- 1Department of Diagnostic and Interventional Neuroradiology, Klinikum rechts der Isar, Technische Universität München, Munich, Germany
- 2TUM-Neuroimaging Center, Klinikum rechts der Isar, Technische Universität München, Munich, Germany
- 3Department of Diagnostic and Interventional Radiology, Klinikum rechts der Isar, Technische Universität München, Munich, Germany
Purpose: Advanced magnetic resonance imaging (MRI) methods enable non-invasive quantification of body fat situated in different compartments. At the level of the lumbar spine, the paraspinal musculature is the compartment spatially and functionally closely related to the vertebral column, and both vertebral bone marrow fat (BMF) and paraspinal musculature fat contents have independently shown to be altered in various metabolic and degenerative diseases. However, despite their close relationships, potential correlations between fat compositions of these compartments remain largely unclear.
Materials and Methods: Thirty-nine female subjects (38.5% premenopausal women, 29.9 ± 7.1 years; 61.5% postmenopausal women, 63.2 ± 6.3 years) underwent MRI at 3T of the lumbar spine using axially- and sagittally-prescribed gradient echo sequences for chemical shift encoding-based water-fat separation. The erector spinae muscles and vertebral bodies of L1–L5 were segmented to determine the proton density fat fraction (PDFF) of the paraspinal and vertebral bone marrow compartments. Correlations were calculated between the PDFF of the paraspinal muscle and bone marrow compartments.
Results: The average PDFF of the paraspinal muscle and bone marrow compartments were significantly lower in premenopausal women when compared to postmenopausal women (11.6 ± 2.9% vs. 24.6 ± 7.1% & 28.8 ± 8.3% vs. 47.2 ± 8.5%; p < 0.001 for both comparisons). In premenopausal women, no significant correlation was found between the PDFF of the erector spinae muscles and the PDFF of the bone marrow of lumbar vertebral bodies (p = 0.907). In contrast, a significant correlation was shown in postmenopausal women (r = 0.457, p = 0.025). Significance was preserved after inclusion of age and body mass index (BMI) as control variables (r = 0.472, p = 0.027).
Conclusion: This study revealed significant correlations between the PDFF of paraspinal and vertebral bone marrow compartments in postmenopausal women. The PDFF of the paraspinal and vertebral bone marrow compartments and their correlations might potentially serve as biomarkers; however, future studies including more subjects are required to evaluate distinct clinical value and reliability. Future studies should also follow up our findings in patients suffering from metabolic and degenerative diseases to clarify how these correlations change in the course of such diseases.
Introduction
Vertebral bone marrow, located in the cavities of trabecular bone and consisting predominantly of adipocytes (yellow marrow regions) or adipocytes and hematopoietic red blood cells (red marrow regions), plays a considerable role for bone health. Various metabolic diseases have shown to be associated with changes in fat compositions of vertebral bone marrow in humans. For instance, patients with osteoporosis showed significantly increased bone marrow fat (BMF) fractions when compared to healthy subjects, which suggests a shift of differentiation of mesenchymal stem cells to adipocytes rather than to osteoblasts (1, 2). Furthermore, patients suffering from type 2 diabetes mellitus (T2DM) showed significantly lower BMF unsaturation levels, and high HbA1c levels were associated with elevated BMF (3–5). In anorexia nervosa, vertebral BMF contents demonstrated to be increased (6). Importantly, the finding of elevated BMF in metabolic diseases has clinical relevance: alterations of bone marrow adiposity have already been associated with bone weakness and prevalent vertebral fractures (7–10).
Furthermore, metabolic diseases have also demonstrated independently to have an impact on other compartments of the body containing fat including paraspinal musculature, which is the compartment spatially and functionally closely related to the vertebral column, thus being particularly important for spine stabilization, balance keeping, and mobility (11, 12). In this context, T2DM, degenerative changes, low back pain (LBP), neuromuscular diseases (NMDs), and injuries, amongst others, have demonstrated to entail transformation of muscle tissue (13–18). Furthermore, osteoporosis, mainly known as a bone disease characterized by low bone mass, has also shown to have associations with changes in the composition of paraspinal musculature in terms of increased muscle fat infiltration (MFI), thus suggesting close interactions between vertebrae and paraspinal muscles (19). However, evidence of such interaction is very scarce. Increased paraspinal MFI principally harbors negative implications on muscle function and movement (11, 20, 21).
Regarding the assessment of the body's fat compositions, various imaging techniques including dual energy X-ray absorptiometry (DXA), computed tomography (CT), and magnetic resonance imaging (MRI) are available to date. DXA can differentiate bone, fat, and lean soft tissue, while CT also enables determinations of the amount of fat depositions and adipose tissue volumes (22–24). MRI enables both qualitative and quantitative evaluation without ionizing radiation when compared to DXA and CT, and it makes the evaluation of more sophisticated parameters possible, which include assessments of regional body fat distributions and fat phenotyping (25, 26). Up to now, most studies investigated patho-anatomical features by means of conventional T1-weighted or T2-weighted MRI, thus primarily using qualitative analyses. However, advanced MRI-based techniques allowing quantitative assessments have been introduced, such as magnetic resonance spectroscopy (MRS) and chemical shift encoding-based water-fat MRI (25–27). Chemical shift encoding-based water-fat MRI represents a time-efficient, field map-insensitive technique to determine fat and water signal of the human body, thus making non-invasive quantification of water-fat compositions possible (28–32). In this context, determination of the proton density fat fraction (PDFF) derived from chemical shift encoding-based water-fat MRI is a reliable approach to extract quantitative information about fat compositions of different body compartments, with good concordance between PDFF measurements and histology or MRS (33, 34).
Despite its evident close spatial and functional relationships, evidence for interactions between lumbar vertebral body and paraspinal muscle fat compositions, investigated in-vivo by means of non-invasive, quantitative imaging, is mostly lacking. However, it is now technically possible to determine the PDFF of different compartments by means of chemical shift encoding-based water-fat MRI derived from the same imaging sequences, allowing intra-subject correlations. Thus, the present study systematically investigates the correlation between vertebral body and paraspinal muscle PDFF in a cohort of female subjects without spinal pathology.
Materials and Methods
Study Population
This study was approved by the Institutional Review Board. All subjects provided written informed consent prior to study inclusion.
Overall, 39 subjects were recruited. Inclusion criteria were age above 18 years, female gender, clear pre- or postmenopausal status based on a self-reported questionnaire, and physiological spine anatomy according to clinical examination and MRI. Exclusion criteria were any history of vertebral body fractures, any history of high-performance sports, LBP, NMD, anorexia nervosa, T2DM, or other metabolic diseases (except for osteopenia or osteoporosis), and general MRI contraindications. Enrolled subjects were divided into premenopausal and postmenopausal women for data analyses. The body height and weight of each subject was documented for the calculation of the body mass index (BMI), which was obtained by dividing the individual body weight by height squared.
Fifteen subjects (38.5%) were categorized as young, premenopausal women, whereas the remaining 24 subjects (61.5%) consisted of postmenopausal women. Within 1 month in relation to study inclusion, 22 postmenopausal women (91.7%) underwent a clinically indicated DXA exam (Prodigy, GE Healthcare, Chalfont St Giles, Buckinghamshire, UK), which was done in addition to the MRI scanning performed for this study's purposes. According to the resulting T-scores, 72.7% of these postmenopausal women had a normal bone status, while 18.2% and 9.1% of these women were classified as osteopenic and osteoporotic, respectively.
Magnetic Resonance Imaging
MRI was performed at 3T on the same scanner in all enrolled subjects (Ingenia, Philips Healthcare, Best, Netherlands). Subjects were positioned head-first in a supine position. During image acquisition, we used a built-in 12-channel posterior coil, a 16-channel anterior coil, and a whole-body coil.
For chemical shift encoding-based water-fat separation at the level of the lumbar spine, two sequences were acquired. For the later assessment of the paraspinal musculature, an axially-prescribed six-echo sequence was acquired with the following parameters: repetition time (TR)/echo time (TE)/ΔTE = 6.4/1.1/0.8 ms, voxel size = 3.2 × 2.0 × 4.0 mm3, field of view (FOV) = 220 × 401 × 252 mm3, frequency encoding direction = L/R, scan time = 1 min and 25 s. Regarding the assessment of bone marrow of vertebral bodies, a sagittally-prescribed eight-echo sequence was acquired with the following sequence adjustments: TR/TE/ΔTE = 11/1.4/1.1 ms, voxel size = 1.8 × 1.8 × 4.0 mm3, FOV = 220 × 220 × 80 mm3, frequency encoding direction = A/P (to minimize breathing artifacts), scan time = 1 min and 17 s. Both sequences acquired the echoes in a single TR using bipolar readout gradients. To reduce T1 bias effects, a flip angle of 3° was applied (28, 35).
Fat Quantification and Image Segmentation
First, the MRI data were processed online using the vendor's routines. Phase error correction and a complex-based water-fat decomposition considering a precalibrated seven-peak fat spectrum and a single T2* were performed within the context of the multi-echo mDIXON algorithm. The imaging-based PDFF maps were then the result of defining the ratio of the fat signal over the sum of fat and water signals.
Within the PDFF maps of each subject, manual regions of interest (ROIs) were carefully placed using MITK ([http://mitk.org/wiki/The_Medical_Imaging_Interaction_Toolkit_(MITK)]; German Cancer Research Center, Division of Medical and Biological Informatics, Medical Imaging Interaction Toolkit, Heidelberg, Germany). This was achieved separately for the following seven structures: right erector spinae muscle (ESR), left erector spinae muscle (ESL), vertebral bodies L1, L2, L3, L4 and L5. Segmentations were performed by a radiologist.
Concerning ROI drawing in the ESR and ESL, images derived from the axially-prescribed six-echo sequence were separately segmented from the upper endplate level of L2 to the lower endplate level of L5, with ROIs being placed at the muscle contour whilst avoiding the inclusion of subcutaneous fat or the muscle-fat interface (Figure 1). Regarding ROI definition in the vertebral bodies, images stemming from the sagittally-prescribed sequence were opened to place the ROIs in each sagittal slice for L1–L5 (Figure 2). The posterior elements and any sclerotic changes of the endplates were excluded.
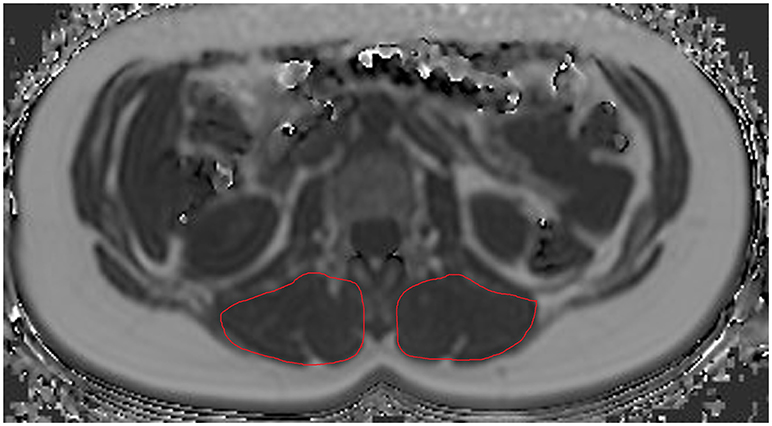
Figure 1. Region of interest (ROI) placement in the paraspinal compartment. Representative segmentation of the left erector spinae muscle (ESL) and right erector spinae muscle (ESR) in the proton density fat fraction (PDFF) map of a 39-year-old woman.
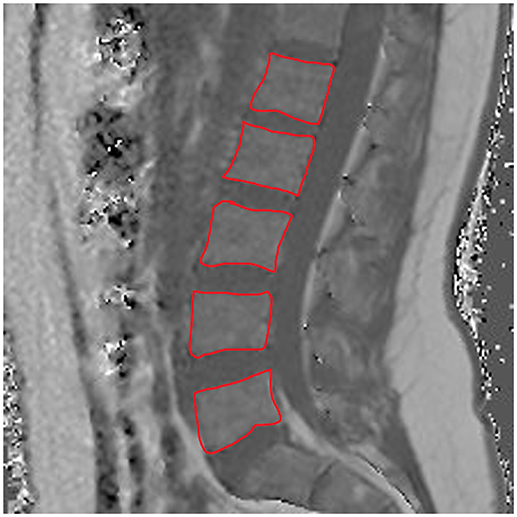
Figure 2. Region of interest (ROI) placement in the bone marrow compartment. Representative segmentation of lumbar vertebral bodies 1–5 (L1–L5) in the proton density fat fraction (PDFF) map of a 25-year-old woman.
Subsequent to ROI placements, PDFFs were extracted by averaging the values derived from the single ROIs placed in the consecutive slices. Furthermore, the PDFFs of the ESR and ESL were averaged to obtain one PDFF value per subject regarding the paraspinal muscle compartment. Analogously, the vertebral PDFFs of L1/L2, L2/L3, L3/L4, L4/L5, and L1–L5 were also averaged, resulting in segment-specific and total lumbar PDFF measurements for the bone marrow compartments per subject.
Statistical Analyses
SPSS (version 20.0; IBM SPSS Statistics for Windows, Armonk, NY, USA) and GraphPad Prism (version 6.04; GraphPad Software Inc., La Jolla, CA, USA) were used for statistical data analyses and graph generation. For all statistical tests, the level of significance was set at p < 0.05 (two-sided).
First, descriptive statistics including mean ± standard deviation (SD), median, minimum, and maximum were calculated for subject-related characteristics and the PDFF measurements of the paraspinal and bone marrow compartments, which was performed separately for pre- and postmenopausal women. To compare premenopausal vs. postmenopausal women in terms of subject characteristics and obtained PDFF measurements, Mann-Whitney tests were applied. Furthermore, coefficients of variation (CVs) were calculated as measures for dispersion regarding the PDFF measurements. Then, Pearson correlations were calculated considering age, BMI, PDFFs of the paraspinal muscle compartment, and PDFFs of the bone marrow compartment (segment-specific and total vertebral measurements), which was again achieved for pre- and postmenopausal women, respectively. Partial correlations with age and BMI as control variables were added to further investigate the correlations between the PDFF of the paraspinal and bone marrow compartments.
Results
Enrolled Subjects
MRI with chemical shift encoding-based water-fat separation was successfully acquired in all enrolled subjects. There was a statistically significant difference between both groups (pre- and postmenopausal women) in terms of age (premenopausal women: 29.9 ± 7.1 years, range: 21–42 years; postmenopausal women: 63.2 ± 6.3 years, range: 54–78 years; p < 0.001), but not regarding BMI (premenopausal women: 26.0 ± 1.6 kg/m2, range: 23.7–28.4 kg/m2; postmenopausal women: 25.7 ± 4.2 kg/m2, range: 18.8–35.3 kg/m2; p = 0.573).
Measurements of the PDFF
The average PDFF of the erector spinae muscles was 11.6 ± 2.9% (range: 5.2–15.6%) in premenopausal women and 24.6 ± 7.1% (range: 16.5–43.7%) in postmenopausal women (p < 0.001). Thus, we observed a CV of 24.3% in premenopausal women and a CV of 28.0% in postmenopausal women. Furthermore, in premenopausal women, the PDFF of the bone marrow of lumbar vertebral bodies was 28.8 ± 8.3% (range: 18.7–45.9%), whereas it was 47.2 ± 8.5% (range: 30.7–61.8%) in postmenopausal subjects (p < 0.001). Correspondingly, PDFF measurements showed a CV of 27.7% in premenopausal women and a CV of 17.6% in postmenopausal women. Representative PDFF maps of the erector spinae muscles and lumbar bone marrow derived from pre- and postmenopausal women are shown in Figure 3.
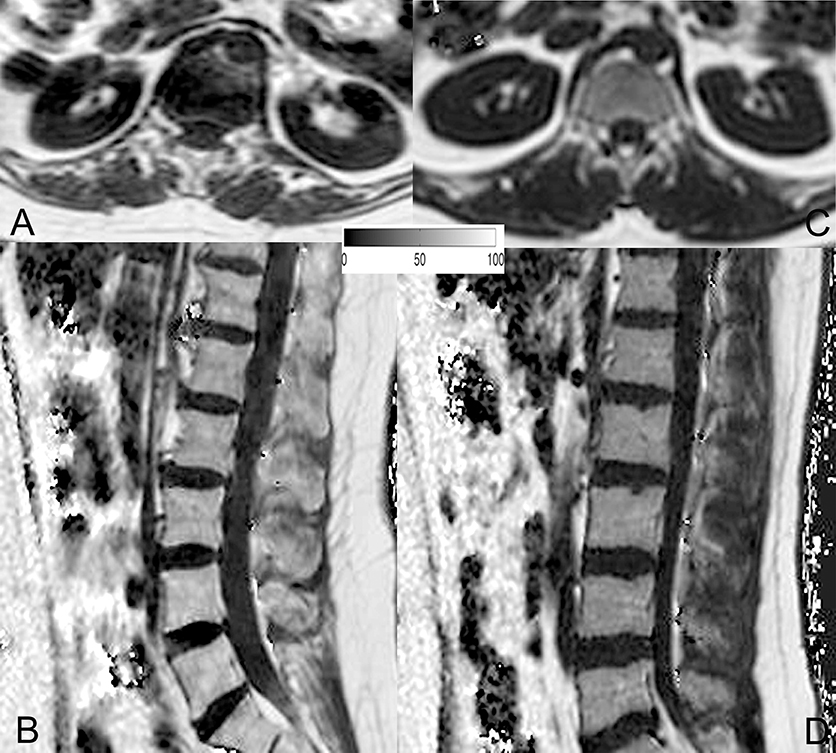
Figure 3. Representative proton density fat fraction (PDFF) maps. Representative axial and sagittal PDFF maps of the erector spinae muscles and the lumbar bone marrow of a postmenopausal woman [(A,B); age: 59 years; mean erector spinae muscle PDFF: 6.1%; mean L1–L5 bone marrow PDFF: 53.6%] and a premenopausal woman [(C,D); age: 28 years; mean erector spinae muscle PDFF: 4.5%; mean L1–L5 bone marrow PDFF: 42.7%] are shown in this figure.
Correlations Between the PDFF of the Paraspinal and Bone Marrow Compartments
In the cohort of premenopausal women, no statistically significant correlation was found for the PDFF of the erector spinae muscles and the PDFF of the bone marrow of lumbar vertebral bodies (p = 0.907). Correspondingly, partial correlation considering age and BMI as control variables did not result in a significant correlation either (p = 0.760).
In contrast to premenopausal women, a significant correlation between the PDFF of the paraspinal and bone marrow compartments was observed in postmenopausal women (L1–L5: r = 0.457, p = 0.025; Table 1). Furthermore, significant correlations were also revealed between the PDFF of the paraspinal compartment and different spinal segments (L1/L2: r = 0.422, p = 0.040; L2/L3: r = 0.502, p = 0.012; L3/L4: r = 0.496, p = 0.014; L4/L5: r = 0.406, p = 0.049). After inclusion of age and BMI as control variables, statistical significance was preserved for partial correlation (L1–L5: r = 0.472, p = 0.027).
Figure 4 plots the obtained PDFFs of the paraspinal against the bone marrow compartments for pre- and postmenopausal women, respectively.
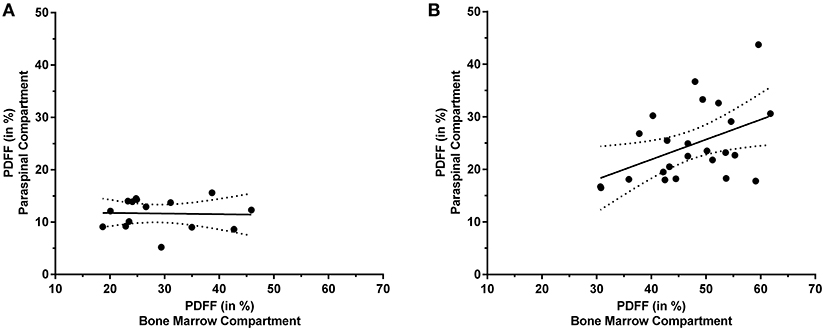
Figure 4. Proton density fat fraction (PDFF) of the paraspinal and bone marrow compartments. This figure plots the PDFF measurements of the paraspinal against the PDFF measurements of the bone marrow compartments for premenopausal women [(A); y = −0.012*x + 11.970] and postmenopausal women [(B); y = 0.380*x + 6.695], respectively. The areas between the dotted lines represent the 95% confidence band of the best-fit line.
Discussion
This study used chemical shift encoding-based water-fat MRI at the lumbar spine to extract PDFF measurements of paraspinal muscles (ESR and ESL) and vertebral bodies (L1–L5) in pre- and postmenopausal women. An age-dependent correlation between the PDFF of the paraspinal and bone marrow compartments was observed, with postmenopausal women showing statistically significant correlations even after consideration of age and BMI as control variables. Although several diseases have independently demonstrated to affect vertebral body or paraspinal muscle fat compositions, interactions between these compartments have not yet been investigated by means of advanced quantitative imaging in subjects without spinal diseases. However, close spatial and functional relationships between paraspinal musculature and the vertebral column are evident, most prominently reflected by major interactions in terms of body movement, spine stabilization, and balance keeping (11, 12).
Subjects diagnosed with osteoporosis have shown to harbor significantly elevated BMF when compared to healthy individuals (25, 26, 36, 37). Griffith et al. used MRS to investigate associations between vertebral bone marrow fat and osteoporosis, with significantly increased BMF in osteoporotic subjects (1, 2). More recently, Kuhn et al. were able to show that the PDFF was greater in osteoporotic than in healthy vertebrae (38). It has also been shown repeatedly that with increasing age, especially females are prone to accelerated fatty conversion of the bone marrow compared to males, with the menopause potentially representing the main trigger (39–42). The finding of reduced bone mineral density and high incidences of vertebral fragility fractures particularly among postmenopausal women on the one hand and clear changes in BMF on the other hand suggests strong correlations between vertebral bone marrow composition and osteoporosis (37, 43, 44). In addition, osteoporosis has also demonstrated to have associations with changes in composition of paraspinal musculature: Kim et al. used MRI in postmenopausal women, showing that vertebral fractures were associated with profound changes of paraspinal musculature, represented by reductions of the cross sectional area and increased MFI (19). The study of Kim et al. applied semi-quantitative measurements with three visual scale grades (19).
Taken together, especially postmenopausal women seem to be predisposed for investigations of interactions between vertebrae and paraspinal musculature. Indeed, we found significant correlations between the PDFF of paraspinal muscles and vertebral bodies in our cohort. Furthermore, in contrast to the investigation of Kim et al., correlations were observed using more advanced, high-resolution MRI with chemical shift encoding-based water-fat separation, thus allowing multi-segmental evaluations of the PDFF, a parameter that has not been evaluated in their study (19). More importantly, results were obtained in postmenopausal women without vertebral fractures, thus providing first evidence for associations between vertebral body and paraspinal muscle fat compositions in subjects without any history of spinal fractures.
Other conditions have also proven impact on bone marrow composition (25, 26, 36). In T2DM, vertebral BMF was comparable between diabetic postmenopausal women and healthy subjects; however, mean unsaturation levels were significantly lower in subjects diagnosed with T2DM, and significant correlations of vertebral BMF content with adjusted visceral adipose tissue and HbA1c were observed in these subjects (3). Similarly, T2DM in postmenopausal women was associated with lower unsaturation and higher saturation levels, with diabetic subjects having fragility fractures showing the lowest marrow unsaturation and highest saturation (4). Furthermore, degenerative changes at the spine and LBP demonstrated relationships to alterations in muscle composition, with paraspinal MFI being associated with LBP, and disc degeneration and paraspinal muscle atrophy being positively correlated (14, 16). Again, correlations of the paraspinal muscle and bone marrow compartments in terms of quantitative BMF evaluations have not been routinely achieved yet. Significant positive correlations between vertebral body and paraspinal muscle PDFF measurements, as observed in the present study among postmenopausal women, might also be observed in these conditions, with the PDFF then potentially serving as an easily assessable, non-invasive biomarker of clinically relevant changes in fat content. However, future studies enrolling patients with such diseases have to clarify the role of correlations between PDFF measurements derived from different fat compartments at the level of the lumbar spine.
As aforementioned, we found a significant correlation between the PDFF of the paraspinal and bone marrow compartments in postmenopausal women, but not in young, premenopausal women. The reason for this finding might be related to the observation that for premenopausal women, the PDFF ranges were relatively small, thus indicating rather homogeneous PDFF distributions in young women. In contrast, the PDFF ranges were considerably wider in postmenopausal women, most probably reflecting more distinct changes of fat compositions of both paraspinal musculature and vertebral bone marrow. In this context, it has already been revealed that a relevant age-dependent increase in fatty conversion is present particularly in women (39–42). However, exploration of the distinct biological causes for the observed results goes beyond the scope of this imaging study and has to be the purpose of future investigations.
When interpreting the results of the present study, we also have to bear some limitations in mind. First, the comparatively small cohort size has to be regarded as a main limitation. Thus, the results presented have to be interpreted with caution and should be confirmed by follow-up studies enrolling more subjects. In this context, the absence of a statistically significant correlation in young, premenopausal women could be related to the comparatively low number of subjects assigned to this group. Hence, future studies might focus on larger cohorts including particularly more premenopausal women to clarify whether the subtler changes of vertebral body and paraspinal muscle fat compositions in younger years truly do not show significant correlations. Second, the enrolment of women only might be regarded as a limitation, and further studies might explore potential correlations of PDFF measurements among men. Previous studies indicated clear sex differences in terms of vertebral body and paraspinal muscle fat compositions (39–42). Therefore, separate, sex-specific analyses seem justified, with this study focusing on women first. The results of the present study provide a good basis for further exploration of PDFF correlations in both sexes. Third, we did not include subjects suffering from metabolic diseases such as T2DM. As a consequence, upcoming investigations may distinctly explore correlations in pathological conditions to determine whether vertebral body and paraspinal muscle PDFFs and their correlations might serve as biomarkers in the clinical setting. Additionally, interventions should be performed to evaluate whether physical exercise or medication, for instance, might have an effect upon the correlations between vertebral body and paraspinal muscle PDFF measurements. Effects have been observed regarding the one or the other compartment in a limited amount of studies so far (45–47). Moreover, it would be interesting to further explore the distribution of lipids within the paraspinal muscles and to distinctly quantify the intramyocellular lipid and extramyocellular lipid levels. However, such investigations would require the acquisition of MRS, which was not available for the present study.
Conclusion
Using chemical shift encoding-based water-fat MRI at the lumbar spine for measurements of PDFF, this study revealed significant correlations between the PDFF of paraspinal muscles and the PDFF of vertebral bodies in postmenopausal women. The present investigation suggests a potentially close and clinically relevant relationship between spatially and functionally related compartments at the level of the lumbar spine in terms of fat compositions. However, follow-up studies are needed to further evaluate such correlations in larger cohorts, particularly consisting of premenopausal women as well as male subjects, in order to further explore clinical value and reliability of the reported correlations in terms of a potential role as biomarkers. Moreover, the distinct biological causes for the observed correlations between the PDFF of paraspinal and vertebral bone marrow compartments go beyond the scope of the present study and have yet to be elucidated.
Author Contributions
TB, DK, and JK designed the study. MD, SS, AR, JS, MND, DW, SR, EK, and DF coordinated subject enrolment, image acquisition, and data storage. NS, MD, SS, JS, and TB were responsible for data analysis, NS and TB performed statistics. NS, SS, JK, DK, and TB were involved in literature research. NS, MD, SS, AR, JS, MND, DW, SR, EK, DF, ER, CZ, JK, DK, and TB drafted the manuscript. The study was supervised by ER, CZ, JK, DK, and TB. All authors reviewed the manuscript before submission.
Funding
This work was supported by a TUM Faculty of Medicine KKF grant H01 (TB). Furthermore, the present work was supported by the European Research Council (grant agreement No 677661- ProFatMRI [DK] and grant agreement No 637164 - iBack [JK]) and Philips Healthcare.
Conflict of Interest Statement
JK received speaker honoraria from Philips Healthcare. DK received grant support from Philips Healthcare.
The remaining authors declare that the research was conducted in the absence of any commercial or financial relationships that could be construed as a potential conflict of interest.
Acknowledgments
The authors would like to thank Mrs. Marianne Schoenbach-Engmann for proofreading the manuscript.
Abbreviations
BMF, bone marrow fat; BMI, body mass index; CT, computed tomography; CV, coefficient of variation; DXA, dual energy X-ray absorptiometry; ESL, left erector spinae muscle; ESR, right erector spinae muscle; FOV, field of view; LBP, low back pain; MFI, muscle fat infiltration; MRI, magnetic resonance imaging; MRS, magnetic resonance spectroscopy; NMD, neuromuscular disease; PDFF, proton density fat fraction; ROI, region of interest; SD, standard deviation; T2DM, type 2 diabetes mellitus; TE, echo time; TR, repetition time.
References
1. Griffith JF, Yeung DK, Antonio GE, Lee FK, Hong AW, Wong SY, et al. Vertebral bone mineral density, marrow perfusion, and fat content in healthy men and men with osteoporosis: dynamic contrast-enhanced MR imaging and MR spectroscopy. Radiology (2005) 236:945–51. doi: 10.1148/radiol.2363041425
2. Griffith JF, Yeung DK, Antonio GE, Wong SY, Kwok TC, Woo J, et al. Vertebral marrow fat content and diffusion and perfusion indexes in women with varying bone density: MR evaluation. Radiology (2006) 241:831–8. doi: 10.1148/radiol.2413051858
3. Baum T, Yap SP, Karampinos DC, Nardo L, Kuo D, Burghardt AJ, et al. Does vertebral bone marrow fat content correlate with abdominal adipose tissue, lumbar spine bone mineral density, and blood biomarkers in women with type 2 diabetes mellitus? J Magn Reson Imaging (2012) 35:117–24. doi: 10.1002/jmri.22757
4. Patsch JM, Li X, Baum T, Yap SP, Karampinos DC, Schwartz AV, et al. Bone marrow fat composition as a novel imaging biomarker in postmenopausal women with prevalent fragility fractures. J Bone Miner Res. (2013) 28:1721–8. doi: 10.1002/jbmr.1950
5. Yu EW, Greenblatt L, Eajazi A, Torriani M, Bredella MA. Marrow adipose tissue composition in adults with morbid obesity. Bone (2017) 97:38–42. doi: 10.1016/j.bone.2016.12.018
6. Bredella MA, Fazeli PK, Miller KK, Misra M, Torriani M, Thomas BJ, et al. Increased bone marrow fat in anorexia nervosa. J Clin Endocrinol Metab. (2009) 94:2129–36. doi: 10.1210/jc.2008-2532
7. Wehrli FW, Hopkins JA, Hwang SN, Song HK, Snyder PJ, Haddad JG. Cross-sectional study of osteopenia with quantitative MR imaging and bone densitometry. Radiology (2000) 217:527–38. doi: 10.1148/radiology.217.2.r00nv20527
8. Schellinger D, Lin CS, Lim J, Hatipoglu HG, Pezzullo JC, Singer AJ. Bone marrow fat and bone mineral density on proton MR spectroscopy and dual-energy X-ray absorptiometry: their ratio as a new indicator of bone weakening. AJR Am J Roentgenol. (2004) 183:1761–5. doi: 10.2214/ajr.183.6.01831761
9. Schwartz AV, Sigurdsson S, Hue TF, Lang TF, Harris TB, Rosen CJ, et al. Vertebral bone marrow fat associated with lower trabecular BMD and prevalent vertebral fracture in older adults. J Clin Endocrinol Metab. (2013) 98:2294–300. doi: 10.1210/jc.2012-3949
10. Justesen J, Stenderup K, Ebbesen EN, Mosekilde L, Steiniche T, Kassem M. Adipocyte tissue volume in bone marrow is increased with aging and in patients with osteoporosis. Biogerontology (2001) 2:165–71. doi: 10.1023/A:1011513223894
11. Hicks GE, Simonsick EM, Harris TB, Newman AB, Weiner DK, Nevitt MA, et al. Cross-sectional associations between trunk muscle composition, back pain, and physical function in the health, aging and body composition study. J Gerontol A Biol Sci Med Sci. (2005) 60:882–7. doi: 10.1093/gerona/60.7.882
12. Hansen L, de Zee M, Rasmussen J, Andersen TB, Wong C, Simonsen EB. Anatomy and biomechanics of the back muscles in the lumbar spine with reference to biomechanical modeling. Spine (2006) 31:1888–99. doi: 10.1097/01.brs.0000229232.66090.58
13. Karampinos DC, Baum T, Nardo L, Alizai H, Yu H, Carballido-Gamio J, et al. Characterization of the regional distribution of skeletal muscle adipose tissue in type 2 diabetes using chemical shift-based water/fat separation. J Magn Reson Imaging (2012) 35:899–907. doi: 10.1002/jmri.23512
14. Teichtahl AJ, Urquhart DM, Wang Y, Wluka AE, Wijethilake P, O'Sullivan R, et al. Fat infiltration of paraspinal muscles is associated with low back pain, disability, and structural abnormalities in community-based adults. Spine J. (2015) 15:1593–601. doi: 10.1016/j.spinee.2015.03.039
15. Elliott JM, Courtney DM, Rademaker A, Pinto D, Sterling MM, Parrish TB. The rapid and progressive degeneration of the cervical multifidus in whiplash: an MRI study of fatty infiltration. Spine (2015) 40:E694–700. doi: 10.1097/BRS.0000000000000891
16. Sun D, Liu P, Cheng J, Ma Z, Liu J, Qin T. Correlation between intervertebral disc degeneration, paraspinal muscle atrophy, and lumbar facet joints degeneration in patients with lumbar disc herniation. BMC Musculoskelet Disord. (2017) 18:167. doi: 10.1186/s12891-017-1522-4
17. Dahlqvist JR, Vissing CR, Thomsen C, Vissing J. Severe paraspinal muscle involvement in facioscapulohumeral muscular dystrophy. Neurology (2014) 83:1178–83. doi: 10.1212/WNL.0000000000000828
18. Janssen BH, Voet NB, Nabuurs CI, Kan HE, de Rooy JW, Geurts AC, et al. Distinct disease phases in muscles of facioscapulohumeral dystrophy patients identified by MR detected fat infiltration. PloS ONE (2014) 9:e85416. doi: 10.1371/journal.pone.0085416
19. Kim JY, Chae SU, Kim GD, Cha MS. Changes of paraspinal muscles in postmenopausal osteoporotic spinal compression fractures: magnetic resonance imaging study. J Bone Metab. (2013) 20:75–81. doi: 10.11005/jbm.2013.20.2.75
20. Goodpaster BH, Carlson CL, Visser M, Kelley DE, Scherzinger A, Harris TB, et al. Attenuation of skeletal muscle and strength in the elderly: the health ABC study. J Appl Physiol. (2001) 90:2157–65. doi: 10.1152/jappl.2001.90.6.2157
21. Goodpaster BH, Park SW, Harris TB, Kritchevsky SB, Nevitt M, Schwartz AV, et al. The loss of skeletal muscle strength, mass, and quality in older adults: the health, aging and body composition study. J Gerontol A Biol Sci Med Sci. (2006) 61:1059–64. doi: 10.1093/gerona/61.10.1059
22. Petak S, Barbu CG, Yu EW, Fielding R, Mulligan K, Sabowitz B, et al. The official positions of the International Society for Clinical Densitometry: body composition analysis reporting. J Clin Densitom. (2013) 16:508–19. doi: 10.1016/j.jocd.2013.08.018
23. Wang H, Chen YE, Eitzman DT. Imaging body fat: techniques and cardiometabolic implications. Arterioscler Thromb Vasc Biol. (2014) 34:2217–23. doi: 10.1161/ATVBAHA.114.303036
24. Seabolt LA, Welch EB, Silver HJ. Imaging methods for analyzing body composition in human obesity and cardiometabolic disease. Ann N Y Acad Sci. (2015) 1353:41–59. doi: 10.1111/nyas.12842
25. Baum T, Cordes C, Dieckmeyer M, Ruschke S, Franz D, Hauner H, et al. MR-based assessment of body fat distribution and characteristics. Eur J Radiol. (2016) 85:1512–8. doi: 10.1016/j.ejrad.2016.02.013
26. Paccou J, Penel G, Chauveau C, Cortet B, Hardouin P. Marrow adiposity and bone: review of clinical implications. Bone (2018). doi: 10.1016/j.bone.2018.02.008. [Epub ahead of print].
27. Karampinos DC, Ruschke S, Dieckmeyer M, Diefenbach M, Franz D, Gersing AS, et al. Quantitative MRI and spectroscopy of bone marrow. J Magn Reson Imaging (2018) 47:332–53. doi: 10.1002/jmri.25769
28. Karampinos DC, Yu H, Shimakawa A, Link TM, Majumdar S. T(1)-corrected fat quantification using chemical shift-based water/fat separation: application to skeletal muscle. Magn Reson Med. (2011) 66:1312–26. doi: 10.1002/mrm.22925
29. Reeder SB, Hu HH, Sirlin CB. Proton density fat-fraction: a standardized MR-based biomarker of tissue fat concentration. J Magn Reson Imaging (2012) 36:1011–4. doi: 10.1002/jmri.23741
30. Hu HH, Kan HE. Quantitative proton MR techniques for measuring fat. NMR Biomed. (2013) 26:1609–29. doi: 10.1002/nbm.3025
31. Baum T, Yap SP, Dieckmeyer M, Ruschke S, Eggers H, Kooijman H, et al. Assessment of whole spine vertebral bone marrow fat using chemical shift-encoding based water-fat MRI. J Magn Reson Imaging (2015) 42:1018–23. doi: 10.1002/jmri.24854
32. Ruschke S, Pokorney A, Baum T, Eggers H, Miller JH, Hu HH, et al. Measurement of vertebral bone marrow proton density fat fraction in children using quantitative water-fat MRI. MAGMA (2017) 30:449–60. doi: 10.1007/s10334-017-0617-0
33. Fischer MA, Nanz D, Shimakawa A, Schirmer T, Guggenberger R, Chhabra A, et al. Quantification of muscle fat in patients with low back pain: comparison of multi-echo MR imaging with single-voxel MR spectroscopy. Radiology (2013) 266:555–63. doi: 10.1148/radiol.12120399
34. Smith AC, Parrish TB, Abbott R, Hoggarth MA, Mendoza K, Chen YF, et al. Muscle-fat MRI: 1.5 Tesla and 3.0 Tesla versus histology. Muscle Nerve (2014) 50:170–6. doi: 10.1002/mus.24255
35. Liu CY, McKenzie CA, Yu H, Brittain JH, Reeder SB. Fat quantification with IDEAL gradient echo imaging: correction of bias from T(1) and noise. Magn Reson Med. (2007) 58:354–64. doi: 10.1002/mrm.21301
36. Cordes C, Baum T, Dieckmeyer M, Ruschke S, Diefenbach MN, Hauner H, et al. MR-based assessment of bone marrow fat in osteoporosis, diabetes, and obesity. Front Endocrinol. (2016) 7:74. doi: 10.3389/fendo.2016.00074
37. Rosen CJ, Bouxsein ML. Mechanisms of disease: is osteoporosis the obesity of bone? Nat Clin Pract Rheumatol. (2006) 2:35–43. doi: 10.1038/ncprheum0070
38. Kuhn JP, Hernando D, Meffert PJ, Reeder S, Hosten N, Laqua R, et al. Proton-density fat fraction and simultaneous R2* estimation as an MRI tool for assessment of osteoporosis. Eur Radiol. (2013) 23:3432–9. doi: 10.1007/s00330-013-2950-7
39. Baum T, Rohrmeier A, Syvari J, Diefenbach MN, Franz D, Dieckmeyer M, et al. Anatomical variation of age-related changes in vertebral bone marrow composition using chemical shift encoding-based water-fat magnetic resonance imaging. Front Endocrinol (Lausanne). (2018) 9:141. doi: 10.3389/fendo.2018.00141
40. Griffith JF, Yeung DK, Ma HT, Leung JC, Kwok TC, Leung PC. Bone marrow fat content in the elderly: a reversal of sex difference seen in younger subjects. J Magn Reson Imaging (2012) 36:225–30. doi: 10.1002/jmri.23619
41. Ishijima H, Ishizaka H, Horikoshi H, Sakurai M. Water fraction of lumbar vertebral bone marrow estimated from chemical shift misregistration on MR imaging: normal variations with age and sex. AJR Am J Roentgenol. (1996) 167:355–8. doi: 10.2214/ajr.167.2.8686603
42. Kugel H, Jung C, Schulte O, Heindel W. Age- and sex-specific differences in the 1H-spectrum of vertebral bone marrow. J Magn Reson Imaging (2001) 13:263–8. doi: 10.1002/1522-2586(200102)13:2<263::AID-JMRI1038>3.0.CO;2-M
43. Sanchez-Riera L, Wilson N, Kamalaraj N, Nolla JM, Kok C, Li Y, et al. Osteoporosis and fragility fractures. Best Pract Res Clin Rheumatol. (2010) 24:793–810. doi: 10.1016/j.berh.2010.10.003
44. Compston JE, Flahive J, Hooven FH, Anderson FA Jr, Adachi JD, Boonen S, et al. Obesity, health-care utilization, and health-related quality of life after fracture in postmenopausal women: Global Longitudinal Study of Osteoporosis in Women (GLOW). Calcif Tissue Int. (2014) 94:223–31. doi: 10.1007/s00223-013-9801-z
45. Syed FA, Oursler MJ, Hefferanm TE, Peterson JM, Riggs BL, Khosla S. Effects of estrogen therapy on bone marrow adipocytes in postmenopausal osteoporotic women. Osteoporos Int. (2008) 19:1323–30. doi: 10.1007/s00198-008-0574-6
46. Styner M, Thompson WR, Galior K, Uzer G, Wu X, Kadari S, et al. Bone marrow fat accumulation accelerated by high fat diet is suppressed by exercise. Bone (2014) 64:39–46. doi: 10.1016/j.bone.2014.03.044
Keywords: bone marrow, chemical shift encoding-based water-fat imaging, lumbar spine, muscle fat, paraspinal musculature, proton density fat fraction
Citation: Sollmann N, Dieckmeyer M, Schlaeger S, Rohrmeier A, Syvaeri J, Diefenbach MN, Weidlich D, Ruschke S, Klupp E, Franz D, Rummeny EJ, Zimmer C, Kirschke JS, Karampinos DC and Baum T (2018) Associations Between Lumbar Vertebral Bone Marrow and Paraspinal Muscle Fat Compositions—An Investigation by Chemical Shift Encoding-Based Water-Fat MRI. Front. Endocrinol. 9:563. doi: 10.3389/fendo.2018.00563
Received: 15 June 2018; Accepted: 04 September 2018;
Published: 28 September 2018.
Edited by:
Xijie Yu, West China Hospital of Sichuan University, ChinaReviewed by:
Janet M. Hock, Tufts University, United StatesGuojing Luo, Sichuan University, China
Guanwu Li, Shanghai University of Traditional Chinese Medicine, China
Copyright © 2018 Sollmann, Dieckmeyer, Schlaeger, Rohrmeier, Syvaeri, Diefenbach, Weidlich, Ruschke, Klupp, Franz, Rummeny, Zimmer, Kirschke, Karampinos and Baum. This is an open-access article distributed under the terms of the Creative Commons Attribution License (CC BY). The use, distribution or reproduction in other forums is permitted, provided the original author(s) and the copyright owner(s) are credited and that the original publication in this journal is cited, in accordance with accepted academic practice. No use, distribution or reproduction is permitted which does not comply with these terms.
*Correspondence: Nico Sollmann, Tmljby5Tb2xsbWFubkB0dW0uZGU=