- State Key Laboratory of Biocontrol, Institute of Aquatic Economic Animals and Guangdong Province Key Laboratory for Aquatic Economic Animals, School of Life Sciences, Sun Yat-Sen University, Guangzhou, China
It is well established that estrogens regulate female reproduction through estrogen receptors (ERs) in the ovary. However, the precise physiological role of estrogen/ER signaling in reproduction processes remains poorly defined in zebrafish. In this study, we successfully generated an ERα (esr1) mutant line in zebrafish via transcription activator-like effectors nucleases (TALENs). It was found in the mutant females that the fertility was enhanced and the ovarian histology was normal at 90 days post-fertilization (dpf). However, the number of fertile females decreased with age. By 180 dpf, esr1 mutant females were infertile with degenerated ovaries, while the age-matched wild-type females were still fertile. Additionally, few large vitellogenic granules can be found in full grown (FG) follicles at 90 dpf and the expression of vtg genes were down-regulated at both 90 and 180 dpf in esr1 mutant zebrafish. Moreover, steroidogenesis pathway and mTOR signaling pathway were over-activated at 90 dpf, but declined prematurely in esr1 mutant zebrafish by 180 dpf. Collectively, the present study provides evidence that esr1 is fundamental for ovarian maintenance in zebrafish.
Introduction
Estrogens play key roles in ovarian maintenance in vertebrates (1). The biological functions of estrogens are exerted via the estrogen receptors (ERs), a series of ligand-dependent nuclear transcription factors. In vertebrates, there are two cognate subtypes of nuclear ER, estrogen receptor alpha (ERα) and estrogen receptor beta (ERβ), the ligand-activated transcription factors (2), and one subtype of membrane ER, GPER, a G-protein coupled receptor (3). Classically, the genomic actions are primarily mediated by nuclear ERs, while the non-genomic effect was mediated by membrane-associated ERs (4). In mammals, the two subtypes of nuclear ER exhibit differential or overlapping tissue distribution as well as remarkable differences in ligand-binding and transcriptional properties (5). Traditionally, ERβ is considered to be the predominant estrogen receptor form in ovary (6, 7). It is essential for ovulation efficiency and embryogenesis rather than sexual differentiation and fertility (8–11). However, genetic disruption of ERβ (Esr2) in mice did not cause severe fertility defects (8, 12). On the other hand, accumulating evidence has pointed out that ERα is a critical factor in ovarian development and functional maintenance.
In human, defects of the ERα encoding gene, ESR1, were reported to be closely related to premature ovarian failure (POF) syndrome (13–17), an ovarian defect characterized by premature depletion of ovarian follicles or arrested folliculogenesis (18). Genetic evidences were further provided by mammalian models that different lines of Esr1 knockout mice displayed diverse degrees of fertility defects in adulthood with severe ovarian disorders. Global Esr1 knockout female mice were generally considered to be infertile in adulthood, which is similar to acyclic adult animals (12, 19–22). By 10 days age, the ovaries of Esr1 null mice were indistinguishable with that in wild-type ones, containing histologically normal primordial and primary follicles. However, functional maturation of pre-ovulatory follicles in 20–50 days age was arrested, resulting in atresia or anovulatory follicles, which formed large, hemorrhagic cysts in many cases. In addition, corpora lutea were absent, indicating that the biochemical and mechanical processes for ovulation were compromised in global Esr1 knockout mice (12). Furthermore, both neuron-specific and pituitary-specific deletion of Esr1 impaired the feedback regulation on gonadotropin by estrogen, which consequently impaired the fertility of female mice (23, 24). Interestingly, female mice with specific deletion of Esr1 gene in ovarian theca cells were fertile and cycling until 4-month old. But thereafter they began to display an erratic pattern of estrous cycles and finally lost fertility prematurely by the age of 6 months (7). In light of these, there is sufficient evidence to conclude that ERα plays an important role in the process of female reproduction in mammals.
ERα is also deeply involved in diverse reproductive processes in teleosts. It comprehensively elevated in hypothalamus-pituitary-gonadal (HPG) axis during the sexual maturation period in female eels (25). In addition, it was reported in many teleost species that ERα tightly coupled to up-regulation of vitellogenins upon estrogen stimulation (25–28). More than one alpha subtype of estrogen receptors were identified in teleost due to the genome duplication, but one of these duplicated ERαs has been lost in many fish species (29). In zebrafish, ERα is the only one alpha subtype of estrogen receptor (30). Similar to mammals, ERα enriched in the follicle layer in the ovary of zebrafish. It increased at pre-vitellogenic stage and later peaks at mid-vitellogenic stage follicles temporally (31). Furthermore, ERα is an essential factor widespread in HPG axis and reproduction system of zebrafish. Our recent study has confirmed that esr1 was colocalized with lhb in pituitary and mediates the direct estrogenic effects on lhb in pituitary (32). Taken together, lines of evidence suggests that ERα is located comprehensively in reproduction system of teleosts and deeply involved in regulation of various reproductive processes.
However, the role of ERα in ovary development and maintenance has not yet been completely clarified. In this study, we generated an ERα (esr1) mutant zebrafish line via TALENs and evaluated the fertility and ovarian histology of the females. It was found that esr1 deficient zebrafish developed enhanced fertility with normal ovarian histology at 90 dpf, but developed POF with degenerated ovaries at 180 dpf. As well, defect in vitellogenesis was found in these zebrafish. Further investigation showed that the alteration of steroidogenesis pathway and mTOR signaling pathway may be relevant to the reproduction disorder in ERα deficient zebrafish.
Materials and Methods
Zebrafish Husbandry
AB strain zebrafish (Danio rerio) were maintained in a 14 h light (8:00–22:00): 10 h dark (22:00–8:00) cycle at 28 ± 1°C in our laboratory in the Sun Yat-Sen University, following the protocols described in The zebrafish book: a guide for the laboratory use of zebrafish (33). The wild-type and esr1 mutant zebrafish were raised separately in tanks with the same size in the same system. About 35 zebrafish were maintained in each tank containing 10 L water. The larvae and adult zebrafish were fed with brine shrimp (hatched from eggs in 30 mL in 8 L saline water) twice daily. All animal experiments conducted were in accordance with the guidelines and approval of the respective Animal Research and Ethics Committees of Sun Yat-Sen University.
Establishment of esr1 Gene Disruption Zebrafish Line via TALENs
To obtain the zebrafish mutant line, specific TALEN target sites were designed locating on the third exon (Figure 1A) of zebrafish esr1 (Gene ID: 259252), which encodes the DNA-binding domain (Figure 1C). Paired TALENs were constructed with the Golden Gate TALEN Kit (Addgene, Cambridge, MA, USA) as reported (34, 35). Approximately 300 pg TALEN mRNAs were microinjected into one-cell stage zebrafish embryos and then reared at the temperature of 28.5°C. Twenty four hours after injection, 10 embryos were collected for DNA extraction to check whether the targeted genomic fragment was deleted. The target genomic regions were amplified by PCR and subcloned into the pTZ57R/T vector (Fermentas). Single colonies were genotyped by sequencing.
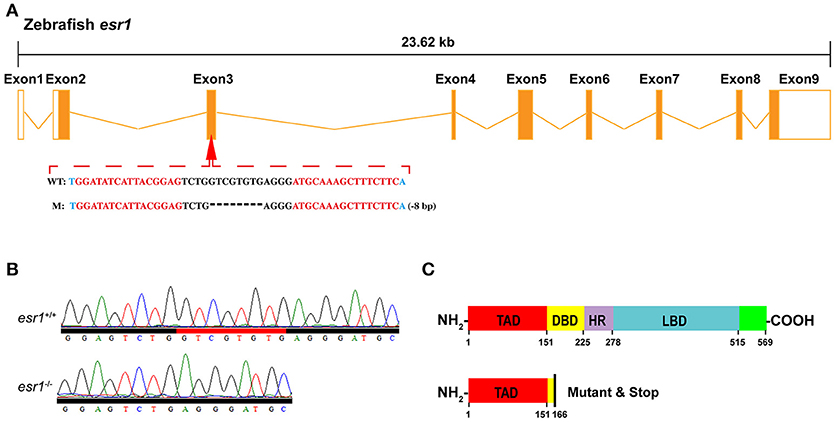
Figure 1. Targeted disruption of zebrafish esr1 gene. (A) Schematic representation of genomic structure of zebrafish esr1 gene and the TALENs target sites on zebrafish esr1 gene. (B) Sequencing results of esr1 gene from wild-type (esr1+/+) and homozygous (esr1−/−) zebrafish. (C) Mutation in exon 3 of esr1 causes early termination of translation. TAD, transactivation domain; DBD, DNA-binding domain; HD, hinge domain; LBD, ligand binding domain.
To obtain germline mutations, the P0 generation zebrafish were raised to adulthood and mated to wild-type fish to generate heterozygous F1 offspring. The heterozygous F1 generation fish were genotyped via fin clip assay and the individuals with frame-shift sequence alterations were selected. Males and females of F1generation carrying the same mutation were mated to produce F2 homozygous mutants which were genotyped via PCR and sequencing. Specific primers for PCR genotyping are listed in Supplementary Table 1.
Fertility, Fecundity, and Fertilization Rate Assessment
A tracing fertility assessment was conducted with 10 wild-type and 10 mutant female zebrafish for 3 months (from 90 to 180 days post-fertilization). The wild-type and mutant females were one-to-one paired with wild-type males every 5 days interval. The number of spawned individuals and non-spawned individuals were recorded for each batch and the spawning ratio was calculated as (number of spawned individuals/sum accessed) × 100%.
To induce spawning, one female was paired with one male in a spawning tray. One hour after light on in the morning, spawned eggs were collected and counted. Fecundity is the count of number of eggs spawned (36). The developing embryos were maintained in 30% Danieau's solution at 28°C. The number of fertilized and unfertilized eggs were identified with a dissecting microscope at 4 h post-fertilization (hpf). The fertilization ratio was calculated as (fertilized eggs/total eggs) × 100%. Data of fecundity and fertilization rate were obtained from 10 independent crosses.
In vivo Exposure Experiment
For in vivo drug exposure, wild-type female zebrafish (n = 6) were exposed to 50 nM of rapamycin (MCE, USA) for 14 days. The water in each tank was replaced daily. After drug treatment, zebrafish were anesthetized and sacrificed. Body weight and gonad weight were measured and the ovaries were prepared for histological analyses.
Morphological and Histological Analyses
Morphological and histological analyses were performed as described (36, 37). Briefly, morphology and histology of the zebrafish ovaries (n = 6) was examined at 60, 90, 120, and 180 days post-fertilization (dpf). Zebrafish were euthanized with MS-222 and images were taken with a digital camera. Body length and body weight were measured. Then the ovary was dissected for gonad weight measurement and histological examination. The gonad-somatic index (GSI) was calculated as (gonad weight/body weight) × 100%. For gonad histology analyses, the ovary samples were fixed in paraformaldehyde overnight at 4°C. The samples were dehydrated through a graded series of ethanol and embedded in paraffin and serially cut into 7 μm sections on a Leica microtome. After rehydration, the sections were stained in hematoxylin and eosin in turn and mounted with Canada balsam (Sigma-Aldrich, USA) for microscopic examination. The division of follicle stages was referred to previous works (38, 39).
Whole-Body Steroid Hormone Determination
Whole-body steroid measurement was performed as described previously (37). Firstly, female zebrafish (n = 6) were anesthetized with MS-222. Body weight of each zebrafish was measured prior to homogenization. They were then homogenized in 3 mL of PBS in glass tubes. Five millilitre of diethyl ether was added to each tube, vortexed for 1 min, centrifuged at 3,000 g for 2 min, and then frozen in a methanol/dry ice bath. The ether layer was poured into another set of tubes and evaporate the diethyl ether to dryness in a fuming hood. This procedure was repeated twice to enhance the extraction efficiency and the final product was dissolved in PBS solution. The estradiol level was accessed with Estradiol ELISA Kit (Cayman Chemical Company, Ann Arbor, MI, USA) and 11-KT with 11-keto Testosterone ELISA Kit (Cayman Chemical Company, Ann Arbor, MI, USA). The assays were conducted following the manufacturer's instructions. The standard series and all samples were conducted triplicated.
RNA Isolation and RT-PCR
Total RNA was extracted from the ovary and liver samples (n = 6) with TRIzol reagent (Invitrogen, USA). As follow, 1 μg total RNA was used as template to produce cDNAs with Rever Tra Acea-first strand cDNA Synthesis Kit (TOYOBO, Japan). The transcriptional level of the target genes was measured by the ABI Real-Time PCR Fast System with SYBR Green PCR Master Mix Kit. Procedure of quantitative RT-PCR was shown: denaturation at 95°C for 10 min, followed by 40 cycles of 95°C for 15 s, 58°C for 15 s, 72°C for 20 s, and then 84°C for 10 s (fluorescent data collection). All mRNA quantification data were normalized to ef1a and presented as fold changes to relative control groups. The specific primers used in this study are listed in Supplemental Table 1.
Western Blot Analysis
Western blot analysis was performed as previously described (32, 40). Firstly, the pituitary samples (n = 3) were lysed separately in 40 μL of RIPA lysis buffer (Fudebio-Tech, China) containing a protease inhibitor cocktail (1:100, Fudebio-Tech), mixed with 10 μL of 5× DualColor Protein Loading Buffer (Fudebio-Tech). Then the mixture was heated at 100°C for 10 min. Secondly, the lysates and PageRulerTM prestained protein ladders (Thermo Fisher, USA) were separated on 12% SDS-PAGE gels and transferred to PVDF membranes (Pall, USA). Thirdly, the membranes were incubated 30 min in 5% BSA resolved in TBST prior to the incubation of the primary antibody for blocking and cut into pieces according to visible marker bands (25 and 35 KD). The membranes with larger Mw proteins were incubated with GAPDH mouse monoclonal primary antibodies (1:4,000, Proteintech, RRID: AB_2107436), and the ones with smaller Mw proteins were incubated with zebrafish specific FSHb (follicle-stimulating hormones, 1:3,000, RRID: AB_2651068) or LHb (luteinizing hormones, 1:6,000, RRID: AB_2651067) rabbit polyclonal primary antibodies, as previously reported (40). After rinsed with TBST solution for 5 times and 3 min each, they were incubated in peroxidase-conjugated second antibody and lastly visualized with enhanced chemiluminescence (ECL) reagents (Millipore, USA). All Western blot analyses were repeated twice at least.
Statistical Analyses
All data are expressed as mean values ± SEM, and the data were analyzed by Student's t-test method with the GraphPad 6.0 software (GraphPad Software, San Diego, CA). P < 0.05 was considered statistically significant.
Results
Establishment of esr1 Deficient Zebrafish Line
To disrupt esr1 gene in zebrafish, TALEN target site for the zebrafish esr1 gene was designed. There are 9 exons in zebrafish esr1 gene. The TALEN target site was located on the third exon (Figure 1A). Twenty four hours after injection of paired TALENs, 45.8% (11/24) of embryos sampled carried mutations. The remaining P0 embryos were raised to adulthood and genotyped by sequencing. The mutation rate was 50% (9/18) in the adult P0 fish. The P0 zebrafish are genetically mosaic and the ones carrying germline mutations were crossed with wild-types to produce F1 offspring. An 8-bp deletion mutation (Figure 1A), inducing an open reading frame (ORF) shift (Figure 1B) and early termination of translation (Figure 1C), was chosen to establish the esr1 mutant line.
Morphology and Ovarian Histology of esr1 Mutant Female Zebrafish
Although the body length of both lines did not show significant difference during lifespan (Figure 2A), the body weight of esr1 mutant female zebrafish was significantly higher at 90 dpf (Figure 2B). As well, the GSI of esr1 mutant female zebrafish significantly increased at 60 and 90 dpf but dramatically declined at 180 dpf compared to wild-type zebrafish (Figure 2C).
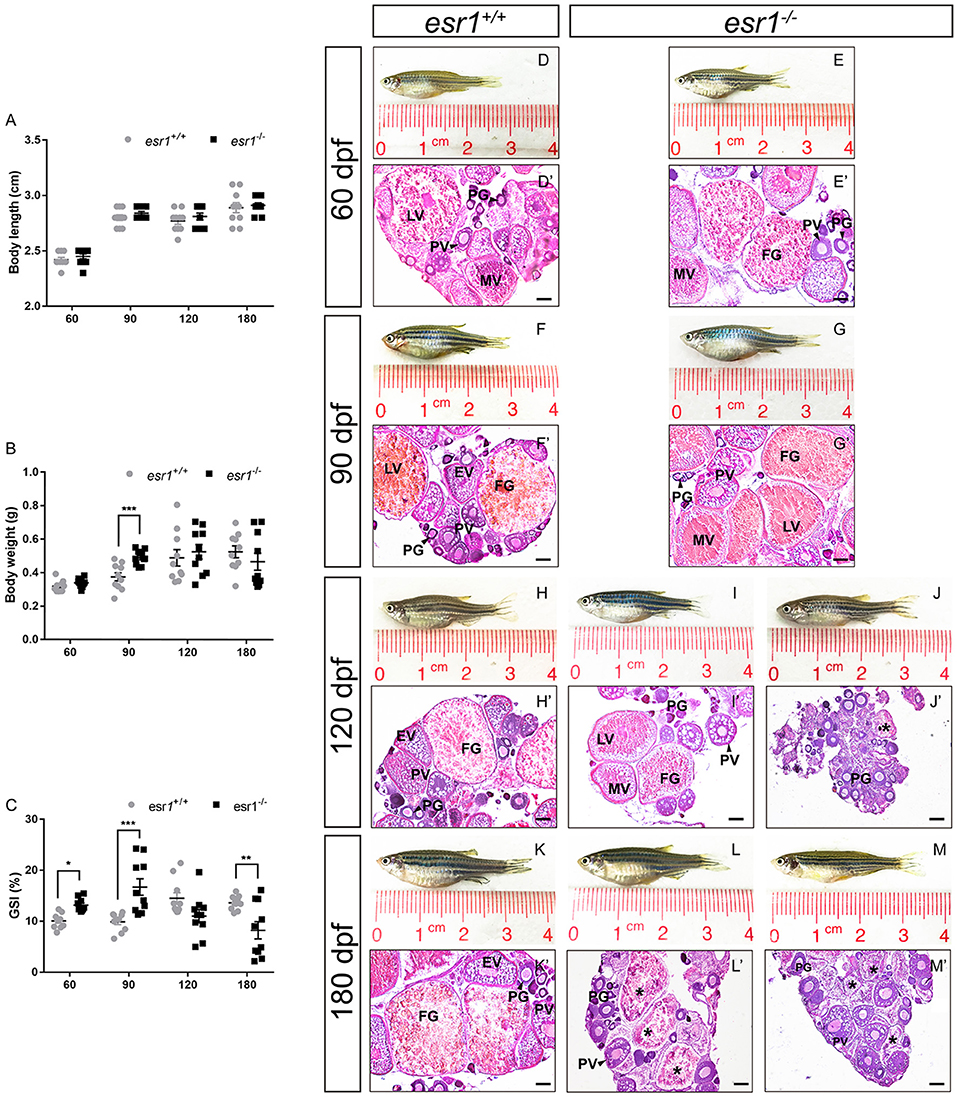
Figure 2. Morphological and histological analyses of esr1 mutant female zebrafish. (A) Body length of esr1+/+ and esr1−/− female zebrafish. (B) Body weight of esr1+/+ and esr1−/− female zebrafish. (C) GSI of esr1+/+ and esr1−/− female zebrafish. (D–M) Gross morphology of female zebrafish. (D′-G′) Ovaries of esr1+/+ and esr1 mutant were normal at 60 and 90 dpf. (H′-M′) Part of esr1 mutant ovaries of at 120 dpf and all esr1 mutant ovaries were degenerated by the 180 dpf. In these degenerated ovaries, MV and FG follicles were absent and PG follicles, PV follicles and atretic follicles (asterisk) were found. PG, primary growth follicle; PV, pre-vitelligenic follicle; EV, early-vitelligenic follicle; MV, middle-vetelligenic follicle; LV, late-vitelligenic follicle; FG, full grown follicle; *, atretic follicle.
Morphological and histological analyses were conducted to pubertal (60 dpf) wild-type and esr1 mutant females. No significant signs of abnormality in the gross morphology of both genotypes of zebrafish were observed (Figures 2D,E). Furthermore, histological analyses showed similar ovarian developmental stage in both zebrafish lines that follicles undergoing primary growth stage (PG), pre-vitellogenic stage (PV), early vitellogenic stage (EV), mid-vitellogenic stage (MV), late vitellogenic stage (LV), and full grown stage (FG) were all found (Figures 2D′,E′). A prolonged observation on the gross morphology and histology of the ovaries was conducted in adult wild-type and esr1 mutant females from 90 to 180 dpf. The body and the abdomen size of the esr1 mutant females were indistinguishable with wild-type zebrafish at 90 dpf (Figures 2F,G). But some mutant females with smaller abdomen size were observed at 120 and 180 dpf (Figures 2H–M). Histological analyses showed that similar to wild-type zebrafish, follicles ranged from PG to FG stage were all present in esr1 mutant ovaries at 90 dpf (Figures 2F′,G′). However, in part of ovaries at 120 dpf and all ovaries at 180 dpf, MV, LV, and FG follicles were absent with only early stage follicles (PG and PV stage follicles) and atretic follicles (Figures 2H′-M′).
esr1 Mutant Female Zebrafish Developed Enhanced Fertility but Premature Ovarian Failure
Tracing assessment of fertility was conducted to both wild-type and esr1 mutant females by pairing them with wild-type males. As illustrated, esr1 mutant female zebrafish possessed normal fertility as wild-type females at 90 dpf. However, they showed signs of an age-dependent fertility loss from 105 dpf that the number of spawned esr1 mutant zebrafish reduced with age. By 180 dpf, all esr1 mutant females were infertile, while the age-matched wild-type females were still fertile (Figure 3A). Moreover, esr1 deficient zebrafish produced more eggs compared to age-matched wild-type zebrafish from 90 to 150 dpf (Figure 3B). Nevertheless, disruption of esr1 did not affect the fertilization rate in zebrafish (Figure 3C).
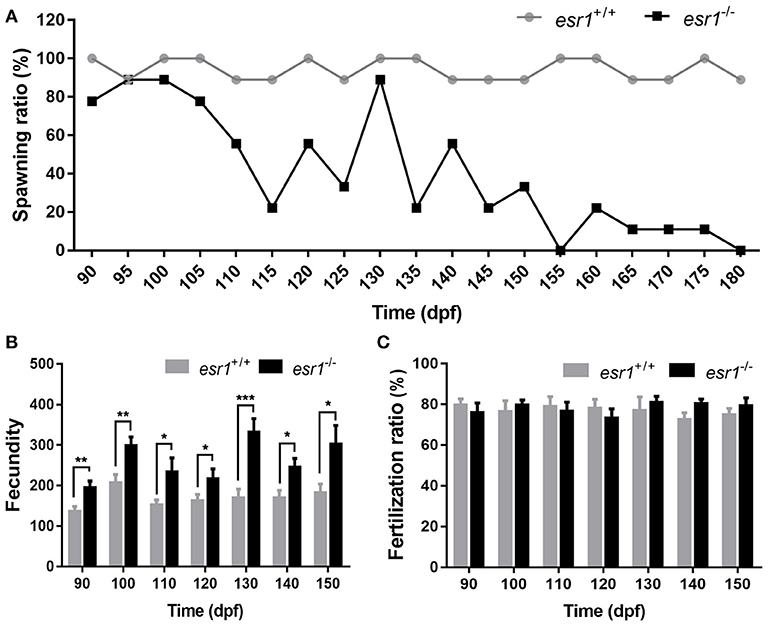
Figure 3. Tracing assessment of fertility on esr1 mutant female zebrafish. (A) Spawning rate of esr1 mutant female zebrafish was normal at 90 dpf but violently declined at 180 dpf. (B) Fecundity was significantly higher in esr1 mutant female zebrafish. (C) Fertilization rate was normal in esr1 mutant female zebrafish. (*P < 0.05; **P < 0.01; ***P < 0.001; n = 10; Mean ± SEM).
Vitellogenesis in esr1 Mutant Female Zebrafish
It was noted that a majority number of LV stage follicles in esr1 mutant female ovaries were abnormal morphologically. LV stage follicles of wild-type zebrafish were filled with vitellogenic granules (Figure 4A). However, vitellogenic granules were only found in the lateral part of esr1 mutant LV stage follicles (Figure 4B). Similarly, FG stage follicles were filled with large size vitellogenic granules in wild-type follicles (Figure 4C), while few large vitellogenic granules were found in esr1 mutant FG stage follicles (Figure 4D). To further analyze the molecular mechanism underlying, the expression levels of vtg genes in liver were evaluated. The expression level of vtg1 was significantly decreased at 90 dpf in spite of the other six vtg genes (Figure 4E). However, all vtg genes decreased violently in the liver by the age of 180 dpf (Figure 4F).
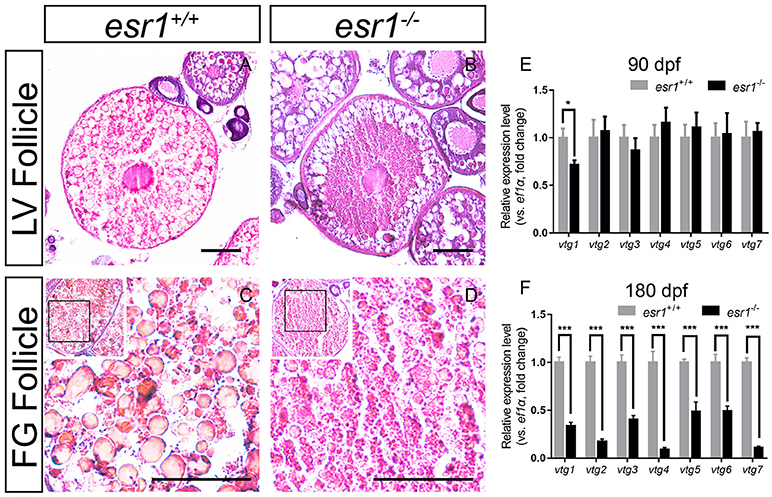
Figure 4. Vitellogenesis in esr1 deficient female zebrafish. (A) LV stage follicles were filled with vitellogenic granules in wild-type zebrafish. (B) Vitellogenic granules were found in the lateral part of LV stage follicles in esr1 mutants. (C) FG stage follicles were filled with big full vitellogenic granules in wild-types. (D) Only small vitellogenic granules were found in FG stage follicles of esr1 mutants. (E,F) Expression level of seven vtg genes at 90 dpf (E) and 180 dpf (F). The mRNA levels were normalized to ef1a. Average mRNA levels in ovaries of wild-types were defined as 1.0. (*P < 0.05; ***P < 0.001; Mean ± SEM).
Steroidogenesis Pathway in esr1 Mutant Female Zebrafish
Western blot analyses showed that the pituitary protein levels of both FSHb and LHb remained unchanged in esr1 mutant zebrafish at 90 dpf, yet they both significantly declined by the age of 180 dpf (Figure 5A). Then, E2 and 11-KT levels in whole body were measured. E2 level was significantly higher in esr1 mutant females at 90 dpf, while significantly lower at 180 dpf (Figure 5B). The 11-KT concentration was unchanged at 90 dpf, but significantly decreased at 180 dpf in esr1 mutant females (Figure 5C). Furthermore, panels of genes involved in steroidogenesis in the ovary were analyzed. At 90 dpf, expression levels of genes in steroidogenesis pathway were significantly up-regulated in esr1 mutant females, including star, hsd17b1, hsd17b3, cyp19a1a, and fshr (Figures 5D,F–I). Expression of lhcgr and cyp11a2 mRNA levels were unchanged (Figures 5E,J) and the expression level of hsd11b2 decreased significantly at this age (Figure 5K). However, the expression of all these genes significantly decreased in esr1 mutant females at 180 dpf (Figures 5D–K).
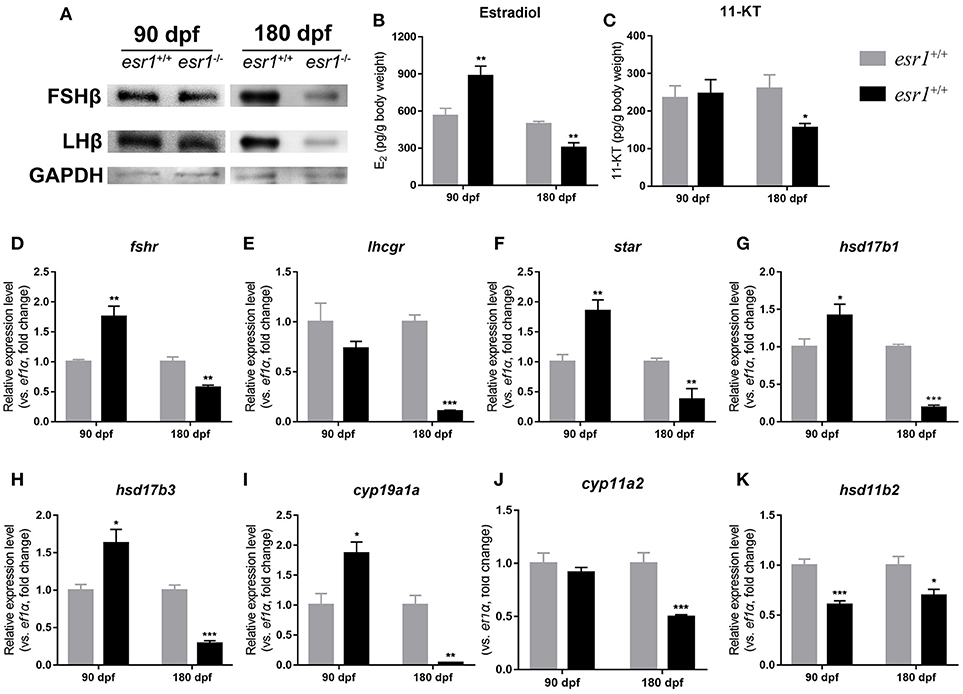
Figure 5. Steroidogenesis in esr1 mutant female zebrafish. (A) FSHb and LHb levels at 90 dpf and 180 dpf were measured via Western bolt analysis. GAPDH was used as the reference. (B,C) Whole-body estradiol (B) and 11-KT (C) levels were measured via ELISA. (D–K) The relative expression levels of steroidogenesis pathway genes in the ovaries of wild-type and esr1 mutant zebrafish at 90 dpf and 180 dpf. The mRNA levels were normalized to ef1a. Average mRNA levels in the ovaries of wild-type were defined as 1.0. (*P < 0.05; **P < 0.01; ***P < 0.001; Mean ± SEM).
IGF System and mTOR Signaling Pathway in esr1 Mutant Female Zebrafish
At 90 dpf, the transcriptional levels of tsc genes were significantly down-regulated (Figures 6A–C). Expression of mtor significantly increased in esr1 mutant female zebrafish (Figure 6D). The downstream transcriptional factors including ribosomal protein S6 kinase b (rps6kb1a and rps6kb1b) and eukaryotic translation initiation factor 4e binding protein 1 (4ebp1) were unchanged (Figures 6E–G). The mRNA levels of eukaryotic translation initiation factor 4e (eif4ea) and ribosomal protein S6 (rps6) significantly elevated in esr1 mutant females (Figures 6H,I). Furthermore, igf1, igf2a, and igf2b were significantly up-regulated compared to wild-type females (Figures 6J–L). But the expression levels of two igf1r genes (igfr1a and igfr1b) did not show significant difference between wild-type and esr1 mutant females (Figures 6M,N). However, by the age of 180 dpf, the expression levels of both mTOR signaling pathway and the IGF system were comprehensively down-regulated (Figures 6A–N). Diagrammatic sketch of mTOR signaling pathway was presented in Figure 6O.
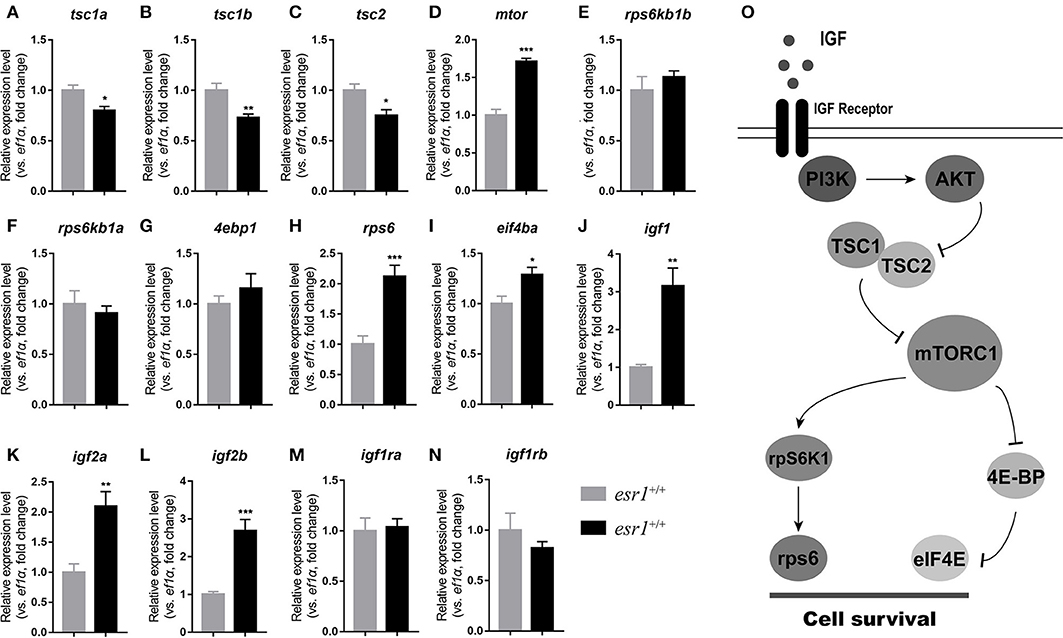
Figure 6. IGF system and mTOR signaling pathway in esr1 mutant female zebrafish. (A–I) The relative expression levels of mTOR signaling pathway genes in the ovaries of wild-type and esr1 mutant zebrafish at 90 and 180 dpf. (J–N) The relative expression levels of igf /igfr genes in the ovaries of wild-type and esr1 mutant zebrafish at 90 and 180 dpf. The mRNA levels were normalized to ef1a. Average mRNA levels in the ovaries of wild-type were defined as 1.0. (*P < 0.05; **P < 0.01; ***P < 0.001; Mean ± SEM). (O) Diagrammatic sketch of mTOR signaling pathway.
Inhibition of mTOR Signaling Pathway Led to Ovarian Failure
In order to clarify the role of mTOR signaling pathway in ovarian maintenance, in vivo exposure to rapamycin, an mTOR inhibitor, was conducted to adult wild-type female zebrafish. After treatment with 50 nM rapamycin for 14 days, the gross morphology (Figures 7A,B) and the body weight (Figure 7C) of zebrafish were unchanged, yet these zebrafish were infertile. In this case, the GSI of the rapamycin treatment group was significantly lower compared to the control group (Figure 7D). Moreover, MV, LV, and FG stage follicles were absent in ovaries of rapamycin treated zebrafish. Only PG and PV stage follicles and a few atretic follicles were found, while the ovaries in control group were normal (Figures 7A′,B′).
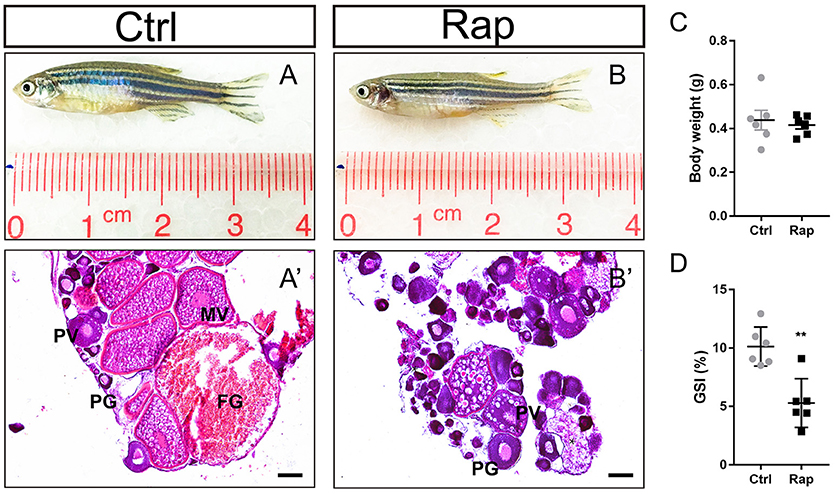
Figure 7. Morphology and ovarian histology of rapamycin treated zebrafish (A,B) Gross morphology of control and rapamycin treated zebrafish. (A′,B′) In rapamycin treated zebrafish, only PG follicles, PV follicles and atretic follicles (asterisk) were found in the ovaries. (C,D) Body weight (C,D) GSI of control and rapamycin treated zebrafish. (*P < 0.05; **P < 0.01; ***P < 0.001; Mean ± SEM). PG, primary growth follicle; PV, pre-vitelligenic follicle; *, atretic follicle.
Discussion
ERα was shown to be significant in female reproduction in human and mice models. Evidence in teleost fish also suggested its significance in female reproduction. Thus esr1 mutant zebrafish line was generated to investigate the function of esr1 in fish ovary. In mammals, global knockout of Esr1 in mice resulted in infertility with primary amenorrhea (12, 19). Whereas, conditional knockout of Esr1 in several positions of HPG axis caused milder degree of fertility impairment [Table 1; (7, 23, 24)]. In zebrafish, ERα is the unique alpha subtype of estrogen receptor. The expression of esr1 is detected in ovary of zebrafish. However, its function in ovary remains unclarified. In a recent work, an esr1 mutant line of zebrafish was generated via CRISPR/Cas9 system. Sex differentiation, gonad development and reproductive function were normal in esr1 deficient zebrafish. Fertility assessment found that they were fertile by the age of 120 dpf (Table 1). However, double knockout of ERβs (esr2a−/−; esr2b−/−) resulted in completely arrested follicle development and female-to-male sex reversal, suggesting the significance of ERβs in maintenance of female sex status in zebrafish (42). In addition, disruption of esr1 in medaka did not cause defects in gonadal development or sexual characteristics, which is similar to the phenotype in zebrafish [Table 1; (41)]. In the present study, we found that the esr1 deficient zebrafish produced more eggs with normal ovarian histology at 90 dpf. But the number of fertile females decreased with age. By the age of 180 dpf, esr1 mutant female zebrafish developed POF with highly degenerated ovaries, which is consistent with the phenotype in a theca cell specific Esr1 knockout mice model (7). In zebrafish suffering from POF, MV, LV, and FG follicles were absent and only PG, PV and atretic follicles were found in their ovaries. Similarly, theca cell specific Esr1 knockout mice over 4-month age possessed fewer corpora lutea but more antral follicles in their ovaries than the age-matche wild-type mice (7). The female reproductive phenotypes of rodent and teleost models were summarized in Table 1, and the similarities between mice and teleosts suggest that the function of ERα in ovary may be significant and conserved in vertebrates.
The expression levels of vitellogenin (vtg) genes were down-regulated in esr1 mutant zebrafish. Similarly, the estrogenic effect on vtg in esr1 knockout medaka was significantly attenuated (41). To date, at least seven vtg genes (vtg1–7) are identified in zebrafish, encoding heterogeneous vitellogenins with three distinct types of Vtgs: type I (Vtg1, 4–7), type II (Vtg2), and type III (Vtg3). All seven vtgs are predominantly expressed in female liver, among which vtg1 is the highest expressed one and type I Vtg is considered to be the predominant form in zebrafish (43–46). As demonstrated in the present work, vtg1 was suppressed as a result of esr1 deletion regardless of the other six vtg genes, which may be a cause for the histological alteration in esr1 mutant zebrafish follicles. However, work on protein level is warranted to draw a conclusion.
In teleosts, steroid hormones are deeply involved in the regulation of folliculogenesis (47). Synthesis of steroid hormones is under the control of gonadotropins (38, 47). As illustrated, the protein levels of FSHb and LHb did not alter significantly at 90 dpf, which differs from the Esr1 deficient mice models (Table 1). However, the mRNA level of fshr increased. As a result, the steroidogenesis pathway was up-regulated. Rather than LH, FSH stimulates follicular growth and steroid hormone production which is mainly mediated by fshr (48). Fshr has been shown in mammals that it can mediate the effect of FSH through the Pi3k/Akt/mTOR signaling pathway (49). Additionally, the involvement of ERα in FSH feedback regulation is clearly elucidated in mice (24, 50). In zebrafish, both FSH and fshr are sensitive to estradiol (48, 51). Therefore, loss of esr1 caused disorders in FSH and fshr regulation, which may up-regulate steroidogenesis pathway and production of estradiol at 90 dpf.
The Pi3k/Akt/mTOR signaling pathway is generally considered to be closely related to POF in mammals. In human, activation of this signaling pathway leads to premature and irreversible initiation of primordial follicle pool, and eventually results in premature follicle depletion and ovarian failure (52). Similarly, conditional knockout of Tsc1, Tsc2, and Pten specific in mice oocytes leads to up-regulation of mTOR signaling pathway and global activation of primordial follicles prematurely, which eventually causes POF (53–56). In the present work, the expression levels of genes in mTOR signaling pathway were assessed. At 90 dpf, the expression levels of rps6 and eif4ea increased significantly. And the igf /igfr system, the direct upstream of the PI3K/AKT/mTOR pathway (57–59), was also up-regulated. It has been shown that the IGF system is present and dynamic in the zebrafish ovary, which functions directly or indirectly in follicle development and ovarian maintenance (60, 61). Thus, the up-regulation of IGF system in esr1 mutant zebrafish may activate the mTOR signaling pathway, and eventually over-activated follicle development in these zebrafish. Taken together, the up-regulation of IGF system and mTOR signaling pathway is probably led by FSH/fshr disorders and results in fertility enhancement at 90 dpf.
On the other hand, by the age of 180 dpf, the IGF system and mTOR signaling pathway were comprehensively down-regulated in esr1 mutant zebrafish, accompanied with ovarian failure. Treatment with rapamycin, an mTOR inhibitor, caused a similar phenotype in adult female zebrafish, indicating that the ovarian failure in esr1 mutant zebrafish is closely relevant to the down-regulation of mTOR signaling pathway. Moreover, fshr deficient female zebrafish showed a complete failure in follicle activation with follicles arrested in PG to PV transition (39), which is highly consistent with our results. Therefore, down-regulation of fshr and mTOR signaling pathway in esr1 deficient zebrafish may be relevant to ovarian failure. In this work, ovary samples were used for analysis and the expression profiles of genes in ovary were related to age and developmental stages. Indeed, using samples with the same age and same developmental stage would provide more precise evidence for understanding the mechanism by which esr1 works. However, the stage-matched follicles were not easily available in this case. To clarify this mechanism, further studies were warranted.
Similar atretic follicle presented in this work was also reported in environmental toxicology cases. Chronic, dietary exposure to 2,3,7,8-tetrachlorodibenzo-p-dioxin (TCDD) decreased egg production and spawning success in zebrafish. In this case, transition of pre-vitellogenic stage follicles to vitellogenic stage follicles were inhibited, resulting in follicular atresia (62), which is similar to ovaries of esr1 deficient zebrafish at 180 dpf. Moreover, long-term exposure to bromophenol 2,4,6-tribromophenol (TBP) caused defects in vitellogenesis in zebrafish (63). These endocrine disruption chemicals (EDCs) are shown to comprehensively influence the reproduction system and cause severe physiological disorder. However, the mechanisms are still unclear. Our present work provides new insights that these EDCs may effect through ERα as well as the mTOR signaling pathway.
In summary, using the TALEN-mediate gene edition system, we generated the esr1 deficient zebrafish line. Female mutant zebrafish obtained enhanced fertility, but developed POF later. Furthermore, the relevance of mTOR signaling pathway to fertility enhancement and POF was demonstrated. Thus, this mutant line could be a new ideal vertebrate model for clinical research in POF. Collectively, this work provides genetic evidence that ERα is critical for ovarian maintenance in zebrafish, which hence furthers our understanding of the function of ERα in vertebrate reproduction.
Author Contributions
YC, LW, JH, YG, and YL conducted all the experiments and analyzed the data. HT, XL, and HL designed the experiments. YC and HT wrote the manuscript.
Funding
This work was funded by National Natural Science Foundation of China (NSFC Grant No. 31701302, 31372512), Guangdong Provincial Natural Science Foundation (Grant No. 2017A030310312), and Fundamental Research Funds for the Central Universities (Grant No. 17lgpy108).
Conflict of Interest Statement
The authors declare that the research was conducted in the absence of any commercial or financial relationships that could be construed as a potential conflict of interest.
Acknowledgments
We thank Yike Yin, Gaofei Li, and Yali Liu for expert technical assistance. We also thank Christopher H. K. Cheng for supplying us the antibodies for LHb and FSHb.
Supplementary Material
The Supplementary Material for this article can be found online at: https://www.frontiersin.org/articles/10.3389/fendo.2018.00567/full#supplementary-material
References
1. Hewitt SC, Harrell JC, Korach KS. Lessons in estrogen biology from knockout and transgenic animals. Annu Rev Physiol. (2005) 67:285–308. doi: 10.1146/annurev.physiol.67.040403.115914
2. Evans RM. The steroid and thyroid hormone receptor superfamily. Science (1988) 240:889–95. doi: 10.1126/science.3283939
3. Carmeci C, Thompson DA, Ring HZ, Francke U, Weigel RJ. Identification of a gene (GPR30) with homology to the G-protein-coupled receptor superfamily associated with estrogen receptor expression in breast cancer. Genomics (1997) 45:607–17. doi: 10.1006/geno.1997.4972
4. Bjornstrom L, Sjoberg M. Mechanisms of estrogen receptor signaling: convergence of genomic and nongenomic actions on target genes. Mol Endocrinol. (2005) 19:833–42. doi: 10.1210/me.2004-0486
5. Couse JF, Korach KS. Estrogen receptor null mice: what have we learned and where will they lead us? Endocr Rev. (1999) 20:358–417. doi: 10.1210/edrv.20.3.0370
6. Lau KM, Mok SC, Ho SM. Expression of human estrogen receptor-alpha and -beta, progesterone receptor, and androgen receptor mRNA in normal and malignant ovarian epithelial cells. Proc Natl Acad Sci USA. (1999) 96:5722–7. doi: 10.1073/pnas.96.10.5722
7. Lee S, Kang DW, Hudgins-Spivey S, Krust A, Lee EY, Koo Y, et al. Theca-specific estrogen receptor-alpha knockout mice lose fertility prematurely. Endocrinology (2009) 150:3855–62. doi: 10.1210/en.2008-1774
8. Krege JH, Hodgin JB, Couse JF, Enmark E, Warner M, Mahler JF, et al. Generation and reproductive phenotypes of mice lacking estrogen receptor beta. Proc Natl Acad Sci USA. (1998) 95:15677–82. doi: 10.1073/pnas.95.26.15677
9. Inzunza J, Morani A, Cheng G, Warner M, Hreinsson J, Gustafsson JA, et al. Ovarian wedge resection restores fertility in estrogen receptor beta knockout (ERbeta-/-) mice. Proc Natl Acad Sci USA. (2007) 104:600–5. doi: 10.1073/pnas.0608951103
10. Pikulkaew S, De Nadai A, Belvedere P, Colombo L, Dalla Valle L. Expression analysis of steroid hormone receptor mRNAs during zebrafish embryogenesis. Gen Comp Endocrinol. (2010) 165:215–20. doi: 10.1016/j.ygcen.2009.06.024
11. Celeghin A, Benato F, Pikulkaew S, Rabbane MG, Colombo L, Dalla Valle L. The knockdown of the maternal estrogen receptor 2a (esr2a) mRNA affects embryo transcript contents and larval development in zebrafish. Gen Comp Endocrinol. (2011) 172:120–9. doi: 10.1016/j.ygcen.2010.12.020
12. Schomberg DW, Couse JF, Mukherjee A, Lubahn DB, Sar M, Mayo KE, et al. Targeted disruption of the estrogen receptor-alpha gene in female mice: characterization of ovarian responses and phenotype in the adult. Endocrinology (1999) 140:2733–44. doi: 10.1210/endo.140.6.6823
13. Bretherick KL, Hanna CW, Currie LM, Fluker MR, Hammond GL, Robinson WP. Estrogen receptor alpha gene polymorphisms are associated with idiopathic premature ovarian failure. Fertil Steril. (2008) 89:318–24. doi: 10.1016/j.fertnstert.2007.03.008
14. Kim S, Pyun JA, Kang H, Kim J, Cha DH, Kwack K. Epistasis between CYP19A1 and ESR1 polymorphisms is associated with premature ovarian failure. Fertil Steril. (2011) 95:353–6. doi: 10.1016/j.fertnstert.2010.07.1067
15. M'rabet N, Moffat R, Helbling S, Kaech A, Zhang H, De Geyter C. The CC-allele of the PvuII polymorphic variant in intron 1 of the alpha-estrogen receptor gene is significantly more prevalent among infertile women at risk of premature ovarian aging. Fertil Steril. (2012) 98:965–72.e1–5. doi: 10.1016/j.fertnstert.2012.05.048
16. Qin Y, Sun M, You L, Wei D, Sun J, Liang X, et al. ESR1, HK3 and BRSK1 gene variants are associated with both age at natural menopause and premature ovarian failure. Orphanet J Rare Dis. (2012) 7:5. doi: 10.1186/1750-1172-7-5
17. De Mattos CS, Trevisan CM, Peluso C, Adami F, Cordts EB, Christofolini DM, et al. ESR1 and ESR2 gene polymorphisms are associated with human reproduction outcomes in Brazilian women. J Ovarian Res. (2014) 7:114. doi: 10.1186/s13048-014-0114-2
18. Goswami D, Conway GS. Premature ovarian failure. Hum Reprod Update (2005) 11:391–410. doi: 10.1093/humupd/dmi012
19. Lubahn DB, Moyer JS, Golding TS, Couse JF, Korach KS, Smithies O. Alteration of reproductive function but not prenatal sexual development after insertional disruption of the mouse estrogen receptor gene. Proc Natl Acad Sci USA. (1993) 90:11162–6. doi: 10.1073/pnas.90.23.11162
20. Dupont S, Krust A, Gansmuller A, Dierich A, Chambon P, Mark M. Effect of single and compound knockouts of estrogen receptors alpha (ERalpha) and beta (ERbeta) on mouse reproductive phenotypes. Development (2000) 127:4277–91.
21. Couse JF, Korach KS. Contrasting phenotypes in reproductive tissues of female estrogen receptor null mice. Ann N Y Acad Sci. (2001) 948:1–8. doi: 10.1111/j.1749-6632.2001.tb03981.x
22. Couse JF, Yates MM, Walker VR, Korach KS. Characterization of the hypothalamic-pituitary-gonadal axis in estrogen receptor (ER) Null mice reveals hypergonadism and endocrine sex reversal in females lacking ERalpha but not ERbeta. Mol Endocrinol. (2003) 17:1039–53. doi: 10.1210/me.2002-0398
23. Wintermantel TM, Campbell RE, Porteous R, Bock D, Grone HJ, Todman MG, et al. Definition of estrogen receptor pathway critical for estrogen positive feedback to gonadotropin-releasing hormone neurons and fertility. Neuron (2006) 52:271–80. doi: 10.1016/j.neuron.2006.07.023
24. Singh SP, Wolfe A, Ng Y, Divall SA, Buggs C, Levine JE, et al. Impaired estrogen feedback and infertility in female mice with pituitary-specific deletion of estrogen receptor alpha (ESR1). Biol Reprod. (2009) 81:488–96. doi: 10.1095/biolreprod.108.075259
25. Lafont AG, Rousseau K, Tomkiewicz J, Dufour S. Three nuclear and two membrane estrogen receptors in basal teleosts, Anguilla sp.: Identification, evolutionary history and differential expression regulation. Gen. Comp. Endocrinol. (2016) 235:177–91. doi: 10.1016/j.ygcen.2015.11.021
26. Pakdel F, Feon S, Le Gac F, Le Menn F, Valotaire Y. In vivo estrogen induction of hepatic estrogen receptor mRNA and correlation with vitellogenin mRNA in rainbow trout. Mol Cell Endocrinol. (1991) 75:205–12. doi: 10.1016/0303-7207(91)90162-L
27. Menuet A, Le Page Y, Torres O, Kern L, Kah O, Pakdel F. Analysis of the estrogen regulation of the zebrafish estrogen receptor (ER) reveals distinct effects of ERalpha, ERbeta1 and ERbeta2. J Mol Endocrinol. (2004) 32:975–86. doi: 10.1677/jme.0.0320975
28. Griffin LB, January KE, Ho KW, Cotter KA, Callard GV. Morpholino-mediated knockdown of ERalpha, ERbetaa, and ERbetab mRNAs in zebrafish (Danio rerio) embryos reveals differential regulation of estrogen-inducible genes. Endocrinology (2013) 154:4158–69. doi: 10.1210/en.2013-1446
29. Tohyama S, Miyagawa S, Lange A, Ogino Y, Mizutani T, Ihara M, et al. Evolution of estrogen receptors in ray-finned fish and their comparative responses to estrogenic substances. J Steroid Biochem Mol Biol. (2016) 158:189–97. doi: 10.1016/j.jsbmb.2015.12.009
30. Menuet A, Pellegrini E, Anglade I, Blaise O, Laudet V, Kah O, et al. Molecular characterization of three estrogen receptor forms in zebrafish: binding characteristics, transactivation properties, and tissue distributions. Biol Reprod. (2002) 66:1881–92. doi: 10.1095/biolreprod66.6.1881
31. Liu KC, Lau SW, Ge W. Spatiotemporal expression analysis of nuclear estrogen receptors in the zebrafish ovary and their regulation in vitro by endocrine hormones and paracrine factors. Gen Comp Endocrinol. (2017) 246:218–25. doi: 10.1016/j.ygcen.2016.12.011
32. Li G, Tang H, Chen Y, Yin Y, Ogawa S, Liu M, et al. Estrogen directly stimulates LHb expression at the pituitary level during puberty in female zebrafish. Mol Cell Endocrinol. (2018) 461:1–11. doi: 10.1016/j.mce.2017.08.003
33. Westerfield M. The Zebrafish Book: A Guide for the Laboratory Use of Zebrafish (Brachydanio rerio). Eugene, OR: M. Westerfield (1993).
34. Liu Y, Luo D, Zhao H, Zhu Z, Hu W, Cheng CH. Inheritable and precise large genomic deletions of non-coding RNA genes in zebrafish using TALENs. PLoS ONE (2013) 8:e76387. doi: 10.1371/journal.pone.0076387
35. Liu Y, Luo D, Lei Y, Hu W, Zhao H, Cheng CH. A highly effective TALEN-mediated approach for targeted gene disruption in Xenopus tropicalis and zebrafish. Methods (2014) 69:58–66. doi: 10.1016/j.ymeth.2014.02.011
36. Tang H, Liu Y, Luo D, Ogawa S, Yin Y, Li S, et al. The kiss/kissr systems are dispensable for zebrafish reproduction: evidence from gene knockout studies. Endocrinology (2015) 156:589–99. doi: 10.1210/en.2014-1204
37. Tang H, Chen Y, Liu Y, Yin Y, Li G, Guo Y, et al. New insights into the role of estrogens in male fertility based on findings in aromatase-deficient zebrafish. Endocrinology (2017) 158:3042–54. doi: 10.1210/en.2017-00156
38. Lubzens E, Young G, Bobe J, Cerda J. Oogenesis in teleosts: how eggs are formed. Gen Comp Endocrinol. (2010) 165:367–89. doi: 10.1016/j.ygcen.2009.05.022
39. Zhang Z, Lau SW, Zhang L, Ge W. Disruption of zebrafish follicle-stimulating hormone receptor (fshr) but not luteinizing hormone receptor (lhcgr) gene by talen leads to failed follicle activation in females followed by sexual reversal to males. Endocrinology (2015) 156:3747–62. doi: 10.1210/en.2015-1039
40. Chu L, Li J, Liu Y, Cheng CH. Gonadotropin signaling in zebrafish ovary and testis development: insights from gene knockout study. Mol Endocrinol. (2015) 29:1743–58. doi: 10.1210/me.2015-1126
41. Tohyama S, Ogino Y, Lange A, Myosho T, Kobayashi T, Hirano Y, et al. Establishment of estrogen receptor 1 (ESR1)-knockout medaka: ESR1 is dispensable for sexual development and reproduction in medaka, Oryzias latipes. Dev Growth Differ. (2017) 59:552–61. doi: 10.1111/dgd.12386
42. Lu H, Cui Y, Jiang L, Ge W. Functional analysis of nuclear estrogen receptors in zebrafish reproduction by genome editing approach. Endocrinology (2017) 158:2292–308. doi: 10.1210/en.2017-00215
43. Wang H, Yan T, Tan JT, Gong Z. A zebrafish vitellogenin gene (vg3) encodes a novel vitellogenin without a phosvitin domain and may represent a primitive vertebrate vitellogenin gene. Gene (2000) 256:303–10. doi: 10.1016/S0378-1119(00)00376-0
44. Tong Y, Shan T, Poh YK, Yan T, Wang H, Lam SH, et al. Molecular cloning of zebrafish and medaka vitellogenin genes and comparison of their expression in response to 17beta-estradiol. Gene (2004) 328:25–36. doi: 10.1016/j.gene.2003.12.001
45. Wang H, Tan JT, Emelyanov A, Korzh V, Gong Z. Hepatic and extrahepatic expression of vitellogenin genes in the zebrafish, Danio rerio. Gene (2005) 356:91–100. doi: 10.1016/j.gene.2005.03.041
46. Chen YY, Chan KM. Regulation of vitellogenin (vtg1) and estrogen receptor (er) gene expression in zebrafish (Danio rerio) following the administration of Cd(2)(+) and 2,3,7,8-tetrachlorodibenzo-p-dioxin (TCDD). Chemosphere (2016) 147:467–76. doi: 10.1016/j.chemosphere.2015.12.083
48. Liu KC, Lin SW, Ge W. Differential regulation of gonadotropin receptors (fshr and lhcgr) by estradiol in the zebrafish ovary involves nuclear estrogen receptors that are likely located on the plasma membrane. Endocrinology (2011) 152:4418–30. doi: 10.1210/en.2011-1065
49. Gloaguen P, Crepieux P, Heitzler D, Poupon A, Reiter E. Mapping the follicle-stimulating hormone-induced signaling networks. Front Endocrinol. (2011) 2:45. doi: 10.3389/fendo.2011.00045
50. Couse JF, Korach KS. Reproductive phenotypes in the estrogen receptor-alpha knockout mouse. Ann Endocrinol. (1999) 60:143–8.
51. Lin SW, Ge W. Differential regulation of gonadotropins (FSH and LH) and growth hormone (GH) by neuroendocrine, endocrine, and paracrine factors in the zebrafish–an in vitro approach. Gen Comp Endocrinol. (2009) 160:183–93. doi: 10.1016/j.ygcen.2008.11.020
52. Adhikari D, Liu K. Molecular mechanisms underlying the activation of mammalian primordial follicles. Endocr Rev. (2009) 30:438–64. doi: 10.1210/er.2008-0048
53. Reddy P, Liu L, Adhikari D, Jagarlamudi K, Rajareddy S, Shen Y, et al. Oocyte-specific deletion of Pten causes premature activation of the primordial follicle pool. Science (2008) 319:611–3. doi: 10.1126/science.1152257
54. Adhikari D, Flohr G, Gorre N, Shen Y, Yang H, Lundin E, et al. Disruption of Tsc2 in oocytes leads to overactivation of the entire pool of primordial follicles. Mol Hum Reprod. (2009) 15:765–70. doi: 10.1093/molehr/gap092
55. Adhikari D, Liu K. mTOR signaling in the control of activation of primordial follicles. Cell Cycle (2010) 9:1673–4. doi: 10.4161/cc.9.9.11626
56. Adhikari D, Zheng W, Shen Y, Gorre N, Hamalainen T, Cooney AJ, et al. Tsc/mTORC1 signaling in oocytes governs the quiescence and activation of primordial follicles. Hum Mol Genet. (2010) 19:397–410. doi: 10.1093/hmg/ddp483
57. Pozios KC, Ding J, Degger B, Upton Z, Duan C. IGFs stimulate zebrafish cell proliferation by activating MAP kinase and PI3-kinase-signaling pathways. Am J Physiol Regul Integr Comp Physiol. (2001) 280:R1230–1239. doi: 10.1152/ajpregu.2001.280.4.R1230
58. Samani AA, Yakar S, Leroith D, Brodt P. The role of the IGF system in cancer growth and metastasis: overview and recent insights. Endocr Rev. (2007) 28:20–47. doi: 10.1210/er.2006-0001
59. Miller TW, Balko JM, Arteaga CL. Phosphatidylinositol 3-kinase and antiestrogen resistance in breast cancer. J Clin Oncol. (2011) 29:4452–61. doi: 10.1200/JCO.2010.34.4879
60. Nelson SN, Van Der Kraak G. The role of the insulin-like growth factor (IGF) system in zebrafish (Danio rerio) ovarian development. Gen Comp Endocrinol. (2010) 168:103–10. doi: 10.1016/j.ygcen.2010.04.021
61. Nelson SN, Van Der Kraak G. Characterization and regulation of the insulin-like growth factor (IGF) system in the zebrafish (Danio rerio) ovary. Gen Comp Endocrinol. (2010) 168:111–20. doi: 10.1016/j.ygcen.2010.04.020
62. Heiden TK, Carvan MJ III, Hutz RJ. Inhibition of follicular development, vitellogenesis, and serum 17beta-estradiol concentrations in zebrafish following chronic, sublethal dietary exposure to 2,3,7,8-tetrachlorodibenzo-p-dioxin. Toxicol. Sci. (2006) 90:490–9. doi: 10.1093/toxsci/kfj085
Keywords: esr1, fertility, POF, mTOR pathway, zebrafish
Citation: Chen Y, Tang H, Wang L, He J, Guo Y, Liu Y, Liu X and Lin H (2018) Fertility Enhancement but Premature Ovarian Failure in esr1-Deficient Female Zebrafish. Front. Endocrinol. 9:567. doi: 10.3389/fendo.2018.00567
Received: 08 June 2018; Accepted: 05 September 2018;
Published: 24 September 2018.
Edited by:
Yong Zhu, East Carolina University, United StatesReviewed by:
Taisen Iguchi, National Institute for Basic Biology, JapanWei Ge, University of Macau, Macau
Shinichi Miyagawa, Tokyo University of Science, Japan
Copyright © 2018 Chen, Tang, Wang, He, Guo, Liu, Liu and Lin. This is an open-access article distributed under the terms of the Creative Commons Attribution License (CC BY). The use, distribution or reproduction in other forums is permitted, provided the original author(s) and the copyright owner(s) are credited and that the original publication in this journal is cited, in accordance with accepted academic practice. No use, distribution or reproduction is permitted which does not comply with these terms.
*Correspondence: Haipei Tang, dGFuZ2hwM0BtYWlsLnN5c3UuZWR1LmNu
Xiaochun Liu, bHNzbHhjQG1haWwuc3lzdS5lZHUuY24=