- 1Department of Endocrinology, Zhongnan Hospital of Wuhan University, Wuhan, China
- 2Department of Endocrinology, Puren Hospital, Wuhan University of Science and Technology, Wuhan, China
- 3Department of Critical Care Medicine, Zhongnan Hospital, Wuhan University, Wuhan, China
Activation of thioredoxin-interacting protein (TXNIP)/nod-like receptor protein 3 (NLRP3) inflammasome plays a critical role in pathogenesis of non-alcoholic fatty liver disease. This study investigated the protective effects of verapamil on hepatic metaflammation in a rodent model of high-fat (HF) diet-induced obesity (DIO). DIO was induced in a subset of mice provided with HF diet (45% kcal fat). After 10 weeks of HF diet, verapamil was administered by intraperitoneal injection. The experimental groups included the following: (1) normal diet group, (2) normal diet + treatment with verapamil (VER) group, (3) HF control group, (4) HF+VER (25 mg/kg/day) group. After 1 week of each treatment, blood and liver tissues were collected, and glucose control, serum triglyceride (TG) level, inflammation, and TXNIP/NLRP3 inflammasome were analyzed. Verapamil administration caused no alteration in food intake. HF diet impaired glucose control and increased body weight and serum TG levels. Hepatic inflammation was aggravated in HF-fed mice, as demonstrated by increased levels of pro-inflammatory markers interleukin-1β (IL-1β) and IL-18 in the liver. On the other hand, verapamil administration significantly improved glucose control, body weight, and serum TG levels. Verapamil treatment also reduced pro-inflammatory marker levels. These improvements were accompanied by alterations in activation of TXNIP/NLRP3 inflammasome. The observed results demonstrate that verapamil ameliorates hepatic metaflammation by inhibiting TXNIP/NLRP3 pathways.
Introduction
Non-alcoholic fatty liver disease (NAFLD) and non-alcoholic steatohepatitis (NASH) are often accompanied by systemic and hepatic metaflammation (1); these conditions are some of the most common chronic liver illnesses and occur in 20% of the general population worldwide (2). Several therapeutic approaches to NAFLD have been suggested; these approaches include weight reduction (3), the use of phytonutrients (4), and pharmacological intervention (5). However, the underlying mechanisms have not been elucidated.
Inflammation is regarded a central component in NAFLD pathogenesis (6). Interleukin-1 (IL-1) cytokine family includes various inflammatory cytokines, such as IL-1β and IL-18, which are crucially involved in NAFLD pathogenesis (7, 8). Maturation and secretion of IL-1β and IL-18 mainly depend on inflammasome activation (9). Nod-like receptor protein 3 (NLRP3) inflammasome is the most extensively studied inflammasome, which comprises NLRP3, apoptosis-associated speck-like protein (ASC) and caspase-1 (Casp-1) (10). NLRP3 inflammasome activation is closely related to NAFLD development (11). Thioredoxin-interacting protein (TXNIP) is linked to NLRP3 inflammasome activation (12). Studies indicated that the TXNIP/NLRP3 inflammasome pathway is involved in NAFLD pathogenesis (13, 14). Inhibitors of TXNIP/NLRP3 inflammasome functions may therefore attenuate NAFLD.
Verapamil, a calcium channel blocker, improves hyperglycaemia and insulin resistance in metabolism syndromes (15). Shalev et al. showed that verapamil inhibits TXNIP expression in β cell and thus promotes β-cell survival and function (16). Park et al. claimed that verapamil reduces hepatic inflammation and improves metabolic homeostasis in NAFLD (17), but the related mechanism remains unknown. Therefore, we hypothesize that verapamil exerts a protective effect against high-fat (HF) diet-induced NAFLD by inhibiting TXNIP/NLRP3 pathways.
Materials and Methods
Experimental Animals
Male C57BL/6 mice (7 weeks old) were purchased from the Center for Disease Control and Prevention, Hubei, China. The mice were housed in a light- and temperature- controlled room (21–23°C; 12 h cycle) supplied with food and water as needed. HF diet feed with 45% of energy from lipids was purchased from Mediscience, Ltd. (Yangzhou, China). The Animal Care and Use Committee of the Wuhan University of Science and Technology approved all protocols (No. 02515065V). All animals received humane care according to the Principles of Laboratory Animal Care.
Reagents
Verapamil was purchased from Shanghai Harvest Pharmaceutical Co., Ltd (Shanghai, China; No. H31021343).Antibodies against TXNIP, ASC and Casp-1 were purchased from Proteintech (Chicago, USA). NLRP3 antibody was purchased from R&D Systems (Minneapolis, MN, USA). Enzyme-linked immunosorbent assay (ELISA) kits for measurement of IL-1β, IL-18, and insulin contents in mice were purchased from Elabscience Biotechnology Co., Ltd (Wuhan, China). Biochemical parameter assay kits were purchased from Nanjing Jiancheng Biotechnology Co. Ltd (Nanjing, China).
Experiment Protocols
After 1 week of acclimatization, the animals were randomly divided into four groups, namely, normal diet (ND) group; normal diet + verapamil treatment (ND+VER) group; HF diet (HFD) group; and HF diet + verapamil treatment (HFD+VER) group, with ten mice in each group. After providing the mice with normal or HF diet for 10 weeks, verapamil (25 mg/kg/d) was intraperitoneally injected to the ND+VER and HFD+VER groups daily (17) for 1 week. Normal saline was administered to ND and HFD groups in the same manner. Mice were weighed weekly. At the end of the experimental period, the animals were fasted overnight and sacrificed under anesthetic conditions. Blood samples were rapidly obtained by eye removal. Livers were removed and weighed. Hepatic tissues were immediately snap-frozen in liquid nitrogen and stored at −80°C for further analysis.
Measurement of Serum Content Levels and Cytokine Assays
Blood samples were centrifuged at 4°C (2,000 r, 20 min) and measured for content levels of the following biochemical properties: glucose, triglyceride (TG), total cholesterol (TC), alanine aminotransferase (ALT), and aspartate aminotransferase (AST) by using different biochemical assay kits. IL-1β, IL-18, and insulin levels were measured by ELISA using ELISA kits. Homeostatic model assessment for insulin resistance (HOMA-IR) was calculated as follows: fasting insulin (μU/ml) × fasting glucose (mmol/l)/22.5.
Histological Analysis of Liver and Immunohistochemistry
Hepatic tissue samples were fixed with 10% formalin solution and embedded in paraffin. Paraffin-embedded samples were sectioned at 5 μm thickness and stained with haematoxylin–eosin (H&E). NAFLD activity score (NAS) was determined through steatosis, lobular inflammation and ballooning degeneration (18). To examine hepatic lipid deposition, frozen sections of liver tissues were stained with 0.5% oil red O reagent for 10 min and subsequently washed with isopropanol. Sections were counterstained with haematoxylin.
Analysis of mRNA Expression
Total RNA from hepatic tissues was extracted using Trizol reagent (Beyotime Biotechnology, Shanghai, China) according to manufacturer's instructions. Quality and purity of RNA were determined by U.V. spectrophotometry at 260 and 280 nm, respectively. β-Actin expression was used as endogenous control. Quantitative real-time polymerase chain reaction(RTPCR) was performed with an input of cDNA converted from 4 μg of total RNA. Primer sequences of mice mRNA are as follows: forward 5′-GATACCCCAGAAGCTCCTCC-3′, reverse5′-ACCTCAGTGTAAGTGGGTGG-3′ for TXNIP gene; forward 5′-CTCGCATTGGTTCTGAGCTC-3′, reverse5′-AGTAAGGCCGGAATTCACCA-3′ forNLRP3 gene; forward 5′-CTATCTGGAGTCGTATGGCTTGG-3′, reverse 5′-ATGAGTGCTTGCCTGTGCTGGTC-3′ forASC gene; forward 5′-GGCAAGCCAAATCTTTATCAC-3′, reverse 5′-GCCATCTTCTTTGTTCTGTTC-3′ for Casp-1 gene; and forward 5′-CACGATGGAGGGGCCGGACTCATC-3′, reverse5′-TAAAGACCTCTATGCCAACACAGT-3′ for β-actin gene. mRNA levels were evaluated by quantitative RT PCR using an ABI 7500 Fast Real-time PCR system with SYBR Green detection function(Bio, Inc.). Quantitative measurements were obtained using 2−ΔΔCt method. All samples were measured in triplicate, and mean values were considered for comparative analysis.
Western Blot Analyses
Liver tissues were harvested, and protein extracts were prepared according to established methods (19). The homogenates were centrifuged at 14,000 rpm for 5 min, and the supernatant nuclear extracts were then harvested and stored at −70°C. The extracted proteins were quantified by Lowry-Kalckar assays (20). Equal amounts of proteins were then separated by 10% sodium dodecyl sulfate polyacrylamide gel and then transferred to a polyvinylidene difluoride membrane. The membrane was incubated with primary antibodies at 4°C overnight and with secondary antibodies at room temperature for 2 h. Signals were detected by chemiluminescence method, and band intensities were analyzed by Quantity One Software (Bio-Rad Laboratories). Mean area density was expressed for target proteins relative to β-actin expression.
Statistical Analysis
Data are presented as mean ± standard deviation (SD). Measurement data were processed through Two-way analysis of variance. A Bonferroni post-hoc multiple comparison test was used to assess significant differences between groups. P < 0.05 indicates a significant difference.
Results
Body Weights, Liver Weights, and Food Intake
At the end of the experiment, mice in the HFD group presented significantly higher average body weight than those of the ND group (**p < 0.01). Verapamil treatment reduced the body weight of HF-fed mice (#p < 0.01) compared with those treated with HF diet. No changes were observed in mice body weight in the ND+VER group compared with that of the ND group. Verapamil treatment showed no effect on food intake in HF diet-fed mice. Liver weights increased significantly in HFD group, compared to that of ND group (**p < 0.01). Verapamil treatment reduced the liver weight of HF diet-fed mice (##p < 0.01; Table 1).
Verapamil Improves Hepatic Steatosis and Insulin Resistance in HF Diet-Fed Mice
High TG and TC concentrations in the serum were observed in HF-fed mice (**p < 0.01), but these levels decreased significantly after verapamil administration (#p < 0.01, ##p < 0.01, Table 2). Hepatic steatosis induced by HF diet was evidently ameliorated by verapamil, as indicated by normal levels of lipid accumulation and regular morphology (size and shape) of liver sections obtained from HFD+VER mice (Figure 1 and Table 3). Reduced levels of serum ALT and AST after verapamil administration supported hepatic and histological analysis results (Table 2).
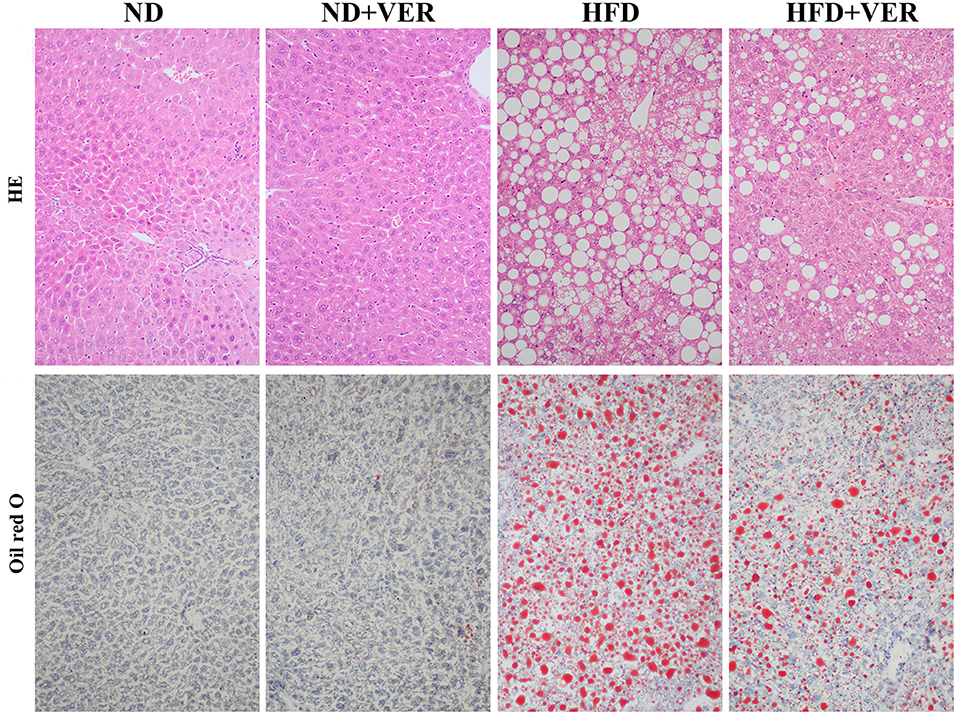
Figure 1. Verapamil ameliorates hepatic steatosis induced by HF diet treatment. Liver sections (n = 3) from each experimental group were processed for histological evaluation. Representative photographs of liver sections with H&E staining (200x) and oil red O staining (400x). ND, normal diet; VER, verapamil; HFD, high-fat diet.
Levels of glucose and insulin levels and HOMA-IR index under fasting condition were measured to detect insulin resistance. The HFD group exhibited higher levels of serum glucose and insulin and HOMA-IR index than the ND group (**p < 0.01). On the other hand, levels of serum glucose and insulin and HOMA-IR index in the HFD+VER group significantly decreased compared with those of the HFD group(##p < 0.01).
Verapamil Inhibits Activation of NLRP3 Inflammasome and Hepatic Metaflammation in HF Diet-Fed Mice
Components of the NLRP3 inflammasome complex and proinflammatory markers were analyzed in livers to test whether NPRP3 inflammasome and related hepatic metaflammation participate in verapamil-mediated improvements in hepatic steatosis and insulin resistance. HF diet activated hepatic NLRP3, ASC and Casp-1 in livers of HF diet-fed mice (Figures 2A–D). Activation of NLRP3 inflammasome resulted in upregulated IL-1β levels in the HFD group (Figure 2E); these results were accompanied by high levels of pro-inflammatory cytokine IL-18 (Figure 2F). One week of verapamil administration inhibited expression of NLRP3 inflammasome components, IL-1β and IL-18, in the livers of HF diet-fed mice (Figure 2).
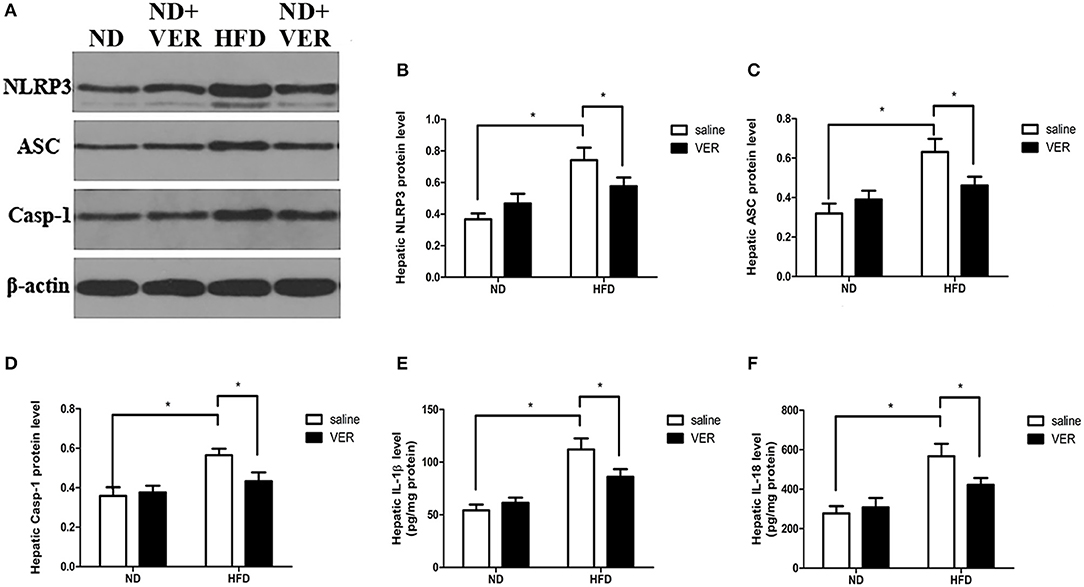
Figure 2. Effects of verapamil on protein expression of NOD-like receptor family, pyrin domain containing 3 (NLRP3) inflammasome complex in livers of HF diet-fed mice. (A) Representative Western blots for NLRP3, ASC, Casp-1 and β-actin. Densitometric analysis of (B) NLRP3, (C) ASC, and (D) Casp-1. Protein expression of each target was normalized to that of β-actin. Similar results were obtained among three independent experiments.The result of one of the experiments is shown. (E) IL-1β and (F) IL-18 concentrations in livers. The values were presented as means ± SD (n = 10 per group, *p < 0.05). ND, normal diet; VER, verapamil; HFD, high-fat diet.
Verapamil Inhibits TXNIP Expression in HF Diet-Fed Mice
We examined the effects of verapamil on TXNIP expression to investigate the mechanisms of NAFLD prevention by verapamil. Figure 3 shows that TXNIP expression was upregulated at protein and mRNA levels in HF-fed mice but was suppressed by verapamil treatment.
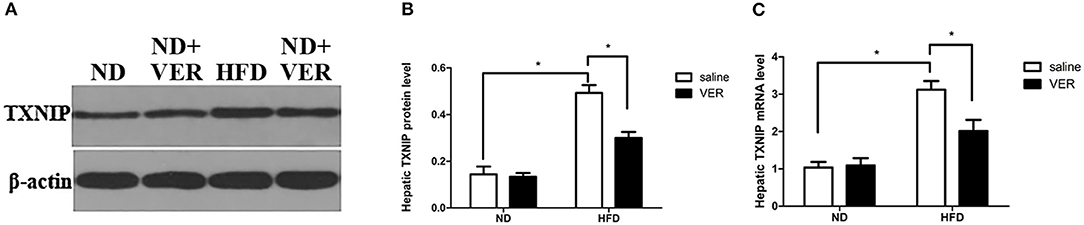
Figure 3. Effects of verapamil on TXNIP expression in the liver of HF diet-fed mice. (A) Representative Western blots for TXNIP and β-actin. Densitometric analysis of (B) TXNIP. Protein expression of the target was normalized to that of β-actin. Similar results were obtained among three independent experiments.The result of one of the experiments is shown. (C) TXNIP mRNA expression as measured by quantitative RTPCR, bars represent changes in means ± SD (n = 10 per group, *p < 0.05). ND, normal diet; VER, verapamil; HFD, high-fat diet.
Discussion
NAFLD is characterized by hepatic lipid accumulation and inflammation (21). In the present study, we observed the effects of verapamil on HF diet-induced NAFLD in mice. Verapamil treatment reduced serum glucose levels and improved insulin resistance, liver function, dyslipidaemia, hepatic steatosis, and inflammation. Histological analysis further revealed that verapamil significantly attenuated hepatic lipid accumulation and inflammation. However, verapamil did not have any effect on normal diet mice.
Xu et al. (22) investigated the effect of verapamil on liver fibrosis induced by multiple hepatotoxic factors in male Wistar rats with different dosages- at doses of 20, 40, and 80 mg.kg−1 daily for 4 weeks. It indicated that the effect was dose-dependent. A subsequent study (23) showed that verapamil was administrated as a sublethal dose (60 mg.kg−1) on Wistar-Albino rats for cancer therapy, but only caused miscarriage on the third day. Therefore we chose verapamil dose 25 mg.kg−1 as our experimental dose according to the recent research (17). We also used 25 and 10 mg.kg−1 in our preliminary experiment and found that the administration of 10 mg.kg−1 verapamil did not have protective effect on hepatic metaflammation while 25 mg.kg−1 verapamil could, so we finally chose the dose of 25 mg.kg−1.
Excessive proinflammatory cytokine-induced inflammation may play a critical role in NAFLD pathogenesis (24). Previous studies have shown that patients with NAFLD may exhibit increased IL-1β levels (25, 26). IL-1β contributed to metabolism disorder, liver injury and steatosis in animal experiments (27). Inhibition of IL-1β expression attenuates severity of hyperglycaemia in obesity (28). IL-18 levels are also associated with insulin resistance, glucolipid metabolism, and obesity (29, 30). Inhibition of IL-18 expression attenuates NAFLD development (31). In the present study, verapamil administration significantly reduced production of IL-1β and IL-18 in the HFD group. We thus recognize that protective effects of verapamil on HF diet-induced NAFLD may be ascribed to reduction of IL-1β and IL-18 levels.
The NLRP3 inflammasome pathway is a primary intracellular multiprotein inflammatory pathway of the innate immune system; this pathway responds to different exogenous and endogenous stimuli. NLRP3 inflammasome forms through activation of NLRP3 and recruitment of ASC and pro-Casp-1 (32). Such phenomena result in Casp-1 activation and subsequent processing of pro-IL-1β and pro-IL-18 to attain their active forms (33). Hepatic lipid accumulation was significantly reduced in HF diet-induced NAFLD of NLRP3−/− mice (34). ASC is a crucial adaptor protein required for Casp-1 recruitment to the NLRP3 platform of inflammasome; such a process is important for activation of Casp-1and IL-1β (10). Hepatic steatosis and injury of liver function increased in Casp1(−/−) mice on HF diet compared with their wild-type counterparts (35). In the present study, verapamil treatment significantly inhibited NLRP3 inflammasome protein expression, implying that the protective effects of verapamil on HF diet-induced NAFLD may be attributed to its influence on suppression of NLRP3 inflammasome activation.
TXNIP regulates cellular oxidative stress, which has been associated with activation of NLRP3 inflammasome (36). As a result of NLRP3 inflammasome activation, TXNIP upregulation caused hepatocellular secretion of IL-1β and IL-18, thereby initiating a hepatocyte-driven sterile immune response on fructose exposure and possibly driving NASH progression (37). Xiao et al. demonstrated that inhibition of the TXNIP–NLRP3 inflammasome pathway may attenuate NAFLD progression (38). The present study demonstrated that verapamil inhibited TXNIP expression in the liver of mice with NAFLD, suggesting that the protective effects of verapamil may be due to inhibition of TXNIP/NLRP3 pathways.
Verapamil relieves hepatosteatosis of obese mice by reducing hepatic TNF- α and IL-6 levels (17), this effect may be correlated with down-regulating NF-kB activation and regulating PRMT1 and PGC-1 α (39). In our future studies, we may investigate how verapamil affects TNF- α, IL-6, NF-kB, PGC-1 α, and its underlying mechanisms.
In conclusion, verapamil treatment attenuates HF diet-induced NAFLD. The mechanisms behind the beneficial effect of verapamil are correlated with inhibition of TXNIP/NARP3 pathways, thereby remarkably reducing the expressions of proinflammatory cytokines IL-1β and IL-18. The obtained data suggest that verapamil can be a potential treatment for HF diet-induced NAFLD.
Author Contributions
FZ, YZ, JC, and YH designed the study. FZ, YZ, JC, YH, and YX conducted the experiments and wrote and revised the manuscript. YX and FZ also contributed to the design. All authors approved the final version to be published.
Funding
This work was financially supported by the National Natural Science Foundation of China (NO.81571946).
Conflict of Interest Statement
The authors declare that the research was conducted in the absence of any commercial or financial relationships that could be construed as a potential conflict of interest.
The reviewer GL and handling Editor declared their shared affiliation.
References
1. Asrih M, Jornayvaz FR. Inflammation as a potential link between nonalcoholic fatty liver disease and insulin resistance. J Endocrinol. (2013) 218:R25–36. doi: 10.1530/JOE-13-0201
2. Chalasani N, Younossi Z, Lavine JE, Diehl AM, Brunt EM, Cusi K, et al. The diagnosis and management of non-alcoholic fatty liver disease: practice Guideline by the American Association for the Study of Liver Diseases, American College of Gastroenterology, and the American Gastroenterological Association. Hepatology (2012) 55:2005–23. doi: 10.1002/hep.25762
3. Dowman JK, Armstrong MJ, Tomlinson JW, Newsome PN. Current therapeutic strategies in non-alcoholic fatty liver disease. Diabetes Obes Metab. (2011) 13:692–702. doi: 10.1111/j.1463-1326.2011.01403.x
4. Xiao J, Ho CT, Liong EC, Nanji AA, Leung TM, Lau TY, et al. Epigallocatechin gallate attenuates fibrosis, oxidative stress, and inflammation in non-alcoholic fatty liver disease rat model through TGF/SMAD, PI3 K/Akt/FoxO1, and NF-kappa B pathways. Eur J Nutr. (2014) 53:187–99. doi: 10.1007/s00394-013-0516-8
5. Yang SJ, Lim Y. Resveratrol ameliorates hepatic metaflammation and inhibits NLRP3 inflammasome activation. Metabolism (2014) 63:693–701. doi: 10.1016/j.metabol.2014.02.003
6. Peverill W, Powell LW, Skoien R. Evolving concepts in the pathogenesis of NASH: beyond steatosis and inflammation. Int J Mol Sci. (2014) 15:8591–638. doi: 10.3390/ijms15058591
7. Tilg H, Moschen AR, Szabo G. Interleukin-1 and inflammasomes in ALD/AAH and NAFLD/NASH. Hepatology (2016) 64:955–65. doi: 10.1002/hep.28456
8. Xiao J, Tipoe GL. Inflammasomes in non-alcoholic fatty liver disease. Front Biosci. (2016) 21:683–95. doi: 10.2741/4414
9. Schroder K, Tschopp J. The inflammasomes. Cell (2010) 140:821–32. doi: 10.1016/j.cell.2010.01.040
10. Schroder K, Zhou R, Tschopp J. The NLRP3 inflammasome: a sensor for metabolic danger? Science (2010) 327:296–300. doi: 10.1126/science.1184003
11. Szabo G, Csak T. Inflammasomes in liver diseases. J Hepatol. (2012) 57:642–54. doi: 10.1016/j.jhep.2012.03.035
12. Zhou R, Tardivel A, Thorens B, Choi I, Tschopp J. Thioredoxin-interacting protein links oxidative stress to inflammasome activation. Nat Immunol. (2010) 11:136–40. doi: 10.1038/ni.1831
13. Wang W, Wang C, Ding XQ, Pan Y, Gu TT, Wang MX, et al. Quercetin and allopurinol reduce liver thioredoxin-interacting protein to alleviate inflammation and lipid accumulation in diabetic rats. Br J Pharmacol. (2013) 169:1352–71. doi: 10.1111/bph.12226
14. Xiao J, Liu Y, Xing F, Leung TM, Liong EC, Tipoe GL. Bee's honey attenuates non-alcoholic steatohepatitis-induced hepatic injury through the regulation of thioredoxin-interacting protein-NLRP3 inflammasome pathway. Eur J Nutr. (2016) 55:1465–77. doi: 10.1007/s00394-015-0964-4
15. Fukao K, Shimada K, Hiki M, Kiyanagi T, Hirose K, Kume A, et al. Effects of calcium channel blockers on glucose tolerance, inflammatory state, and circulating progenitor cells in non-diabetic patients with essential hypertension: a comparative study between azelnidipine and amlodipine on glucose tolerance and endothelial function–a crossover trial (AGENT). Cardiovasc Diabetol. (2011) 10:79. doi: 10.1186/1475-2840-10-79
16. Xu G, Chen J, Jing G, Shalev A. Preventing beta-cell loss and diabetes with calcium channel blockers. Diabetes (2012) 61:848–56. doi: 10.2337/db11-0955
17. Park HW, Park H, Semple IA, Jang I, Ro SH, Kim M, et al. Pharmacological correction of obesity-induced autophagy arrest using calcium channel blockers. Nat Commun. (2014) 5:4834. doi: 10.1038/ncomms5834
18. Kleiner DE, Brunt EM, Van Natta M, Behling C, Contos MJ, Cummings OW, et al. Design and validation of a histological scoring system for nonalcoholic fatty liver disease. Hepatology (2005) 41:1313–21. doi: 10.1002/hep.20701
19. Zhang Y, Li J. Carbachol ameliorates lipopolysaccharide-induced intestinal epithelial tight junction damage by down-regulating NF-kappabeta and myosin light-chain kinase pathways. Biochem Biophys Res Commun. (2012) 428:321–6. doi: 10.1016/j.bbrc.2012.10.056
20. Andrews NC, Faller DV. A rapid micropreparation technique for extraction of DNA-binding proteins from limiting numbers of mammalian cells. Nucleic Acids Res. (1991) 19:2499. doi: 10.1093/nar/19.9.2499
21. Byrne CD, Targher G. NAFLD: a multisystem disease. J Hepatol. (2015) 62:S47–64. doi: 10.1016/j.jhep.2014.12.012
22. Xu D, Wu Y, Liao ZX, Wang H. Protective effect of verapamil on multiple hepatotoxic factors-induced liver fibrosis in rats. Pharmacol Res. (2007) 55:280–6. doi: 10.1016/j.phrs.2006.12.003
23. Uslu S, Uysal A, Bilir A, Soner BC, Oktem G. Hepatic progenitor cell inhibition during embryonic period with high dose verapamil; liable joint to the cancer therapy. Bratisl Lek Listy. (2013) 114:369–75. doi: 10.4149/BLL_2013_079
24. Buzzetti E, Pinzani M, Tsochatzis EA. The multiple-hit pathogenesis of non-alcoholic fatty liver disease (NAFLD). Metabolism (2016) 65:1038–48. doi: 10.1016/j.metabol.2015.12.012
25. Kamari Y, Shaish A, Vax E, Shemesh S, Kandel-Kfir M, Arbel Y, et al. Lack of interleukin-1alpha or interleukin-1beta inhibits transformation of steatosis to steatohepatitis and liver fibrosis in hypercholesterolemic mice. J Hepatol. (2011) 55:1086–94. doi: 10.1016/j.jhep.2011.01.048
26. Kumar R, Prakash S, Chhabra S, Singla V, Madan K, Gupta SD, et al. Association of pro-inflammatory cytokines, adipokines & oxidative stress with insulin resistance & non-alcoholic fatty liver disease. Indian J Med Res. (2012) 136:229–36.
27. Stienstra R, Saudale F, Duval C, Keshtkar S, Groener JE, van Rooijen N, et al. Kupffer cells promote hepatic steatosis via interleukin-1beta-dependent suppression of peroxisome proliferator-activated receptor alpha activity. Hepatology (2010) 51:511–22. doi: 10.1002/hep.23337
28. Osborn O, Brownell SE, Sanchez-Alavez M, Salomon D, Gram H, Bartfai T. Treatment with an Interleukin 1 beta antibody improves glycemic control in diet-induced obesity. Cytokine (2008) 44:141–8. doi: 10.1016/j.cyto.2008.07.004
29. Netea MG, Joosten LA, Lewis E, Jensen DR, Voshol PJ, Kullberg BJ, et al. Deficiency of interleukin-18 in mice leads to hyperphagia, obesity and insulin resistance. Nat Med. (2006) 12:650–6. doi: 10.1038/nm1415
30. Feve B, Bastard JP. The role of interleukins in insulin resistance and type 2 diabetes mellitus. Nat Rev Endocrinol. (2009) 5:305–11. doi: 10.1038/nrendo.2009.62
31. Wang HN, Wang YR, Liu GQ, Liu Z, Wu PX, Wei XL, et al. Inhibition of hepatic interleukin-18 production by rosiglitazone in a rat model of nonalcoholic fatty liver disease. World J Gastroenterol. (2008) 14:7240–6. doi: 10.3748/wjg.14.7240
32. Zhou F, Zhang Y, Chen J, Hu X, Xu Y. Liraglutide attenuates lipopolysaccharide-induced acute lung injury in mice. Eur J Pharmacol. (2016) 791:735–40. doi: 10.1016/j.ejphar.2016.10.016
33. Kolliputi N, Galam L, Parthasarathy PT, Tipparaju SM, Lockey RF. NALP-3 inflammasome silencing attenuates ceramide-induced transepithelial permeability. J Cell Physiol. (2012) 227:3310–6. doi: 10.1002/jcp.24026
34. Stienstra R, van Diepen JA, Tack CJ, Zaki MH, van de Veerdonk FL, Perera D, et al. Inflammasome is a central player in the induction of obesity and insulin resistance. Proc Natl Acad Sci USA. (2011) 108:15324–9. doi: 10.1073/pnas.1100255108
35. Dixon LJ, Flask CA, Papouchado BG, Feldstein AE, Nagy LE. Caspase-1 as a central regulator of high fat diet-induced non-alcoholic steatohepatitis. PLoS ONE (2013) 8:e56100. doi: 10.1371/journal.pone.0056100
36. Oslowski CM, Hara T, O'Sullivan-Murphy B, Kanekura K, Lu S, Hara M, et al. Thioredoxin-interacting protein mediates ER stress-induced beta cell death through initiation of the inflammasome. Cell Metab. (2012) 16:265–73. doi: 10.1016/j.cmet.2012.07.005
37. Zhang X, Zhang JH, Chen XY, Hu QH, Wang MX, Jin R, et al. Reactive oxygen species-induced TXNIP drives fructose-mediated hepatic inflammation and lipid accumulation through NLRP3 inflammasome activation. Antioxid Redox Signal (2015) 22:848–70. doi: 10.1089/ars.2014.5868
38. Ding C, Zhao Y, Shi X, Zhang N, Zu G, Li Z, et al. New insights into salvianolic acid A action: Regulation of the TXNIP/NLRP3 and TXNIP/ChREBP pathways ameliorates HFD-induced NAFLD in rats. Sci Rep. (2016) 6:28734. doi: 10.1038/srep28734
Keywords: hepatic metaflammation, NLRP3 inflammasome, non-alcoholic fatty liver disease, verapamil, thioredoxin-interacting protein(TXNIP)
Citation: Zhou F, Zhang Y, Chen J, Hu Y and Xu Y (2018) Verapamil Ameliorates Hepatic Metaflammation by Inhibiting Thioredoxin-Interacting Protein/NLRP3 Pathways. Front. Endocrinol. 9:640. doi: 10.3389/fendo.2018.00640
Received: 14 June 2018; Accepted: 09 October 2018;
Published: 31 October 2018.
Edited by:
Anna C. Calkin, Baker Heart and Diabetes Institute, AustraliaReviewed by:
Sarah M. Turpin-Nolan, Biomedicine Discovery Institute, Monash University, AustraliaGraeme Lancaster, Baker Heart and Diabetes Institute, Australia
Copyright © 2018 Zhou, Zhang, Chen, Hu and Xu. This is an open-access article distributed under the terms of the Creative Commons Attribution License (CC BY). The use, distribution or reproduction in other forums is permitted, provided the original author(s) and the copyright owner(s) are credited and that the original publication in this journal is cited, in accordance with accepted academic practice. No use, distribution or reproduction is permitted which does not comply with these terms.
*Correspondence: Yancheng Xu, MjAxMHp5MTZAc2luYS5jbg==
†These authors have contributed equally to this work