regulatory role of IL-33 in metabolic diseases. IL-33/ST2 axis regulates metabolic diseases through: (1) promoting AAM polarization; (2) regulating the differentiation and functions of Treg and Th2; and (3) regulating the function of ILC2.
LT and LY reviewed the literature and wrote the first draft. LY finalized the manuscript. LT and LY have read and approved the final manuscript.
This work was supported by the National Natural Science Foundation of China 81700490 to LT.
The authors declare that the research was conducted in the absence of any commercial or financial relationships that could be construed as a potential conflict of interest.
1. Schmitz J, Owyang A, Oldham E, Song Y, Murphy E, McClanahan TK, et al. IL-33, an interleukin-1-like cytokine that signals via the IL-1 receptor-related protein ST2 and induces T helper type 2-associated cytokines. Immunity (2005) 23:479–90. doi: 10.1016/j.immuni.2005.09.015
2. Baekkevold ES, Roussigne M, Yamanaka T, Johansen FE, Jahnsen FL, Amalric F, et al. Molecular characterization of NF-HEV, a nuclear factor preferentially expressed in human high endothelial venules. Am J Pathol. (2003) 163:69–79. doi: 10.1016/S0002-9440(10)63631-0
3. Louten J, Rankin AL, Li Y, Murphy EE, Beaumont M, Moon C, et al. Endogenous IL-33 enhances Th2 cytokine production and T-cell responses during allergic airway inflammation. Int Immunol. (2011) 23:307–15. doi: 10.1093/intimm/dxr006
4. Moussion C, Ortega N, Girard JP. The IL-1-like cytokine IL-33 is constitutively expressed in the nucleus of endothelial cells and epithelial cells in vivo: a novel ‘alarmin’? PLoS ONE (2008) 3:e3331. doi: 10.1371/journal.pone.0003331
5. Dalmas E, Lehmann FM, Dror E, Wueest S, Thienel C, Borsigova M, et al. Interleukin-33-activated islet-resident innate lymphoid cells promote insulin secretion through myeloid cell retinoic acid production. Immunity (2017) 47:928–42.e7. doi: 10.1016/j.immuni.2017.10.015
6. Luthi AU, Cullen SP, McNeela EA, Duriez PJ, Afonina IS, Sheridan C, et al. Suppression of interleukin-33 bioactivity through proteolysis by apoptotic caspases. Immunity (2009) 31:84–98. doi: 10.1016/j.immuni.2009.05.007
7. Calder PC. n-3 polyunsaturated fatty acids, inflammation, and inflammatory diseases. Am J Clin Nutr. (2006) 83 (Suppl. 6):1505S−19S. doi: 10.1093/ajcn/83.6.1505S
8. Zeyda M, Wernly B, Demyanets S, Kaun C, Hammerle M, Hantusch B, et al. Severe obesity increases adipose tissue expression of interleukin-33 and its receptor ST2, both predominantly detectable in endothelial cells of human adipose tissue. Int J Obes. (2013) 37:658–65. doi: 10.1038/ijo.2012.118
9. Lee MW, Odegaard JI, Mukundan L, Qiu Y, Molofsky AB, Nussbaum JC, et al. Activated type 2 innate lymphoid cells regulate beige fat biogenesis. Cell (2015) 160:74–87. doi: 10.1016/j.cell.2014.12.011
10. Mills CD. Anatomy of a discovery: m1 and m2 macrophages. Front Immunol. (2015) 6:212. doi: 10.3389/fimmu.2015.00212
11. Weisberg SP, McCann D, Desai M, Rosenbaum M, Leibel RL, Ferrante AW Jr. Obesity is associated with macrophage accumulation in adipose tissue. J Clin Investig. (2003) 112:1796–808. doi: 10.1172/JCI19246
12. Xu H, Barnes GT, Yang Q, Tan G, Yang D, Chou CJ, et al. Chronic inflammation in fat plays a crucial role in the development of obesity-related insulin resistance. J Clin Investig. (2003) 112:1821–30. doi: 10.1172/JCI19451
13. Wynn TA, Chawla A, Pollard JW. Macrophage biology in development, homeostasis and disease. Nature (2013) 496:445–55. doi: 10.1038/nature12034
14. Chawla A, Nguyen KD, Goh YP. Macrophage-mediated inflammation in metabolic disease. Nat Rev Immunol. (2011) 11:738–49. doi: 10.1038/nri3071
15. Espinassous Q, Garcia-de-Paco E, Garcia-Verdugo I, Synguelakis M, von Aulock S, Sallenave JM, et al. IL-33 enhances lipopolysaccharide-induced inflammatory cytokine production from mouse macrophages by regulating lipopolysaccharide receptor complex. J Immunol. (2009) 183:1446–55. doi: 10.4049/jimmunol.0803067
16. Miller AM, Asquith DL, Hueber AJ, Anderson LA, Holmes WM, McKenzie AN, et al. Interleukin-33 induces protective effects in adipose tissue inflammation during obesity in mice. Circ Res. (2010) 107:650–8. doi: 10.1161/CIRCRESAHA.110.218867
18. McCarty DJ, Hollander JL. Identification of urate crystals in gouty synovial fluid. Ann Intern Med. (1961) 54:452–60.
19. Takahashi S, Yamamoto T, Moriwaki Y, Tsutsumi Z, Higashino K. Impaired lipoprotein metabolism in patients with primary gout–influence of alcohol intake and body weight. Br J Rheumatol. (1994) 33:731–4.
20. Duan L, Huang Y, Su Q, Lin Q, Liu W, Luo J, et al. Potential of IL-33 for preventing the kidney injury via regulating the lipid metabolism in gout patients. J Diabetes Res. (2016) 2016:1028401. doi: 10.1155/2016/1028401
21. Chen J, Muntner P, Hamm LL, Jones DW, Batuman V, Fonseca V, et al. The metabolic syndrome and chronic kidney disease in U.S. adults. Ann Intern Med. (2004) 140:167–74. doi: 10.7326/0003-4819-140-3-200402030-00007
22. Kurella M, Lo JC, Chertow GM. Metabolic syndrome and the risk for chronic kidney disease among nondiabetic adults. J Am Soc Nephrol. (2005) 16:2134–40. doi: 10.1681/ASN.2005010106
23. Li D, Guabiraba R, Besnard AG, Komai-Koma M, Jabir MS, Zhang L, et al. IL-33 promotes ST2-dependent lung fibrosis by the induction of alternatively activated macrophages and innate lymphoid cells in mice. J Allergy Clin Immunol. (2014) 134:1422–32.e11. doi: 10.1016/j.jaci.2014.05.011
24. Kurowska-Stolarska M, Stolarski B, Kewin P, Murphy G, Corrigan CJ, Ying S, et al. IL-33 amplifies the polarization of alternatively activated macrophages that contribute to airway inflammation. J Immunol. (2009) 183:6469–77. doi: 10.4049/jimmunol.0901575
25. Kurowska-Stolarska M, Kewin P, Murphy G, Russo RC, Stolarski B, Garcia CC, et al. IL-33 induces antigen-specific IL-5+ T cells and promotes allergic-induced airway inflammation independent of IL-4. J Immunol. (2008) 181:4780–90. doi: 10.4049/jimmunol.181.7.4780
26. Moro K, Yamada T, Tanabe M, Takeuchi T, Ikawa T, Kawamoto H, et al. Innate production of T(H)2 cytokines by adipose tissue-associated c-Kit+Sca-1+ lymphoid cells. Nature (2010) 463:540–4. doi: 10.1038/nature08636
27. Mjosberg JM, Trifari S, Crellin NK, Peters CP, van Drunen CM, Piet B, et al. Human IL-25- and IL-33-responsive type 2 innate lymphoid cells are defined by expression of CRTH2 and CD161. Nat Immunol. (2011) 12:1055–62. doi: 10.1038/ni.2104
28. Lefrancais E, Duval A, Mirey E, Roga S, Espinosa E, Cayrol C, et al. Central domain of IL-33 is cleaved by mast cell proteases for potent activation of group-2 innate lymphoid cells. Proc Nat Acad Sci USA. (2014) 111:15502–7. doi: 10.1073/pnas.1410700111
29. Johansson K, Malmhall C, Ramos-Ramirez P, Radinger M. Bone marrow type 2 innate lymphoid cells: a local source of interleukin-5 in interleukin-33-driven eosinophilia. Immunology (2018) 153:268–78. doi: 10.1111/imm.12842
30. Camelo A, Rosignoli G, Ohne Y, Stewart RA, Overed-Sayer C, Sleeman MA, et al. IL-33, IL-25, and TSLP induce a distinct phenotypic and activation profile in human type 2 innate lymphoid cells. Blood Adv. (2017) 1:577–89. doi: 10.1182/bloodadvances.2016002352
31. Hayakawa H, Hayakawa M, Tominaga SI. Soluble ST2 suppresses the effect of interleukin-33 on lung type 2 innate lymphoid cells. Biochem Biophys Rep. (2016) 5:401–7. doi: 10.1016/j.bbrep.2016.02.002
32. Molofsky AB, Nussbaum JC, Liang HE, Van Dyken SJ, Cheng LE, Mohapatra A, et al. Innate lymphoid type 2 cells sustain visceral adipose tissue eosinophils and alternatively activated macrophages. J Exp Med. (2013) 210:535–49. doi: 10.1084/jem.20121964
33. Duffen J, Zhang M, Masek-Hammerman K, Nunez A, Brennan A, Jones JEC, et al. Modulation of the IL-33/IL-13 axis in obesity by IL-13Ralpha2. J Immunol. (2018) 200:1347–59. doi: 10.4049/jimmunol.1701256
34. Miyara M, Ito Y, Sakaguchi S. TREG-cell therapies for autoimmune rheumatic diseases. Nat Rev Rheumatol. (2014) 10:543–51. doi: 10.1038/nrrheum.2014.105
35. Zdravkovic N, Shahin A, Arsenijevic N, Lukic ML, Mensah-Brown EP. Regulatory T cells and ST2 signaling control diabetes induction with multiple low doses of streptozotocin. Mol Immunol. (2009) 47:28–36. doi: 10.1016/j.molimm.2008.12.023
36. Biton J, Khaleghparast Athari S, Thiolat A, Santinon F, Lemeiter D, Herve R, et al. In vivo expansion of activated Foxp3+ regulatory T cells and establishment of a type 2 immune response upon IL-33 treatment protect against experimental arthritis. J Immunol. (2016) 197:1708–19. doi: 10.4049/jimmunol.1502124
37. Matta BM, Turnquist HR. Expansion of regulatory T cells in vitro and in vivo by IL-33. Methods Mol Biol. (2016) 1371:29–41. doi: 10.1007/978-1-4939-3139-2_3
38. Matta BM, Lott JM, Mathews LR, Liu Q, Rosborough BR, Blazar BR, et al. IL-33 is an unconventional Alarmin that stimulates IL-2 secretion by dendritic cells to selectively expand IL-33R/ST2+ regulatory T cells. J Immunol. (2014) 193:4010–20. doi: 10.4049/jimmunol.1400481
39. Turnquist HR, Zhao Z, Rosborough BR, Liu Q, Castellaneta A, Isse K, et al. IL-33 expands suppressive CD11b+ Gr-1(int) and regulatory T cells, including ST2L+ Foxp3+ cells, and mediates regulatory T cell-dependent promotion of cardiac allograft survival. J Immunol. (2011) 187:4598–610. doi: 10.4049/jimmunol.1100519
40. Duan L, Chen J, Zhang H, Yang H, Zhu P, Xiong A, et al. Interleukin-33 ameliorates experimental colitis through promoting Th2/Foxp3+ regulatory T-cell responses in mice. Mol Med. (2012) 18:753–61. doi: 10.2119/molmed.2011.00428
41. Schiering C, Krausgruber T, Chomka A, Frohlich A, Adelmann K, Wohlfert EA, et al. The alarmin IL-33 promotes regulatory T-cell function in the intestine. Nature (2014) 513:564–8. doi: 10.1038/nature13577
42. Zhu J, Xu Y, Zhu C, Zhao J, Meng X, Chen S, et al. IL-33 induces both regulatory B cells and regulatory T cells in dextran sulfate sodium-induced colitis. Int Immunopharmacol. (2017) 46:38–47. doi: 10.1016/j.intimp.2017.02.006
43. Feuerer M, Herrero L, Cipolletta D, Naaz A, Wong J, Nayer A, et al. Lean, but not obese, fat is enriched for a unique population of regulatory T cells that affect metabolic parameters. Nat Med. (2009) 15:930–9. doi: 10.1038/nm.2002
44. Vasanthakumar A, Moro K, Xin A, Liao Y, Gloury R, Kawamoto S, et al. The transcriptional regulators IRF4, BATF and IL-33 orchestrate development and maintenance of adipose tissue-resident regulatory T cells. Nat Immunol. (2015) 16:276–85. doi: 10.1038/ni.3085
45. Kolodin D, van Panhuys N, Li C, Magnuson AM, Cipolletta D, Miller CM, et al. Antigen- and cytokine-driven accumulation of regulatory T cells in visceral adipose tissue of lean mice. Cell Metab. (2015) 21:543–57. doi: 10.1016/j.cmet.2015.03.005
46. Molofsky AB, Van Gool F, Liang HE, Van Dyken SJ, Nussbaum JC, Lee J, et al. Interleukin-33 and interferon-gamma counter-regulate group 2 innate lymphoid cell activation during immune perturbation. Immunity (2015) 43:161–74. doi: 10.1016/j.immuni.2015.05.019
47. Anand G, Vasanthakumar R, Mohan V, Babu S, Aravindhan V. Increased IL-12 and decreased IL-33 serum levels are associated with increased Th1 and suppressed Th2 cytokine profile in patients with diabetic nephropathy (CURES-134). Int J Clin Exp Pathol. (2014) 7:8008–15.
48. Xu D, Chan WL, Leung BP, Huang F, Wheeler R, Piedrafita D, et al. Selective expression of a stable cell surface molecule on type 2 but not type 1 helper T cells. J Exp Med. (1998) 187:787–94.
49. Yin H, Li XY, Jin XB, Zhang BB, Gong Q, Yang H, et al. IL-33 prolongs murine cardiac allograft survival through induction of TH2-type immune deviation. Transplantation (2010) 89:1189–97. doi: 10.1097/TP.0b013e3181d720af
50. Hansson GK, Libby P. The immune response in atherosclerosis: a double-edged sword. Nat Rev Immunol. (2006) 6:508–19. doi: 10.1038/nri1882
51. Lusis AJ, Mar R, Pajukanta P. Genetics of atherosclerosis. Ann Rev Genomics Hum Genet. (2004) 5:189–218. doi: 10.1146/annurev.genom.5.061903.175930
53. de Boer OJ, van der Wal AC, Verhagen CE, Becker AE. Cytokine secretion profiles of cloned T cells from human aortic atherosclerotic plaques. J Pathol. (1999) 188:174–9. doi: 10.1002/(SICI)1096-9896(199906)188:2<174::AID-PATH333>3.0.CO;2-3
54. Miller AM, Xu D, Asquith DL, Denby L, Li Y, Sattar N, et al. IL-33 reduces the development of atherosclerosis. J Exp Med. (2008) 205:339–46. doi: 10.1084/jem.20071868
Keywords: IL-33, metabolism, diabetes, innate & adaptive immune response, ST2
Citation: Tu L and Yang L (2019) IL-33 at the Crossroads of Metabolic Disorders and Immunity. Front. Endocrinol. 10:26. doi: 10.3389/fendo.2019.00026
Received: 23 October 2018; Accepted: 15 January 2019;
Published: 30 January 2019.
Edited by:
Jixin Zhong, Case Western Reserve University, United StatesReviewed by:
Yanbo Yu, Qilu Hospital of Shandong University, ChinaCopyright © 2019 Tu and Yang. This is an open-access article distributed under the terms of the Creative Commons Attribution License (CC BY). The use, distribution or reproduction in other forums is permitted, provided the original author(s) and the copyright owner(s) are credited and that the original publication in this journal is cited, in accordance with accepted academic practice. No use, distribution or reproduction is permitted which does not comply with these terms.
*Correspondence: Lijing Yang, eWFuZ2xpamluZzIzQDEyNi5jb20=
Disclaimer: All claims expressed in this article are solely those of the authors and do not necessarily represent those of their affiliated organizations, or those of the publisher, the editors and the reviewers. Any product that may be evaluated in this article or claim that may be made by its manufacturer is not guaranteed or endorsed by the publisher.
Research integrity at Frontiers
Learn more about the work of our research integrity team to safeguard the quality of each article we publish.