- Department of Internal Medicine, Erasmus MC, Rotterdam, Netherlands
Bone has long been regarded as a static organ, simply providing protection and support. However, this mindset has changed radically in recent years and bone is becoming increasingly recognized for its endocrine function of secreting several hormones, thereby controlling various physiological pathways. One of the factors released by the skeleton is osteocalcin. Importantly, osteocalcin is secreted solely by osteoblasts but only has minor effects on bone mineralization and density. Instead, it has been reported to control several physiological processes in an endocrine manner, such as glucose homeostasis and exercise capacity, brain development, cognition, and male fertility. The aim of this review is to provide an overview of the currently known roles of osteocalcin and their underlying mechanisms. At present, one of the major goals in this field is translating basic research into therapeutic applications, therefore ongoing efforts to bring these findings to the clinics will also be discussed.
Introduction
Bone is well-known for its established role as connective tissue providing not only support and protection for vital organs but also mobility to the body. These functions are known to be exerted by the three major cell types of the skeleton: osteoblasts, osteoclasts, and osteocytes. Osteoblasts, originating from skeletal stem cells, are responsible for synthesis and secretion of type I collagen to synthesize and maintain the extracellular matrix and are able to control osteoclast differentiation. Beside this thoroughly studied role, a new line of research has emerged in recent years, suggesting that osteoblasts secrete factors possessing hormonal function and are thereby able to control other organs. Based on this, numerous studies have been performed, proposing that bone is an essential endocrine organ controlling a panoply of physiological processes, such as energy metabolism, adipogenesis, neuronal development, muscle growth, and male fertility.
One of the key players in bone endocrinology is osteocalcin, or bone γ-carboxyglutamic acid protein, a factor expressed and secreted solely by osteoblasts (1). Following protein synthesis, the mature peptide first undergoes several splicing events and then gets γ-carboxylated at three residues, resulting in a peptide with high affinity toward bone and the extracellular matrix (2). Due to the low pH inside the osteoclast resorption compartments, however, osteocalcin gets decarboxylated again, which reduces its affinity for bone and triggers the release of uncarboxylated osteocalcin into the circulation (3).
Initially, osteocalcin was hypothesized to act in extracellular matrix mineralization and was used as a serum marker of osteoblastic bone formation (4). However, in the late 90s osteocalcin depletion was found to have minor effects on bone density and mineralization in mice (5). Since then, great efforts have been made to determine the actual function of this protein. Up to now, several roles of osteocalcin have been revealed and current reports, which are mainly based on murine and in vitro studies, suggest that the uncarboxylated form of osteocalcin controls physiological pathways in an endocrine manner.
The purpose of this review is to summarize the currently known key functions of osteocalcin, which are its role in glucose metabolism and adaptation to exercise, neuronal development, male fertility and a very recent hypothesis linking osteocalcin to tumorigenesis (Figure 1). Furthermore, current efforts to verify these observations in humans and to ultimately translate these findings from basic research into the clinic will be discussed.
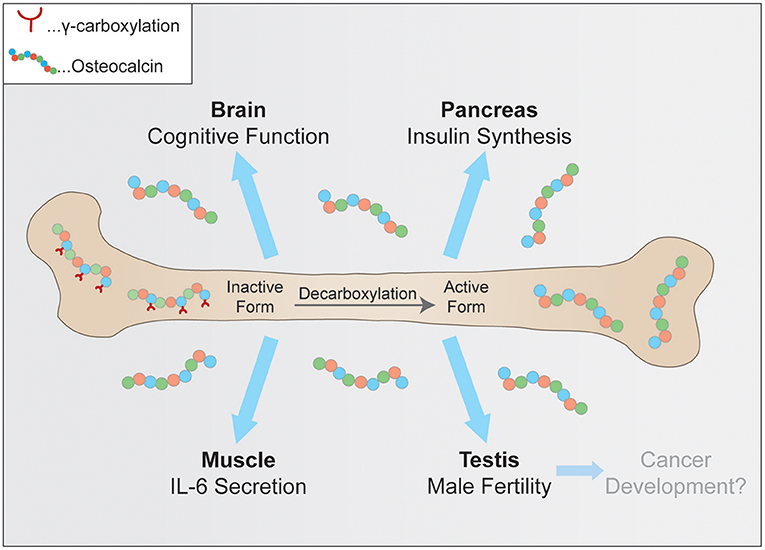
Figure 1. Physiological pathways controlled by osteocalcin. Uncarboxylated osteocalcin is released by osteoclasts and then binds its receptors to influence cognitive function, insulin synthesis in pancreatic β-cells, coinciding with exercise adaptation and testosterone secretion.
Osteocalcin Regulates Insulin Secretion
In mammals, glucose metabolism is known to be the major source of energy generation. For a long time, it could not be explained why a hormone secreted by the skeleton would be involved in this metabolic pathway. However, looking closer at the ongoing processes in bone, such as bone formation during childhood, the repair of fractures upon injury or the constant remodeling of the skeleton in adults, this clearly indicates that bone demands a significant amount of energy to fulfill its functions. The first evidence of osteocalcin regulating glucose homeostasis was provided from the investigation of osteocalcin-deficient mice in a study by Ducy et al. (5). In contrast to the expected phenotype of bone fragility, mice only showed moderately increased bone mass, but also significantly elevated blood glucose and an increased number of adipocytes together with a higher fat mass compared to their wild-type (WT) littermates (6). Following up on this, the Karsenty group showed that secreted, uncarboxylated osteocalcin induces insulin production in pancreatic islets as well as adiponectin expression in adipocytes, both in vitro and in vivo (6–8). Further confirmation of this mechanism was provided by a different group, proposing that deletion of the insulin receptor in osteoblasts mimics the observed phenotypes of osteocalcin deletion (9). These findings support the idea of an endocrine bone-pancreas loop where insulin signaling induces osteocalcin expression in osteoblasts, which further stimulates insulin secretion in pancreatic islet cells (9). This hypothesis is further strengthened by studies reporting that insulin sensitivity and glucose tolerance in mice kept on a high fat diet could be restored by daily injections of osteocalcin (10, 11). Whether this feedback loop is also functional in humans is still poorly understood. Until now, osteocalcin has been shown to stimulate human β-cell proliferation ex vivo (12) and polymorphisms in the human Bglap (osteocalcin) locus have been associated with type 2 diabetes mellitus (T2DM) and obesity (13, 14). Furthermore, several retrospective studies have suggested osteocalcin to negatively correlate with fat mass in several nationalities and age groups (15–19) and to positively correlate with insulin sensitivity and adiponectin levels (20). It is critical to mention though that these studies only investigated subgroups of patients, such as T2DM patients and specific age groups, which may favor the detection of correlations even if relatively weak. Moreover, total levels of osteocalcin are frequently used as a read out in clinical studies, whereas murine studies have clearly shown that it is mainly the uncarboxylated form influencing glucose metabolism. A distinction between these two forms will be critical to detect possible functional relationships. Importantly, confirming the role of osteocalcin during glucose metabolism in humans may have direct therapeutic implications for T2DM patients. All in all, the role of osteocalcin in glucose metabolism is not yet fully supported by human studies due to the above-mentioned pitfalls.
A Role for Osteocalcin in Brain Development and Cognitive Function
Apart from the well-studied role of osteocalcin in energy homeostasis, it was also found to regulate proper brain development and function. Interestingly, osteocalcin-deficient mice were found to be more passive and to suffer from increased anxiety and decreased memory compared to their WT littermates. Also anatomically, the brain, especially the hippocampus, was shown to be smaller and less developed. (21). Osteocalcin was described to regulate the synthesis of neurotransmitters in the brain, such as dopamine, serotonin, or norepinephrine. Importantly, these altered expression levels and behavioral phenotypes could be rescued by injecting mice with uncarboxylated, active osteocalcin but the developmental defects in the brain remained (21). Therefore, osteocalcin-deficient (Ocn−/−) mice were crossed and osteocalcin was supplemented to the mothers, which alleviated the poor neuronal development of the hippocampus. This experiment suggests that osteocalcin is required during embryonic development for proper neuronal development and is not synthesized by the embryo itself but provided by the mother. The fact that osteocalcin is able to rescue memory loss and defective neuronal development also sparked interest in the medical field. Considering the fact that Western society is aging disproportionately and increasing numbers of people are suffering from cognitive decline, osteocalcin may be a promising, novel therapeutic agent to alleviate these symptoms. Interestingly, murine studies are already pointing toward that direction because all symptoms of Ocn−/− mice can be rescued by exogenously providing uncarboxylated osteocalcin (21). Additionally, a recent report proposed that plasma injections from WT mice, as well as mini osmotic pumps delivering osteocalcin over a longer period of time, could rescue the cognitive defects and anxiety-related features in Ocn−/− mice (22). Further mechanistic investigations suggest that the target receptor of osteocalcin in the brain is the G-protein coupled receptor Gpr158. However, this finding should be handled with caution, as Gpr158 is not expressed in all the brain regions where osteocalcin is active, indicating that other receptors and mechanisms are contributing to osteocalcin signaling in the mouse brain. In order to validate this novel role for osteocalcin in humans, several follow-up studies have been performed to find correlations between osteocalcin and cognitive performance in humans. According to current literature, though, a possible correlation in humans is still under debate. On the one hand, reports have been published stating a positive correlation between osteocalcin levels and cognitive performance in aged women (23) and obese patients (24), but on the other hand a recent study in aged individuals with memory concerns (25) could not observe any association with either uncarboxylated or total osteocalcin levels. Considering that the initial finding is very recent, the coming years will bring more studies looking for correlations between osteocalcin levels and cognitive function. Considering this severe effect of osteocalcin on cognitive function, the question arises why a bone-derived hormone may control the brain. Literature indicates that the brain controls bone mass acquisition and that patients suffering from neuropsychiatric disorders often suffer from osteoporosis or low bone mass (26, 27). Furthermore, mice lacking osteocalcin have also been reported to suffer from decreased dopamine and serotonin levels. This suggests that as in glucose metabolism, osteocalcin may function as a feedback signal to the brain to stimulate bone mass acquisition. However, further investigations are required to better investigate this hypothesis. Moreover, larger studies looking at participants differing in age, sex, health status, and ethnic heritage are needed to draw a definite conclusion and will reveal whether osteocalcin is a promising target for aging-related diseases.
Accurate Control of Osteocalcin Levels Is Essential for Male Fertility and Cancer Prevention
Since sex hormones are known to be important regulators of bone strength during several stages of life, such as adolescence or menopause, it seems likely that osteocalcin may also function in a feedback loop in this metabolic process. Active osteocalcin signaling might indicate bone strength and health, which together with many other fitness markers in the body may contribute to fertility. From an evolutionary perspective this would then favor healthy and strong individuals in order to generate offspring. Indeed, Ocn−/− mice were found to have significantly smaller litter sizes when compared to WT animals. By performing co-culture experiments using ovary and testis explants incubated with supernatants of mouse osteoblasts, osteoblasts were found to strongly induce testosterone secretion but not estradiol or progesterone production. To further confirm that it is indeed osteocalcin mediating this effect, testosterone-secreting Leydig cells were incubated with supernatants of WT or Ocn−/− osteoblast cultures. Interestingly, the supernatants lacking osteocalcin were no longer able to induce testosterone secretion. Furthermore, supplementing Leydig cells with uncarboxylated osteocalcin increased testosterone secretion in a dose-dependent manner. Switching to an in vivo model, Oury and colleagues reported that Ocn−/− mice suffer from low testosterone levels and reduced weight of the reproductive organs and oligospermia, which could be rescued by supplementing the mice with exogenous, uncarboxylated osteocalcin (7). In the process of deciphering the molecular mechanism, this study and others identified the receptor required for osteocalcin-mediated testosterone secretion: the G-protein coupled receptor GPRC6A, which was also found to be critical for osteocalcin controlling insulin secretion (7, 8, 28). For further studies, Gprc6a−/− mice were generated and were found to display the same symptoms as Ocn−/− mice, including poor breeding and altered insulin sensitivity. However, no behavioral abnormalities were observed, which corroborates previous reports postulating that osteocalcin signaling in the brain is mediated by GPR158 (22). To further investigate this, two patients carrying a missense mutation in the Gprc6a locus were characterized. Confirming the observations made in mice, these individuals also suffered from testicular failure and showed increased glucose tolerance (29). Importantly, the reason why this effect of osteocalcin on fertility is only observed in males remains elusive. Therefore, it is essential that these findings are reproduced in independently generated mouse models and by other groups to rule out the cause for these sex differences. A query in the current literature underscores the importance of an accurate regulation of osteocalcin and Gprc6a. Low levels of these two factors result in testicular insufficiency but high serum levels of osteocalcin (30–32) as well as overexpression of Gprc6a (33) have been linked to the development of prostate cancer. Furthermore, knocking out Gprc6a using CRISPR/Cas9 technology abolished osteocalcin-mediated activation of ERK, AKT, mTOR signaling and cell proliferation in human prostate cancer xenograft models (34). This further emphasizes the importance of osteocalcin regulation and the fact that targeting GPCR6A may provide a novel strategy to inhibit cancer cell migration. Another role of osteocalcin in lung cancer development was recently shown by Engblom et al. They postulate that osteocalcin expressing cells become activated by factors secreted from lung adenocarcinoma, which recruits neutrophils to the site of the tumor and promotes tumor growth. Interestingly, deletion of cells expressing osteocalcin triggered significant tumor suppression (35). However, the authors do not show a direct effect of osteocalcin on tumor progression, so whether it is osteocalcin itself promoting activation of neutrophils or whether another factor expressed by these cells mediates this process, remains elusive. Nevertheless, these studies provide an interesting basis for further investigations concerning the role of osteocalcin in tumorigenesis and a possibility to use osteocalcin as a biomarker for cancer development.
Osteocalcin Is Required for Optimal Adaptation to Exercise
Since osteocalcin has been reported to regulate glucose metabolism, which provides energy to muscles during exercise, it may be involved in the communication between these two tissues. Initially, osteocalcin levels were found to increase in mice and humans during exercise (36). Furthermore, the Karsenty group demonstrated that osteocalcin levels decline during aging, coinciding with a diminished exercise capacity and a decrease in muscle mass. In order to prove that these phenotypes are caused by osteocalcin, mice were injected with uncarboxylated osteocalcin, which could rescue the exercise capacity of these mice and partially reverse the age-dependent decrease in muscle weight (37). Next, a mouse model specifically lacking the osteocalcin receptor Gprc6a in muscle cells was generated (Gprc6a) to assess whether these findings are caused by a direct effect of osteocalcin on muscle cells. Injections of osteocalcin could not increase the exercise capacity of these animals, indicating that osteocalcin signaling is specifically required in myofibers for exercise adaptation. Furthermore, Mera and colleagues stated that osteocalcin signaling supports glucose and fatty acid utilization during exercise, which is in line with previous reports showing that muscle glucose uptake was increased upon exposure to osteocalcin in vitro (38, 39). Transcriptome analysis of Gprc6a mice revealed that the myokine Interleukin-6 (IL-6), which is secreted by skeletal muscles into the blood upon exercise (40), was found to be significantly downregulated. Therefore, the authors suggested that osteocalcin binds its receptor GPRC6A in muscle cells and is required for ATP generation during exercise by controlling the uptake of glucose and fatty acids. Moreover, a feedforward loop between muscle and bone may exist where IL-6 is secreted from the muscle in order to further stimulate glucose and fatty acid production and the secretion of undercarboxylated osteocalcin. However, further research is needed to elucidate this mechanism of regulation. From a translational point of view, multiple clinical studies already suggest that osteocalcin levels are elevated upon exercise in different gender and age groups (41–44). Furthermore, uncarboxylated osteocalcin levels in women above 70 years have been reported to positively correlate with muscle strength (45). This suggests that osteocalcin and its receptor may be a promising target to combat the age-related decline of muscle strength or to alleviate muscle diseases. Considering that this role of osteocalcin has been reported only very recently, further mechanistic studies are needed to prove that this feedforward loop is also present in humans, and more clinical studies are required to draw definite conclusions about possible therapeutic applications in bone-muscle communication.
Conclusion
The literature presented above provides evidence that osteocalcin is not only involved in bone remodeling but plays a critical function in multiple physiological processes. Importantly, these findings should be handled with care because most initial studies were performed by one laboratory and are still waiting to be reproduced by other research groups. The fact that osteocalcin is solely regulating male fertility and does not affect female reproduction seems especially counterintuitive and requires further research. Moreover, many discoveries are still lacking mechanistic insight and thorough validation in humans. Considering the highly standardized conditions of murine studies and the high genetic similarity of animals, these findings may not accurately reflect the human situation. Furthermore, most clinical studies investigate groups predefined by age, ethnicity, or gender, which may favor the detection of a relationship. It is also worth mentioning that correlation does not imply a direct function for osteocalcin in humans, therefore investigating larger patient cohorts as well as thoroughly executed genetic studies are needed to gain more mechanistic insights. Given the rapidly advancing field of omics technologies, such as genome-wide screening platforms in combination with powerful next-generation sequencing technology and the ability to study cellular processes at single-cell level, it is feasible that new studies will shed light on the role(s) of human osteocalcin soon. Furthermore, these techniques may aid in discovering previously unknown roles of osteocalcin. Considering the strong regulatory effects of osteocalcin on various organs of our body, it is highly likely that other organs or pathways will be affected by osteocalcin signaling as well. Even when assuming that human data correlate well with the observations made in the initial studies, it may still be a laborious journey until clinical trials are initiated, using osteocalcin to treat metabolic diseases such as T2DM or obesity.
Author Contributions
SM performed the literature research, wrote the manuscript, and designed the figure. BvdE revised the manuscript.
Conflict of Interest Statement
The authors declare that the research was conducted in the absence of any commercial or financial relationships that could be construed as a potential conflict of interest.
References
1. Hauschka PV, Lian JB, Cole DE, Gundberg CM. Osteocalcin and matrix Gla protein: vitamin K-dependent proteins in bone. Physiol Rev. (1989) 69:990–1047. doi: 10.1152/physrev.1989.69.3.990
2. Poser JW, Esch FS, Ling NC, Price PA. Isolation and sequence of the vitamin K-dependent protein from human bone. Undercarboxylation of the first glutamic acid residue. J Biol Chem. (1980) 255:8685–91. doi: 10.1006/bbrc.1995.1918
3. Malashkevich VN, Almo SC, Dowd TL. X-ray crystal structure of bovine 3 glu-osteocalcin. Biochemistry (2013) 52:8387–92. doi: 10.1021/bi4010254
4. Delmas PD, Eastell R, Garnero P, Seibel MJ, Stepan J Committee of Scientific Advisors of the International Osteoporosis Foundation. The use of biochemical markers of bone turnover in osteoporosis. Committee of Scientific Advisors of the International Osteoporosis Foundation. Osteoporos Int. (2000) 11(Suppl. 6):S2–17. doi: 10.1007/s001980070002
5. Ducy P, Desbois C, Boyce B, Pinero G, Story B, Dunstan C, et al. Increased bone formation in osteocalcin-deficient mice. Nature (1996) 382:448–52. doi: 10.1038/382448a0
6. Lee NK, Sowa H, Hinoi E, Ferron M, Ahn JD, Confavreux C, et al. Endocrine regulation of energy metabolism by the skeleton. Cell (2007) 130:456–69. doi: 10.1016/j.cell.2007.05.047
7. Oury F, Sumara G, Sumara O, Ferron M, Chang H, Smith CE, et al. Endocrine regulation of male fertility by the skeleton. Cell (2011) 144:796–809. doi: 10.1016/j.cell.2011.02.004
8. Wei J, Hanna T, Suda N, Karsenty G, Ducy P. Osteocalcin promotes β-cell proliferation during development and adulthood through Gprc6a. Diabetes (2014) 63:1021–31. doi: 10.2337/db13-0887
9. Fulzele K, Riddle RC, DiGirolamo DJ, Cao X, Wan C, Chen D, et al. Insulin receptor signaling in osteoblasts regulates postnatal bone acquisition and body composition. Cell (2010) 142:309–19. doi: 10.1016/j.cell.2010.06.002
10. Ferron M, McKee MD, Levine RL, Ducy P, Karsenty G. Intermittent injections of osteocalcin improve glucose metabolism and prevent type 2 diabetes in mice. Bone (2012) 50:568–75. doi: 10.1016/j.bone.2011.04.017
11. Huang L, Yang L, Luo L, Wu P, Yan S. Osteocalcin improves metabolic profiles, body composition and arterial stiffening in an induced diabetic rat model. Exp Clin Endocrinol Diab. (2017) 125:234–40. doi: 10.1055/s-0042-122138
12. Sabek OM, Nishimoto SK, Fraga D, Tejpal N, Ricordi C, Gaber AO. Osteocalcin effect on human β-cells mass and function. Endocrinology (2015) 156:3137–46. doi: 10.1210/EN.2015-1143
13. Xu H, Xiao W, Luo D, Liu YM, Zou L, Kuang H -bin. Association analysis of genetic polymorphisms and potential interaction of the osteocalcin (BGP) and ER-α genes with body mass index (BMI) in premenopausal Chinese women. Acta Pharmacol Sin. (2010) 31:455–60. doi: 10.1038/aps.2010.1
14. Korostishevsky M, Malkin I, Trofimov S, Pei Y, Deng HW, Livshits G. Significant association between body composition phenotypes and the osteocalcin genomic region in normative human population. Bone (2012) 51:688–94. doi: 10.1016/j.bone.2012.07.010
15. Chin KY, Ima-Nirwana S, Mohamed IN, Ahmad F, Mohd Ramli ES, Aminuddin A, et al. Serum osteocalcin is significantly related to indices of obesity and lipid profile in Malaysian men. Int J Med Sci. (2014) 11:151–7. doi: 10.7150/ijms.7152
16. Reinehr T, Roth CL. A new link between skeleton, obesity and insulin resistance: relationships between osteocalcin, leptin and insulin resistance in obese children before and after weight loss. Int J Obes. (2010) 34:852–8. doi: 10.1038/ijo.2009.282
17. Sarkar PD, Choudhury AB. Relationships between serum osteocalcin levels versus blood glucose, insulin resistance and markers of systemic inflammation in central Indian type 2 diabetic patients. Eur Rev Med Pharmacol Sci. (2013) 17:1631–5.
18. Wang J-W, Tang Q-Y, Ruan H-J, Cai W. Relation between serum osteocalcin levels and body composition in obese children. J Pediatr Gastroenterol Nutr. (2014) 58:729–32. doi: 10.1097/MPG.0000000000000243
19. Wang Q, Zhang B, Xu Y, Xu H, Zhang N. The relationship between serum osteocalcin concentration and glucose metabolism in patients with Type 2 diabetes mellitus. Int J Endocrinol. (2013) 2013:1–7. doi: 10.1155/2013/842598
20. Fernández-Real JM, Izquierdo M, Ortega F, Gorostiaga E, Gómez-Ambrosi J, Moreno-Navarrete JM, et al. The relationship of serum osteocalcin concentration to insulin secretion, sensitivity, and disposal with hypocaloric diet and resistance training. J Clin Endocrinol Metab. (2009) 94:237–45. doi: 10.1210/jc.2008-0270
21. Oury F, Khrimian L, Denny CA, Gardin A, Chamouni A, Goeden N, et al. Maternal and offspring pools of osteocalcin influence brain development and functions. Cell (2013) 155:228–41. doi: 10.1016/j.cell.2013.08.042
22. Khrimian L, Obri A, Ramos-Brossier M, Rousseaud A, Moriceau S, Nicot A-S, et al. Gpr158 mediates osteocalcin's regulation of cognition. J Exp Med. (2017) 214:2859–73. doi: 10.1084/jem.20171320
23. Bradburn S, McPhee JS, Bagley L, Sipila S, Stenroth L, Narici MV, et al. Association between osteocalcin and cognitive performance in healthy older adults. Age Ageing (2016) 45:844–9. doi: 10.1093/ageing/afw137
24. Puig J, Blasco G, Daunis-I-Estadella J, Moreno M, Molina X, Alberich-Bayarri A, et al. Lower serum osteocalcin concentrations are associated with brain microstructural changes and worse cognitive performance. Clin Endocrinol. (2016) 84:756–63. doi: 10.1111/cen.12954
25. Ross RD, Shah RC, Leurgans S, Bottligieri T, Wilson RS, Sumner DR. Circulating Dkk1 and TRAIL are associated with cognitive decline in community-dwelling, older adults with cognitive concerns. J Gerontol Ser A (2018) 3:1688–94. doi: 10.1093/gerona/glx252
26. Yirmiya R, Bab I. Major depression is a risk factor for low bone mineral density: a meta-analysis. Biol Psychiatry (2009) 66:423–32. doi: 10.1016/j.biopsych.2009.03.016
27. Schweiger U, Weber B, Deuschle M, Heuser I. Lumbar bone mineral density in patients with major depression: evidence of increased bone loss at follow-up. Am J Psychiatry (2000) 157:118–120. doi: 10.1176/ajp.157.1.118
28. Pi M, Wu Y, Quarles LD. GPRC6A mediates responses to osteocalcin in β-cells in vitro and pancreas in vivo. J Bone Miner Res. (2011) 26:1680–3. doi: 10.1002/jbmr.390
29. Oury F, Ferron M, Huizhen W, Confavreux C, Xu L, Lacombe J, et al. Osteocalcin regulates murine and human fertility through a pancreas-bone-testis axis. J Clin Invest. (2013) 123:2421–33. doi: 10.1172/JCI65952
30. Nimptsch K, Rohrmann S, Nieters A, Linseisen J. Serum undercarboxylated osteocalcin as biomarker of vitamin K intake and risk of prostate cancer: a nested case-control study in the Heidelberg cohort of the European prospective investigation into cancer and nutrition. Cancer Epidemiol Biomarkers Prev. (2009) 18:49–56. doi: 10.1158/1055-9965.EPI-08-0554
31. Hayashi Y, Kawakubo-Yasukochi T, Mizokami A, Takeuchi H, Nakamura S, Hirata M. Differential roles of carboxylated and uncarboxylated osteocalcin in prostate cancer growth. J Cancer (2016) 7:1605–9. doi: 10.7150/jca.15523
32. Gardner TA, Lee S-J, Lee S-D, Li X, Shirakawa T, Kwon DD, et al. Differential expression of osteocalcin during the metastatic progression of prostate cancer. Oncol Rep. (2009) 21:903–8. doi: 10.3892/or_00000302
33. Pi M, Quarles LD. GPRC6A regulates prostate cancer progression. Prostate (2012) 72:399–409. doi: 10.1002/pros.21442
34. Ye R, Pi M, Cox JV, Nishimoto SK, Quarles LD. CRISPR/Cas9 targeting of GPRC6A suppresses prostate cancer tumorigenesis in a human xenograft model. J Exp Clin Cancer Res. (2017) 36:1–13. doi: 10.1186/s13046-017-0561-x
35. Engblom C, Pfirschke C, Zilionis R, Da Silva Martins J, Bos SA, Courties G, et al. Osteoblasts remotely supply lung tumors with cancer-promoting SiglecF high neutrophils. Science (2017) 358:eaal5081. doi: 10.1126/science.aal5081
36. Mera P, Laue K, Ferron M, Confavreux C, Wei J, Galán-Díez M, et al. Osteocalcin signaling in myofibers is necessary and sufficient for optimum adaptation to exercise. Cell Metab. (2016) 23:1078–92. doi: 10.1016/j.cmet.2016.05.004
37. Mera P, Laue K, Wei J, Berger JM, Karsenty G. Osteocalcin is necessary and sufficient to maintain muscle mass in older mice. Mol Metab. (2016) 5:1042–7. doi: 10.1016/j.molmet.2016.07.002
38. Lin X, Parker L, McLennan E, Zhang X, Hayes A, McConell G, et al. Recombinant uncarboxylated osteocalcin per se enhances mouse skeletal muscle glucose uptake in both extensor digitorum longus and soleus muscles. Front Endocrinol. (2017) 8:330. doi: 10.3389/fendo.2017.00330
39. Tsuka S, Aonuma F, Higashi S, Ohsumi T, Nagano K, Mizokami A, et al. Promotion of insulin-induced glucose uptake in C2C12 myotubes by osteocalcin. Biochem Biophys Res Commun. (2015) 459:437–42. doi: 10.1016/j.bbrc.2015.02.123
40. Steensberg A, Van Hall G, Osada T, Sacchetti M, Saltin B, Pedersen BK. Production of interleukin-6 in contracting human skeletal muscles can account for the exercise-induced increase in plasma interleukin-6. J Physiol. (2000) 529:237–42. doi: 10.1111/j.1469-7793.2000.00237.x
41. Kim Y-S, Nam JS, Yeo D-W, Kim KR, Suh S-H, Ahn CW. The effects of aerobic exercise training on serum osteocalcin, adipocytokines and insulin resistance on obese young males. Clin Endocrinol. (2015) 82:686–94. doi: 10.1111/cen.12601
42. Ahn N, Kim K. Effects of 12-week exercise training on osteocalcin, high-sensitivity C-reactive protein concentrations, and insulin resistance in elderly females with osteoporosis. J Phys Ther Sci. (2016) 28:2227–31. doi: 10.1589/jpts.28.2227
43. Jürimäe J, Rämson R, Mäestu J, Jürimäe T, Arciero PJ, Braun WA, et al. Interactions between adipose, bone, and muscle tissue markers during acute negative energy balance in male rowers. J Sports Med Phys Fitness (2011) 51:347–54.
44. Lin C-F, Huang T, Tu K-C, Lin LL, Tu Y-H, Yang R-S. Acute effects of plyometric jumping and intermittent running on serum bone markers in young males. Eur J Appl Physiol. (2012) 112:1475–84. doi: 10.1007/s00421-011-2108-8
Keywords: osteocalcin, osteoblasts, bone, energy metabolism, exercise, cognition, fertility, endocrine regulation
Citation: Moser SC and van der Eerden BCJ (2019) Osteocalcin—A Versatile Bone-Derived Hormone. Front. Endocrinol. 9:794. doi: 10.3389/fendo.2018.00794
Received: 11 September 2018; Accepted: 18 December 2018;
Published: 10 January 2019.
Edited by:
Gudrun Stenbeck, Brunel University London, United KingdomReviewed by:
Graziana Colaianni, Università degli Studi di Bari, ItalyColin Farquharson, University of Edinburgh, United Kingdom
Copyright © 2019 Moser and van der Eerden. This is an open-access article distributed under the terms of the Creative Commons Attribution License (CC BY). The use, distribution or reproduction in other forums is permitted, provided the original author(s) and the copyright owner(s) are credited and that the original publication in this journal is cited, in accordance with accepted academic practice. No use, distribution or reproduction is permitted which does not comply with these terms.
*Correspondence: Bram C. J. van der Eerden, b.vandereerden@erasmusmc.nl