- Laboratory of Neurobiology, Institute of Zoology, Poznań University of Life Sciences, Poznań, Poland
Apart from the well-established role of kisspeptin (Kp) in the regulation of reproductive functions, recent data described its action in the control of metabolism. Of particular interest for the review is the population of Kp neurons localized in the arcuate nucleus (ARC) of the hypothalamus, the site of the brain where reproductive and metabolic cross talk occurs. However, within the hypothalamus Kp does not work alone, but rather interacts with other neuropeptides, e.g., neurokinin B, dynorphin A, proopiomelanocortin, the cocaine- and amphetamine-regulated transcript, agouti-related peptide, and neuropeptide Y. Beyond the brain, Kp is expressed in peripheral tissues involved in metabolic functions. In this review, we will mainly focus on the local action of this peptide in peripheral organs such as the pancreas, liver, and the adipose tissue. We will concentrate on dysregulation of the Kp system in cases of metabolic imbalance, e.g., obesity and diabetes. Importantly, these patients besides metabolic health problems often suffer from disruptions of the reproductive system, manifested by abnormalities in menstrual cycles, premature child birth, miscarriages in women, decreased testosterone levels and spermatogenesis in men, hypogonadism, and infertility. We will review the evidence from animal models and clinical data indicating that Kp could serve as a promising agent with clinical applications in regulation of reproductive problems in individuals with obesity and diabetes. Finally, emerging data indicate a role of Kp in regulation of insulin secretion, potentially leading to development of further therapeutic uses of this peptide to treat metabolic problems in patients with these lifestyle diseases.
Double Burden of Malnutrition as a Serious Worldwide Concern
A serious public health challenge facing many countries worldwide, recognized by the World Health Organization (WHO), is the double burden of malnutrition. It is characterized by the coexistence of contrasting forms of malnourishment: undernutrition along with overweight (1). Actions proposed by the WHO include interventions, programs and policies to reduce the risk or this burden. Understanding the neuroendocrine background for such conditions could lead to improvement of therapeutic strategies.
Kisspeptin (Kp) as a Possible Link between Metabolism and Reproduction
All organisms need food to meet their functional requirements for immediate metabolic needs and conserve energy excess in the form of fat for times of food scarcity (2). Another crucial and energy demanding process, i.e., reproduction, is needed for the perpetuation of species (3). Kp, encoded by KISS1 in the human and non-human primates and Kiss1 in non-humans, its receptor KISS1R and Kiss1r or GPR54, respectively, are involved in the regulation of the hypothalamic–pituitary–gonadal (HPG) axis (4, 5). Importantly, energy metabolism is tuned to distinct sex-specific functions. Whereas in males metabolism may represent a default state, in females it is linked to specific requirements during gestation, parturition, and lactation (6). The arcuate nucleus (ARC) of the hypothalamus, expressing numerous neuropeptides including Kp, has been an area of focus for both circuits regulating metabolism and reproduction (7, 8).
Role of Kp and Other Neuropeptides in Metabolism Control: Focus on the Brain
Extensive data support a major role of the ARC Kp neurons population in conveying information on metabolism to the gonadotropin-releasing hormone (GnRH) neurons, localized at the apex of the HPG axis (9). Disturbances in positive and negative energy balance often result in impairments of fertility, such as hypothalamic hypogonadism, frequently found in case of obesity and diabetes (10–12). However, Kp does not work alone, as Kp immunoreactive (-ir) neurons in the ARC also express neurokinin B (NKB) and dynorphin A (DYN A) and are termed KNDy neurons, and their degree of colocalization varies among species (13–18). NKB belongs to tachykinins, is encoded by the Tac2 or TAC3 gene (in rodents and humans, respectively), and works via the NK3R receptor [Tacr3 encoded by Tacr3 and TACR3, animals and human genes, respectively (19)]. DYN A is an endogenous opioid (encoded by pDyn and PDYN, animal and human genes, respectively) working alongside the kappa-opioid receptor, encoded by the Oprk1 in animals and OPRK1 in humans genes (13, 20). KNDy neurons regulate the secretion of GnRH, while NKB stimulates and DYN A inhibits Kp (21–24).
Neuropeptide expression in KNDy neurons depends on the metabolic status. In chronically obese female mice a decrease was observed in Kiss1 mRNA in the ARC (25), while fasting reduced Kiss1 mRNA expression in the hypothalamus in rats and mice (10, 26, 27). In diabetic male rats, a decrease in Kiss1 mRNA levels was found in the hypothalamus and a lack of an increase in Kiss1 mRNA after gonadectomy [GDX (28)]. Finally, an increased number of Kp-ir neurons and an altered response after GDX in the ARC were reported in streptozotocin (STZ)-induced diabetic rats (11). Current studies in our laboratory are exploring the effects of obesity, diabetes and GDX in female rats (29).
Neurokinin B expression is also dependent on the metabolic status, as in pubertal female rats fed high-fat diet (HFD) higher expression of Tac2 mRNA in the ARC was found (30). In db/db diabetic mice, Tac2 mRNA levels increased in the hypothalamus (31), while in diabetic type 2 (DM2) male rats the number of NKB-ir neurons in the ARC increased (11). By contrast, under fasting conditions in female rats the expression of Tacr3 and Tac2 mRNA in the ARC decreased (19). The metabolic status was found to influence the DYN system, with an increase in DYN concentrations in the dorsomedial hypothalamic nucleus reported in obese ob/ob mice (32). In HFD-fed adult mice and obese rats, no changes were observed in prodynorphin (proDyn) expression in the ARC (33, 34). In STZ-induced diabetic rats, an increase in proDyn mRNA and protein level in the hypothalamus was shown (35, 36), while DM2 male rats had higher numbers of DYN A-ir in the ARC (11). Food restriction increased proDyn mRNA levels in the lateral hypothalamus (Figure 1) (37).
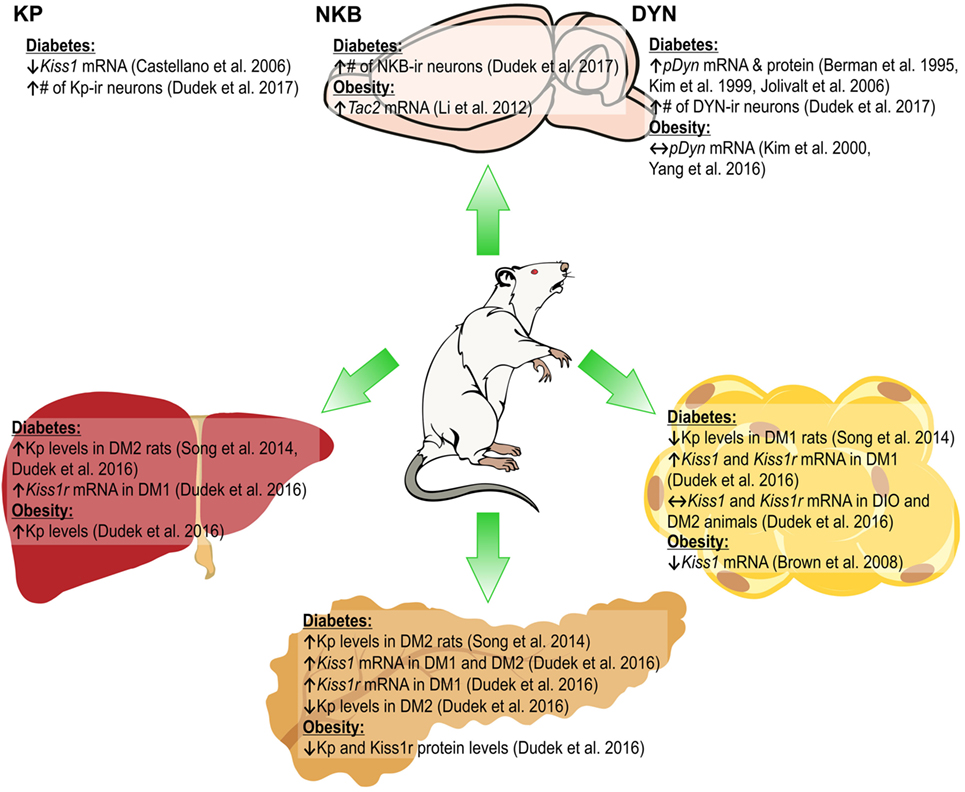
Figure 1. Changes in the expression of KNDy peptides [kisspeptin (Kp), neurokinin B (NKB), and dynorphin (DYN)] in the arcuate nucleus of the hypothalamus (ARC; upper part) and Kiss1/Kiss1r in peripheral organs (lower part) of rat due to diabetes obesity. ↑, increase; ↓, decrease; ↔, no effect (11, 26, 28, 30, 33–36, 54, 56).
These results indicate effects of the metabolic status on the expression of KNDy peptides; however, they may depend on species, strains, sex of animals, as well as the experimental design, e.g., diet composition and duration or diabetes induction methods.
Besides KNDy neurons, in the ARC two other neuron populations may be identified as a link between metabolism and reproduction: cells expressing proopiomelanocortin (POMC) and the cocaine and amphetamine-regulated transcript (CART) and a population expressing agouti-related peptide (AgRP) and neuropeptide Y [NPY (38, 39)]. First, while POMC neurons are suggested to decrease food intake, and catabolic and anabolic effects of CART in the ARC and lateral hypothalamic area, respectively, were reported, NPY/AgRP cells play an important orexigenic role in energy homeostasis (38, 40–42). Second, POMC and NPY/AgRP neurons respond to insulin. Electrophysiological studies have shown that this hormone excites POMC and inhibits NPY/AgRP neurons (43, 44). Third, NPY/AgRP- and POMC-derived peptides, e.g., alpha-melanocyte stimulating hormone, directly influence GnRH neuron excitability (45). Fourth, anatomical data indicate a direct contact of GnRH cells with POMC/CART and NPY/AgRP neurons (8, 46, 47). Fifth, there is evidence that KNDy cells in the ARC are locally connected with AgRP and POMC neurons (48, 49). However, there are differences in the ARC neuropeptides coexpression in the monkey compared to rodents (8). Finally, Kp shows stimulatory effects on POMC and inhibitory effects on NPY neurons in the ARC (50). Thus, both POMC/CART and AgRP/NPY cell populations appear to be good candidates as GnRH regulators (51).
Evidence for the Role of Kp in Metabolism Control: Beyond the Brain
The Kp gene and its receptor are expressed not only in the central nervous system (CNS) but also in the peripheral tissues (52, 53). In this review, we discuss its role in the pancreas, liver, and adipose tissue. In view of two extensive reviews on the subject (52, 53), we will focus mainly on the latest findings in the field.
Several lines of evidence indicate a role of Kp in metabolism regulation in the peripheral tissues (53, 54, 55). First, Kp and its receptor are expressed in peripheral tissues controlling metabolism (26, 54, 56). Second, at times of metabolic imbalance (e.g., undernutrition, obesity, and diabetes), alterations of the Kp system in these tissues were observed (57). Third, in vitro experiments indicate an action of Kp on lipid metabolism (55). Fourth, evidence for a function of Kp in metabolism control came from a study of Kiss1r KO animals. Kiss1r KO females had a greater body weight (BW), hyperinsulinemia, increased adiposity, elevated fasting basal glucose levels as well as impaired glucose tolerance (58). However, the observed obesity cases were not due to hyperphagia, but rather reduced metabolism. Kiss1r KO females had dramatically decreased energy expenditure. By contrast, Kiss1r KO males were characterized by normal BW and glucose tolerance. It would be of interest to study possible insulin resistance in this experimental model. Of particular relevance for this review, in the DM2 state insulin resistance is observed. Moreover, DM2 is often associated with obesity and accounts for roughly 90% of all diabetes cases (59). Adiposity, hyperinsulinemia and decreased metabolism were already seen at a younger age in Kiss1r KO females, with impaired glucose tolerance and feeding developing later in adulthood, after BW was significantly increased. Thus, an early life decrease in metabolism and energy expenditure may underline the later emergency of the obese phenotype of adult Kiss1r KO females (60). This phenotype could arise from defective signaling in the brain, and/or peripherally. Data on the expression of NPY, POMC, leptin receptor, ghrelin receptor, and melanocortin receptor 3 and 4 genes involved in the appetite regulating system of the hypothalamus generally showed no changes in the above-mentioned genes in Kiss1r KO mice with GDX. This suggests that obesity revealed in Kiss1r KO mice may reflect peripheral rather than central metabolic impairments (61). Below, we will update information on Kp in the peripheral tissues involved in control of metabolism.
Kp, Pancreas, and Glucose Metabolism
Glucose homeostasis is regulated by insulin secreted from beta cells in the pancreatic islets (62). Moreover, insulin release is modulated by numerous G protein-coupled receptors including Kiss1r (63). Expression of the Kiss1 gene in the pancreatic tissue was shown by Lee et al. (64), while later Hauge-Evans et al. (65) detected the expression of the Kp gene and its receptor in alpha and beta cells in human and murine islets, respectively. Both Kp and its receptor immunoreactivities colocalized with alpha and beta pancreas cells (65). Kisspeptin-54 (Kp-54) increased glucose-induced insulin secretion from human and murine islets, without any effect on basal secretion (65). Studies on rhesus monkeys (66) and rats (67) using kisspeptin-10 (Kp-10) confirmed in vitro observations. In addition, intracerebroventricular (icv.) administration of the peptide in rats had no effect on insulin levels, suggesting the peripheral site of action of Kp (67). Indeed, Kp-10 and kisspeptin-13 act directly at beta cells to potentiate insulin secretion stimulated by glucose in murine, porcine and human islets (63, 67, 68). Kp enhanced insulin secretion from murine islets in a dose-dependent manner through a Gβγ-dependent pathway (63, 67). However, there are contradictory results indicating that Kp could inhibit (69, 70) or have no effect on insulin secretion (67), which may be related to the used doses and differences in protocols. Data from our laboratory confirmed expression of Kiss1 and Kiss1r in the pancreas [mRNA and peptide (56)]. We have shown that diabetic type 1 (DM1) and DM2 male rats had increased pancreatic Kiss1 mRNA levels. However, in the case of Kiss1r, an increase was recorded only in the DM1 group. By contrast, protein levels decreased in diet-induced obese (DIO) and DM2 animals. In addition, Kp and its receptor levels were undetected in the DM1 group (as a result of damage to pancreatic cells caused by STZ). Levels of Kiss1r protein were reduced only in the DIO group (56). Thus, in DM2/DM1 animals, the Kiss1/Kiss1r system may not function properly, thus being unable to control insulin levels (Figures 1 and 2).
Kp in the Liver
The liver is an important organ, which acts as the body’s glucose reservoir (71). It is also responsible for maintaining steady circulating blood sugar levels by storing and producing glucose depending upon the body’s need (71). Only few studies focused on Kp in the liver. Kp-10 administered peripherally in male rats had antioxidant effects and increased the levels of free radical scavengers (superoxide dismutase and adenosine deaminase), suggesting protective effects on liver metabolism (72). Kp-10 in rats exposed to heat-induced oxidative stress decreased plasma corticosterone levels (73). Stressors enhance the activity of the hypothalamus–pituitary–adrenal axis, increasing corticosteroid levels. Since corticosterone may affect glucagon sensitivity and promote glycogenolysis, the authors hypothesized that glycogenolysis may be suppressed by the Kp-dependent reduction in corticosterone release (73). An extensive study conducted by Song et al. (54) revealed that in hyperglucagonemic diabetic animals (in HFD-induced diabetes and genetic leptin receptor-deficient db/db mice) liver Kp levels were increased. In vitro stimulation of mouse hepatocytes also increased Kiss1 mRNA and protein levels. Moreover, DM2 patients exhibit increased liver and plasma Kp levels. In addition, we showed an increase in liver Kp expression in both DIO and DM2 male rats (56). Kp-10 and Kp-54 also impaired glucose tolerance, while selective liver Kp knock-down depressed glucose-sensitive insulin secretion (GSIS) and improved glucose tolerance and mice lacking liver Kiss1r exhibited improved glucose tolerance when fed HFD (54).
Based on the above findings, a tri-hormonal regulatory circuit between pancreatic alpha cells, hepatocytes, and beta cells was proposed, with Kp playing an important role in the liver to islet endocrine signaling. It was suggested that activation of the liver glucagon receptor stimulates insulin secretion by increased hepatic glucose production and hyperglycemia. However, liver glucagon action may also inhibit insulin secretion by stimulating Kp production. According to this model, in pancreatic beta cells in DM2 patients, the GSIS can be stimulated by hyperglycemia but inhibited by Kp. These findings extend a potential for Kp antagonism as a therapeutic tool to improve beta cell function in diabetic patients (Figure 2) (54).
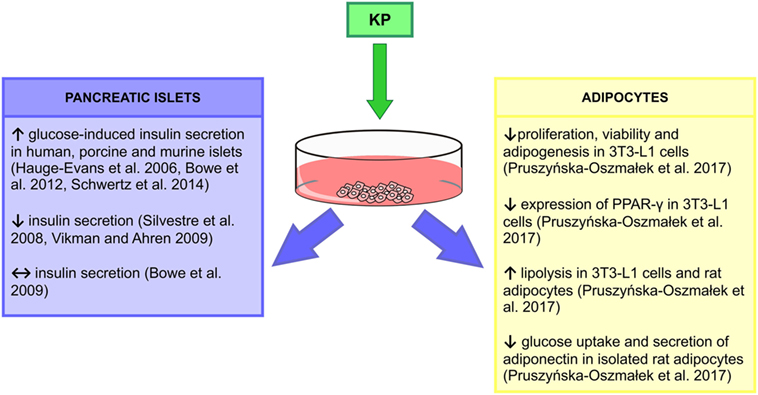
Figure 2. In vitro actions of kisspeptin (Kp). ↑, increase; ↓, decrease; ↔, no effect (55, 63, 65, 67–70).
Kp in the Adipose Tissue
The major function of adipocytes is to store fat for periods of greater energy requirements (74). Control of these lipogenic and lipolytic processes is modulated by hormonal signals, with the adipose tissue emerging as an active participant in regulating physiologic and pathologic processes (75).
Kiss1 mRNA was detected in rat (26, 56) and KISS1 mRNA in human (76) adipose tissue and was shown to regulate the metabolic status. While fasting of rats increased adipose Kiss1 mRNA expression in both sexes, a decrease was reported in Kiss1 mRNA expression in adipose tissue of HFD and obese Zucker rats. These alterations were seen in visceral (epididymal) and subcutaneous (in the case of Zucker rats) adipose tissue (26). In women, a positive correlation between body mass index (BMI) and KISS1 mRNA levels was found in the omental adipose tissue, but not in subcutaneous fat (76). Kiss1 mRNA expression in adipose tissue is sensitive to sex steroids; while GDX in both sexes had no effect on Kiss1 mRNA, supplementation of castrated animals with testosterone (males) and estradiol (females) increased Kiss1 mRNA in rats (26).
We have revealed expression of mRNA and peptide for Kp in gonadal fat of male rats (56). While in DIO and DM2 animals expression of Kiss1 and Kiss1r mRNA did not change, we were not able to detect peptides in these animals. By contrast, in DM1 rats there was a marked decrease in Kp and no change in Kiss1r levels. Our in vitro study showed that isolated rat adipocytes and mouse 3T3-L1 cells express mRNA and peptide for Kiss1 and Kiss1r genes, and we found multiple actions of Kp-10 in these cells (55). First, Kp inhibited proliferation, viability, and adipogenesis in 3T3-L1 cells and decreased expression of PPAR-γ and CEBPβ—genes, involved in differentiation processes and adipogenesis. Second, Kp-10 increased lipolysis in rat adipocytes and 3T3-L1 cells by enhancing the expression of perilipin and hormone-sensitive lipase. Third, Kp-10 modulated lipogenesis. Finally, Kp-10 decreased glucose uptake and adiponectin secretion, while it stimulated leptin secretion from rat adipocytes (55). Thus, Kp could be a major factor in the regulation of adipocyte metabolism, at least in vitro. In particular, Kp-10 may slow the process of lipid accumulation via decreasing lipogenesis and increasing lipolysis. Further experiments are required to reveal the effects of Kp on adipose tissue in vivo. The stimulatory effect of Kp-10 on adiponectin, but not leptin levels was already shown in male rhesus monkeys, both fed and fasting (Figures 1 and 2) (66).
Concluding Remarks and Future Directions
We reviewed data supporting the hypothesis that Kp is a link between metabolic cues and reproductive functions. On one hand, there have to be a proper amount of fat in the body to enter puberty, but on the other hand obesity can cause disturbances in puberty, followed by irregularities in testosterone production in men and ovulation in women (77, 78). Moreover, both puberty onset and obesity seems to be related to changes in Kp expression in the hypothalamus (7). However, peripheral Kp signaling may also play an important role in obesity or increasing body mass during adolescence. Within the hypothalamus, Kp does not act alone, but with other neuropeptides, e.g., NKB and DYN A, also being sensitive to the metabolic status. Moreover, relationships between the expression of Kp, NPY/AgRP, and POMC/CART were found. These neuropeptides have an impact on the hypothalamic GnRH functions, and complex interactions between these neurons in integration of metabolic and reproductive functions need to be revealed.
Although Kp is successfully used as a therapeutic target in human reproduction, including treatment of delayed and precocious puberty, subfertility, and contraception, much less is known on its role in the metabolic syndrome (79, 80). Kp-10 administered to men with DM2, and central hypogonadism enhanced endogenous testosterone secretion (81). Studies in animals indicate that Kp may be involved in the regulation of insulin secretion (54, 55, 63, 65, 69, 70). Recently, it was also revealed that Kp plasma concentration in the human is negatively correlated with BMI and waist circumference. Moreover, in non-diabetic individuals, Kp was shown to be associated with reduced glucose-stimulated insulin secretion (82). Further studies are needed to explore interactions between Kp and insulin in diabetic patients.
As most experiments are conducted on males and differences between sexes exist, there is a need to untangle alterations between females and males in the regulation of metabolic and reproductive functions. More research is required to understand interactions between Kp and metabolism in the peripheral tissues, where it can act in the paracrine manner. In addition, as tachykinins (neurokinins B and A, substance P) are extensively expressed within the CNS and at the periphery (83) and studies suggest a role of substance P in the regulation of metabolic functions (84, 85), it would be interesting to assess potential NKB/NK3R expression in peripheral tissues related to metabolism. More in vivo studies are needed on Kp action in the pancreas, liver, and fat tissue, while genetically modified animal models should be developed to elucidate mechanism(s) of its action. Basic scientists should participate in the WHO actions that have the potential to reduce the risk or burden of undernutrition and overweight by revealing the underlying mechanism(s). This may lead to the development of individually oriented therapeutic strategies against these lifestyle diseases.
Author Contributions
Both MD and KZ participated in drafting, writing, and editing the manuscript. JHS participated in writing and editing the manuscript.
Conflict of Interest Statement
The authors declare that the research was conducted in the absence of any commercial or financial relationships that could be construed as a potential conflict of interest.
Funding
This study was supported by the National Science Centre in Krakow, Poland (OPUS grant 2015/17/B/NZ4/02021 to JHS).
References
1. World Health Organization. The Double Bourden of Malnutition. Policy Brief. Geneva: World Health Organization (2017). p. 1–10. WHO/NMH/NHD/17.3.
3. Calow P. The cost of reproduction – a physiological approach. Biol Rev (1979) 54(1):23–40. doi:10.1111/j.1469-185X.1979.tb00866.x
4. de Roux N, Genin E, Carel J-C, Matsuda F, Chaussain J-L, Milgrom E. Hypogonadotropic hypogonadism due to loss of function of the KiSS1-derived peptide receptor GPR54. Proc Natl Acad Sci U S A (2003) 100(19):10972–6. doi:10.1073/pnas.1834399100
5. Seminara SB, Messager S, Chatzidaki EE, Thresher RR, Acierno JS Jr, Shagoury JK, et al. The GPR54 gene as a regulator of puberty. N Engl J Med (2003) 349(17):1614–27. doi:10.1056/NEJMoa035322
6. Varlamov O, Bethea CL, Roberts CT Jr. Sex-specific differences in lipid and glucose metabolism. Front Endocrinol (2014) 5:241. doi:10.3389/fendo.2014.00241
7. Manfredi-Lozano M, Roa J, Tena-Sempere M. Connecting metabolism and gonadal function: novel central neuropeptide pathways involved in the metabolic control of puberty and fertility. Front Neuroendocrinol (2017) 48:37–49. doi:10.1016/j.yfrne.2017.07.008
8. True C, Takahashi D, Kirigiti M, Lindsley SR, Moctezuma C, Arik A, et al. Arcuate nucleus neuropeptide coexpression and connections to gonadotropin-releasing hormone neurons in the female rhesus macaque. J Neuroendocrinol (2017) 29:1–15. doi:10.1111/jne.12491
9. De Bond JA, Smith JT. Kisspeptin and energy balance in reproduction. Reproduction (2014) 147(3):53–63. doi:10.1530/REP-13-0509
10. Castellano JM, Navarro VM, Fernandez-Fernandez R, Nogueiras R, Tovar S, Roa J, et al. Changes in hypothalamic KiSS-1 system and restoration of pubertal activation of the reproductive axis by kisspeptin in undernutrition. Endocrinology (2005) 146(9):3917–25. doi:10.1210/en.2005-0337
11. Dudek M, Kołodziejski P, Pruszyńska-Oszmałek E, Ziarniak K, Sliwowska J. Effects of orchidectomy and testosterone replacement on numbers of kisspeptin-, neurokinin B-, and dynorphin A-immunoreactive neurones in the arcuate nucleus of the hypothalamus in obese and diabetic rats. J Neuroendocrinol (2017) 29(2):1–11. doi:10.1111/jne.12453
12. Lie ME, Overgaard A, Mikkelsen JD. Effect of a postnatal high-fat diet exposure on puberty onset, estrous cycle regularity, and kisspeptin expression in female rats. Reprod Biol (2013) 13(4):298–308. doi:10.1016/j.repbio.2013.08.001
13. Burke MC, Letts PA, Krajewski SJ, Rance NE. Coexpression of dynorphin and neurokinin B immunoreactivity in the rat hypothalamus: morphologic evidence of interrelated function within the arcuate nucleus. J Comp Neurol (2006) 498(5):712–26. doi:10.1002/cne.21086
14. Goodman RL, Lehman MN, Smith JT, Coolen LM, de Oliveira CV, Jafarzadehshirazi MR, et al. Kisspeptin neurons in the arcuate nucleus of the ewe express both dynorphin A and neurokinin B. Endocrinology (2007) 148(12):5752–60. doi:10.1210/en.2007-0961
15. Hrabovszky E, Ciofi P, Vida B, Horvath MC, Keller E, Caraty A, et al. The kisspeptin system of the human hypothalamus: sexual dimorphism and relationship with gonadotropin-releasing hormone and neurokinin B neurons. Eur J Neurosci (2010) 31(11):1984–98. doi:10.1111/j.1460-9568.2010.07239.x
16. Lehman MN, Coolen LM, Goodman RL. Minireview: kisspeptin/neurokinin B/dynorphin (KNDy) cells of the arcuate nucleus: a central node in the control of gonadotropin-releasing hormone secretion. Endocrinology (2010) 151(8):3479–89. doi:10.1210/en.2010-0022
17. Overgaard A, Ruiz-Pino F, Castellano JM, Tena-Sempere M, Mikkelsen JD. Disparate changes in kisspeptin and neurokinin B expression in the arcuate nucleus after sex steroid manipulation reveal differential regulation of the two KNDy peptides in rats. Endocrinology (2014) 155(10):3945–55. doi:10.1210/en.2014-1200
18. Ramaswamy S, Seminara SB, Ali B, Ciofi P, Amin NA, Plant TM. Neurokinin B stimulates GnRH release in the male monkey (Macaca mulatta) and is colocalized with kisspeptin in the arcuate nucleus. Endocrinology (2010) 151(9):4494–503. doi:10.1210/en.2010-0223
19. Navarro VM, Ruiz-Pino F, Sanchez-Garrido MA, Garcia-Galiano D, Hobbs SJ, Manfredi-Lozano M, et al. Role of neurokinin B in the control of female puberty and its modulation by metabolic status. J Neurosci (2012) 32(7):2388–97. doi:10.1523/JNEUROSCI.4288-11.2012
20. Goodman RL, Coolen LM, Anderson GM, Hardy SL, Valent M, Connors JM, et al. Evidence that dynorphin plays a major role in mediating progesterone negative feedback on gonadotropin-releasing hormone neurons in sheep. Endocrinology (2004) 145(6):2959–67. doi:10.1210/en.2003-1305
21. Keen KL, Wegner FH, Bloom SR, Ghatei MA, Terasawa E. An increase in kisspeptin-54 release occurs with the pubertal increase in luteinizing hormone-releasing hormone-1 release in the stalk-median eminence of female rhesus monkeys in vivo. Endocrinology (2008) 149(8):4151–7. doi:10.1210/en.2008-0231
22. Messager S, Chatzidaki EE, Ma D, Hendrick AG, Zahn D, Dixon J, et al. Kisspeptin directly stimulates gonadotropin-releasing hormone release via G protein-coupled receptor 54. Proc Natl Acad Sci U S A (2005) 102(5):1761–6. doi:10.1073/pnas.0409330102
23. Navarro VM, Gottsch ML, Chavkin C, Okamura H, Clifton DK, Steiner RA. Regulation of gonadotropin-releasing hormone secretion by kisspeptin/dynorphin/neurokinin B neurons in the arcuate nucleus of the mouse. J Neurosci (2009) 29(38):11859–66. doi:10.1523/JNEUROSCI.1569-09.2009
24. Wakabayashi Y, Nakada T, Murata K, Ohkura S, Mogi K, Navarro VM, et al. Neurokinin B and dynorphin A in kisspeptin neurons of the arcuate nucleus participate in generation of periodic oscillation of neural activity driving pulsatile gonadotropin-releasing hormone secretion in the goat. J Neurosci (2010) 30(8):3124–32. doi:10.1523/JNEUROSCI.5848-09.2010
25. Quennell JH, Howell CS, Roa J, Augustine RA, Grattan DR, Anderson GM. Leptin deficiency and diet-induced obesity reduce hypothalamic kisspeptin expression in mice. Endocrinology (2011) 152(4):1541–50. doi:10.1210/en.2010-1100
26. Brown R, Imran S, Ur E, Wilkinson M. KiSS-1 mRNA in adipose tissue is regulated by sex hormones and food intake. Mol Cell Endocrinol (2008) 281(1):64–72. doi:10.1016/j.mce.2007.10.011
27. Luque RM, Kineman RD, Tena-Sempere M. Regulation of hypothalamic expression of KiSS-1 and GPR54 genes by metabolic factors: analyses using mouse models and a cell line. Endocrinology (2007) 148(10):4601–11. doi:10.1210/en.2007-0500
28. Castellano JM, Navarro VM, Fernandez-Fernandez R, Roa J, Vigo E, Pineda R, et al. Expression of hypothalamic KiSS-1 system and rescue of defective gonadotropic responses by kisspeptin in streptozotocin-induced diabetic male rats. Diabetes (2006) 55(9):2602–10. doi:10.2337/db05-1584
29. Ziarniak K, Pruszyńska-Oszmałek E, Matuszewska J, Sliwowska JH. Influence of obesity and type 2 diabetes on the number of kisspeptin-, neurokinin B- and dynorphin A-immunoreactive neurons in the arcuate nucleus of female rats. Acta Neurobiol Exp (2017) 77:I–CXLII.
30. Li XF, Lin YS, Kinsey-Jones JS, O’Byrne KT. High-fat diet increases LH pulse frequency and kisspeptin-neurokinin B expression in puberty-advanced female rats. Endocrinology (2012) 153(9):4422–31. doi:10.1210/en.2012-1223
31. Sheffer-Babila S, Sun Y, Israel DD, Liu SM, Neal-Perry G, Chua SC Jr. Agouti-related peptide plays a critical role in leptin’s effects on female puberty and reproduction. Am J Physiol (2013) 305(12):1512–20. doi:10.1152/ajpendo.00241.2013
32. Khawaja XZ, Chattopadhyay AK, Green IC. Increased β-endorphin and dynorphin concentrations in discrete hypothalamic regions of genetically obese (ob/ob) mice. Brain Res (1991) 555(1):164–8. doi:10.1016/0006-8993(91)90874-U
33. Kim EM, O’Hare E, Grace MK, Welch CC, Billington CJ, Levine AS. ARC POMC mRNA and PVN alpha-MSH are lower in obese relative to lean Zucker rats. Brain Res (2000) 862(1–2):11–6. doi:10.1016/S0006-8993(00)02060-6
34. Yang JA, Yasrebi A, Snyder M, Roepke TA. The interaction of fasting, caloric restriction, and diet-induced obesity with 17β-estradiol on the expression of KNDy neuropeptides and their receptors in the female mouse. Mol Cell Endocrinol (2016) 437:35–50. doi:10.1016/j.mce.2016.08.008
35. Berman Y, Devi L, Carr KD. Effects of streptozotocin-induced diabetes on prodynorphin-derived peptides in rat brain regions. Brain Res (1995) 685(1–2):129–34. doi:10.1016/0006-8993(95)00419-Q
36. Kim EM, Grace MK, Welch CC, Billington CJ, Levine AS. STZ-induced diabetes decreases and insulin normalizes POMC mRNA in arcuate nucleus and pituitary in rats. Am J Physiol (1999) 276(5,2):1320–6.
37. Berman Y, Devi L, Spangler R, Kreek MJ, Carr KD. Chronic food restriction and streptozotocin-induced diabetes differentially alter prodynorphin mRNA levels in rat brain regions. Brain Res Mol Brain Res (1997) 46(1):25–30. doi:10.1016/S0169-328X(96)00175-1
38. Hill JW, Elmquist JK, Elias CF. Hypothalamic pathways linking energy balance and reproduction. Am J Physiol Endocrinol Metab (2008) 294(5):827–32. doi:10.1152/ajpendo.00670.2007
39. Myers MG Jr, Olson DP. Central nervous system control of metabolism. Nature (2012) 491(7424):357–63. doi:10.1038/nature11705
40. Cowley MA, Smart JL, Rubinstein M, Cerdán MG, Diano S, Horvath TL, et al. Leptin activates anorexigenic POMC neurons through a neural network in the arcuate nucleus. Nature (2001) 411(6836):480–4. doi:10.1038/35078085
41. Heisler LK, Cowley MA, Kishi T, Tecott LH, Fan W, Low MJ, et al. Central serotonin and melanocortin pathways regulating energy homeostasis. Ann N Y Acad Sci (2003) 994:169–74. doi:10.1111/j.1749-6632.2003.tb03177.x
42. Lau J, Farzi A, Qi Y, Heilbronn R, Mietzsch M, Shi YC, et al. CART neurons in the arcuate nucleus and lateral hypothalamic area exert differential controls on energy homeostasis. Mol Metab (2017) 7:102–18. doi:10.1016/j.molmet.2017.10.015
43. Qiu J, Zhang C, Borgquist A, Nestor CC, Smith AW, Bosch MA, et al. Insulin excites anorexigenic proopiomelanocortin neurons via activation of canonical transient receptor potential channels. Cell Metab (2014) 19(4):682–93. doi:10.1016/j.cmet.2014.03.004
44. Sato I, Arima H, Ozaki N, Watanabe M, Goto M, Hayashi M, et al. Insulin inhibits neuropeptide Y gene expression in the arcuate nucleus through GABAergic systems. J Neurosci (2005) 25(38):8657–64. doi:10.1523/JNEUROSCI.2739-05.2005
45. Roa J, Herbison AE. Direct regulation of GnRH neuron excitability by arcuate nucleus POMC and NPY neuron neuropeptides in female mice. Endocrinology (2012) 153(11):5587–99. doi:10.1210/en.2012-1470
46. Leranth C, MacLusky N, Shanabrough M, Naftolin F. Immunohistochemical evidence for synaptic connections between pro-opiomelanocortin-immunoreactive axons and LH-RH neurons in the preoptic area of the rat. Brain Res (1988) 449(1):167–76. doi:10.1016/0006-8993(88)91035-9
47. Norgren R Jr, Lehman M. A double-label pre-embedding immunoperoxidase technique for electron microscopy using diaminobenzidine and tetramethylbenzidine as markers. J Histochem Cytochem (1989) 37(8):1283–9. doi:10.1177/37.8.2666511
48. Backholer K, Smith JT, Rao A, Pereira A, Iqbal J, Ogawa S, et al. Kisspeptin cells in the ewe brain respond to leptin and communicate with neuropeptide Y and proopiomelanocortin cells. Endocrinology (2010) 151(5):2233–43. doi:10.1210/en.2009-1190
49. True C, Verma S, Grove KL, Smith MS. Cocaine-and amphetamine-regulated transcript is a potent stimulator of GnRH and kisspeptin cells and may contribute to negative energy balance-induced reproductive inhibition in females. Endocrinology (2013) 154(8):2821–32. doi:10.1210/en.2013-1156
50. Fu L-Y, van den Pol AN. Kisspeptin directly excites anorexigenic proopiomelanocortin neurons but inhibits orexigenic neuropeptide Y cells by an indirect synaptic mechanism. J Neurosci (2010) 30(30):10205–19. doi:10.1523/JNEUROSCI.2098-10.2010
51. Sliwowska JH, Fergani C, Gawałek M, Skowronska B, Fichna P, Lehman MN. Insulin: its role in the central control of reproduction. Physiol Behav (2014) 133:197–206. doi:10.1016/j.physbeh.2014.05.021
52. Bhattacharya M, Babwah AV. Kisspeptin: beyond the brain. Endocrinology (2015) 156(4):1218–27. doi:10.1210/en.2014-1915
53. Hussain MA, Song W-J, Wolfe A. There is kisspeptin – and then there is kisspeptin. Trends Endocrinol Metab (2015) 26(10):564–72. doi:10.1016/j.tem.2015.07.008
54. Song WJ, Mondal P, Wolfe A, Alonso LC, Stamateris R, Ong BW, et al. Glucagon regulates hepatic kisspeptin to impair insulin secretion. Cell Metab (2014) 19(4):667–81. doi:10.1016/j.cmet.2014.03.005
55. Pruszyńska-Oszmałek E, Kołodziejski PA, Sassek M, Sliwowska JH. Kisspeptin-10 inhibits proliferation and regulates lipolysis and lipogenesis processes in 3T3-L1 cells and isolated rat adipocytes. Endocrine (2017) 56(1):54–64. doi:10.1007/s12020-017-1248-y
56. Dudek M, Kołodziejski P, Pruszyńska-Oszmałek E, Sassek M, Ziarniak K, Nowak K, et al. Effects of high-fat diet-induced obesity and diabetes on Kiss1 and GPR54 expression in the hypothalamic-pituitary-gonadal (HPG) axis and peripheral organs (fat, pancreas and liver) in male rats. Neuropeptides (2016) 56:41–9. doi:10.1016/j.npep.2016.01.005
57. Gawałek M, Sliwowska JH. Neuronal basis of reproductive dysfunctions associated with diet and alcohol: from the womb to adulthood. Reprod Biol (2015) 15(2):69–78. doi:10.1016/j.repbio.2015.04.001
58. Tolson KP, Garcia C, Yen S, Simonds S, Stefanidis A, Lawrence A, et al. Impaired kisspeptin signaling decreases metabolism and promotes glucose intolerance and obesity. J Clin Invest (2014) 124(7):3075. doi:10.1172/JCI71075
59. Chen L, Magliano DJ, Zimmet PZ. The worldwide epidemiology of type 2 diabetes mellitus-present and future perspectives. Nat Rev Endocrinol (2011) 8(4):228–36. doi:10.1038/nrendo.2011.183
60. Tolson KP, Garcia C, Delgado I, Marooki N, Kauffman AS. Metabolism and energy expenditure, but not feeding or glucose tolerance, are impaired in young Kiss1r KO female mice. Endocrinology (2016) 157(11):4192–9. doi:10.1210/en.2016-1501
61. De Bond JP, Tolson KP, Nasamran C, Kauffman AS, Smith JT. Unaltered hypothalamic metabolic gene expression in Kiss1r knockout mice despite obesity and reduced energy expenditure. J Neuroendocrinol (2016) 28(10):1–20. doi:10.1111/jne.12430
62. Plum L, Belgardt BF, Brüning JC. Central insulin action in energy and glucose homeostasis. J Clin Invest (2006) 116(7):1761–6. doi:10.1172/JCI29063
63. Schwetz TA, Reissaus CA, Piston DW. Differential stimulation of insulin secretion by GLP-1 and kisspeptin-10. PLoS One (2014) 9(11):113020. doi:10.1371/journal.pone.0113020
64. Lee JH, Miele ME, Hicks DJ, Phillips KK, Trent JM, Weissman BE, et al. KiSS-1, a novel human malignant melanoma metastasis-suppressor gene. J Natl Cancer Inst (1996) 88(23):1731–7. doi:10.1093/jnci/88.23.1731
65. Hauge-Evans A, Richardson C, Milne H, Christie M, Persaud S, Jones P. A role for kisspeptin in islet function. Diabetologia (2006) 49(9):2131–5. doi:10.1007/s00125-006-0343-z
66. Wahab F, Bano R, Jabeen S, Irfan S, Shahab M. Effect of peripheral kisspeptin administration on adiponectin, leptin, and resistin secretion under fed and fasting conditions in the adult male rhesus monkey (Macaca mulatta). Horm Metab Res (2010) 42(08):570–4. doi:10.1055/s-0030-1252016
67. Bowe JE, King AJ, Kinsey-Jones JS, Foot VL, Li XF, O’Byrne KT, et al. Kisspeptin stimulation of insulin secretion: mechanisms of action in mouse islets and rats. Diabetologia (2009) 52(5):855–62. doi:10.1007/s00125-009-1283-1
68. Bowe JE, Foot VL, Amiel SA, Huang GC, Lamb M, Lakey J, et al. GPR54 peptide agonists stimulate insulin secretion from murine, porcine and human islets. Islets (2012) 4(1):20–3. doi:10.4161/isl.18261
69. Silvestre R, Egido E, Hernández R, Marco J. Kisspeptin-13 inhibits insulin secretion without affecting glucagon or somatostatin release: study in the perfused rat pancreas. J Endocrinol (2008) 196(2):283–90. doi:10.1677/JOE-07-0454
70. Vikman J, Ahren B. Inhibitory effect of kisspeptins on insulin secretion from isolated mouse islets. Diabetes Obes Metab (2009) 11(4):197–201. doi:10.1111/j.1463-1326.2009.01116.x
71. Nordlie RC, Foster JD, Lange AJ. Regulation of glucose production by the liver. Annu Rev Nutr (1999) 19(1):379–406. doi:10.1146/annurev.nutr.19.1.379
72. Aydin M, Oktar S, Yonden Z, Ozturk OH, Yilmaz B. Direct and indirect effects of kisspeptin on liver oxidant and antioxidant systems in young male rats. Cell Biochem Funct (2010) 28(4):293–9. doi:10.1002/cbf.1656
73. Hou Y, Wang X, Ping J, Lei Z, Gao Y, Ma Z, et al. Metabonomics approach to assessing the modulatory effects of kisspeptin-10 on liver injury induced by heat stress in rats. Sci Rep (2017) 7(1):7020. doi:10.1038/s41598-017-06017-1
74. Ahima RS, Flier JS. Adipose tissue as an endocrine organ. Trends Endocrinol Metab (2000) 11(8):327–32. doi:10.1016/S1043-2760(00)00301-5
75. Ntambi JM, Young-Cheul K. Adipocyte differentiation and gene expression. J Nutr (2000) 130(12):3122–6. doi:10.1093/jn/130.12.3122S
76. Cockwell H, Wilkinson DA, Bouzayen R, Imran SA, Brown R, Wilkinson M. KISS1 expression in human female adipose tissue. Arch Gynecol Obstet (2013) 287(1):143–7. doi:10.1007/s00404-012-2514-0
77. Glass AR, Swerdloff RS, Bray GA, Dahms WT, Atkinson RL. Low serum testosterone and sex-hormone-binding-globulin in massively obese men. J Clin Endocrinol Metab (1977) 45(6):1211–9. doi:10.1210/jcem-45-6-1211
78. Lake JK, Power C, Cole TJ. Women’s reproductive health: the role of body mass index in early and adult life. Int J Obes Relat Metab Disord (1997) 21(6):432–8. doi:10.1038/sj.ijo.0800424
79. Tng EL. Kisspeptin signalling and its roles in humans. Singapore Med J (2015) 56(12):649–56. doi:10.11622/smedj.2015183
80. Yang L, Dhillo W. Kisspeptin as a therapeutic target in reproduction. Expert Opin Ther Targets (2016) 20(5):567–75. doi:10.1517/14728222.2016.1124858
81. George JT, Veldhuis JD, Tena-Sempere M, Millar RP, Anderson RA. Exploring the pathophysiology of hypogonadism in men with type 2 diabetes: kisspeptin-10 stimulates serum testosterone and LH secretion in men with type 2 diabetes and mild biochemical hypogonadism. Clin Endocrinol (2013) 79(1):100–4. doi:10.1111/cen.12103
82. Andreozzi F, Mannino GC, Mancuso E, Spiga R, Perticone F, Sesti G. Plasma kisspeptin levels are associated with insulin secretion in nondiabetic individuals. PLoS One (2017) 12(6):0179834. doi:10.1371/journal.pone.0179834
83. Pinto FM, Almeida TA, Hernandez M, Devillier P, Advenier C, Candenas ML. mRNA expression of tachykinins and tachykinin receptors in different human tissues. Eur J Pharmacol (2004) 494(2):233–9. doi:10.1016/j.ejphar.2004.05.016
84. Karagiannides I, Stavrakis D, Bakirtzi K, Kokkotou E, Pirtskhalava T, Nayeb-Hashemi H, et al. Substance P (SP)-neurokinin-1 receptor (NK-1R) alters adipose tissue responses to high-fat diet and insulin action. Endocrinology (2011) 152(6):2197–205. doi:10.1210/en.2010-1345
Keywords: kisspeptin, obesity, diabetes, undernutrition, metabolism, reproduction
Citation: Dudek M, Ziarniak K and Sliwowska JH (2018) Kisspeptin and Metabolism: The Brain and Beyond. Front. Endocrinol. 9:145. doi: 10.3389/fendo.2018.00145
Received: 15 December 2017; Accepted: 19 March 2018;
Published: 16 April 2018
Edited by:
Rosaria Meccariello, Università degli Studi di Napoli Parthenope, ItalyReviewed by:
Satoshi Ogawa, Monash University Malaysia, MalaysiaFazal Wahab, Deutsches Primatenzentrum, Germany
Copyright: © 2018 Dudek, Ziarniak and Sliwowska. This is an open-access article distributed under the terms of the Creative Commons Attribution License (CC BY). The use, distribution or reproduction in other forums is permitted, provided the original author(s) and the copyright owner are credited and that the original publication in this journal is cited, in accordance with accepted academic practice. No use, distribution or reproduction is permitted which does not comply with these terms.
*Correspondence: Joanna H. Sliwowska, joanna.sliwowska@gmail.com