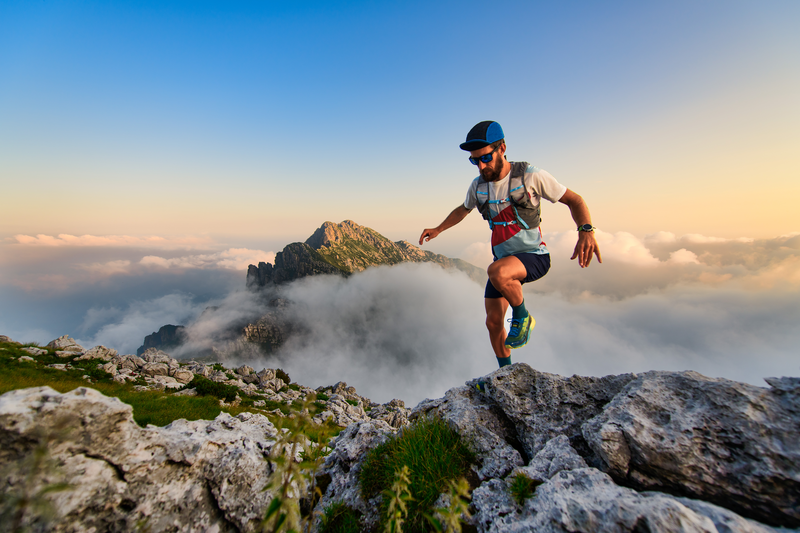
95% of researchers rate our articles as excellent or good
Learn more about the work of our research integrity team to safeguard the quality of each article we publish.
Find out more
REVIEW article
Front. Endocrinol. , 15 June 2018
Sec. Pituitary Endocrinology
Volume 9 - 2018 | https://doi.org/10.3389/fendo.2018.00318
This article is part of the Research Topic Targeted Therapy for Pituitary Adenomas View all 8 articles
Temozolomide, an alkylating agent, initially used in the treatment of gliomas was expanded to include pituitary tumors in 2006. After 12 years of use, temozolomide has shown a notable advancement in pituitary tumor treatment with a remarkable improvement rate in the 5-year overall survival and 5-year progression-free survival in both aggressive pituitary adenomas and pituitary carcinomas. In this paper, we review the mechanism of action of temozolomide as alkylating agent, its interaction with deoxyribonucleic acid repair systems, therapeutic effects in pituitary tumors, unresolved issues, and future directions relating to new possibilities of targeted therapy.
Since its development, temozolomide (TMZ), a monofunctional alkylating agent, has shown remarkable efficacy in the treatment of a variety of solid tumors and it has become an essential component of adjuvant therapy for the most frequent adult brain tumor type, glioblastoma multiforme (GBM) (1, 2). Its unique chemical structure and pharmacokinetic properties confer distinctive advantages over other alkylating agents. In 2006, TMZ began to be used for the treatment of aggressive pituitary adenomas and pituitary carcinomas (3–5). In this paper, we review the mechanism of action of TMZ, the deoxyribonucleic acid (DNA) repair systems triggered after its administration, the current understanding of TMZ activity, and the unresolved issues of its clinical use in pituitary tumors.
During World War I, alkylating agents were used as chemical weapons. Sulfur mustard, commonly known as mustard gas, when released into the air was a threat to skin and mucous membranes. Its vesicant effect caused large blisters on exposed skin, eye irritation, upper and lower airway inflammatory reactions, bone marrow aplasia, and pancytopenia (6). Even the slightly less toxic nitrogen mustards also exhibited cytotoxic effects causing bone marrow suppression after exposure and subsequent absorption into the body. In the 1940s, given their ability to produce significant tumor regression, alkylating agents became the earliest types of drugs used to treat cancer. The first clinical use as a chemotherapeutic agent was performed in 1942 but it was not reported for several years (7).
Alkylating agents are ubiquitous, highly reactive compounds that transfer alkyl radicals into a broad range of biologically active nucleophilic molecules (8). They are electrophilic compounds (behave as electron acceptors) that react with nucleophilic moieties (behave as electron donors) of DNA or proteins. This results in the covalent transfer of an alkyl group (from the alkylating agent) to the DNA or the protein (9). The alkyl substituents are acyclic, saturated, hydrocarbon chains that have lost one hydrogen. The main cellular target of alkylation is DNA, and cytotoxicity is due to the alkylation of its bases which impairs its function as a template during replication and transcription (9). Alkylating agents are a diverse group of chemical substances. Due to their unique properties, they can be genotoxic, but may be used in the treatment of tumors as well (8).
Alkylating agents can be mono- or bifunctional. Monofunctional agents have a single reactive group that interacts with a single center in DNA, whereas bifunctional agents have two groups which can react with two different sites in DNA (10, 11). Consequently, one monofunctional alkylating molecule produces a covalent adduct on one base only but with bifunctional alkylating agents, alkylation can occur on two bases within the same DNA strand (intrastrand crosslinking), or from opposite strands (interstrand crosslinking) (10, 12, 13).
Virtually all heteroatoms (non-carbon or hydrogen atoms) in DNA can be alkylated and, depending on the nucleophile and the alkylating agent, there are different sites of alkylation in double-stranded DNA (9, 12, 14–16). Base alkylation occurs mainly on position N7 and O6 in guanine (N7-MeG and O6-MeG), N1 and N3 in adenine (N1-MeA and N3-MeA), and N3 in cytosine (N3-MeC) (9) (Figure 1A). Additional alkylation sites do arise but are less frequent. Every site of alkylation has different consequences in chemical stability, mutagenesis, and cytotoxicity (8, 9). DNA adducts O6-MeG, N1 in guanine (N1-MeG), N7-MeG and O4 in thymine (O4-MeT) are both stable and mutagenic, whereas alkylation on other sites (i.e., at certain endocyclic nitrogen) yield chemically unstable adducts, making the purine/pyrimidine base-rings in the DNA susceptible to hydrolytic attack and base-ring opening (9, 15) (Figure 1B). Because alkylating agents produce more than one type of adduct, the exact consequence of base alkylation on the function of the cell is difficult to evaluate (9, 15). From the various types of DNA adducts produced, O6-MeG, which is the least frequent (5–10%), is the most cytotoxic lesion (17, 18).
Figure 1. (A) Sites of alkylation on the various bases in the DNA. Temozolomide produces alkylation on position N7 and O6 in guanine and N3 in adenine (blue arrows). Other alkylating agents act on different sites of alkylation (small gray arrows). (B) Chemical stability, mutagenesis, cytotoxicity, and repair mechanisms in different sites of alkylation. Based on Ref. (8, 9, 16, 19). Abbreviations: BER, base excision repair; DR, direct repair; MMR, mismatch repair; NER, nucleotide excision repair; DNA, deoxyribonucleic acid.
Alkylating agents were the first chemotherapeutic anti-cancer agents developed and are the largest group of drugs among cytotoxic chemotherapeutics (10). They consist of three different groups: classical, non-classical, and alkylating-like agents. Many alkylating agents are categorized as “classical” alkylating agents. They include true alkyl groups. Alkylating-like agents are platinum-based analogs and do not have an alkyl group. They can also interfere with DNA repair and can permanently damage it; hence, they are referred to as “alkylating-like.” The third group is known as “non-classical.” To date, there is no consensus on which agents belong to this category. Most classical alkylating agents and alkylating-like agents cause cytotoxicity by inducing DNA crosslinking while non-classical alkylating agents such as dacarbazine, procarbazine, and TMZ, induce O6-MeG, N7-MeG, N3-MeA, without triggering DNA crosslinking because they are monofunctional (10).
Temozolomide is a monofunctional alkylating agent belonging to the triazene group of non-classical alkylating agents, characterized by the presence of three adjacent nitrogen atoms (20) (Figure 2). It is related to a series of imidazotetrazines developed by Stevens and his associates in Birmingham, United Kingdom (21). Temozolomide is a lipophilic, low molecular weight (194 Da) prodrug with no pharmacological activity until it is hydrolyzed. It is orally administered, stable at acidic stomach pH levels, and reactive at pH levels above 7.0 (22). Its activation starts with the hydrolytic cleavage of the tetrazinone ring (Figure 2, green circle). The effect of water at the C4 position of TMZ opens the ring (Figure 2, orange arrow), releases carbon dioxide, and produces 5-(3-monomethyl-1-triazeno) imidazole-4-carboxamide (MTIC) which is an unstable and short-lived active compound. It undergoes further cleavage, to generate 5-aminoimidazole-4-carboxamide (AIC), and the highly reactive methyl diazonium methylating species (2, 23) (Figure 2). This cation methylates DNA producing the adducts N7-MeG (60–80%), N3-MeA (10–20%), and O6-MeG (5–10%). During the next cycle of DNA replication, the sequence of mismatch repair events results in cell cycle arrest which leads to cell death, as will be discussed below. Temozolomide activity increases as its accumulation in tumors rises. It has been shown that brain tumors have a more alkaline pH than healthy tissues, which may favor the activation of TMZ (19, 24). Hence, the pH levels in the microenvironment may be an essential factor modulating its cytotoxic effects and resistance.
Figure 2. Schematic of mode of action of temozolomide (TMZ). The activation of TMZ in physiologic pH levels within the blood stream into its reactive state that is responsible for methylating DNA. Based on Ref. (8, 19, 23). Abbreviations: MTIC, 5-(3-monomethyl-1-triazeno) imidazole-4-carboxamide; AIC, 5-aminoimidazole-4-carboxamide; MGMT, O6-methylguanine-DNA methyltransferase.
Deoxyribonucleic acid can be damaged by external agents or by unwanted products arising from the cell chemistry (14, 15, 25–29). Internal chemical events that threaten DNA stability are depurination, deamination, reactive oxygen species (ROS), and non-enzymatic methylation. In addition, DNA replication has a spontaneous and inevitable error rate that may persist despite proofreading/DNA editing mechanisms (30). External agents that likely cause DNA damage are ionizing and ultraviolet radiation, environmental chemicals, and chemotherapeutic agents such as TMZ.
During DNA replication, various types of damage can cause the replication fork to stall. These events may be potentially lethal to the cell, but complex and specialized multi-protein mechanisms continuously monitor the DNA to detect and repair any damage. If the cell is not able to restore DNA integrity, it may sustain cytotoxic or mutagenic perturbations. Cytotoxicity usually arises when progression through the cell cycle is delayed at repair checkpoints; if the damage inflicted to the genome overwhelms the repair capacity of the cell, apoptosis is triggered. By contrast, mutagenesis can occur if the cell sustains minor damages in the DNA yet it survives, and after cell division, these unrepaired damages may cause mispairing of bases that result in base-pair substitution which become a consolidated mutational change only after successive DNA replication cycles (8).
There are several mechanisms to correct or prevent alkylation-induced DNA damage. One of them occurs during DNA replication, and relies on the catalytic feature of the DNA polymerases delta and epsilon (Pol δ, Pol ε), which have a 3′ → 5′ exonuclease activity (known as proofreading) (30, 31). When DNA has an alkylated base, a mispairing is generated; subsequently, during DNA synthesis the incorrect base pair is recognized, the direction of DNA polymerase is reversed by one base pair, the mismatched base is excised, and the correct one is inserted. Then, DNA replication can continue forward. Proofreading is only one of several systems to prevent mutations or to repair damaged DNA (15, 25–28, 32, 33).
Direct repair (DR) of DNA can be achieved by removing only the abnormal alkyl group, without removing the base or nucleotide. This typically occurs in the G1 phase of the cell cycle. The cell uses this mechanism in the repair of O6-MeG by O6-methylguanine-DNA methyltransferase (MGMT), which removes the methyl group leaving the base intact (9, 15, 32) (Figure 2).
Another group of mechanisms involves excision repair, where a single base, nucleotide, or a segment of the damaged DNA strand is excised, and the gap is filled by a combination of DNA polymerase and ligase. Three well-known excision repair systems used to detect and correct cellular DNA damage include: base excision repair (BER), nucleotide excision repair (NER), and mismatch repair (MMR).
Base excision repair includes DNA glycosylases recognition of small distortions in DNA caused by damage to a single base or uracil misincorporation (19). Lesion-specific glycosylases recognize the damaged base, and the N-glycosidic bond is hydrolytically cleaved. This generates an internal abasic site with a remaining external phosphodiester backbone which is then cut by a specific endonuclease (AP-endonuclease). The gap is finally repaired by polymerase beta and ligase-III. Nucleotide excision repair involves the initial enzymatic recognition of local distortions of the DNA helix caused by mismatched bases or bulky adducts, followed by the removal of a short single-stranded DNA segment containing the lesion. Afterward, DNA polymerase fills the gap by synthesizing the short complementary sequence, completing the repair (9). Mismatch repair is a complex system for recognizing and repairing erroneous insertion, deletion, and misincorporation of bases. It involves removal of tens or hundreds of bases at both sides of the damaged base, or of the bases that do not form Watson–Crick base pairing. It is a highly conserved repair pathway in both eukaryotes and prokaryotes (34). Hence, human MMR proteins are homologs to the E. coli MMR proteins: MutL homologs 1 and 3 (MLH1 and MLH3), MutS homologs 2, 3, and 6 (MSH2, MSH3, and MSH6), and postmeiotic segregation increased proteins (PMS1 and PMS2). Therefore, the MMR is strand-specific (35). Loss of MMR function results in Lynch Syndrome (OMIM #120435) caused by heterozygous mutations in MMR genes. Although the DR mechanism is mainly involved during the repair of TMZ induced damages, all systems mentioned above do participate.
Direct repair of the effects of TMZ is carried out by MGMT, a small enzymatic protein (22 kDa) that removes the methyl group from the O6-MeG adduct (19). It is a stable protein with a half-life of more than 24 h. The O6-methyl group is transferred from the guanine to an internal cysteine residue (Cys 145) on the MGMT, in a one-step reaction without relying on cofactors or enzymes (36) (Figure 2). This process removes methyl/alkyl molecules at a 1:1 ratio and restores guanine to its normal structure, thereby eliminating any further DNA strand breaks. In this manner, MGMT acts as an acceptor molecule by removing (sequestering/accepting) the methyl group from the O6-MeG. It then becomes inactivated and degraded through ubiquitination (19). For this reason, it has been called a suicide enzyme (19, 37). Although MGMT protects healthy cells from alkylating carcinogens, it also protects tumoral cells from the same kind of chemical genotoxicity. The non-discriminating effect of MGMT on both healthy and tumoral cells is of concern since it can counteract the effects of TMZ treatment. In some tumors, low-level expression of MGMT may result from epigenetic silencing of its gene. On the contrary, some tumors may display increased MGMT activity when compared to their corresponding healthy tissue (38) thereby presenting an increase in tumor resistance to TMZ treatment. From this perspective, low-level MGMT expression can be considered a favorable predictive marker in TMZ-treated patients (39).
The MGMT-mediated repair system is unique in many aspects, consequently making it a difficult task to target MGMT within tumor cells. The capacity to repair of MGMT depends on the number of active MGMT molecules. If the number of adducts exceeds the preexisting levels of MGMT molecules, the rate of repair will depend on de novo synthesis. This process is important since the effect of TMZ depends both on the level of MGMT present within the tumor cells, as well as the number of DNA adducts (2) and so, an excess amount of DNA adducts can completely exhaust the MGMT catalytic levels.
Recently it has been reported that the DNA oxidative demethylase ALKBH2, capable of directly reversing N1-MeA and N3-MeC in DNA, was abundantly expressed in established GBM cell lines and human GBM; and that TMZ exposure increased cellular ALKBH2 expression levels (40). Therefore, these authors propose that ALKBH2 as a novel mediator of TMZ resistance in human GBM.
Non-bulky modified or damaged bases are removed and repaired by BER (19, 33, 41). As stated earlier, TMZ generates mostly N7-MeG and N3-MeA adducts (90–94%) (Figure 3). These highly toxic adducts are initially removed by DNA methylpurine-N-glycosylase (MPG), also known as alkylpurine-DNA–N-glycosylase (APNG) (42–44). MPG belongs to one of the four glycosylase families involved in BER (44). Agnihotri et al. (42) showed that APNG, together with MGMT, may provide TMZ resistance in an additive manner since, in TMZ resistant GBM cell lines, they are both expressed. In addition, APNG may be epigenetically regulated due to methylation of the APNG gene promoter which attenuates its expression. They also found that GMB patients with nuclear APNG immunostaining in their biopsies had significantly worse overall survival (OS) (42). On the contrary, GBM patients with a methylated MGMT promoter and good survival, also had a greater number of APNG-negative tumors, suggesting that low MGMT and low APNG immunoexpression lead to better TMZ response. Based on these findings, they proposed that in GBM patients with methylated MGMT promoter, evaluation of APNG immunoexpression would be beneficial (42, 45, 46).
Figure 3. TMZ action and DNA repair mechanisms involved in the prevention and correction of alkylation-induced DNA damage. Based on Ref. (19, 42, 47, 48). Abbreviations: BER, base excision repair; DR, direct repair; MMR, mismatch repair; NER, nucleotide excision repair; MPG, DNA methylpurine-N-glycosylase; MGMT, O6-methylguanine-DNA methyltransferase; TMZ, temozolomide; SSB, single-strand breaks; DSB, double-strand breaks; DNA, deoxyribonucleic acid.
In addition, poly(ADP-ribose) polymerase-1 (PARP-1) is an essential protein for the adequate function of BER. Therefore, if PARP-1 function is disrupted, BER will be inhibited in which case the cytotoxic effects caused by TMZ will be increased (19, 45, 48).
The adduct O6-MeG produced by TMZ, if not repaired by MGMT, creates a structural distortion of DNA recognized by MMR (Figure 3). Mismatch repair can correct the error by replacing O6-MeG with guanine or leave single-strand breaks which in turn can generate potentially lethal double-strand breaks (DSB) during DNA replication. Depending on the type of damage, stalling at the replication forks during S phase, cell cycle arrest, cytotoxicity, and cell death can occur (49). If, on the other hand, MMR does not recognize the O6-MeG-thymine mispairs, O6-MeG lesions will be tolerated, and the cells will survive (50). Mutagenesis of the O6-MeG adduct can also arise if the original [06-MeG]::[C] mispair remains unrepaired until the next cell cycle and then, pairing with thymine [06-MeG]::[T] instead of cytosine. Subsequent replication cycles, will create a true stable mutation due to the formation of a [A]::[T] pair. In short, the mutagenesis sequence would be [G]::[C], [06-MeG]::[C], [06-MeG]::[T], [A]::[T].
A successful MMR system is therefore required for TMZ cytotoxicity (19, 47, 49) and MMR deficient cells are resistant to TMZ treatment (Figure 3) (19, 51–53). Publications focusing on the mechanisms of acquiring TMZ resistance in pituitary tumors have shown that one patient presented progression and resistance to TMZ with loss of MSH6 protein immunoexpression during treatment (54) and in another publication, a patient with germline MSH2 mutation was unresponsive to TMZ therapy (55). Therefore, MSH6 and MGMT immunoexpression analysis has been proposed in the morphologic study of pituitary tumors (56–60).
Pituitary adenomas are a heterogeneous group of lesions with different clinical behaviors. Although current treatment protocols have been able to control the majority of pituitary adenomas, some of them may recur despite repeated surgeries, radiotherapy, and pharmacologic treatments. These “difficult to treat” tumors have been called aggressive pituitary adenomas (61, 62) and their prevalence remains uncertain. According to the current (2017) World Health Organization (WHO) classification of tumors of endocrine organs (58, 59), pituitary adenomas can only be characterized as pituitary carcinomas when craniospinal or systemic metastases are found. Pituitary carcinomas are rare and account for approximately 0.12% of pituitary adenomas in the German Pituitary Tumor Registry (63).
The previous (2014) WHO classification characterized a subtype of adenomas as “atypical” if they presented an elevated mitotic index, a Ki-67 labeling index greater than 3%, and extensive nuclear staining for p53 immunoreactivity (64). It was assumed that these atypical adenomas might have an uncertain clinical and biological behavior. This assumption has not been proven and, to date, it also has not been able to accurately predict tumor recurrence or resistance to therapy (65, 66). Thus, in the recent 2017 WHO classification of pituitary adenomas, the term “atypical adenomas” is no longer recommended (59, 67, 68).
In the past, the terms atypical, invasive, and aggressive have been applied to pituitary adenomas in different contexts with diverse interpretations. The terms typical and atypical adenoma should refer only to pathologic features. Invasive and non-invasive to radiological, surgical or morphological findings of invasion, and finally, aggressive and non-aggressive to the clinical behavior (69).
Since 2006, TMZ has been used for the treatment of both aggressive adenomas and pituitary carcinomas. Based on literature search results, to date and to our knowledge, approximately 160 cases have been treated. Numerous publications outlining case reports, retrospective patient studies, clinical practice guidelines, and an international survey have reviewed the outcome of TMZ treatment (39, 66, 70–77). In a recent meta-analysis, the 5-year OS for aggressive pituitary adenomas treated with TMZ was 57.4% and for pituitary carcinomas 56.2% (72). The 5-year progression-free survival was 21.9% for patients with aggressive pituitary adenomas and 36.1% for patients with pituitary carcinomas (72). The mean survival rate of pituitary carcinomas before TMZ was introduced as a treatment option was 1.9 years (78). Hence, TMZ has signified an enormous advancement in the treatment of pituitary tumors (66).
Many parameters may influence response, therefore, with any treatment option, data regarding response rates is of critical importance for clinicians and surgeons. Unfortunately, lack of standardization regarding the criteria to evaluate response rate has generated discrepant results. Some studies consider only complete and partial radiological response as a successful outcome. If the stable radiological disease is included, the response rate for aggressive adenomas varies from 50 to 80.6% and for carcinomas from 50 to 87.6% (72). In aggressive adenomas, TMZ is used as a last resort after various therapeutic options have been exhausted without inhibiting the progression of the tumor. In these cases, as well as in pituitary carcinomas, it should be taken into consideration that a stable disease response may be considered a good response (66).
Remarkably, as it was demonstrated in the largest cohort published (77), when comparing demographic characteristics, functional status of the tumors, previous surgeries, previous radiotherapy, and pathological features, no statistically significant differences were found between patients with aggressive pituitary adenomas and pituitary carcinomas. The only difference was the presence of metastases. Data from this study are illustrated in Figures 4 and 5 (77). Due to the lack of morphologic, biochemical, or molecular biomarkers that can indicate in advance which tumors will behave in an aggressive manner, different designations have been used to describe them: premetastatic lesions in the sellar phase (79), carcinomas in situ (80), localized pituitary carcinomas (81), and invasive/proliferative tumors with high risk of recurrence—individualizing grade 2b tumors, suspected to be carcinomas without metastases (82). It is possible that some of the tumors diagnosed as aggressive adenomas have already undergone the “malignant switch” and have transformed to carcinomas without recognized metastases. Their identification would be of practical significance because it would bring to light better prognosis and treatment options. To recognize the variable behavior and impact of pituitary tumors on patients, a recent proposal to replace the term pituitary adenoma to pituitary neuroendocrine tumor (PitNET) has been made (83).
Figure 4. Temozolomide-treated patients. Demographics, clinical presentation, previous surgeries, and radiotherapy comparing aggressive pituitary adenomas and pituitary carcinomas. Data from Ref. (77).
Figure 5. Pathological subtype of tumors, proliferative markers, and O6-methylguanine-DNA methyltransferase (MGMT) immunoexpression in aggressive pituitary adenomas and pituitary carcinomas treated with temozolomide. Data from Ref. (77).
The decision to start TMZ in pituitary carcinomas is clear (66, 76) but in aggressive pituitary adenomas other factors such as age, previous medical therapy, radiotherapy, number of previous surgical interventions, invasion, proliferation markers, and histologic subtype of the tumor must be considered (66, 84). The benefits of starting TMZ must outweigh the risks of repeated surgeries, re-irradiation, and potential complications with standard treatments, and in all cases, the decision must be considered from an interdisciplinary perspective (74, 76).
Since studies have shown that pituitary tumors with low MGMT protein expression have had positive response to TMZ (39, 76, 77), it is prudent, but not necessary, to perform MGMT immunohistochemical analysis before starting TMZ therapy (76). If this is not possible, or even if MGMT immunoexpression is high, TMZ can be started for 3–6 cycles to determine the response of the tumor to therapy (76). Standard dosage of TMZ is 150–200 mg/m2/day for 5 of every 28 days (5/28). To increase the efficacy of TMZ and tumor response, levels of MGMT should be diminished or depleted. TMZ response is schedule dependent, and alternative dosing regimens may enhance its effectiveness (37, 85). No serious side effects have been reported in TMZ-treated patients with pituitary tumors, and common adverse effects include nausea, vomiting, fatigue, headache, and constipation (66). TMZ is known to affect the formation of sperm in men; thus, couples interested in having children should consider sperm banking before starting treatment. The adverse effects of TMZ and childbearing extend to women as well. Women should not receive TMZ if they expect to become pregnant, are pregnant, or are breastfeeding. In this instance, couples should discuss egg harvesting before treatment initiation.
In TMZ responsive cases, a rapid, early reduction of mass effect has been noted and, in functional tumors, with a remarkable decrease of plasma hormone values (71, 72). As the response can be seen after 3–6 months of therapy, the first imaging evaluation can be performed after three cycles (76). In patients responding to TMZ treatment, cystic change, hemorrhage, tumor necrosis, and shrinkage of the tumor have been seen on MRI (86).
A small number of patients has been treated with a combination of TMZ along with other medications such as capecitabine (87), pasireotide (88, 89), octreotide (90), bevacizumab (91), and thalidomide (76). In patients with functional tumors, the addition of a second medication may be useful. Data regarding these treatment options are limited, and the small number of patients evaluated thus far does not permit an appropriate analysis of these combinations. Their use must be decided according to each case (76).
Drug resistance is a significant problem that restricts the effectiveness of chemotherapeutic therapy. Although TMZ remains part of the gold standard treatment for GBM, it is well known that a subset of tumors does not respond to TMZ treatment despite MGMT inactivation. This is an indication that other mechanisms may also modulate the tolerance to TMZ; therefore, overcoming TMZ resistance is a critical matter that must be considered in order to improve treatment outcomes (48).
Currently, it has been shown that MGMT-mediated repair, counteracts the effects of TMZ treatment (19). The key marker of TMZ resistance is the level of gene methylation and protein expression occurring within the cells. Methylation of the MGMT promoter occurs in approximately 45% of GBM patients, and it has become a reliable prognostic indicator of TMZ therapy in this type of tumor (92). Contrary to GBM, in pituitary tumors, immunohistochemistry analysis of MGMT has been frequently used, and tumors with low MGMT immunoexpression have generally had a better response to treatment (39, 76, 77, 93). Direct repair by MGMT is the most-documented mechanism associated with TMZ drug resistance, but, apart from the level of MGMT present in cells, other mechanisms may also be involved in acquiring resistance to TMZ. To date, various new molecular mechanisms underlying such resistance are emerging.
In pituitary tumors, low-level immunoexpression of MGMT has been associated with a positive response to TMZ, and high MGMT expression, with lack of response. Nevertheless, some cases with low MGMT expression may not respond to TMZ treatment and a few, with high MGMT could respond (39, 72, 76, 77). Temozolomide produces mainly O6-MeG, N7-MeG, and N3-MeA adducts. The first one is repaired by MGMT (by DR) and the other two by MPG (by BER) (Figure 3). Based on recent findings in GBM, it could be hypothesized that MPG may behave in a similar way in pituitary tumors (42, 43, 94). Hence, pituitary tumors showing low MGMT and low MPG expression would be highly responsive to TMZ, because unrepaired O6-MeG, N7-Meg, and N3-MeA adducts, would trigger cytotoxicity by different ways (Table 1). Tumors with high MGMT expression and low MPG could also respond because N7-MeG and N3-MeA adducts, not repaired by BER, would cause cell death. On the other hand, if MPG expression and MGMT were both high, the tumor would be highly resistant because all adducts would be repaired. Finally, if the tumor presented low MGMT and high MPG expression, cytotoxicity or resistance would depend on several factors: an integral MMR pathway, the persistence of mismatch pairing, the tolerance of mutations, and the adequate repair of adducts by BER (Table 1). According to this, in pituitary tumors, it would be advisable to perform immunohistochemical analysis for MGMT as well as for MSH2, MSH6, MLH1, PMS2, and MPG, to evaluate the DNA repair pathways—DR, MMR, and BER, respectively—and the possible response to TMZ therapy (42).
Table 1. Response to temozolomide treatment according to immunoexpression of MGMT, MPG, and functional MMR.
Tumors may be intrinsically resistant to TMZ before initial treatment; however, resistance may also be acquired during treatment in tumors that were previously sensitive to it. This acquired resistance may be the result of drug-induced genetic changes within tumor cells which impart a survival advantage to certain cells (19). Hence, tumor cells that remain sensitive to TMZ treatment will be eradicated while those that have acquired resistance proceed to divide and produce daughter cells that are also resilient to the effects of TMZ. This acquired resistance may be responsible for TMZ treatment failure in individual patients (19, 95), either because those cells already existed before the onset of treatment as natural phenotypes, or due to the drug-induced mutagenicity. The result is an internal natural selection of genetically heterogeneous clones (96–98).
In GBM cases, it has been shown that TMZ treatment failure may also be the result of an inherent resistance conferred by various mechanisms. It was demonstrated that multi-drug resistance proteins (MRP) intrinsically expressed in gliomas did not respond to chemotherapy (99). Multi-drug resistance proteins along with ABC transporter proteins such as P-glycoprotein 1 (permeability glycoprotein, Pgp) have been shown to cause an increase in drug efflux since they can actively transport various drugs out of the cells (99–101). In this instance, resistance may also be the result of decreased drug influx, although the exact mechanism is still unclear.
The Sonic Hedgehog (SHH) pathway plays a crucial role in neural development. Recently it was found that tumor cells use this pathway to acquire and maintain resistance to TMZ. In studies focusing on GBM, SHH signaling in tumor cells was mediated through an overexpression of the MDR1 gene which can cause an increase in drug efflux by actively transporting drugs out of cells (99–102).
Another area that may trigger TMZ resistance is the overall mutagenesis—caused by the drug itself—to the entire genomic cellular DNA, impairing, among others, the capacity of tumor cells to repair its DNA. The ability for cells to counter DNA damage is the determining factor of whether these cells are killed by TMZ treatment or if they can become resistant and survive. As mentioned, TMZ treatment causes methylation mainly in guanine but also in adenine. If these adducts remain unrepaired, they will cause replication fork collapse and DNA DSB, resulting in cell cycle arrest (G2/M) and apoptosis (49). For instance, the MMR system plays a significant role in TMZ treatment since it can recognize and remove mispaired bases and insertion/deletion loops produced during DNA synthesis (Figure 3). Therefore, the MMR system must function properly for TMZ to carry out its cytotoxic effects. In a study by Goellner et al., they looked at the relationship between MMR system failure and TMZ resistance in GBM cases (103). Tumors with low-level MGMT expression responded well, initially, to TMZ treatments but concurrently accumulated mutations within the DNA of surviving cells. It was believed that the surviving tumor cells acquired MMR mutations that then resulted in TMZ resistance during further TMZ treatments.
Adaptive response to alkylating agents may have a role in TMZ resistance, especially patients in which TMZ treatment has been discontinued, resulting in tumor progression, and resistance to a second course of treatment (77). The adaptive response to alkylating damage was demonstrated many years ago in studies using E. coli. It was noted that E. coli exposed to non-toxic doses of alkylating agents, became more resistant to mutations and death. They also became more capable of dealing with higher subsequent doses of the same agent (104–106). This response was also confirmed in human cells (107). Methylating agents triggered the adaptive response in E. coli by generating an intracellular signal for its induction (108). If a tumor progresses, tumoral cells may have a similar adaptive response to TMZ, rendering a second course of treatment ineffective (77). In tumor microenvironment MGMT, after its inactivation, may interact with transcription factors of different adaptive response genes, as it has been shown with ROS (109), which induce resistance to subsequent courses of TMZ.
Molecular drug resistance remains a difficult issue to resolve. It can only be overcome by developing new technologies that allow better characterization of novel signaling pathways, involved in tumor cell response to chemotherapeutic agents. This, in turn, will enable researchers and clinicians to design new treatment regimens that will maximize drug efficacy, while eliminating the mechanism behind drug resistance and minimizing unfavorable health effects.
Temozolomide has shown to be an effective treatment for aggressive pituitary adenomas and pituitary carcinomas, yet some tumors escape its anti-tumor activity. This chemoresistance can be counteracted by targeting several biochemical mechanisms. It has been shown that MGMT is an important TMZ resistance factor. Future studies should focus on developing therapeutic agents that can work in conjunction with TMZ to suppress the effects of MGMT in tumor cells or to attenuate TMZ resistance. For example, an ideal MGMT inhibitor would be a molecule that could specifically dock in the MGMT amino acid sequence (Pro-Cys-His-Arg) that contains the active site (110). Second, novel imidazotetrazine analogs have been recently tested in orthotopic mouse xenografts that showed significantly better brain to plasma ratios compared with TMZ (111). Researchers used imidazotetrazine analogs that acted in the same manner as TMZ yet treatment using these compounds showed lipophilic binding to the entire brain tissue, reducing its availability to the target tumor cells. Nevertheless, chemical modifications are attainable and are under investigation (111). Future research should target the competition of TMZ with the P-glycoprotein (P-gp) which acts as a drug efflux pump that expels the drugs from the cell, reducing its effectiveness in the membrane of tumor cells. Different P-gp inhibitors have been discovered thereby paving the way for an alternative form of combined therapies (112). High levels of MGMT in tumor tissue may be depleted with pseudo-substrates that resemble 06-MeG. O6-benzylguanine and lomeguatrib can deplete MGMT and increase TMZ cytotoxicity (113, 114). Manipulating the BER pathway using methoxyamine can increase TMZ efficacy. To begin the repair process of alkylation, the BER pathway removes the alkylated DNA base, creating an apurinic/apyrimidinic (AP) site which is then repaired. Methoxyamine can form a stable adduct in the AP site, refractory to the downstream members of the BER pathway (46). Future research should focus on developing drug combinations that target various mechanisms. Bevacizumab has been used alone as an alternative in a case of one pituitary carcinoma resistant to TMZ therapy (115). Combined anti-angiogenic therapy along with TMZ treatment could be beneficial, but at this time, the small number of patients treated with this option does not allow proper analysis of the effects of this combination (77). Because of the limited availability of clinical samples and the high cost of performing clinical trials, the use of preclinical in vitro human cell line models should be encouraged (116).
The etiology from aggressive to malignant pituitary tumor remains largely unknown. Genomic studies to decipher the driving events are ongoing but are hampered by the limited understanding of the mechanism underlining the tumorigenesis of pituitary adenomas. Novel studies are focusing on changes in microRNAs expression—such as miR-183 and KIAA0101—that result from inhibition of specific transcription factors which would, in turn, lead to overexpression of genes and other molecular events that are upregulated in aggressive tumors. This “aggressive pathway” leads to the activation of cell proliferation responsible for pituitary tumor progression (117). Researchers have also focused on a protein which is involved in estrogen receptor transactivation and metalloproteinase-9 (MMP-9) expression. It was shown that elevated expression of metastasis-associated gene-1 (MTA1) was linked to the aggressive nature of pituitary tumors (118).
A complex network of DNA damage response (DDR) is triggered after damage to tumoral DNA (119). This DDR involves multiple repair mechanisms, cell cycle checkpoints, and tolerance to damage. According to the nature of the DNA injury and the phase of the cell cycle in which the lesion is produced, the cell cycle can be arrested at the G1/S transition, within the S-phase, or at the G2/M transition (119). While potentiation of TMZ treatment can be enhanced by inhibiting the mechanisms behind drug resistance, novel approaches to inhibit the effects of MGMT, BER, and MMR, that invariably arm tumor cells with the ability to combat TMZ activity, must be developed and implemented. Dysfunction of one DNA repair pathway may be compensated for another one, which may contribute to resistance to TMZ treatment in pituitary tumors.
Temozolomide has shown to be effective in the treatment of aggressive pituitary adenomas and pituitary carcinomas. Overcoming and treating TMZ resistance has been a difficult clinical challenge. Although medical management of TMZ resistance is limited at this time, great strides to understand its underlying mechanisms are being taken. It is evident that arduous challenges persist and that it is crucial that research focus on the development of novel drugs that either enhance the effectiveness of TMZ or overcome its resistance. This will also be beneficial in an attempt for personalized and targeted therapy for pituitary tumors.
LS, FR, and KK conceived the study. LS, FR, MC, LO, and CS searched the literature and extracted the data. LS, FR, and MC wrote the manuscript. LO, CS, and KK contributed to the initial revision of the manuscript. All authors contributed to the critical revision of the manuscript before publication and approved the final version.
The authors declare that the research was conducted in the absence of any commercial or financial relationships that could be construed as a potential conflict of interest.
Authors are grateful to the Jarislowsky and Lloyd Carr-Harris Foundations for their continuous support.
1. Stupp R, Mason WP, van den Bent MJ, Weller M, Fisher B, Taphoorn MJ, et al. Radiotherapy plus concomitant and adjuvant temozolomide for glioblastoma. N Engl J Med (2005) 352(10):987–96. doi:10.1056/NEJMoa043330
2. Friedman HS, Kerby T, Calvert H. Temozolomide and treatment of malignant glioma. Clin Cancer Res (2000) 6(7):2585–97.
3. Lim S, Shahinian H, Maya MM, Yong W, Heaney AP. Temozolomide: a novel treatment for pituitary carcinoma. Lancet Oncol (2006) 7(6):518–20. doi:10.1016/S1470-2045(06)70728-8
4. Syro LV, Uribe H, Penagos LC, Ortiz LD, Fadul CE, Horvath E, et al. Antitumour effects of temozolomide in a man with a large, invasive prolactin-producing pituitary neoplasm. Clin Endocrinol (2006) 65(4):552–3. doi:10.1111/j.1365-2265.2006.02653.x
5. Fadul CE, Kominsky AL, Meyer LP, Kingman LS, Kinlaw WB, Rhodes CH, et al. Long-term response of pituitary carcinoma to temozolomide. Report of two cases. J Neurosurg (2006) 105(4):621–6. doi:10.3171/jns.2006.105.4.621
6. Krumbhaar EB, Krumbhaar HD. The blood and bone marrow in yelloe cross gas (mustard gas) poisoning: changes produced in the bone marrow of fatal cases. J Med Res (1919) 40(3):497–508.3.
7. Goodman LS, Wintrobe MM, Dameshek W, Goodman MJ, Gilman A, McLennan MT Nitrogen mustard therapy; use of methyl-bis(beta-chloroethyl) amine hydrochloride and tris (beta-chloroethyl) amine hydrochloride for Hodgkin’s disease, lymphosarcoma, leukemia and certain allied and miscellaneous disorders. J Am Med Assoc (1946) 132:126–32. doi:10.1001/jama.1946.02870380008004
8. Fu D, Calvo JA, Samson LD. Balancing repair and tolerance of DNA damage caused by alkylating agents. Nat Rev Cancer (2012) 12(2):104–20. doi:10.1038/nrc3185
9. Puyo S, Montaudon D, Pourquier P. From old alkylating agents to new minor groove binders. Crit Rev Oncol Hematol (2014) 89(1):43–61. doi:10.1016/j.critrevonc.2013.07.006
10. Kim K-W, Roh JK, Wee H-J, Kim C. Alkylating Anticancer Drugs. Cancer Drug Discovery Science and History. 1st ed. Seoul: Springer (2016). p. 71–94.
11. Cheng J, Ye F, Dan G, Zhao Y, Wang B, Zhao J, et al. Bifunctional alkylating agent-mediated MGMT-DNA cross-linking and its proteolytic cleavage in 16HBE cells. Toxicol Appl Pharmacol (2016) 305:267–73. doi:10.1016/j.taap.2016.06.022
12. Beranek DT. Distribution of methyl and ethyl adducts following alkylation with monofunctional alkylating agents. Mutat Res (1990) 231(1):11–30. doi:10.1016/0027-5107(90)90173-2
13. Kondo N, Takahashi A, Ono K, Ohnishi T. DNA damage induced by alkylating agents and repair pathways. J Nucleic Acids (2010) 2010:543531. doi:10.4061/2010/543531
14. Drabløs F, Feyzi E, Aas PA, Vaagbø CB, Kavli B, Bratlie MS, et al. Alkylation damage in DNA and RNA—repair mechanisms and medical significance. DNA Repair (2004) 3(11):1389–407. doi:10.1016/j.dnarep.2004.05.004
15. Shrivastav N, Li D, Essigmann JM. Chemical biology of mutagenesis and DNA repair: cellular responses to DNA alkylation. Carcinogenesis (2010) 31(1):59–70. doi:10.1093/carcin/bgp262
16. Gates KS. An overview of chemical processes that damage cellular DNA: spontaneous hydrolysis, alkylation, and reactions with radicals. Chem Res Toxicol (2009) 22(11):1747–60. doi:10.1021/tx900242k
17. Kaina B, Christmann M, Naumann S, Roos WP. MGMT: key node in the battle against genotoxicity, carcinogenicity and apoptosis induced by alkylating agents. DNA Repair (2007) 6(8):1079–99. doi:10.1016/j.dnarep.2007.03.008
18. Warren JJ, Forsberg LJ, Beese LS. The structural basis for the mutagenicity of O6-methyl-guanine lesions. Proc Natl Acad Sci U S A (2006) 103(52):19701–6. doi:10.1073/pnas.0609580103
19. Zhang J, Stevens MF, Bradshaw TD. Temozolomide: mechanisms of action, repair and resistance. Curr Mol Pharmacol (2012) 5(1):102–14. doi:10.2174/1874467211205010102
20. Marchesi F, Turriziani M, Tortorelli G, Avvisati G, Torino F, De Vecchis L. Triazene compounds: mechanism of action and related DNA repair systems. Pharmacol Res (2007) 56(4):275–87. doi:10.1016/j.phrs.2007.08.003
21. Stevens MF, Hickman JA, Langdon SP, Chubb D, Vickers L, Stone R, et al. Antitumor activity and pharmacokinetics in mice of 8-carbamoyl-3-methyl-imidazo[5,1-d]-1,2,3,5-tetrazin-4(3H)-one (CCRG 81045; M & B 39831), a novel drug with potential as an alternative to dacarbazine. Cancer Res (1987) 47(22):5846–52.
22. Tsang LL, Farmer PB, Gescher A, Slack JA. Characterisation of urinary metabolites of temozolomide in humans and mice and evaluation of their cytotoxicity. Cancer Chemother Pharmacol (1990) 26(6):429–36. doi:10.1007/BF02994094
23. Neidle S, Thurston DE. Chemical approaches to the discovery and development of cancer therapies. Nat Rev Cancer (2005) 5(4):285–96. doi:10.1038/nrc1587
24. Rottenberg DA, Ginos JZ, Kearfott KJ, Junck L, Dhawan V, Jarden JO. In vivo measurement of brain tumor pH using [11C]DMO and positron emission tomography. Ann Neurol (1985) 17(1):70–9. doi:10.1002/ana.410170116
25. Van Houten B, Santa-Gonzalez GA, Camargo M. DNA repair after oxidative stress: current challenges. Curr Opin Toxicol (2018) 7:9–16. doi:10.1016/j.cotox.2017.10.009
26. Friedberg EC. A history of the DNA repair and mutagenesis field: the discovery of base excision repair. DNA Repair (2016) 37:A35–9. doi:10.1016/j.dnarep.2015.12.003
27. Richman S. Deficient mismatch repair: read all about it (review). Int J Oncol (2015) 47(4):1189–202. doi:10.3892/ijo.2015.3119
28. Jiricny J. The multifaceted mismatch-repair system. Nat Rev Mol Cell Biol (2006) 7(5):335–46. doi:10.1038/nrm1907
30. Ganai RA, Johansson E. DNA replication – a matter of fidelity. Mol Cell (2016) 62(5):745–55. doi:10.1016/j.molcel.2016.05.003
31. Kunkel TA, Erie DA. Eukaryotic mismatch repair in relation to DNA replication. Annu Rev Genet (2015) 49:291–313. doi:10.1146/annurev-genet-112414-054722
32. Mitra S, Kaina B. Regulation of repair of alkylation damage in mammalian genomes. Prog Nucleic Acid Res Mol Biol (1993) 44:109–42. doi:10.1016/S0079-6603(08)60218-4
33. Wood RD, Mitchell M, Sgouros J, Lindahl T. Human DNA repair genes. Science (2001) 291(5507):1284–9. doi:10.1126/science.1056154
34. Groothuizen FS, Sixma TK. The conserved molecular machinery in DNA mismatch repair enzyme structures. DNA Repair (2016) 38:14–23. doi:10.1016/j.dnarep.2015.11.012
35. Kunkel TA, Erie DA. DNA mismatch repair. Annu Rev Biochem (2005) 74:681–710. doi:10.1146/annurev.biochem.74.082803.133243
36. Pegg AE. Properties of mammalian O6-alkylguanine-DNA transferases. Mutat Res (1990) 233(1):165–75. doi:10.1016/0027-5107(90)90160-6
37. Wick W, Platten M, Weller M. New (alternative) temozolomide regimens for the treatment of glioma. Neuro Oncol (2009) 11(1):69–79. doi:10.1215/15228517-2008-078
38. Christmann M, Verbeek B, Roos WP, Kaina B. O(6)-Methylguanine-DNA methyltransferase (MGMT) in normal tissues and tumors: enzyme activity, promoter methylation and immunohistochemistry. Biochim Biophys Acta (2011) 1816(2):179–90. doi:10.1016/j.bbcan.2011.06.002
39. Bengtsson D, Schroder HD, Andersen M, Maiter D, Berinder K, Feldt Rasmussen U, et al. Long-term outcome and MGMT as a predictive marker in 24 patients with atypical pituitary adenomas and pituitary carcinomas given treatment with temozolomide. J Clin Endocrinol Metab (2015) 100(4):1689–98. doi:10.1210/jc.2014-4350
40. Johannessen TC, Prestegarden L, Grudic A, Hegi ME, Tysnes BB, Bjerkvig R. The DNA repair protein ALKBH2 mediates temozolomide resistance in human glioblastoma cells. Neuro Oncol (2013) 15(3):269–78. doi:10.1093/neuonc/nos301
41. Ignatov AV, Bondarenko KA, Makarova AV. Non-bulky lesions in human DNA: the ways of formation, repair, and replication. Acta naturae (2017) 9(3):12–26.
42. Agnihotri S, Gajadhar AS, Ternamian C, Gorlia T, Diefes KL, Mischel PS, et al. Alkylpurine-DNA-N-glycosylase confers resistance to temozolomide in xenograft models of glioblastoma multiforme and is associated with poor survival in patients. J Clin Invest (2012) 122(1):253–66. doi:10.1172/JCI59334
43. Fosmark S, Hellwege S, Dahlrot RH, Jensen KL, Derand H, Lohse J, et al. APNG as a prognostic marker in patients with glioblastoma. PLoS One (2017) 12(6):e0178693. doi:10.1371/journal.pone.0178693
44. Jacobs AL, Schar P. DNA glycosylases: in DNA repair and beyond. Chromosoma (2012) 121(1):1–20. doi:10.1007/s00412-011-0347-4
45. Liuzzi M, Talpaert-Borle M. A new approach to the study of the base-excision repair pathway using methoxyamine. J Biol Chem (1985) 260(9):5252–8.
46. Fishel ML, He Y, Smith ML, Kelley MR. Manipulation of base excision repair to sensitize ovarian cancer cells to alkylating agent temozolomide. Clin Cancer Res (2007) 13(1):260–7. doi:10.1158/1078-0432.CCR-06-1920
47. Fan CH, Liu WL, Cao H, Wen C, Chen L, Jiang G. O6-methylguanine DNA methyltransferase as a promising target for the treatment of temozolomide-resistant gliomas. Cell Death Dis (2013) 4:e876. doi:10.1038/cddis.2013.388
48. Yoshimoto K, Mizoguchi M, Hata N, Murata H, Hatae R, Amano T, et al. Complex DNA repair pathways as possible therapeutic targets to overcome temozolomide resistance in glioblastoma. Front Oncol (2012) 2:186. doi:10.3389/fonc.2012.00186
49. Tentori L, Graziani G. Recent approaches to improve the antitumor efficacy of temozolomide. Curr Med Chem (2009) 16(2):245–57. doi:10.2174/092986709787002718
50. Karran P. Mechanisms of tolerance to DNA damaging therapeutic drugs. Carcinogenesis (2001) 22(12):1931–7. doi:10.1093/carcin/22.12.1931
51. Zhang J, Stevens MF, Hummersone M, Madhusudan S, Laughton CA, Bradshaw TD. Certain imidazotetrazines escape O6-methylguanine-DNA methyltransferase and mismatch repair. Oncology (2011) 80(3–4):195–207. doi:10.1159/000327837
52. Hunter C, Smith R, Cahill DP, Stephens P, Stevens C, Teague J, et al. A hypermutation phenotype and somatic MSH6 mutations in recurrent human malignant gliomas after alkylator chemotherapy. Cancer Res (2006) 66(8):3987–91. doi:10.1158/0008-5472.CAN-06-0127
53. Cahill DP, Levine KK, Betensky RA, Codd PJ, Romany CA, Reavie LB, et al. Loss of the mismatch repair protein MSH6 in human glioblastomas is associated with tumor progression during temozolomide treatment. Clin Cancer Res (2007) 13(7):2038–45. doi:10.1158/1078-0432.CCR-06-2149
54. Murakami M, Mizutani A, Asano S, Katakami H, Ozawa Y, Yamazaki K, et al. A mechanism of acquiring temozolomide resistance during transformation of atypical prolactinoma into prolactin-producing pituitary carcinoma: case report. Neurosurgery (2011) 68(6):E1761–7; discussion E7. doi:10.1227/NEU.0b013e318217161a
55. Bengtsson D, Joost P, Aravidis C, Askmalm Stenmark M, Backman AS, Melin B, et al. Corticotroph pituitary carcinoma in a patient with Lynch syndrome (LS) and pituitary tumors in a nationwide LS cohort. J Clin Endocrinol Metab (2017) 102(11):3928–32. doi:10.1210/jc.2017-01401
56. Hirohata T, Asano K, Ogawa Y, Takano S, Amano K, Isozaki O, et al. DNA mismatch repair protein (MSH6) correlated with the responses of atypical pituitary adenomas and pituitary carcinomas to temozolomide: the national cooperative study by the Japan society for hypothalamic and pituitary tumors. J Clin Endocrinol Metab (2013) 98(3):1130–6. doi:10.1210/jc.2012-2924
57. Micko ASG, Wohrer A, Hoftberger R, Vila G, Marosi C, Knosp E, et al. MGMT and MSH6 immunoexpression for functioning pituitary macroadenomas. Pituitary (2017) 20(6):643–53. doi:10.1007/s11102-017-0829-3
58. Osamura RY, Lopes MBS, Grossman A, Kontogeorgos G, Trouillas J. Tumours of the pitutary gland. Introduction. 4th ed. In: Lloyd RV, Osamura RY, Klöppel G, Rosai J, editors. WHO Classification of Tumours of Endocrine Organs. Lyon: IARC Press (2017). 13 p.
59. Osamura RY, Grossman A, Korbonits M, Kovacs K, Lopes MBS, Matsuno A, et al. Pituitary adenoma. 4th ed. In: Lloyd RV, Osamura RY, Klöppel G, editors. WHO Classification of Tumours of Endocrine Organs. Lyon: IARC Press (2017). p. 14–8.
60. Matsuno A, Murakami M, Hoya K, Yamada SM, Miyamoto S, Yamada S, et al. Molecular status of pituitary carcinoma and atypical adenoma that contributes the effectiveness of temozolomide. Med Mol Morphol (2014) 47(1):1–7. doi:10.1007/s00795-013-0050-z
61. Buchfelder M. Management of aggressive pituitary adenomas: current treatment strategies. Pituitary (2009) 12(3):256–60. doi:10.1007/s11102-008-0153-z
62. Di Ieva A, Rotondo F, Syro LV, Cusimano MD, Kovacs K. Aggressive pituitary adenomas – diagnosis and emerging treatments. Nat Rev Endocrinol (2014) 10(7):423–35. doi:10.1038/nrendo.2014.64
63. Saeger W, Ludecke DK, Buchfelder M, Fahlbusch R, Quabbe HJ, Petersenn S. Pathohistological classification of pituitary tumors: 10 years of experience with the German pituitary tumor registry. Eur J Endocrinol (2007) 156(2):203–16. doi:10.1530/eje.1.02326
64. Lloyd RV, Kovacs K, Young WF Jr, Farrell W, Asa SL, Trouillas J, et al. Pituitary tumors: introduction. In: DeLlelis RA, Lloyd RV, Heitz PU, Eng C, editors. Pathology and Genetics of Tumours of Endocrine Organs. Lyon, France: IARC press (2004). p. 10–3.
65. Miermeister CP, Petersenn S, Buchfelder M, Fahlbusch R, Ludecke DK, Holsken A, et al. Histological criteria for atypical pituitary adenomas – data from the German pituitary adenoma registry suggests modifications. Acta Neuropathol Commun (2015) 3:50. doi:10.1186/s40478-015-0229-8
66. Syro LV, Rotondo F, Ortiz LD, Kovacs K. Treatment of pituitary tumors with temozolomide: an update. Endocr Relat Cancer (2018). doi:10.1530/ERC-18-0015
67. Lopes MBS. The 2017 World Health Organization classification of tumors of the pituitary gland: a summary. Acta Neuropathol (2017) 134(4):521–35. doi:10.1007/s00401-017-1769-8
68. Mete O, Lopes MB. Overview of the 2017 WHO classification of pituitary tumors. Endocr Pathol (2017) 28(3):228–43. doi:10.1007/s12022-017-9498-z
69. Sav A, Rotondo F, Syro LV, Di Ieva A, Cusimano MD, Kovacs K. Invasive, atypical and aggressive pituitary adenomas and carcinomas. Endocrinol Metab Clin North Am (2015) 44(1):99–104. doi:10.1016/j.ecl.2014.10.008
70. Almalki MH, Aljoaib NN, Alotaibi MJ, Aldabas BS, Wahedi TS, Ahmad MH, et al. Temozolomide therapy for resistant prolactin-secreting pituitary adenomas and carcinomas: a systematic review. Hormones (Athens) (2017) 16(2):139–49. doi:10.14310/horm.2002.1729
71. Halevy C, Whitelaw BC. How effective is temozolomide for treating pituitary tumours and when should it be used? Pituitary (2017) 20(2):261–6. doi:10.1007/s11102-016-0745-y
72. Ji Y, Vogel RI, Lou E. Temozolomide treatment of pituitary carcinomas and atypical adenomas: systematic review of case reports. Neurooncol Pract (2016) 3(3):188–95. doi:10.1093/nop/npv059
73. Lasolle H, Cortet C, Castinetti F, Cloix L, Caron P, Delemer B, et al. Temozolomide treatment can improve overall survival in aggressive pituitary tumors and pituitary carcinomas. Eur J Endocrinol (2017) 176(6):769–77. doi:10.1530/EJE-16-0979
74. Lin AL, Sum MW, DeAngelis LM. Is there a role for early chemotherapy in the management of pituitary adenomas? Neuro Oncol (2016) 18(10):1350–6. doi:10.1093/neuonc/now059
75. Losa M, Bogazzi F, Cannavo S, Ceccato F, Curto L, De Marinis L, et al. Temozolomide therapy in patients with aggressive pituitary adenomas or carcinomas. J Neurooncol (2016) 126(3):519–25. doi:10.1007/s11060-015-1991-y
76. Raverot G, Burman P, McCormack A, Heaney A, Petersenn S, Popovic V, et al. European society of endocrinology clinical practice guidelines for the management of aggressive pituitary tumours and carcinomas. Eur J Endocrinol (2018) 178(1):G1–24. doi:10.1530/EJE-17-0796
77. McCormack AI, Dekkers O, Petersenn S, Popovic V, Trouillas J, Raverot G, et al. Treatment of aggressive pituitary tumours and carcinomas: results of a European society of endocrinology (ESE) survey 2016. Eur J Endocrinol (2018) 178(3):265–76. doi:10.1530/EJE-17-0933
78. Kaltsas GA, Nomikos P, Kontogeorgos G, Buchfelder M, Grossman AB. Clinical review: diagnosis and management of pituitary carcinomas. J Clin Endocrinol Metab (2005) 90(5):3089–99. doi:10.1210/jc.2004-2231
79. Scheithauer BW, Gaffey TA, Lloyd RV, Sebo TJ, Kovacs KT, Horvath E, et al. Pathobiology of pituitary adenomas and carcinomas. Neurosurgery (2006) 59(2):341–53; discussion 53. doi:10.1227/01.NEU.0000223437.51435.6E
80. Heaney AP. Pituitary carcinoma: difficult diagnosis and treatment. J Clin Endocrinol Metab (2011) 96(12):3649–60. doi:10.1210/jc.2011-2031
81. Syro LV, Rotondo F, Cusimano MD, Kovacs K. Aggressive pituitary tumors or localized pituitary carcinomas. 14th International Pituitary Congress, 2015. San Diego, CA, USA: The Pituitary Society (2015).
82. Trouillas J, Roy P, Sturm N, Dantony E, Cortet-Rudelli C, Viennet G, et al. A new prognostic clinicopathological classification of pituitary adenomas: a multicentric case-control study of 410 patients with 8 years post-operative follow-up. Acta Neuropathol (2013) 126(1):123–35. doi:10.1007/s00401-013-1084-y
83. Asa SL, Casar-Borota O, Chanson P, Delgrange E, Earls P, Ezzat S, et al. From pituitary adenoma to pituitary neuroendocrine tumor (PitNET): an International pituitary pathology club proposal. Endocr Relat Cancer (2017) 24(4):C5–8. doi:10.1530/ERC-17-0004
84. Ceccato F, Regazzo D, Barbot M, Denaro L, Emanuelli E, Borsetto D, et al. Early recognition of aggressive pituitary adenomas: a single-centre experience. Acta Neurochir (Wien) (2018) 160(1):49–55. doi:10.1007/s00701-017-3396-5
85. Liu L, Gerson SL. Targeted modulation of MGMT: clinical implications. Clin Cancer Res (2006) 12(2):328–31. doi:10.1158/1078-0432.CCR-05-2543
86. Syro LV, Ortiz LD, Scheithauer BW, Lloyd R, Lau Q, Gonzalez R, et al. Treatment of pituitary neoplasms with temozolomide: a review. Cancer (2011) 117(3):454–62. doi:10.1002/cncr.25413
87. Zacharia BE, Gulati AP, Bruce JN, Carminucci AS, Wardlaw SL, Siegelin M, et al. High response rates and prolonged survival in patients with corticotroph pituitary tumors and refractory cushing disease from capecitabine and temozolomide (CAPTEM): a case series. Neurosurgery (2014) 74(4):E447–55; discussion E55. doi:10.1227/NEU.0000000000000251
88. Ceccato F, Lombardi G, Manara R, Emanuelli E, Denaro L, Milanese L, et al. Temozolomide and pasireotide treatment for aggressive pituitary adenoma: expertise at a tertiary care center. J Neurooncol (2015) 122(1):189–96. doi:10.1007/s11060-014-1702-0
89. Bode H, Seiz M, Lammert A, Brockmann MA, Back W, Hammes HP, et al. SOM230 (pasireotide) and temozolomide achieve sustained control of tumour progression and ACTH secretion in pituitary carcinoma with widespread metastases. Exp Clin Endocrinol Diabetes (2010) 118(10):760–3. doi:10.1055/s-0030-1253419
90. Vieira Neto L, Chimelli L, Pereira PJ, Gasparetto EL, Bines J, Wildemberg LE, et al. The role of temozolomide in the treatment of a patient with a pure silent pituitary somatotroph carcinoma. Endocr Pract (2013) 19(6):e145–9. doi:10.4158/EP12400.CR
91. Touma W, Hoostal S, Peterson RA, Wiernik A, SantaCruz KS, Lou E. Successful treatment of pituitary carcinoma with concurrent radiation, temozolomide, and bevacizumab after resection. J Clin Neurosci (2017) 41:75–7. doi:10.1016/j.jocn.2017.02.052
92. Hegi ME, Diserens AC, Godard S, Dietrich PY, Regli L, Ostermann S, et al. Clinical trial substantiates the predictive value of O-6-methylguanine-DNA methyltransferase promoter methylation in glioblastoma patients treated with temozolomide. Clin Cancer Res (2004) 10(6):1871–4. doi:10.1158/1078-0432.CCR-03-0384
93. McCormack AI, Wass JA, Grossman AB. Aggressive pituitary tumours: the role of temozolomide and the assessment of MGMT status. Eur J Clin Invest (2011) 41(10):1133–48. doi:10.1111/j.1365-2362.2011.02520.x
94. Liu C, Tu Y, Yuan J, Mao X, He S, Wang L, et al. Aberrant expression of N-methylpurine-DNA glycosylase influences patient survival in malignant gliomas. J Biomed Biotechnol (2012) 2012:760679. doi:10.1155/2012/760679
95. Longley DB, Johnston PG. Molecular mechanisms of drug resistance. J Pathol (2005) 205(2):275–92. doi:10.1002/path.1706
96. Fortunato A, Boddy A, Mallo D, Aktipis A, Maley CC, Pepper JW. Natural selection in cancer biology: from molecular snowflakes to trait hallmarks. Cold Spring Harb Perspect Med (2017) 7(2):a029652. doi:10.1101/cshperspect.a029652
97. Scott J, Marusyk A. Somatic clonal evolution: a selection-centric perspective. Biochim Biophys Acta (2017) 1867(2):139–50. doi:10.1016/j.bbcan.2017.01.006
98. Gatenby RA, Brown J. Mutations, evolution and the central role of a self-defined fitness function in the initiation and progression of cancer. Biochim Biophys Acta (2017) 1867(2):162–6. doi:10.1016/j.bbcan.2017.03.005
99. Calatozzolo C, Gelati M, Ciusani E, Sciacca FL, Pollo B, Cajola L, et al. Expression of drug resistance proteins Pgp, MRP1, MRP3, MRP5 and GST-pi in human glioma. J Neurooncol (2005) 74(2):113–21. doi:10.1007/s11060-004-6152-7
100. Gottesman MM, Fojo T, Bates SE. Multidrug resistance in cancer: role of ATP-dependent transporters. Nat Rev Cancer (2002) 2(1):48–58. doi:10.1038/nrc706
101. Thomas H, Coley HM. Overcoming multidrug resistance in cancer: an update on the clinical strategy of inhibiting p-glycoprotein. Cancer Control (2003) 10(2):159–65. doi:10.1177/107327480301000207
102. Munoz JL, Rodriguez-Cruz V, Walker ND, Greco SJ, Rameshwar P. Temozolomide resistance and tumor recurrence: halting the hedgehog. Cancer Cell Microenviron (2015) 2(2):e747. doi:10.14800/ccm.747
103. Goellner EM, Grimme B, Brown AR, Lin YC, Wang XH, Sugrue KF, et al. Overcoming temozolomide resistance in glioblastoma via dual inhibition of NAD+ biosynthesis and base excision repair. Cancer Res (2011) 71(6):2308–17. doi:10.1158/0008-5472.CAN-10-3213
104. Kleibl K. Molecular mechanisms of adaptive response to alkylating agents in Escherichia coli and some remarks on O6-methylguanine DNA-methyltransferase in other organisms. Mutat Res (2002) 512(1):67–84. doi:10.1016/S1383-5742(02)00025-X
105. Jeggo P, Defais M, Samson L, Schendel P. An adaptive response of E. coli to low levels of alkylating agent: comparison with previously characterised DNA repair pathways. Mol Gen Genet (1977) 157:1–9. doi:10.1007/BF00268680
106. Samson L, Cairns J. A new pathway for DNA repair in Escherichia coli. Nature (1977) 267:281. doi:10.1038/267281a0
107. Olivieri G, Bodycote J, Wolff S. Adaptive response of human lymphocytes to low concentrations of radioactive thymidine. Science (1984) 223(4636):594–7. doi:10.1126/science.6695170
108. Lindahl T, Sedwich B, Sekiguchi M, Nakabeppu Y. Regulation and expression of the adaptive response to alkylating agents. Annu Rev Biochem (1988) 57:133–57. doi:10.1146/annurev.bi.57.070188.001025
109. Santa-Gonzalez GA, Gomez-Molina A, Arcos-Burgos M, Meyer JN, Camargo M. Distinctive adaptive response to repeated exposure to hydrogen peroxide associated with upregulation of DNA repair genes and cell cycle arrest. Redox Biol (2016) 9:124–33. doi:10.1016/j.redox.2016.07.004
110. Chueh LL, Nakamura T, Nakatsu Y, Sakumi K, Hayakawa H, Sekiguchi M. Specific amino acid sequences required for O6-methylguanine-DNA methyltransferase activity: analyses of three residues at or near the methyl acceptor site. Carcinogenesis (1992) 13(5):837–43. doi:10.1093/carcin/13.5.837
111. Rai R, Banerjee M, Wong DH, McCullagh E, Gupta A, Tripathi S, et al. Temozolomide analogs with improved brain/plasma ratios – exploring the possibility of enhancing the therapeutic index of temozolomide. Bioorg Med Chem Lett (2016) 26(20):5103–9. doi:10.1016/j.bmcl.2016.08.064
112. Munoz JL, Walker ND, Scotto KW, Rameshwar P. Temozolomide competes for P-glycoprotein and contributes to chemoresistance in glioblastoma cells. Cancer Lett (2015) 367(1):69–75. doi:10.1016/j.canlet.2015.07.013
113. Ranson M, Middleton MR, Bridgewater J, Lee SM, Dawson M, Jowle D, et al. Lomeguatrib, a potent inhibitor of O6-alkylguanine-DNA-alkyltransferase: phase I safety, pharmacodynamic, and pharmacokinetic trial and evaluation in combination with temozolomide in patients with advanced solid tumors. Clin Cancer Res (2006) 12(5):1577–84. doi:10.1158/1078-0432.CCR-05-2198
114. Curtin NJ. DNA repair dysregulation from cancer driver to therapeutic target. Nat Rev Cancer (2012) 12:801. doi:10.1038/nrc3399
115. Ortiz LD, Syro LV, Scheithauer BW, Ersen A, Uribe H, Fadul CE, et al. Anti-VEGF therapy in pituitary carcinoma. Pituitary (2012) 15(3):445–9. doi:10.1007/s11102-011-0346-8
116. Niu N, Wang L. In vitro human cell line models to predict clinical response to anticancer drugs. Pharmacogenomics (2015) 16(3):273–85. doi:10.2217/pgs.14.170
117. Roche M, Wierinckx A, Croze S, Rey C, Legras-Lachuer C, Morel AP, et al. Deregulation of miR-183 and KIAA0101 in aggressive and malignant pituitary tumors. Front Med (2015) 2:54. doi:10.3389/fmed.2015.00054
118. Jia W, Zhu J, Martin TA, Sanders AJ, Yang X, Cheng S, et al. Expression of metastasis-associated gene-1 is associated with bone invasion and tumor stage in human pituitary adenomas. Cancer Genomics Proteomics (2015) 12(3):113–8.
Keywords: alkylating agents, chemotherapy, DNA repair, neoplasms, neuroendocrine tumors, O(6)-Methylguanine-DNA Methyltransferase, pituitary, temozolomide
Citation: Syro LV, Rotondo F, Camargo M, Ortiz LD, Serna CA and Kovacs K (2018) Temozolomide and Pituitary Tumors: Current Understanding, Unresolved Issues, and Future Directions. Front. Endocrinol. 9:318. doi: 10.3389/fendo.2018.00318
Received: 31 March 2018; Accepted: 28 May 2018;
Published: 15 June 2018
Edited by:
Hidenori Fukuoka, Kobe University, JapanReviewed by:
Maria Mercedes Pineyro, University of the Republic, UruguayCopyright: © 2018 Syro, Rotondo, Camargo, Ortiz, Serna and Kovacs. This is an open-access article distributed under the terms of the Creative Commons Attribution License (CC BY). The use, distribution or reproduction in other forums is permitted, provided the original author(s) and the copyright owner are credited and that the original publication in this journal is cited, in accordance with accepted academic practice. No use, distribution or reproduction is permitted which does not comply with these terms.
*Correspondence: Luis V. Syro, bHZzeXJvQGdtYWlsLmNvbQ==
Disclaimer: All claims expressed in this article are solely those of the authors and do not necessarily represent those of their affiliated organizations, or those of the publisher, the editors and the reviewers. Any product that may be evaluated in this article or claim that may be made by its manufacturer is not guaranteed or endorsed by the publisher.
Research integrity at Frontiers
Learn more about the work of our research integrity team to safeguard the quality of each article we publish.