- Key Laboratory of Endocrinology, Department of Endocrinology, Translational Medicine Center, Ministry of Health, Peking Union Medical College Hospital, Peking Union Medical College, Chinese Academy of Medical Sciences, Beijing, China
Maternal over-nutrition can lead to metabolic disorders in offspring, whereas maternal dietary genistein may have beneficial effects on the metabolic health of offspring. Our objective was to determine whether maternal dietary genistein could attenuate the detrimental effects of a maternal high-fat diet on their offspring's metabolism and to explore the role of the gut microbiota on their offspring's glucose and lipid metabolism. C57BL/6 female mice were fed either a high-fat diet without genistein (HF), high-fat diet with low-dose genistein (0.25 g/kg diet) (HF.LG), high-fat diet with high-dose genistein (0.6 g/kg diet) (HF.HG) or normal control diet (Control) for 3 weeks prior to breeding and throughout gestation and lactation. The female offspring in the HF group had lower birth weights and glucose intolerance and higher serum insulin, triacylglycerol (TG) and total cholesterol (TC) levels at weaning compared with the Control group. Offspring from HF.LG dams had increased birth weight, improved glucose tolerance, and decreased fasting insulin, whereas the serum TG and TC levels were decreased in HF.HG offspring in comparison with HF offspring. The significant enrichment of Bacteroides and Akkermansia in offspring from genistein-fed dams might play vital roles in improving glucose homeostasis and insulin sensitivity, and the significantly increased abundance of Rikenella and Rikenellaceae_RC9_ gut_group in the HF.HG group may be associated with the decreased serum levels of TG and TC. In conclusion, maternal dietary genistein negates the harmful effects of a maternal high-fat diet on glucose and lipid metabolism in female offspring, in which the altered gut microbiota plays crucial roles. The ability of maternal genistein intake to improve offspring metabolism is important since this intervention could fight the transmission of diabetes to subsequent generations.
Introduction
Obesity and type 2 diabetes mellitus (T2DM) are highly prevalent and lead to tremendous health and economic burdens. However, the etiology and pathogenesis of obesity and diabetes are still unclear. Since the developmental origins of health and disease (DOHaD) hypothesis was first put forward in the early 1990s (1), a large number of epidemiological investigations (2–4) and animal studies (5, 6) have highlighted the importance of the developmental environment in early life in determining the trajectories of chronic disease in later life, including obesity and T2DM. Numerous recent studies (7, 8) and our previous research (9) have shown that a maternal high-fat diet during pregnancy and lactation can significantly increase the susceptibility of offspring to obesity, glucose intolerance and insulin resistance. Thus, interventions during early life may reset the disease trajectories and prevent the onset and development of diabetes.
Several large epidemiological studies have shown that intake of soy foods and isoflavones are associated with a lower risk of T2DM (10–12). Soy isoflavones have a weak estrogen-like effect, and the main components of soy isoflavones include genistein, diadzein, and glycitein. Genistein has been widely used as a dietary supplement in the United States and has been explored for the potential effects in cognitive function, cancer therapy, and bone and cardiovascular health (13). In recent years, a growing number of studies have shown that genistein improves glucose and lipid metabolism and have demonstrated that genistein intake reduces the levels of blood glucose, triglycerides (TG) and total cholesterol (TC) as well as prevents weight gain, without side adverse effects (14–16). Modulating the hepatic glucose output, enhancing β-cell proliferation, reducing apoptosis, activating the cAMP/PKA signaling pathway and antioxidant effects are all potential mechanisms for the anti-diabetic functions of genistein (13). However, currently, studies exploring the effects of genistein intervention in early life on glucose and lipid metabolism are rare.
During the last few decades, the gut microbiota has become a focus of medical research. Numerous lines of evidence (17–19) have suggested that the gut microbiota plays an important role in glucose and lipid metabolism. Recently, a growing number of human (20) and animal studies (1, 21, 22) have indicated that the gut microbiota is disordered in offspring from obese mothers and high-fat fed dams. Thus, the gut microbiota may play a pivotal role in a poor maternal intrauterine growth environment, programming the offspring to develop metabolic disturbances. Furthermore, an association between the genistein improvement of glucose tolerance and alterations of the gut microbiota has been shown (23). However, investigations into the effects of maternal genistein intervention on the gut microbiota in offspring are limited.
In the current study, we aimed to research the effects of maternal dietary genistein on metabolic health in the early life of female offspring and determine whether maternal genistein intake could reverse the detrimental metabolic effects of a maternal high-fat diet in female offspring. In addition, we explored the role of the gut microbiota on offspring glucose and lipid metabolism.
Materials and Methods
Animals and Study Design
Four-week-old C57BL/6 female mice were obtained from the National Institutes for Food and Drug Control (Beijing, China; SCXK-2014-0013). Animals were maintained in controlled animal facilities at a room temperature of 22 ± 2°C with a 12 h light/dark cycle and were fed a normal control diet (AIN-93G diet) with corn oil substituted for soybean oil. After 1 week of environmental acclimatization, dams were randomly divided into four groups and were fed a high-fat diet without genistein (HF, n = 6), high-fat diet with low-dose genistein (CAS: 466-72-0, G0272, TCI Development Co., Ltd.) (0.25 g/kg diet) (HF.LG, n = 6), high-fat diet with high-dose genistein (0.6 g/kg diet) (HF.HG, n = 8) or normal control diet (Control, n = 8) for 3 weeks. The soybean oil in the high-fat diet was also substituted by corn oil. The ingredients are shown in Table S1. The high-fat diet included (kcal %): fat, 60%; carbohydrate, 20%; and protein, 20%, with a 5.24 kcal/g energy supply, whereas the control diet contained (kcal %): fat, 15.8%; carbohydrate, 63.9%; and protein, 20%, with a 3.9 kcal/g energy supply.
Female mice were mated to 8-week-old C57BL/6 males and fed a normal diet. The dams were checked for postcopulatory plugs every morning after mating, and the appearance of a plug was recorded as d 0.5 of pregnancy. Females were fed their assigned diet during pregnancy and lactation and had access to food and water ad libitum. The litters were all culled to five pups to ensure that there was no nutritional bias between litters. Offspring were weaned at 3 weeks of age. At weaning, all female offspring (n = 6–8 per group) were sacrificed. Blood samples were collected from the intraorbital retrobulbar plexus after 10 h of fasting from anesthetized mice, and the uterus and ovaries were removed and weighed; the cecal contents were quickly removed, snap frozen in dry ice, and then stored at −80°C for further analysis. All operations were conducted under chloral hydrate anesthesia, and best efforts were done to minimize suffering. All of the procedures were approved by the animal care and use committee of the Peking Union Medical College Hospital (Beijing, China, SYXC-2014-0029). All of the animal operations were conducted in compliance with the Guide for the Care and Use of Laboratory Animals.
Measurement of Body Weight and Food Intake
The body weights of both the mother and offspring were measured once per week. We measured the 3-day food intake each week by the mother, and their food consumption was estimated by weighing the remaining food.
Glucose Tolerance Tests
Oral glucose tolerance tests (OGTT) were performed on both dams and their female offspring at weaning. Mice were fasted for 6 h. Then, a glucose load (2.0 g/kg body weight) was given by gavage. Before (0 min) and at 30, 60, and 120 min after the gavage, the blood glucose (BG) concentration was measured in blood collected from a tail bleed using a Contour TS glucometer (ACCU-CHEK Mobile, Beijing, China). The area under the curve (AUC) of the OGTT was calculated as previously described (9).
Measurement of Serum Insulin, Triacylglycerol, and Total Cholesterol Levels
The blood samples collected from female offspring at weaning were centrifuged at 3,000 × g for 10 min at 4°C, and the serum was stored in aliquots at −80°C. The serum insulin concentrations were measured using an ELISA kit (80-INSMSU-E01, Salem, NH, USA). Insulin sensitivity was assessed using the homeostasis model assessment of insulin resistance (HOMA-IR). The HOMA-IR was calculated as previously described (9). Serum total cholesterol (TC) (K603-100, kits were from BioVision, Inc., Mountain View, CA, USA) and triacylglycerol (TG) (K622-100, kits were from BioVision Inc., Mountain View, CA, USA) were measured by colorimetric methods.
Gut Microbiota Analysis
The gut microbiota was analyzed according to the methods described in our previous publication (24). Microbial DNA was extracted from the cecal content using a QIAamp DNA Stool Mini Kit (Qiagen, Hilden, Germany). The V3-V4 regions of the 16S rRNA gene were amplified using the primers 341F 5′-CCTAYGGGRBGCASCAG-3′ and 806R, 5′-GGACTACNNGG GTATCTAAT-3′. Amplicons were purified using a quick PCR purification kit (Qiagen, Hilden, Germany). Microbial 16S rDNA was sequenced on the Illumina HiSeq 2500 platform (Norcross, GA, USA).
After merging paired-end reads, reads were performed by quality filtering. High quality reads were assigned to operational taxonomic units (OTUs) at the 97% similarity level using UPARSE software (version 7.0.1001) (25), and representative sequences for each OTU were screened using QIIME software (version 1.7.0, Quantitative Insights into Microbial Ecology) (26). Then, the GreenGene Database (27) was used to annotate taxonomic information based on the RDP classifier version 2.2 algorithm (28). The relative abundance of each OTU was analyzed at the phylum, class, order, family, genus and species levels. Alpha and beta diversity were examined using QIIME software (Version 1.7.0) and calculated with R software (Version 2.15.3). For alpha diversity, Chao1, Simpson and the Shannon index were analyzed. For beta diversity, principal component analysis (PCA) plots were performed using both weighted and unweighted UniFrac. In addition, linear discriminant analysis (LDA) of the effect size (LEfSe) and MetaStat were used to determine differences among the groups.
Statistical Analysis
The results are expressed as the mean ± standard error of the mean (S.E.M). The statistics were analyzed by one-way ANOVA and two-way ANOVA, with Tukey and Bonferroni post-hoc analyses. Correlations between the relative abundance of bacterial taxa at different taxonomic levels and metabolic parameters were performed by Spearman correlation coefficient test. Correction for correlation analysis by false discovery rate (FDR) with the Benjamini-Hochberg procedure were displayed by R software (Version 2.15.3) with values of < 0.05 considered statistical significance. Analysis of similarities (ANOSIM) was used to test the statistical significance for β diversity. A p < 0.05 was considered statistically significant. For the MetaStat analysis, a q < 0.05 was considered statistical significance. Prism version 7.0 (GraphPad Software Inc., San Diego, CA, USA) was used for statistical analysis.
Results
Characterization of Dams
During the 9-week of dietary intervention, the energy intake of dams among the four groups was not significantly different. At weaning, the body weights of high-fat fed dams (HF) were higher than that of dams in the control group (p < 0.0001; Table 1). The AUC of the OGTT was significantly larger in dams fed a high-fat diet compared to that of dams in the control group (p < 0.05). However, in contrast to the HF group, the body weights and glucose tolerance were not significantly different in dams fed either a high-fat diet with low-dose genistein (HF.LG) or high-fat diet with high-dose genistein (HF.HG). There was no difference among the four groups in regard to litter size, uterus index (weight ratio of uterus to body weight) or ovary index (weight ratio of ovaries to body weight) of dams.
Birth Weight and Body Weight of Offspring
The birth weights of offspring of high-fat fed dams (HF) were lower than those of offspring of control group dams (p < 0.05, Figure 1A). In offspring of high-fat diet with low-dose genistein dams, the birth weights improved and were higher than those of offspring of HF group dams (p < 0.05, Figure 1A). However, there was no difference among the four groups in regard to the body weight of female offspring at weaning (Figure 1B).
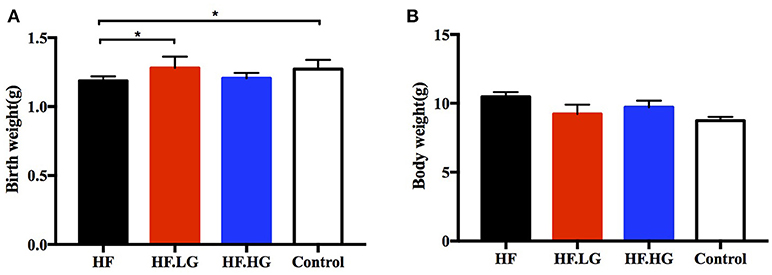
Figure 1. Birth weight and body weight at weaning in offspring. (A) Birth weight, and (B) Body weight of female offspring at weaning. HF, high-fat diet without genistein; HF. LG, high-fat diet with low-dose genistein; HF. HG, high-fat diet with high-dose genistein; Control, normal control diet. Data are expressed as means ± S.E.M. (n = 6–8/group). Mean values were significantly different between other group and the HF group: *p < 0.05.
Dietary Low-Dose Genistein Prevents the Deleterious Effects of a Maternal High-Fat Diet on Glucose Metabolism and Insulin Sensitivity of the Offspring
At weaning, offspring from high-fat diet fed dams (HF) had impaired glucose tolerance as measured by OGTT compared to offspring from normal control diet fed dams (Control). The blood glucose levels were higher at 30 min (p < 0.0001) and the AUC was significantly larger (p < 0.0001) for offspring of HF group (Figures 2A,B). To determine whether maternal dietary genistein affected the glucose metabolism of the female offspring, we compared offspring from dams that were fed a high-fat diet with or without genistein. As shown in Figures 2A,B, female offspring of low-dose genistein fed dams (HF.LG) demonstrated a marked improvement in glucose tolerance. The blood glucose levels at 30 min (p < 0.0001) and AUC of these female offspring were significantly lower (p < 0.0001). Furthermore, to determine whether insulin sensitivity was influenced in female offspring, the level of fasting serum insulin was detected. High-fat fed dams resulted in a significantly higher insulin concentration (p < 0.001) and HOMA-IR index (p < 0.0001) in offspring at weaning. Low-dose genistein fed dams (HF.LG) led to improved insulin sensitivity in their offspring (Figures 2C,D). However, no significant difference was detected in regard to glucose tolerance and insulin sensitivity between female offspring of HF.HG group and HF group (Figures 2A–D). In addition, no significant differences were identified between offspring of HF.HG and HF.LG group dams in regard to blood glucose levels at different times during the glucose tolerance test, serum insulin levels and HOMA-IR, other than the significantly lower AUC in offspring of HF.LG group (p < 0.001).
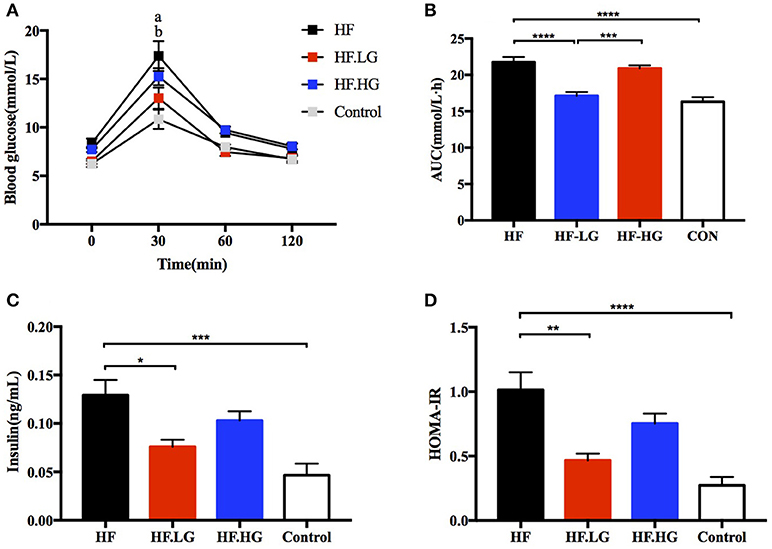
Figure 2. Glucose metabolism of the female offspring at weaning. (A) OGTT; (B) AUC; (C) Serum insulin levels; (D) HOMA-IR. HF, high-fat diet without genistein; HF. LG, high-fat diet with low-dose genistein; HF. HG, high-fat diet with high-dose genistein; Control, normal control diet; OGTT, oral glucose tolerance test; AUC, area under the curve; HOMA-IR, the homeostasis model assessment of insulin resistance. Data are expressed as means ± S.E.M. (n = 6-8/group). Mean values were significantly different between other group and the HF group: *p < 0.05, **p < 0.01, ***p < 0.001, ****p < 0.0001; Mean values were significantly different between Control group and the HF group: ‘a' p < 0.0001; Mean values were significantly different between HF.LG group and the HF group: ‘b' p < 0.001.
Maternal Dietary High-Dose Genistein Improves Lipid Metabolism in the Early Life of Offspring
In addition to glucose metabolism, we detected the levels of serum lipids to evaluate the differences between groups regarding lipid metabolism of female offspring at weaning. The levels of serum TG (p < 0.05, Figure 3A) and TC (p < 0.01, Figure 3B) in the offspring of high-fat fed dams (HF) were higher than those in the offspring of Control group dams. High-dose genistein feeding of dams (HF.HG) resulted in a significant improvement in the serum TG (p < 0.01, Figure 3A) and TC levels (p < 0.01, Figure 3B) in female offspring. In contrast to the changes in glucose tolerance and insulin sensitivity, there was no significant difference in the serum lipid levels between the offspring of HF.LG group and HF group.
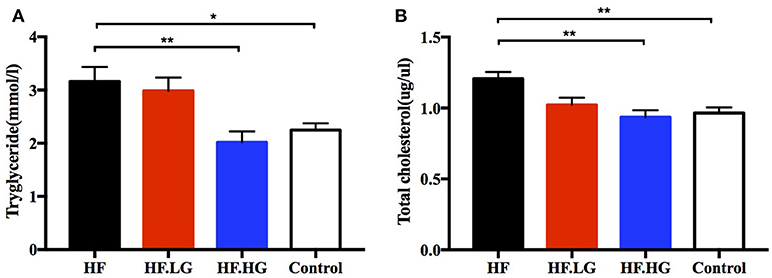
Figure 3. Lipid metabolism of the female offspring at weaning. (A) Serum triacyglycerol; and (B) serum total cholesterol. HF, high-fat diet without genistein; HF. LG, high-fat diet with low-dose genistein; HF. HG, high-fat diet with high-dose genistein; Control, normal control diet. Data are expressed as means ± S.E.M (n = 6-8/group). Mean values were significantly different between other group and the HF group: *p < 0.05, **p < 0.01.
Effects of Maternal Dietary Genistein on Gut Microbiota in Offspring
To explore the mechanisms of maternal dietary genistein improvement of glucose and lipid metabolism in offspring, we analyzed the gut microbiota changes in offspring using 16s rDNA gene sequences. The sequence data in this study has been submitted to the Sequence Read Archive (SRA) database (accession number SRP156380). A total of 1836395 high quality reads were obtained from 28 samples, with an average of 65586 sequences per sample. After clustering at the 97% similarity level, 653 operational taxonomic units (OTU) were identified among the four groups. Alpha diversity analysis showed that there was a parallel community richness (Chao 1) and diversity (Simpson and Shannon index) among groups (Table S2, Figure S1). To compare the overall structure of the gut microbial community, principal component analysis (PCA) was performed to determine differences among groups. As shown in Figure 4, the gut microbial communities were well separated in the HF group compared with both the Control group (p < 0.01) and HF.HG group (p < 0.05), with 55.76 and 22.51% variations explained by principal component (PC) 1 and PC2, respectively. The results were supported by ANOSIM and showed that there significant differences in the microbial structure were caused by the maternal diets during pre-pregnancy, pregnancy and lactation. This result was also verified by a heatmap according to the bacterial genus level among the four groups (Figure 5).
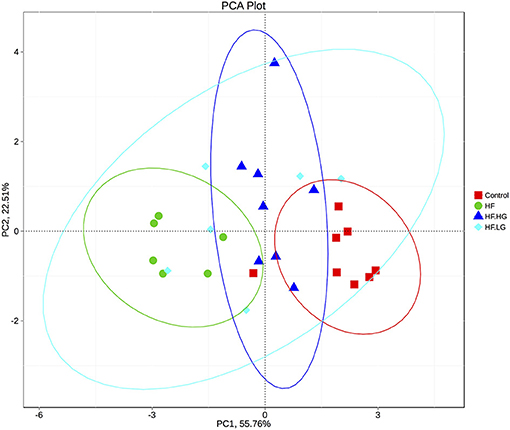
Figure 4. PCA plots of gut communities in the female offspring at weaning (n = 6–8/group). HF, high-fat diet without genistein; HF. LG, high-fat diet with low-dose genistein; HF. HG, high-fat diet with high-dose genistein; Control, normal control diet.
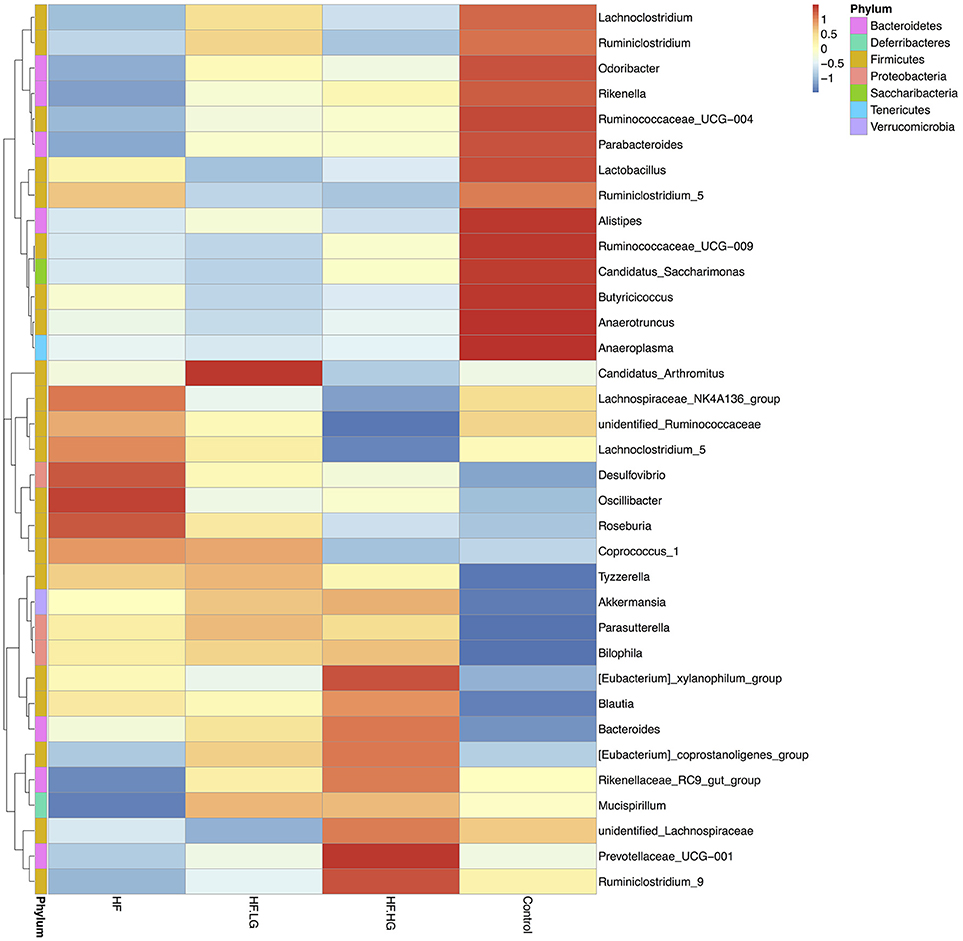
Figure 5. Heat map analyses of abundant genera in each group (n = 6–8/group). HF, high-fat diet without genistein; HF. LG, high-fat diet with low-dose genistein; HF. HG, high-fat diet with high-dose genistein; Control, normal control diet.
There were significant alterations in the composition of the intestinal microbial community among the four groups. MetaStat analysis showed that the phylum Proteobacteria, class Deltaproteobacteria, family Desulfovibrionaceae and genus Desulfovibrio were all significantly increased in the HF group compared with the Control group (q < 0.05) and were also relatively lower in the HF.LG group and HF.HG group compared to the HF group. However, there was a lower relative abundance of the family Porphyromonadaceae, genus Ruminoccaceae_UCG-004, genus [Eubacterium]_brachy_group, genus Rikenella and genus Rikenellaceae_RC9_gut_group in the HF group compared to the Control group (q < 0.05), all of which also tended to increase in female offspring at weaning after a maternal dietary genistein treatment in comparison with offspring from dams fed a high-fat diet without genistein. The genera Rikenella and Rikenellaceae_RC9_gut_group, both from the family Rikenellaceae, were significantly increased in the HF.HG group compared to the HF group and may play important roles in lipid metabolism (Figures 6A–I). Figure 7 lists significant microbiota changes from the phylum level to the species level, as performed by LEfSe. The phylum Firmucutes, class Clostridia, family Rikenellaceae, family Porphyromonadaceae, genus Alistipes and genus Anaerotruncus were significantly enriched in the control group. The phylum Proteobacteria, class Deltaproteobacteria, order Desulfovibrionales, family Desulfovibrionaceae and genus Desulfovibrio exhibited higher abundances in the HF group. Low-dose and high-dose genistein treatment both increased Bacteroides and the Akkermansia at the genus level (Figures 7A,B). By contrast, the species Bacteroides_acidifaciens was uniquely enriched in the HF.HG group (Figure 7B).
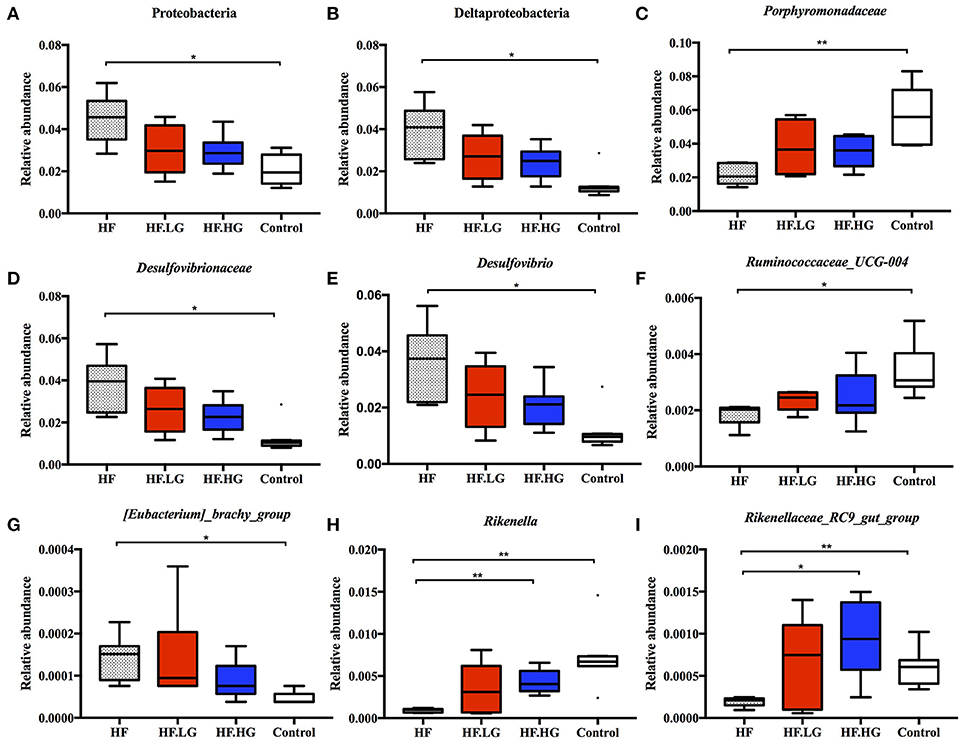
Figure 6. Relative abundance of bacterial taxa at different taxonomic levels in each group. (n = 6–8/group). (A) Proteobacteria; (B) Deltaproteobacteria; (C) Porphyromonadaceae; (D) Desulfovibrionaceae; (E) Desulfovibrio; (F) Ruminococcaceae_UCG-004; (G) [Eubacterium]_brachy_group; (H) Rikenella; and (I) Rikenellaceae_RC9_gut_group. HF, high-fat diet without genistein; HF. LG, high-fat diet with low-dose genistein; HF. HG, high-fat diet with high-dose genistein; Control, normal control diet. Data was analyzed by MetaStat. Mean values were significantly different between other group and the HF group: *q < 0.05, **q < 0.01.
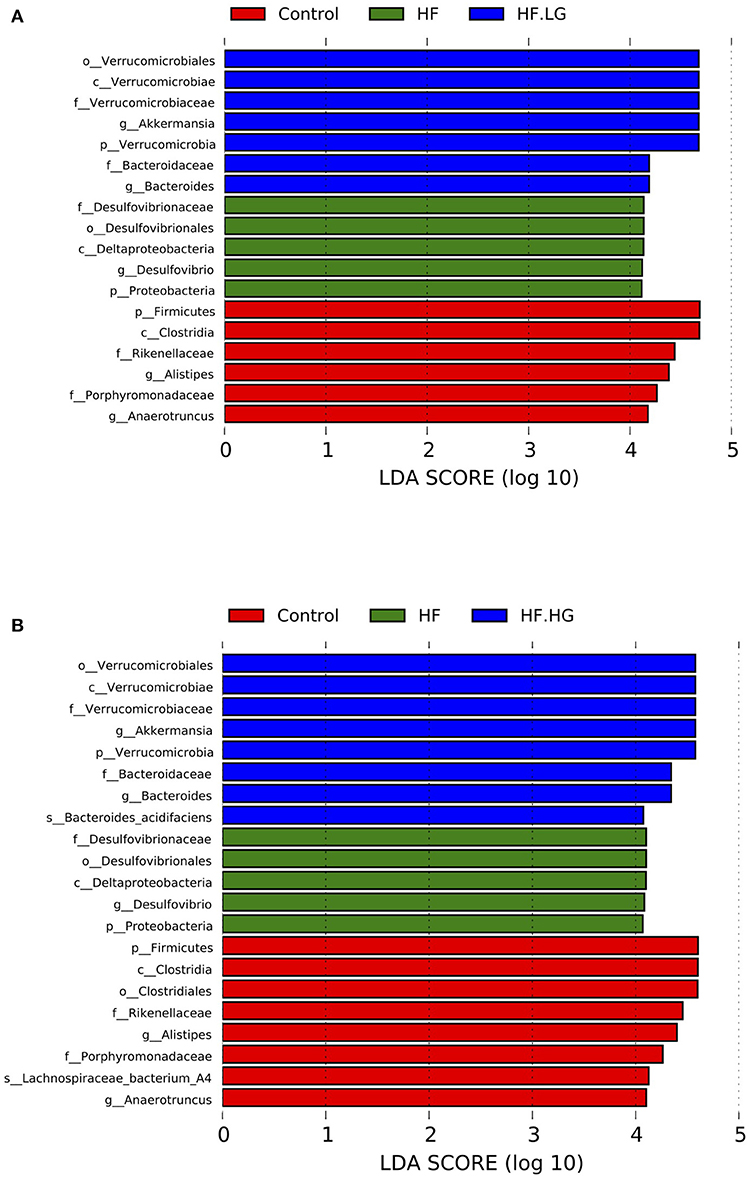
Figure 7. The LEfSe analysis of the different gut microbiota from the phylum level down to the species level (n = 6–8/group). (A) Differently enriched bacteria among the HF-HF.LG-Control group; and (B) Differently enriched bacteria among the HF-HF.HG-Control group. HF, high-fat diet without genistein; HF. LG, high-fat diet with low-dose genistein; HF. HG, high-fat diet with high-dose genistein; Control, normal control diet.
Correlation Analyses of the Gut Microbiota and Glucose and Lipid Metabolic Parameters
To assess associations between glucose and lipid metabolism and the gut microbiota in offspring, the AUC of the OGTT, insulin, HOMA-IR, TC, and TG levels were correlated with the intestinal bacterial relative abundance (Table 2). The AUC of the OGTT, fasting insulin levels, HOMA-IR and serum TC levels were positively correlated with the relative abundance of Proteobacteria at the phylum level and the class Deltaproteobacteria from the phylum Proteobacteria. However, the fasting serum insulin concentration and HOMA-IR were negatively correlated with the class Clostridia (phylum Firmicutes). At the family level, the AUC of the OGTT, insulin levels, HOMA-IR and serum TC levels were positively correlated with the abundance of Desulfovibrionaceae from the class Deltaproteobacteria. By contrast, the AUC of the OGTT, insulin levels, and HOMA-IR were all negatively correlated with the families Porphyromonadaceae and insulin levels, and HOMA-IR were both negatively correlated with Rikenellaceae, both of which belong to the phylum Bacteroidetes. At the genus level, the AUC of the OGTT, insulin and HOMA-IR were positively correlated with the genus Desulfovibrio from the phylum Firmicutes, but insulin and HOMA-IR were negatively correlated with the Alistipes and Rikenella from the phylum Bacteroidetes. In addition, HOMA-IR were also positively correlated with the species Bacteroides_acidifaciens. In regard to lipid metabolism, TC was positively correlated with the genus Desulfovibrio.
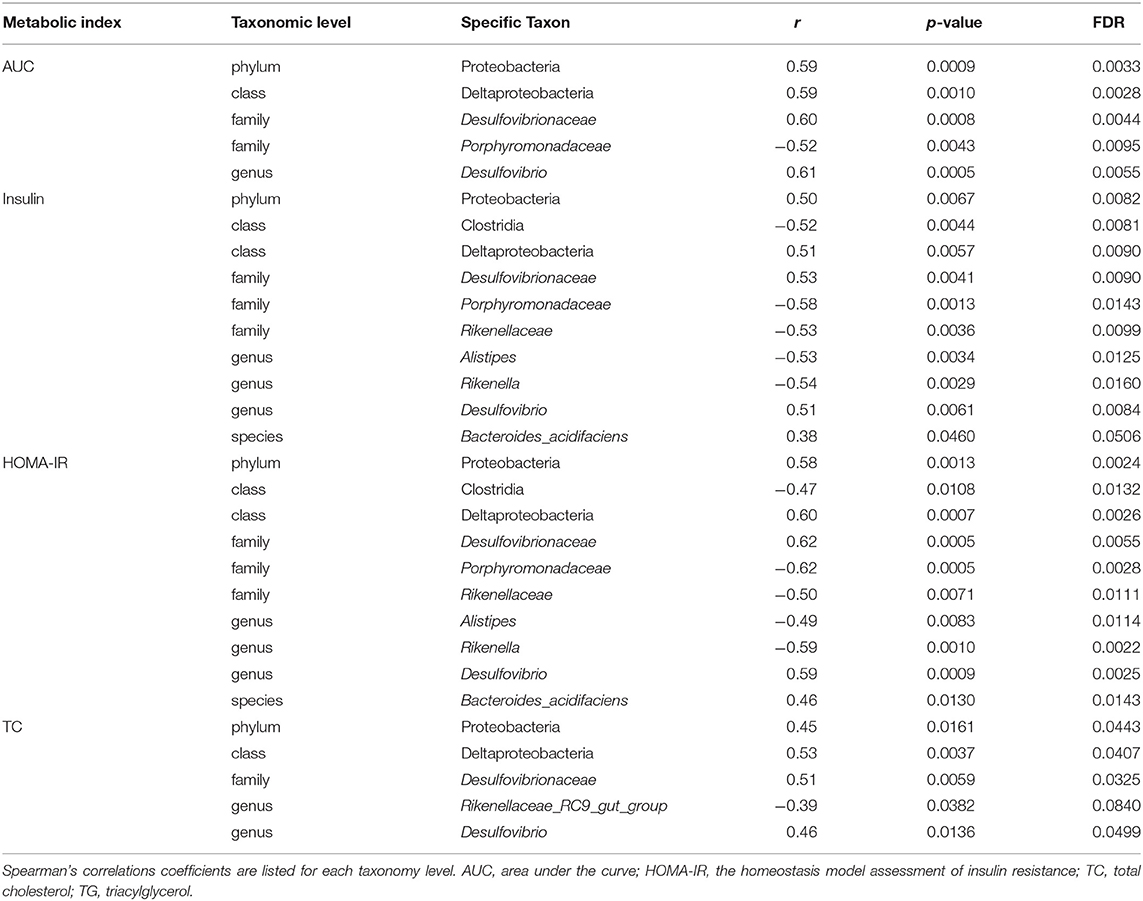
Table 2. Correlation analyses between relative abundance of bacterial taxa at different taxonomic levels and glucose and lipid metabolism parameters (n = 6–8/group).
Discussion
It is well established that a poor maternal diet is an important factor in the development of metabolic disorders in offspring (29–32), which may have contributed to the current rapid increase in the prevalence of obesity and diabetes. Similarly, the present study also demonstrated that a maternal high-fat diet before pregnancy and during pregnancy and lactation could result in glucose intolerance, insulin resistance and higher serum levels of TC and TG in the early life of female offspring. It has been reported that genistein has anti-diabetic (13) and lipid metabolism improvement (33) functions, but the effects of genistein intake during pregnancy and lactation on glucose and lipid metabolism in offspring are poorly understood. In the present study, we explored the effects of maternal dietary genistein on glucose and lipid metabolism in female offspring at weaning. We found that maternal dietary low-dose genistein (0.25 g/kg diet) fully counteracted the detrimental effects of the maternal high-fat diet on glucose tolerance, circulating insulin, HOMA-IR and birth weight in female offspring. Moreover, disorders of the serum lipid profiles in offspring due to a maternal high-fat diet were prevented if dams were fed high-dose genistein (0.6 g/kg diet). The uterus and ovary index showed that the genistein intervention had no adverse effects on dams. These data indicate that maternal dietary genistein is pivotal for improving metabolic health in the early life of female offspring in a dose-dependent manner.
There might be changes in many organizations of the offspring that play important roles in the beneficial effects of maternal dietary genistein and the deleterious effects of a maternal high-fat diet on metabolic disorders in offspring. Given the vital role that the intestinal microbial community play in metabolic health, we hypothesized that the intestinal microbiota of the offspring changed. Indeed, our results showed that maternal high-fat feeding leaded to significant alterations in the overall structure and composition of the intestinal microbial community and that maternal genistein intake reversed these detrimental effects. The present study showed that Proteobacteria, Deltaproteobacteria, Desulfovibrionales, Desulfovibrionaceae and Desulfovibrio significantly increased and were positively correlated with glucose and lipid metabolic parameters, whereas Porphyromonadaceae, Ruminoccaceae_UCG-004, [Eubacterium]_brachy_group, Rikenella and Rikenellaceae_RC9_gut_group significantly decreased and were negatively correlated with glucose and lipid metabolic parameters in female offspring from high-fat fed dams compared to those in female offspring of Control group dams. As previously shown, high-fat feeding resulted in significantly increased abundance of Proteobacteria and Desulfovibrionaceae. Tomas et al. (34) found that a high-fat diet altered the composition of the fecal and cecal microbial community even after 30 d of consumption and that the relative abundance of Proteobacteria significantly increased. In Proteobacteria, the main increase was in class Deltaproteobacteria (Desulfovibrionales order, Desulfovibrionaceae family). Meanwhile, the physical integrity of the epithelial barrier was disrupted and intestinal permeability was increased. Similarly, another previous study showed that the intestinal microbiota of wild-type mice switched to high-fat diets has changed a lot, including an increase in Proteobacteria. The main group of Proteobacteria increased in relative abundance, as did the class DeltaProteobacteria and genus Desulfovibrio (35). Several genera belonging to the Desulfovibrionaceae family are considered to be opportunistic pathogens and have been linked to some inflammatory diseases (36, 37). These genera produce endotoxins and have the capacity to reduce sulfate to H2S (38), thereby damaging the intestinal barrier (39). Maternal dietary genistein decreases the abundance of these bacteria and reverses their detrimental effects on metabolism. In addition, Li et al. (40) analyzed the effect of the antibiotic azithromycin on the gut microbiota and adipogenesis in mice and found that the abundance of Rikenella was significantly lower in the azithromycin group and was associated with a higher body weight and larger percentage of body fat. Another human study also indicated that the abundance of Rikenellaceae, along with other bacterial components, contributed to a lean body type (41). In the present study, maternal dietary high-dose genistein significantly increased the abundance of Rikenella and Rikenellaceae_ RC9_ gut_group and improved the levels of TG and TC in female offspring at weaning. Thus, the genus of Rikenella and Rikenellaceae_ RC9_ gut_group might play crucial roles in the improved lipid metabolism by high-dose genistien.
In the current study, at the genus level, maternal dietary genistein (including HF.LG and HF.HG) significantly enriched Bacteroides and Akkermansia. Several human studies have shown that the relative abundance of Bacteroides was decreased in type 2 diabetes patients in comparison to normal control subjects (42, 43). A fiber-rich macrobiotic Ma-Pi 2 diet increased the abundance of propionate producers (Bacteroides) (42). In addition, another obese mice study showed that resveratrol improved glucose tolerance while increasing the relative abundance of Bacteroides (44). Our study found that Bacteroides was enriched in female offspring from genistein fed dams and might play crucial roles in negating the deleterious effects of a poor maternal diet on the metabolism of offspring. The beneficial effects of maternal genistein intake on the metabolism of offspring were also associated with a significant enrichment in the relative abundance of Akkermansia, which could maintain the mucus layer thickness and reduce leakage of LPS and intestinal permeability (45).
To our knowledge, this is the first study that showed that the phytoestrogen genistein exerts a significant effect on the abundance of Akkermansia in the gut microbial commuity of an animal model of maternal high-fat diet-induced offspring metabolic disorders. Recently, it has been reported that administration of polyphenols was also associated with an increased abundance of Akkermansia in both human and animal studies (46, 47). Moreover, an increase in the gut proportion of this bacterium has also been associated with the beneficial effects of the anti-diabetic drug metformin and gastric bypass surgery on metabolism (48, 49). Although we have not directly determined the causality between the increased proportion of Akkermansia and the improvement of glucose and lipid metabolism in offspring from genistein intake high-fat fed dams, it has been reported that oral administration of Akkermansia reverses the metabolic abnormalities induced by a high-fat diet (45) and also mimics the antidiabetic effects of metformin in diabetic mice (48). More importantly, our results indicated that the increase in Akkermansia associated with genistein might be sufficient to improve metabolic disorders in offspring induced by a maternal high-fat diet without significant alterations in the proportions of Firmicutes and Bacteroidetes.
In addition, LEfSe analysis showed that the species Bacteroides_acidifaciens was uniquely enriched in the HF.HG group. Renouf et al. (50) first identified fecal microbes that were responsible for the degradation of isoflavone using molecular genetic techniques and demonstrated that Bacteroides_acidifaciens increased the disappearance of isoflavone genistein in human fecal incubating under anaerobic and nutrient-rich conditions, which indicated that Bacteroides_acidifaciens played a role in the metabolism of genistein in the intestine. Thus, the enrichment of Bacteroides_acidifaciens in the HF.HG group increased the degradation of isoflavones genistein. Correlation analysis showed that the relative abundance of Bacteroides_acidifaciens was positively related with HOMA-IR, which clarified the differences of the effects of maternal low-dose genistein and high-dose genistein on the glucose metabolism and insulin sensitivity in the offspring.
In summary, maternal dietary genistein provided before and during pregnancy and lactation significantly improved the metabolism of female offspring in early life and compensated for the detrimental effects of a maternal high-fat diet. The improvement in glucose and lipid metabolism is associated with the alterations in the gut microbiota of offspring. This is the first study to report the role that the gut microbiota plays in the effects of maternal dietary genistein on glucose and lipid metabolism in female offspring. However, our study only analyzed the relationship between gut microbiota and metabolism in offspring and the causality is still needed to be further explored. Furthermore, only female offspring was studied in this study. The effects of maternal dietary genistein on metabolic health of male offspring is worth to be studied in the future. Our results suggest that the provision of maternal dietary genistein before pregnancy and during pregnancy and lactation may be an important tool for combating obesity and diabetes in offspring.
Data Availability
The datasets supporting the conclusions of this manuscript are available from the corresponding author on reasonable request.
Author Contributions
XX and JZ designed the experiments. LZ, XW, MD, RL, and XZ performed the experiments. LZ analyzed the data and wrote the original draft. XX, JZ, QZ, ML, and MY reviewed the manuscript. All of the authors had final approval of the submitted version.
Funding
This work was supported by the grants from National Key R&D Program of China (2017YFC1309603), National Key Research and Development Program of China (2016YFA0101002), National Natural Science Foundation of China (Nos. 81170736, 81570715, 81870579, and 81870545), Medical Epigenetics Research Center, Chinese Academy of Medical Sciences (2017PT31036). The funders had no role in the design, analysis or writing of this article.
Conflict of Interest Statement
The authors declare that the research was conducted in the absence of any commercial or financial relationships that could be construed as a potential conflict of interest.
Acknowledgments
We are very grateful to Beijing Compass Biotechnology Company for their technical support with 16s rRNA sequences.
Supplementary Material
The Supplementary Material for this article can be found online at: https://www.frontiersin.org/articles/10.3389/fendo.2018.00516/full#supplementary-material
References
1. Galley JD, Bailey M, Kamp Dush C, Schoppe-Sullivan S, Christian LM. Maternal obesity is associated with alterations in the gut microbiome in toddlers. PLoS ONE (2014) 9:e113026. doi: 10.1371/journal.pone.0113026
2. Jornayvaz FR, Vollenweider P, Bochud M, Mooser V, Waeber G, Marques-Vidal P. Low birth weight leads to obesity, diabetes and increased leptin levels in adults: the CoLaus study. Cardiovasc Diabetol.(2016) 15:73. doi: 10.1186/s12933-016-0389-2
3. Eriksson JG, Sandboge S, Salonen MK, Kajantie E, Osmond C. Long-term consequences of maternal overweight in pregnancy on offspring later health: findings from the Helsinki Birth Cohort Study. Ann Med. (2014) 46:434–8. doi: 10.3109/07853890.2014.919728
4. Xiao X, Zhang ZX, Cohen HJ, Wang H, Li W, Wang T, et al. Evidence of a relationship between infant birth weight and later diabetes and impaired glucose regulation in a Chinese population. Diabetes Care. (2008) 31:483–7. doi: 10.2337/dc07-1130
5. Ohta T, Toriniwa Y, Ryumon N, Inaba N, Hirao T, Yamanaka S, et al. Maternal high-fat diet promotes onset of diabetes in rat offspring. Anim Sci J. (2017) 88:149–55. doi: 10.1111/asj.12606
6. Seet EL, Yee JK, Jellyman JK, Han G, Ross MG, Desai M. Maternal high-fat-diet programs rat offspring liver fatty acid metabolism. Lipids (2015) 50:565–73. doi: 10.1007/s11745-015-4018-8
7. Segovia SA, Vickers MH, Harrison CJ, Patel R, Gray C, Reynolds CM. Maternal high-fat and high-salt diets have differential programming effects on metabolism in adult male rat offspring. Front Nutr. (2018) 5:1. doi: 10.3389/fnut.2018.00001
8. Keleher MR, Zaidi R, Shah S, Oakley ME, Pavlatos C, El Idrissi S, et al. Maternal high-fat diet associated with altered gene expression, DNA methylation, and obesity risk in mouse offspring. PLoS ONE (2018) 13:e0192606. doi: 10.1371/journal.pone.0192606
9. Zheng J, Xiao X, Zhang Q, Yu M, Xu J, Wang Z. Maternal high-fat diet modulates hepatic glucose lipid homeostasis and gene expression in the PPAR pathway in the early life of offspring. Int J Mol. Sci. (2014) 15:14967–83. doi: 10.3390/ijms150914967
10. Nanri A, Mizoue T, Takahashi Y, Kirii K, Inoue M, Noda M, et al. Soy product and isoflavone intakes are associated with a lower risk of type 2 diabetes in overweight Japanese women. J Nutr. (2010) 140:580–6. doi: 10.3945/jn.109.116020
11. Ding M, Pan A, Manson JE, Willett WC, Malik V, Rosner B, et al. Consumption of soy foods and isoflavones and risk of type 2 diabetes: a pooled analysis of three US cohorts. Eur J Clin Nutr. (2016) 70:1381–7. doi: 10.1038/ejcn.2016.117
12. Odegaard AO, Koh WP, Butler LM, Duval S, Gross MD, Yu MC, et al. Dietary patterns and incident type 2 diabetes in chinese men and women: the singapore chinese health study. Diabetes Care (2011) 34:880–5. doi: 10.2337/dc10-2350
13. Gilbert ER, Liu D. Anti-diabetic functions of soy isoflavone genistein: mechanisms underlying its effects on pancreatic beta-cell function. Food Funct. (2013) 4:200–12. doi: 10.1039/c2fo30199g
14. Squadrito F, Marini H, Bitto A, Altavilla D, Polito F, Adamo EB, et al. Genistein in the metabolic syndrome: results of a randomized clinical trial. J Clin Endocrinol Metabol. (2013) 98:3366–74. doi: 10.1210/jc.2013-1180
15. Guo TL, Germolec DR, Zheng JF, Kooistra L, Auttachoat W, Smith MJ, et al. Genistein protects female nonobese diabetic mice from developing type 1 diabetes when fed a soy- and alfalfa-free diet. Toxicol Pathol. (2015) 43:435–48. doi: 10.1177/0192623314526318
16. Simperova A, Al-Nakkash L, Faust JJ, Sweazea KL. Genistein supplementation prevents weight gain but promotes oxidative stress and inflammation in the vasculature of female obese ob/ob mice. Nutr Res. (2016) 36:789–97. doi: 10.1016/j.nutres.2016.03.011
17. Pascale A, Marchesi N, Marelli C, Coppola A, Luzi L, Govoni S, et al. Microbiota and metabolic diseases. Endocrine (2018) 61:357–71. doi: 10.1007/s12020-018-1605-5
18. Lippert K, Kedenko L, Antonielli L, Kedenko I, Gemeier C, Leitner M, et al. Gut microbiota dysbiosis associated with glucose metabolism disorders and the metabolic syndrome in older adults. Benef Microbes (2017) 8:545–556. doi: 10.3920/bm2016.0184
19. Shang Y, Khafipour E, Derakhshani H, Sarna LK, Woo CW, Siow YL, et al. Short term high fat diet induces obesity-enhancing changes in mouse gut microbiota that are partially reversed by cessation of the high fat diet. Lipids (2017) 52:499–511. doi: 10.1007/s11745-017-4253-2
20. Tun HM, Bridgman SL, Chari R, Field CJ, Guttman DS, Becker AB, et al. Roles of birth mode and infant gut microbiota in intergenerational transmission of overweight and obesity from mother to offspring. JAMA Pediatr. (2018) 172:368–77. doi: 10.1001/jamapediatrics.2017.5535
21. Mann PE, Huynh K, Widmer G. Maternal high fat diet and its consequence on the gut microbiome: a rat model. Gut Microbes (2018) 9:143–54. doi: 10.1080/19490976.2017.1395122
22. Chu DM, Meyer KM, Prince AL, Aagaard KM. Impact of maternal nutrition in pregnancy and lactation on offspring gut microbial composition and function. Gut Microbes (2016) 7:459–70. doi: 10.1080/19490976.2016.1241357
23. Huang G, Xu J, Lefever DE, Glenn TC, Nagy T, Guo TL. Genistein prevention of hyperglycemia and improvement of glucose tolerance in adult non-obese diabetic mice are associated with alterations of gut microbiome and immune homeostasis. Toxicol Appl Pharmacol. (2017) 332:138–48. doi: 10.1016/j.taap.2017.04.009
24. Zhang Q, Xiao X, Li M, Yu M, Ping F, Zheng J, et al. Vildagliptin increases butyrate-producing bacteria in the gut of diabetic rats. PLoS ONE (2017) 12:e0184735. doi: 10.1371/journal.pone.0184735
25. Edgar RC. UPARSE: highly accurate OTU sequences from microbial amplicon reads. Nat Methods (2013) 10:996–8. doi: 10.1038/nmeth.2604
26. Caporaso JG, Kuczynski J, Stombaugh J, Bittinger K, Bushman FD, Costello EK, et al. QIIME allows analysis of high-throughput community sequencing data. Nat Methods (2010) 7:335–6. doi: 10.1038/nmeth.f.303
27. DeSantis TZ, Hugenholtz P, Larsen N, Rojas M, Brodie EL, Keller K, et al. Greengenes, a chimera-checked 16S rRNA gene database and workbench compatible with ARB. Appl Environ Microbiol. (2006) 72:5069–72. doi: 10.1128/aem.03006-05
28. Wang Q, Garrity GM, Tiedje JM, Cole JR. Naive Bayesian classifier for rapid assignment of rRNA sequences into the new bacterial taxonomy. Appl Environ Microbiol. (2007) 73:5261–7. doi: 10.1128/aem.00062-07
29. Watkins AJ, Lucas ES, Wilkins A, Cagampang FR, Fleming TP. Maternal periconceptional and gestational low protein diet affects mouse offspring growth, cardiovascular and adipose phenotype at 1 year of age. PLoS ONE (2011) 6:e28745. doi: 10.1371/journal.pone.0028745
30. Shapiro AL, Kaar JL, Crume TL, Starling AP, Siega-Riz AM, Ringham BM, et al. Maternal diet quality in pregnancy and neonatal adiposity: the Healthy Start Study. Int J Obes (Lond) (2016) 40:1056–62. doi: 10.1038/ijo.2016.79
31. Hoffman ML, Peck KN, Wegrzyn JL, Reed SA, Zinn SA, Govoni KE. Poor maternal nutrition during gestation alters the expression of genes involved in muscle development and metabolism in lambs. J Anim Sci. (2016) 94:3093–9. doi: 10.2527/jas.2016-0570
32. Stanford KI, Takahashi H, So K, Alves-Wagner AB, Prince NB, Lehnig AC, et al. Maternal exercise improves glucose tolerance in female offspring. Diabetes (2017) 66:2124–36. doi: 10.2337/db17-0098
33. Tang C, Zhang K, Zhao Q, Zhang J. Effects of dietary genistein on plasma and liver lipids, hepatic gene expression, and plasma metabolic profiles of hamsters with diet-induced hyperlipidemia. J Agric Food Chem. (2015) 63:7929–36. doi: 10.1021/acs.jafc.5b01590
34. Tomas J, Mulet C, Saffarian A, Cavin JB, Ducroc R, Regnault B, et al. High-fat diet modifies the PPAR-gamma pathway leading to disruption of microbial and physiological ecosystem in murine small intestine. Proc Natl Acad Sci USA. (2016) 113:E5934-43. doi: 10.1073/pnas.1612559113
35. Hildebrandt MA, Hoffmann C, Sherrill-Mix SA, Keilbaugh SA, Hamady M, Chen YY, et al. High-fat diet determines the composition of the murine gut microbiome independently of obesity. Gastroenterology (2009) 137:1716–24.e1-2. doi: 10.1053/j.gastro.2009.08.042
36. Loubinoux J, Mory F, Pereira IA, Le Faou AE. Bacteremia caused by a strain of Desulfovibrio related to the provisionally named Desulfovibrio fairfieldensis. J Clin Microbiol. (2000) 38:931–4.
37. Weglarz L, Dzierzewicz Z, Skop B, Orchel A, Parfiniewicz B, Wisniowska B, et al. Desulfovibrio desulfuricans lipopolysaccharides induce endothelial cell IL-6 and IL-8 secretion and E-selectin and VCAM-1 expression. Cell Mol Biol Lett. (2003) 8:991–1003.
38. Wagner M, Roger AJ, Flax JL, Brusseau GA, Stahl DA. Phylogeny of dissimilatory sulfite reductases supports an early origin of sulfate respiration. J Bacteriol. (1998) 180:2975–82.
39. Jakobsson HE, Rodriguez-Pineiro AM, Schutte A, Ermund A, Boysen P, Bemark M, et al. The composition of the gut microbiota shapes the colon mucus barrier. EMBO Rep. (2015) 16:164–77. doi: 10.15252/embr.201439263
40. Li R, Wang H, Shi Q, Wang N, Zhang Z, Xiong C, et al. Effects of oral florfenicol and azithromycin on gut microbiota and adipogenesis in mice. PLoS ONE (2017) 12:e0181690. doi: 10.1371/journal.pone.0181690
41. Oki K, Toyama M, Banno T, Chonan O, Benno Y, Watanabe K. Comprehensive analysis of the fecal microbiota of healthy Japanese adults reveals a new bacterial lineage associated with a phenotype characterized by a high frequency of bowel movements and a lean body type. BMC Microbiol. (2016) 16:284. doi: 10.1186/s12866-016-0898-x
42. Candela M, Biagi E, Soverini M, Consolandi C, Quercia S, Severgnini M, et al. Modulation of gut microbiota dysbioses in type 2 diabetic patients by macrobiotic Ma-Pi 2 diet. Br J Nutr. (2016) 116:80–93. doi: 10.1017/s0007114516001045
43. Zhang X, Shen D, Fang Z, Jie Z, Qiu X, Zhang C, et al. Human gut microbiota changes reveal the progression of glucose intolerance. PLoS ONE (2013) 8:e71108. doi: 10.1371/journal.pone.0071108
44. Sung MM, Kim TT, Denou E, Soltys CM, Hamza SM, Byrne NJ, et al. Improved glucose homeostasis in obese mice treated with resveratrol is associated with alterations in the gut microbiome. Diabetes (2017) 66:418–25. doi: 10.2337/db16-0680
45. Everard A, Belzer C, Geurts L, Ouwerkerk JP, Druart C, Bindels LB, et al. Cross-talk between Akkermansia muciniphila and intestinal epithelium controls diet-induced obesity. Proc Natl Acad Sci USA. (2013) 110:9066–71. doi: 10.1073/pnas.1219451110
46. Anhe FF, Roy D, Pilon G, Dudonne S, Matamoros S, Varin TV, et al. A polyphenol-rich cranberry extract protects from diet-induced obesity, insulin resistance and intestinal inflammation in association with increased Akkermansia spp. population in the gut microbiota of mice. Gut (2015) 64:872–83. doi: 10.1136/gutjnl-2014-307142
47. Kemperman RA, Gross G, Mondot S, Possemiers S, Marzorati M, Van de Wiele T, et al. Impact of polyphenols from black tea and red wine/grape juice on a gut model microbiome. Food Res Int. (2013) 53:659–69. doi: 10.1016/j.foodres.2013.01.034
48. Shin NR, Lee JC, Lee HY, Kim MS, Whon TW, Lee MS, et al. An increase in the Akkermansia spp. population induced by metformin treatment improves glucose homeostasis in diet-induced obese mice. Gut (2014) 63:727–35. doi: 10.1136/gutjnl-2012-303839
49. Liou AP, Paziuk M, Luevano JM Jr, Machineni S, Turnbaugh PJ, Kaplan LM. Conserved shifts in the gut microbiota due to gastric bypass reduce host weight and adiposity. Sci Transl Med. (2013) 5:178ra41. doi: 10.1126/scitranslmed.3005687
Keywords: dietary genistein, glucose and lipid metabolism, gut microbiota, maternal high-fat diet, female offspring
Citation: Zhou L, Xiao X, Zhang Q, Zheng J, Li M, Yu M, Wang X, Deng M, Zhai X and Li R (2018) Improved Glucose and Lipid Metabolism in the Early Life of Female Offspring by Maternal Dietary Genistein Is Associated With Alterations in the Gut Microbiota. Front. Endocrinol. 9:516. doi: 10.3389/fendo.2018.00516
Received: 21 June 2018; Accepted: 17 August 2018;
Published: 04 September 2018.
Edited by:
Wei Bao, University of Iowa, United StatesReviewed by:
Richard R. Rodrigues, Oregon State University, United StatesVenu Lagishetty, University of California, Los Angeles, United States
Copyright © 2018 Zhou, Xiao, Zhang, Zheng, Li, Yu, Wang, Deng, Zhai and Li. This is an open-access article distributed under the terms of the Creative Commons Attribution License (CC BY). The use, distribution or reproduction in other forums is permitted, provided the original author(s) and the copyright owner(s) are credited and that the original publication in this journal is cited, in accordance with accepted academic practice. No use, distribution or reproduction is permitted which does not comply with these terms.
*Correspondence: Xinhua Xiao, xiaoxh2014@vip.163.com