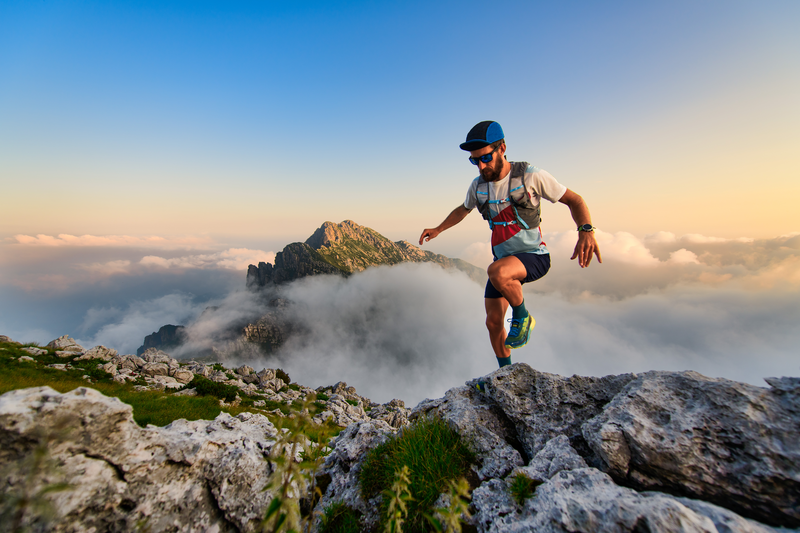
95% of researchers rate our articles as excellent or good
Learn more about the work of our research integrity team to safeguard the quality of each article we publish.
Find out more
MINI REVIEW article
Front. Endocrinol. , 06 March 2018
Sec. Cancer Endocrinology
Volume 9 - 2018 | https://doi.org/10.3389/fendo.2018.00069
This article is part of the Research Topic Personalized Medicine in Cancer Research View all 7 articles
Proteoglycans (PGs), important constituents of the extracellular matrix, have been associated with cancer pathogenesis. Their unique structure consisting of a protein core and glycosaminoglycan chains endowed with fine modifications constitutes these molecules as capable cellular effectors important for homeostasis and contributing to disease progression. Indeed, differential expression of PGs and their interacting proteins has been characterized as specific for disease evolvement in various cancer types. Importantly, PGs to a large extent regulate the bioavailability of hormones, growth factors, and cytokines as well as the activation of their respective receptors which regulate phenotypic diversibility, gene expression and rates of recurrence in specific tumor types. Defining and targeting these effectors on an individual patient basis offers ground for the development of newer therapeutic approaches which may act as either supportive or a substitute treatment to the standard therapy protocols. This review discusses the roles of PGs in cancer progression, developing technologies utilized for the defining of the PG “signature” in disease, and how this may facilitate the generation of tailor-made cancer strategies.
Proteoglycans (PGs) are composite molecules in which a glycosaminoglycan (GAG) chain(s) is covalently bound into a protein core. PGs, produced by, practically all, mammalian cells can be embedded into the plasma membranes, associated to the plasma membrane (pericellular), secreted into the extracellular matrix (ECM proper), or stored in secretory granules (intracellular). The enlistment of PGs to above classes is based on the following criteria: cellular and subcellular location, overall gene/protein homology, and the use of specific protein modules within their respective protein cores. It must, however, be mentioned, that there is a great deal of overlapping among the classes (1–3). We can certainly start with the statement that PGs have an important role in the formation of the ECM super-assembly as well as in determining of its physicochemical properties (1). Additionally, it can be said that PGs are receptors of a varying sensitivity and serve as a reservoir of biologically active mediators including growth factors (4, 5). Noteworthy, a single cell type can produce many different PG types. Osteoblast-derived cells for example secrete various ECM PGs and express on their membranes a number of cell-surface PGs (2, 6). Approximately 45 PGs have been identified to date, but each of the PGs members exhibits enormous variability (3). This inherent variability is determined by protein core modifications and by the number, type, and fine structural modifications of its GAG chains as well as by the different stoichiometry of GAG chain substitution (1, 3, 7). Briefly, PGs may have a number of GAG chain attachment sites which are not utilized equally or may exist as part-time PGs being expressed in both substituted and non-substituted forms. Furthermore, the fine structural modifications of PGs are tissue type and cell type specific. Therefore, each PG, as defined by its core protein, actually stands for a varying population of molecules where each “variant” represents a discrete structural entity (1, 3).
All PGs in respect to their cell location, as mentioned above, can be classified into intracellular, pericellular, ECM proper, and intracellular PGs. The unique intracellular PG serglycin (8) which is mostly involved in inflammatory reactions presents a category on its own. Pericellular PGs, heparan sulfate (HS)-carrying perlecan and agrin as well as Collagens XVIII and XV are mostly involved in the assembly of basement membranes. The largely HS-decorated, cell-surface PGs consist of the seven transmembrane members, e.g., syndecans 1–4 and the six GPI-anchored glypicans (1, 9, 10). Syndecans have important roles in integrin, growth factor, and growth factor receptor signaling (10, 11) whereas glypicans (12) are capable of regulating key cellular signaling pathways including Hedgehog signaling (9) and Wnt signaling (13).
The ECM proper PGs consist of 25 members: the four hyaluronan-binding hyalectans, key structural components of cartilage, blood vessels, and central nervous systems; the 18 small leucine-rich proteoglycans (SLRPs) and the three calcium-binding HSPGs, testicans (1). As regarding hyalectins, early studies demonstrated that these chondroitin sulfate (CS) PGs contain lectin-like and growth factor-like sequences (14, 15), SLRPs are modulators of ligand–receptor binding (2), autophagy regulators, and damage associated molecular patterns (16).
Proteoglycans contribute to cancer pathogenesis. Malignant tumors have individual PG profiles, which are closely associated with their differentiation and biological behavior, mesenchymal tumors showing a different profile from that of epithelial tumors (17, 18). Indeed, it was recently discussed that the role of the HSPG, syndecan-2 in cancer pathogenesis is conditional on cancer tissue origin determining its use as a biomarker/therapeutic target feasible (18). Noteworthy, GAG/PG effects are decidedly contingent on the specific correlation among their localization, expression, and disease type/stage. The unique, as regarding its localization, intracellular PG, serglycin has first been identified in hemopoietic cells (19). A number of later studies indicated its role in the process of malignant transformation of hemopoitic cells culminating with the suggestion that the malignant transformation of lymphoid cells may be characterized by, among other, increased synthesis of serglycin (20). In continuation, higher expression of serglycin was shown in aggressive lung, colon, and breast as well as prostate cancer cell lines (21). Furthermore, serglycin expression has been correlated to highly metastatic nasopharyngeal (22) and hepato-cellular carcinoma (HCC) metastasis to bone (23). Interestingly, it was demonstrated that serglycin has an obligatory participation in multiple myeloma cell in vivo growth and adhesion as well as vascularization (24).
The basement membrane PG, perlecan was initially implicated in cancer pathogenesis in a liver cancer mouse model (25). Further studies, among other by the Kovalszky group, demonstrated that this PG is not expressed by liver parenchyma tissue but rather to tumor blood vessel walls (26). Likewise, early studies demonstrated an increased expression of perlecan in the pericellular matrix of metastatic melanoma tumor samples (27). In continuation it was demonstrated that suppression of perlecan downregulates invasive behavior of melanoma cells (28) and blocks tumor growth and angiogenesis in vivo (29).
The hyalectan versican was demonstrated to be overexpressed in a range of cancers, including prostate, malignant myeloma, breast, glioblastoma, laryngeal, ovarian, pancreatic, cervical, gastric, and testicular germ-cell cancer as reviewed by Binder et al. (30). Five isoforms of versican, with various GAG-binding abilities and signaling properties, are generated through alternative splicing (31). The role of other hyalectans in cancer is not well established, even though evidence shows some contribution. Thus, the hyalectan aggrecan is postulated as tumor suppressor as decreased aggrecan expression correlates with metastasis and poor prognosis in laryngeal cancer (32). As regarding SLRPs, there is an exquisite specificity of discrete members’ role in carcinogenesis (2). Thus, decorin is a strong tumor suppressor (33) whereas, the expression of lumican needs to be specifically correlated to the tumor type and stage during the disease progression to draw more relevant conclusions (7). On the other hand, biglycan, mostly due to its inflammation-regulatory roles has mostly a tumor-promoting function (34). Immediate correlation between cell membrane PGs and cancer pathogenesis has been established. Thus, glypican-3 (GPC3) has emerged as a candidate therapeutic target in HCC, involving cell-cycle arrest at G1 phase through Yes-associated protein signaling (35). Importantly, GPC3 is not expressed by normal hepatic tissue but is expressed during the process of malignant transformation (36). On the other hand, downregulation of GPC3 expression in a mouse model facilitated ovarian cancer cell tumorigenicity (37).
Importantly, PGs can regulate the bioavailability of hormones, growth factors, and cytokines as well as the activation of their respective receptors (38), which affects phenotypic diversibility, gene expression, and rates of recurrence in specific tumor types (39, 40). To the cell membrane syndecans, was annotated the archetypal role in regulating basic fibroblast growth factor-dependent (FGF-2) signaling (41). Subsequently, the ability of syndecans to bind and to present growth factors to their respective growth factor receptors was associated with specific cell functions. Thus, it was shown that the inhibition of myogenic differentiation by FGF-1, FGF-2 as well as Kaposi’s sarcoma FGFs was directly correlated to cellular HS (42). Indeed, to this PG family was annotated the ability to regulate the signaling properties of GFs endowed with the capability to bind heparin. Indeed, to syndecan-1 was attributed the ability to act as a functional co-receptor for hepatocyte growth factor (HGF) that induces the initiation of the receptor tyrosine kinase for HGF (Met). This tyrosine kinase enhances cell viability and growth of multiple myeloma cells through phosphatidylinositol 3-kinase/protein kinase B and RAS/mitogen-activated protein kinase pathways (43). Recent studies, present the exquisite specificity of syndecans signaling in cancer (10, 18). Thus, syndecan-2 was found to regulate a key transforming growth factor beta-2/Smad2-signaling axis in fibrosarcoma adhesion (44). Furthermore, this syndecan was shown to propagate IGF-I/IGF-IR signaling through ezrin/erk downstream activation (45). Anti-GPC3 therapy was found to dowregulate insulin-like growth factor-II expression and downstream signaling involving downregulation of HSPGs, deactivation of sulfase-2 as well as a decrease of caspase-3 gene expression. This was strongly correlated to attenuation of HCC progression (46).
The role of other PG classes in cytokine signaling mediation has been highlighted in numerous studies. Recently, the opposite role of decorin and versican in the regulation of the cross talk between estradiol receptor and EGFR/IGF-IR signaling pathways in estrogen-responsive breast cancers have been discussed (47). Specifically, higher decorin expression in the tumor stroma in node-negative invasive breast cancer is correlated to better prognosis (48) due to the modulation of the EGF family of tyrosine kinase receptors downstream signaling (49). This complex mechanism of decorin action starts with its binding to the EGFR which results in receptor dimerization and protracted phosphorylation of the downstream MAP kinase (50, 51). The cyclin-dependent kinase inhibitor p21WAF1/Cip1 (p21) is thereupon induced which downregulates tumor growth (52). The binding of decorin to EGFR, however, ultimately results in receptor degradation via sustained internalization, executed through caveolar-dependent endocytosis (50). On the other hand, Du et al., showed that the versican G3 domain facilitated breast cancer cell proliferation, mobility, and capability to metastasize to distant sites by enhancing signaling pathways dependent on EGFR activation (53). Specifically, the same authors show that the CDK2 and GSK-3β (S9P) actions dependent on the EGFR/ERK signaling decreased the apoptosis of these cells. Moreover, versican G3 expressing breast cancer cells had a poorer response to chemotherapeutic drugs treatments, including doxorubicin or epirubicin (54). The role of decorin in IGF-IR activation and endocytisis has likewise been analyzed in several cancer models (55). In addition, it was demonstrated that downregulated decorin expression facilities the process of hepatic carcinogenesis in vivo by permitting PDGFRα, EGFR, IGF-IR, and MSPR/RON tumorigenic signaling (56). The basal membrane perlecan and its binding ligands, including VEGF, SHH, KGF, Flt-1, and Flk-1, were found to be differentially expressed in oral epithelial dysplasia and carcinoma in situ indicating to the important contribution of perlecan in the regulation of these factors signaling (57). The effect of PGs on cytokine signaling in cancer and the resulting action on cancer cell function is schematically depicted in Figure 1.
Figure 1. Schematic presentation of proteoglycan (PG) localization and regulation of cancer relevant cytokine signaling. PGs are located to the extracellular matrix (ECM) proper, pericellular matrix, cell membrane, or intracellular granules. Decorin (Dec), bound in to collagen fibers to the ECM specifically binds growth factors including TGF to create ECM “pools”; whereas pericellular decorin binds to EGFR and/or IGFR to attenuate their downstream signaling and induce growth arrest. Versican binds to through its EFG motif to EGFR and facilitates cancer cell growth, migration, and invasion in a CDK2/GSK-3β-dependent manner whereas through hyaluronan–hyaluronan receptor interaction it regulates cancer progression in a positive or negative manner depending on the context. Pericellular matrix, perlecan regulates VEGF, SHH, KGF, Flt-1, and Flk-1 bioavailability to affect cancer progression. Cell membrane, syndecan-2 was found to regulate, in fibrosarcoma, a transforming growth factor beta-2 (TGF-β2)/Smad2-signaling axis and to propagate IGF-I/IGF-IR signaling through ezrin/erk downstream activation.
Due to their key roles in tumorigenesis a number of methods has evolved to determine PG “molecular signature” in cancer. Thus, a combination of comparative genomic expression profiling and immunohistochemical staining of tissue microarrays from patients followed by multivariate analyses exhibited serglycin as an unfavorable independent indicator of distant metastasis-free and disease-free survival (22). This allowed the characterization of serglycin as an independent prognostic indicator of metastasis-free survival and disease-free survival in patients. An innovative approach was the utilization of Matrix-assisted laser desorption ionization-time of flight mass spectrometry to characterize the serum peptide profile of HCC patients with bone metastasis. Indeed, a diagnostic model was determined using a learning algorithm of radial basis function neural network which identified a serglycin-derived peptide as one of seven peptides which may be utilized as a diagnosis tool for HCC metastasis to bone (23). Furthermore, the usage of quantitative tissue proteomics analysis indicated versican as a promising biomarker for the detection of HCC at an early stage (58).
Genome-wide methylation analysis of bladder cancer tissues discovered hypermethylation in the promoter region of a number of genes; with the combined hypermethylation of SOX1, PITX2, or versican identifying patients with a higher risk of bladder cancer morbidity (59). The utilization of microarray expression profiling on a group of 84 matched clear cell renal carcinoma and normal renal tissues determined a higher expression of the hyalectan, versican in tumor tissues. This was positively correlated to metastasis and worse prognosis (60).
Furthermore, upon applying an unsupervised method of data analysis, using singular value decomposition to microarray data sets of ovarian cancer tissues, a total of 151 targets was identified. Evaluation of the selected target genes by Real-time PCR showed that dermatan sulfate PG3 and LOX were strongly associated with overall as well as with disease-free survival (61).
Upon utilizing multiple microarray cohorts for genome screening, especially focused on genes correlated to disease relapse in stage II-III colon cancer patients, stromal versican expression was identified as a biomarker (62). When the Cancer Genome Atlas data set of 540 glioblastoma patients was analyzed with a R2 analysis webtool (R2 Genomics and Visualization Platform, Oncogenomics, AMC) and correlated to matching transcriptome and survival data their serglycin expression was shown to be grade dependent as well as positively associated with tumor tissue infiltration by mast cells (63).
Recently, Brézillon et al., have demonstrated that Raman micro-spectroscopy allows recording of the discrete GAG profiles of individual live cells making feasible its use for cell screening purposes. This method can potentially be utilized for identifying specific molecular signatures of GAGs as a marker of cancer progression in tissues (64). This is important as during tumor progression there is a remodeling of PG glycosylation of both cell surfaces and the ECM, among other, through heparanase action (65). Heparanase, is an enzyme that cleaves PG HS-side chains (66), thus strongly modulating various regulatory pathways, including the bio accessibility of HS-binding growth factors and cytokines (10). A clinical application of “glycan signature” is illustrated by a study showing that the type of PG glycosylation can affect the ability of immune cells to infiltrate tumor tissues and engage in the immune response (67).
However, previously well-established methods can likewise detect changes in PG molecular signature in some tumor types. Indeed, in urothelial bladder carcinoma the utilization of enzyme-linked immunosorbent assays (ELISA) showed that serum levels of SLRPs lumican, biglycan, and decorin were significantly altered in patients as compared with healthy controls (68). Additionally, the increased expression of lumican in urothelial cancer patients has been suggested as potential non-invasive marker for early detection of bladder cancer (68). Moreover, measurement of shedded syndecan-1 serum levels by ELISA in prostate patients revealed a correlation of higher levels with advanced cancer stage as well as with adverse overall survival and DSS in a multivariable pre-operative model. These findings suggest that the evaluation of sSDC1-levels is a promising tool for pre-operative risk-stratification in this group of patients (69).
Potential utilization of PGs as markers for therapy evaluation has also been proposed. Thus syndecan-4 mRNA expression has been indicated as a novel marker for the prediction of glioblastoma multiforme patient’s response to treatment with the WT1 peptide vaccine (70). Furthermore, an increase in plasma glypican-1 positive exosomes and a reduction in plasma miR-96-5p and miR-149 expression were correlated to colorectal cancer diagnosis; whereas a normalization of these markers’ levels was achieved after successful colorectal cancer surgery. The exosomes were isolated by ExoCapTM Exosome Isolation and Enrichment kit and analyzed by transmission electron microscopy and flow cytometry. Through this approach, glypican-1 positive exosomes as well as plasma miR-96-5p and miR-149 are suggested as markers for colorectal cancer diagnosis and therapy evaluation (71) Furthermore, in the case of colorectal cancer patients with peritoneal metastases, it was found that high epithelial versican expression in combination with high vascular endothelial growth factor levels were markers of better response to cytoreductive surgery and hyperthermic intraperitoneal chemotherapy, resulting in higher overall survival (72). PG expression and mechanisms of action in cancer are summarized in Table 1.
The idea of using PGs as therapeutical targets in cancer dates more than 30 years as Real et al in 1985 identified CSPG4 as a specific surface antigen of melanoma cells (73). This early report was followed by numerous studies performed by cancer research-oriented groups which resulted in multilevel approaches targeting PGs during cancer progression. Indeed, as recently discussed by Ilieva et al., CSPG4 “has been associated with the pathology of multiple types of cancer such as melanoma, breast cancer, squamous cell carcinoma, mesothelioma, neuroblastoma, adult and pediatric sarcomas, and some hematological cancers” and has become the goal of different, under development, therapeutical strategies (74).
Recently, cancer-selective tetra-branched peptides, with the ability to bind with high specificity to the GAG components of HSPGs have been developed. These peptides’ chemical structure allows coupling with various functional units utilized in cancer therapy. Noteworthy, peptides bearing methotrexate were found to by-pass the obtained, by breast cancer cells, resistance to the drug (75). The HSPG glypican-2 was recently shown to be highly expressed in neuroblastoma but not detectable in normal childhood tissues. Moreover, Bosse et al. produced an antibody-drug conjugate directed against glypican-2 with a high cytotoxic potential for neuroblastoma cells (76). Another, promising approach employs the putative immunomodulatory effects of PGs. Thus, proteolysis of versican deposited to colorectal cancer microenvironment resulted in the generation of novel matrikines and facilitated the infiltration of T cells to tumor tissues (77). A recent, preclinical proof of concept study shows that targeting PGs abundant in chondrosarcoma ECM results in a significant enhancement of the therapeutic index (78).
Even though, a large pool of data on PGs roles in cancer has been obtained limited progress has been made in translating basic research to clinical practice. The up to date performed clinical trials have focused on PG-directed vaccines. Patients with advanced HCC and high expression of GPC3 exhibited increased survival as compared to patients with low tumor GPC3 expression upon treatment with a novel recombinant humanized monoclonal antibody against GPC3 (79). Likewise, in two separate studies temporary disease stabilization was achieved after treating HCC patients with a peptide vaccine against GPC3 (80, 81). Moreover, blockade of the programmed death-1/PD-L1 pathway in combination with a GPC3 vaccine increased the immune response of vaccine-induced T lymphocytes in HCC patients (82). Furthermore, treatment of two recurrent ovarian clear cell carcinoma patients with a GPC3-derived peptide vaccine resulted in the stabilization of disease for over 1 year (83) (Table 2).
The evidence that PGs have a key role in pathogenesis of cancer has led to the conclusion that understanding the changes in PG expression, fine structure as well as localization may lead to the development of innovative biomarkers and selective, more efficient therapies.
DN participated in drafting, writing, and editing the manuscript. AB and IS participated in writing and editing the manuscript. TK participated in writing and illustration preparation. AT and GT participated in drafting and editing the manuscript.
The authors declare that the research was conducted in the absence of any commercial or financial relationships that could be construed as a potential conflict of interest.
Some of research executed by our laboratories has been co-financed by the European Union (European Social Fund—ESF) and Greek National Funds (MIS 380222) awarded to GT. The authors express their gratitude to University Spin-Off, TOXPLUS S.A. for financially supporting this study.
1. Iozzo RV, Schaefer L. Proteoglycan form and function: a comprehensive nomenclature of proteoglycans. Matrix Biol (2015) 42:11–55. doi:10.1016/j.matbio.2015.02.003
2. Nikitovic D, Aggelidakis J, Young MF, Iozzo RV, Karamanos NK, Tzanakakis GN. The biology of small leucine-rich proteoglycans in bone pathophysiology. J Biol Chem (2012) 287(41):33926–33. doi:10.1074/jbc.R112.379602
3. Lindahl U, Couchman J, Kimata K, Esko JD. Proteoglycans and sulfated glycosaminoglycans. In: Varki A, Cummings RD, Esko JD, Stanley P, Hart GW, Aebi M, et al. editors. Essentials of Glycobiology [Internet]. 3rd ed. (Chap. 17), Cold Spring Harbor, NY: Cold Spring Harbor Laboratory Press (2015-2017). Available from: https://www.ncbi.nlm.nih.gov/books/NBK453033/
4. Filla MS, Dam P, Rapraeger AC. The cell surface proteoglycan syndecan-1 mediates fibroblast growth factor-2 binding and activity. J Cell Physiol (1998) 174(3):310–21. doi:10.1002/(SICI)1097-4652(199803)174:3<310::AID-JCP5>3.0.CO;2-R
5. Nikitovic D, Chalkiadaki G, Berdiaki A, Aggelidakis J, Katonis P, Karamanos NK, et al. Lumican regulates osteosarcoma cell adhesion by modulating TGFβ2 activity. Int J Biochem Cell Biol (2011) 43(6):928–35. doi:10.1016/j.biocel.2011.03.008
6. Kumarasuriyar A, Murali S, Nurcombe V, Cool SM. Glycosaminoglycan composition changes with MG-63 osteosarcoma osteogenesis in vitro and induces human mesenchymal stem cell aggregation. J Cell Physiol (2009) 218(3):501–11. doi:10.1002/jcp.21620
7. Nikitovic D, Papoutsidakis A, Karamanos NK, Tzanakakis GN. Lumican affects tumor cell functions, tumor-ECM interactions, angiogenesis and inflammatory response. Matrix Biol (2014) 35:206–14. doi:10.1016/j.matbio.2013.09.003
8. Kolset SO, Tveit H. Serglycin – structure and biology. Cell Mol Life Sci (2008) 65(7–8):1073–85. doi:10.1007/s00018-007-7455-6
9. Filmus J, Capurro M. The role of glypicans in Hedgehog signaling. Matrix Biol (2014) 35:248–52. doi:10.1016/j.matbio.2013.12.007
10. Afratis NA, Nikitovic D, Multhaupt HA, Theocharis AD, Couchman JR, Karamanos NK. Syndecans – key regulators of cell signaling and biological functions. FEBS J (2017) 284(1):27–41. doi:10.1111/febs.13940
11. Tumova S, Woods A, Couchman JR. Heparan sulfate proteoglycans on the cell surface: versatile coordinators of cellular functions. Int J Biochem Cell Biol (2000) 32:269–88. doi:10.1016/S1357-2725(99)00116-8
12. Fujihara Y, Ikawa M. GPI-AP release in cellular, developmental, and reproductive biology. J Lipid Res (2016) 57(4):538–45. doi:10.1194/jlr.R063032
13. Pez F, Lopez A, Kim M, Wands JR, Caron de Fromentel C, Merle P. Wnt signaling and hepatocarcinogenesis: molecular targets for the development of innovative anticancer drugs. J Hepatol (2013) 59(5):1107–17. doi:10.1016/j.jhep.2013.07.001
14. Krusius T, Gehlsen KR, Ruoslahti E. A fibroblast chondroitin sulfate proteoglycan core protein contains lectin-like and growth factor-like sequences. J Biol Chem (1987) 262(27):13120–5.
15. Knudson CB, Toole BP. Fluorescent morphological probe for hyaluronate. J Cell Bio (1985) 100(5):1753–8. doi:10.1083/jcb.100.5.1753
16. Gubbiotti MA, Neill T, Frey H, Schaefer L, Iozzo RV. Decorin is an autophagy-inducible proteoglycan and is required for proper in vivo autophagy. Matrix Bio (2015) 48:14–25. doi:10.1016/j.matbio.2015.09.001
17. Szatmári T, Dobra K. The role of syndecan-1 in cellular signaling and its effects on heparan sulfate biosynthesis in mesenchymal tumors. Front Oncol (2013) 3:310. doi:10.3389/fonc.2013.00310
18. Mytilinaiou M, Nikitovic D, Berdiaki A, Kostouras A, Papoutsidakis A, Tsatsakis AM, et al. Emerging roles of syndecan 2 in epithelial and mesenchymal cancer progression. IUBMB Life (2017) 69(11):824–33. doi:10.1002/iub.1678
19. Kolset SO, Gallagher JT. Proteoglycans in haemopoietic cells. Biochim Biophys Acta (1990) 1032(2–3):191–211.
20. Fadnes B, Husebekk A, Svineng G, Rekdal Ø, Yanagishita M, Kolset SO, et al. The proteoglycan repertoire of lymphoid cells. Glycoconj J (2012) 29(7):513–23. doi:10.1007/s10719-012-9427-9
21. Korpetinou A, Papachristou D, Lampropoulou A, Bouris P, Labropoulou VT, Noulas A, et al. Increased expression of serglycin in specific carcinomas and aggressive cancer cell lines. Biomed Res Int (2015) 2015:690721. doi:10.1155/2015/690721
22. Li XJ, Ong CK, Cao Y, Xiang YQ, Shao JY, Ooi A, et al. Serglycin is a theranostic target in nasopharyngeal carcinoma that promotes metastasis. Cancer Res (2011) 71(8):3162–72. doi:10.1158/0008-5472.CAN-10-3557
23. He J, Zeng ZC, Xiang ZL, Yang P. Mass spectrometry-based serum peptide profiling in hepatocellular carcinoma with bone metastasis. World J Gastroentero (2014) 20(11):3025–32. doi:10.3748/wjg.v20.i11.3025
24. Purushothaman A, Toole BP. Serglycin proteoglycan is required for multiple myeloma cell adhesion, in vivo growth, and vascularization. J Biol Chem (2014) 289(9):5499–509. doi:10.1074/jbc.M113.532143
25. Rescan PY, Loréal O, Hassell JR, Yamada Y, Guillouzo A, Clément B. Distribution and origin of the basement membrane component perlecan in rat liver and primary hepatocyte culture. Am J Pathol (1993) 142(1):199–208.
26. Tátrai P, Egedi K, Somorácz A, van Kuppevelt TH, Ten Dam G, Lyon M, et al. Quantitative and qualitative alterations of heparan sulfate in fibrogenic liver diseases and hepatocellular cancer. J Histochem Cytochem (2010) 58:429–41. doi:10.1369/jhc.2010.955161
27. Cohen IR, Murdoch AD, Naso MF, Marchetti D, Berd D, Iozzo RV. Abnormal expression of perlecan proteoglycan in metastatic melanomas. Cancer Res (1994) 54(22):5771–4.
28. Adatia R, Albini A, Carlone S, Giunciuglio D, Benelli R, Santi L, et al. Suppression of invasive behavior of melanoma cells by stable expression of anti-sense perlecan cDNA. Ann Oncol (1997) 8(12):1257–61. doi:10.1023/A:1008243115385
29. Sharma B, Handler M, Eichstetter I, Whitelock JM, Nugent MA, Iozzo RV. Antisense targeting of perlecan blocks tumor growth and angiogenesis in vivo. J Clin Invest (1998) 102(8):1599–608. doi:10.1172/JCI3793
30. Binder MJ, McCoombe S, Williams ED, McCulloch DR, Ward AC. The extracellular matrix in cancer progression: role of hyalectan proteoglycans and ADAMTS enzymes. Cancer Lett (2017) 385:55–64. doi:10.1016/j.canlet.2016.11.001
31. Wight TN. Versican: a versatile extracellular matrix proteoglycan in cell biology. Curr Opin Cell Biol (2002) 14:617–23. doi:10.1016/S0955-0674(02)00375-7
32. Skandalis SS, Theocharis AD, Vynios DH, Papageorgakopoulou N, Hjerpe A, Karamanos NK, et al. Cartilage aggrecan undergoes significant compositional and structural alterations during laryngeal cancer. Biochim Biophys Acta (2006) 1760:1046–53. doi:10.1016/j.bbagen.2006.02.007
33. Neill T, Schaefer L, Iozzo RV. Oncosuppressive functions of decorin. Mol Cell Oncol (2015) 2(3):e975645. doi:10.4161/23723556.2014.975645
34. Neill T, Schaefer L, Iozzo RV. Decoding the matrix: instructive roles of proteoglycan receptors. Biochemistry (2015) 54:4583–98. doi:10.1021/acs.biochem.5b00653
35. Feng M, Gao W, Wang R, Chen W, Man YG, Figg WD, et al. Therapeutically targeting glypican-3 via a conformation-specific single-domain antibody in hepatocellular carcinoma. Proc Natl Acad Sci U S A (2013) 110(12):E1083–91. doi:10.1073/pnas.1217868110
36. Zhu ZW, Friess H, Wang L, Abou-Shady M, Zimmermann A, Lander AD, et al. Enhanced glypican-3 expression differentiates the majority of hepatocellular carcinomas from benign hepatic disorders. Gut (2001) 48(4):558–64. doi:10.1136/gut.48.4.558
37. Liu Y, Zheng D, Liu M, Bai J, Zhou X, Gong B, et al. Downregulation of glypican-3 expression increases migration, invasion, and tumorigenicity of human ovarian cancer cells. Tumour Biol (2015) 36(10):7997–8006. doi:10.1007/s13277-015-3528-6
38. Nikitovic D, Mytilinaiou M, Berdiaki A, Karamanos NK, Tzanakakis GN. Heparan sulfate proteoglycans and heparin regulate melanoma cell functions. Biochim Biophys Acta (2014) 1840(8):2471–81. doi:10.1016/j.bbagen.2014.01.031
39. Voudouri K, Berdiaki A, Tzardi M, Tzanakakis GN, Nikitovic D. Insulin-like growth factor and epidermal growth factor signaling in breast cancer cell growth: focus on endocrine resistant disease. Anal Cell Pathol (Amst) (2015) 2015:975495. doi:10.1155/2015/975495
40. Farabaugh SM, Boone DN, Lee AV. Role of IGF1R in breast cancer subtypes, stemness, and lineage differentiation. Front Endocrinol (2015) 6:59. doi:10.3389/fendo.2015.00059
41. Bernfield M, Sanderson RD. Syndecan, a developmentally regulated cell surface proteoglycan that binds extracellular matrix and growth factors. Philos Trans R Soc Lond B Biol Sci (1990) 327:171–86. doi:10.1098/rstb.1990.0052
42. Olwin BB, Rapraeger A. Repression of myogenic differentiation by aFGF, bFGF, and K-FGF is dependent on cellular heparan sulfate. J Cell Biol (1992) 118:631–9. doi:10.1083/jcb.118.3.631
43. Derksen PW, Keehnen RM, Evers LM, van Oers MH, Spaargaren M, Pals ST. Cell surface proteoglycan syndecan-1 mediates hepatocyte growth factor binding and promotes Met signaling in multiple myeloma. Blood (2002) 99:1405–10. doi:10.1182/blood.V99.4.1405
44. Mytilinaiou M, Bano A, Nikitovic D, Berdiaki A, Voudouri K, Kalogeraki A, et al. Syndecan-2 is a key regulator of transforming growth factor beta 2/Smad2-mediated adhesion in fibrosarcoma cells. IUBMB Life (2013) 65(2):134–43. doi:10.1002/iub.1112
45. Mytilinaiou M, Nikitovic D, Berdiaki A, Papoutsidakis A, Papachristou DJ, Tsatsakis A, et al. IGF-I regulates HT1080 fibrosarcoma cell migration through a syndecan-2/erk/ezrin signaling axis. Exp Cell Res (2017) 361(1):9–18. doi:10.1016/j.yexcr.2017.09.035
46. Zaghloul RA, El-Shishtawy MM, El Galil KH, Ebrahim MA, Metwaly AA, Al-Gayyar MM. Evaluation of antiglypican-3 therapy as a promising target for amelioration of hepatic tissue damage in hepatocellular carcinoma. Eur J Pharmacol (2015) 746:353–62. doi:10.1016/j.ejphar.2014.11.008
47. Skandalis SS, Afratis N, Smirlaki G, Nikitovic D, Theocharis AD, Tzanakakis GN, et al. Cross-talk between estradiol receptor and EGFR/IGF-IR signaling pathways in estrogen-responsive breast cancers: focus on the role and impact of proteoglycans. Matrix Biol (2014) 35:182–93. doi:10.1016/j.matbio.2013.09.002
48. Troup S, Njue C, Kliewer EV, Parisien M, Roskelley C, Chakravarti S, et al. Reduced expression of the small leucine-rich proteoglycans, lumican, and decorin is associated with poor outcome in node-negative invasive breast cancer. Clin Cancer Res (2003) 9:207–14.
49. Moscatello DK, Santra M, Mann DM, McQuillan DJ, Wong AJ, Iozzo RV. Decorin suppresses tumor cell growth by activating the epidermal growth factor receptor. J Clin Invest (1998) 101:406–12. doi:10.1172/JCI846
50. Zhu JX, Goldoni S, Bix D, Owens RT, McQuillan DJ, Reed CC, et al. Decorin evokes protracted internalization and degradation of the epidermal growth factor receptor via caveolar endocytosis. J Biol Chem (2005) 280:32468–79. doi:10.1074/jbc.M503833200
51. Iozzo RV, Moscatello DK, McQuillan DJ, Eichstetter I. Decorin is a biological ligand for the epidermal growth factor receptor. J Biol Chem (1999) 274:4489–92. doi:10.1074/jbc.274.8.4489
52. De Luca A, Santra M, Baldi A, Giordano A, Iozzo RV. Decorin-induced growth suppression is associated with up-regulation of p21, an inhibitor of cyclin-dependent kinases. J Biol Chem (1996) 271:18961–5. doi:10.1074/jbc.271.31.18961
53. Du WW, Yang BB, Shatseva TA, Yang BL, Deng Z, Shan SW, et al. Versican G3 promotes mouse mammary tumor cell growth, migration, and metastasis by influencing EGF receptor signaling. PLoS One (2010) 5:e13828. doi:10.1371/journal.pone.0013828
54. Du WW, Yang BB, Yang BL, Yang Z, Deng L, Fang SW, et al. Versican G3 domain modulates breast cancer cell apoptosis: a mechanism for breast cancer cell response to chemotherapy and EGFR therapy. PLoS One (2011) 6:e26396. doi:10.1371/journal.pone.0026396
55. Morcavallo A, Stefanello M, Iozzo RV, Belfiore A, Morrione A. Ligand-mediated endocytosis and trafficking of the insulin-like growth factor receptor I and insulin receptor modulate receptor function. Front Endocrinol (2014) 5:220. doi:10.3389/fendo.2014.00220
56. Baghy K, Horvath Z, Regos E, Kiss K, Schaff Z, Iozzo RV, et al. Decorin interferes with platelet-derived growth factor receptor signaling in experimental hepatocarcinogenesis. FEBS J (2013) 280(10):2150–64. doi:10.1111/febs.12215
57. Hasegawa M, Cheng J, Maruyama S, Yamazaki M, Abé T, Babkair H, et al. Differential immunohistochemical expression profiles of perlecan-binding growth factors in epithelial dysplasia, carcinoma in situ, and squamous cell carcinoma of the oral mucosa. Pathol Res Pract (2016) 212(5):426–36. doi:10.1016/j.prp.2016.02.016
58. Naboulsi W, Megger DA, Bracht T, Kohl M, Turewicz M, Eisenacher M, et al. Quantitative tissue proteomics analysis reveals versican as potential biomarker for early-stage hepatocellular carcinoma. J Proteome Res (2016) 15(1):38–47. doi:10.1021/acs.jproteome.5b00420
59. López JI, Angulo JC, Martín A, Sánchez-Chapado M, González-Corpas A, Colás B, et al. A DNA hypermethylation profile reveals new potential biomarkers for the evaluation of prognosis in urothelial bladder cancer. APMIS (2017) 125(9):787–96. doi:10.1111/apm.12719
60. Mitsui Y, Shiina H, Kato T, Maekawa S, Hashimoto Y, Shiina M, et al. Versican promotes tumor progression, metastasis and predicts poor prognosis in renal carcinoma. Mol Cancer Res (2017) 15(7):884–95. doi:10.1158/1541-7786
61. Lisowska KM, Olbryt M, Student S, Kujawa KA, Cortez AJ, Simek K, et al. Unsupervised analysis reveals two molecular subgroups of serous ovarian cancer with distinct gene expression profiles and survival. J Cancer Res Clin Oncol (2016) 142(6):1239–52. doi:10.1007/s00432-016-2147-y
62. Chida S, Okayama H, Noda M, Saito K, Nakajima T, Aoto K, et al. Stromal VCAN expression as a potential prognostic biomarker for disease recurrence in stage II-III colon cancer. Carcinogenesis (2016) 37(9):878–87. doi:10.1093/carcin/bgw069
63. Roy A, Attarha S, Weishaupt H, Edqvist PH, Swartling FJ, Bergqvist M, et al. Serglycin as a potential biomarker for glioma: association of serglycin expression, extent of mast cell recruitment and glioblastoma progression. Oncotarget (2017) 8(15):24815–27. doi:10.18632/oncotarget.15820
64. Brézillon S, Untereiner V, Mohamed HT, Hodin J, Chatron-Colliet A, Maquart FX, et al. Probing glycosaminoglycan spectral signatures in live cells and their conditioned media by Raman microspectroscopy. Analyst (2017) 142(8):1333–41. doi:10.1039/c6an01951j
65. Sanderson RD, Elkin M, Rapraeger AC, Ilan N, Vlodavsky I. Heparanase regulation of cancer, autophagy and inflammation: new mechanisms and targets for therapy. FEBS J (2017) 284(1):42–55. doi:10.1111/febs.13932
66. Vlodavsky I, Gross-Cohen M, Weissmann M, Ilan N, Sanderson RD. Opposing functions of Heparanase-1 and Heparanase-2 in cancer progression. Trends Biochem Sci (2017) 43(1):18–31. doi:10.1016/j.tibs.2017.10.007
67. El Ghazal R, Yin X, Johns SC, Swanson L, Macal M, Ghosh P, et al. Glycan sulfation modulates dendritic cell biology and tumor growth. Neoplasia (2016) 18(5):294–306. doi:10.1016/j.neo.2016.04.004
68. Appunni S, Anand V, Khandelwal M, Seth A, Mathur S, Sharma A. Altered expression of small leucine-rich proteoglycans (Decorin, Biglycan and Lumican): plausible diagnostic marker in urothelial carcinoma of bladder. Tumour Biol (2017) 39(5):1010428317699112. doi:10.1177/1010428317699112
69. Szarvas T, Reis H, Vom Dorp F, Tschirdewahn S, Niedworok C, Nyirady P, et al. Soluble syndecan-1 (SDC1) serum level as an independent pre-operative predictor of cancer-specific survival in prostate cancer. Prostate (2016) 76(11):977–85. doi:10.1002/pros.23186
70. Takashima S, Oka Y, Fujiki F, Morimoto S, Nakajima H, Nakae Y, et al. Syndecan-4 as a biomarker to predict clinical outcome for glioblastoma multiforme treated with WT1 peptide vaccine. Future Sci OA (2016) 2(4):FSO96. doi:10.4155/fsoa-2015-0008
71. Li J, Chen Y, Guo X, Zhou L, Jia Z, Peng Z, et al. GPC1 exosome and its regulatory miRNAs are specific markers for the detection and target therapy of colorectal cancer. J Cell Mol Med (2017) 21(5):838–47. doi:10.1111/jcmm.12941
72. Sluiter NR, de Cuba EM, Kwakman R, Meijerink WJ, Delis-van Diemen PM, Coupé VM, et al. Versican and vascular endothelial growth factor expression levels in peritoneal metastases from colorectal cancer are associated with survival after cytoreductive surgery and hyperthermic intraperitoneal chemotherapy. Clin Exp Metastasis (2016) 33(4):297–307. doi:10.1007/s10585-016-9779-9
73. Real FX, Houghton AN, Albino AP, Cordon-Cardo C, Melamed MR, Oettgen HF, et al. Surface antigens of melanomas and melanocytes defined by mouse monoclonal antibodies: specificity analysis and comparison of antigen expression in cultured cells and tissues. Cancer Res (1985) 45(9):4401–11.
74. Ilieva KM, Cheung A, Mele S, Chiaruttini G, Crescioli S, Griffin M, et al. Chondroitin sulfate proteoglycan 4 and its potential as an antibody immunotherapy target across different tumor types. Front Immunol (2017) 8:1911. doi:10.3389/fimmu.2017.01911
75. Depau L, Brunetti J, Falciani C, Scali S, Riolo G, Mandarini E, et al. Coupling to a cancer-selective heparan-sulfate-targeted branched peptide can by-pass breast cancer cell resistance to methotrexate. Oncotarget (2017) 8(44):76141–52. doi:10.18632/oncotarget.19056
76. Bosse KR, Raman P, Zhu Z, Lane M, Martinez D, Heitzeneder S, et al. Identification of GPC2 as an oncoprotein and candidate immunotherapeutic target in high-risk neuroblastoma. Cancer Cell (2017) 32(3):295–309 e12. doi:10.1016/j.ccell.2017.08.003
77. Hope C, Emmerich PB, Papadas A, Pagenkopf A, Matkowskyj KA, Van De Hey DR, et al. Versican-derived matrikines regulate Batf3-dendritic cell differentiation and promote T cell infiltration in colorectal cancer. J Immunol (2017) 199(5):1933–41. doi:10.4049/jimmunol.1700529
78. Peyrode C, Weber V, Voissiere A, Maisonial-Besset A, Vidal A, Auzeloux P, et al. Proteoglycans as target for an innovative therapeutic approach in chondrosarcoma: preclinical proof of concept. Mol Cancer Ther (2016) 15(11):2575–85. doi:10.1158/1535-7163.MCT-16-0003
79. Zhu AX, Gold PJ, El-Khoueiry AB, Abrams TA, Morikawa H, Ohishi N, et al. First-in-man phase I study of GC33, a novel recombinant humanized antibody against glypican-3, in patients with advanced hepatocellular carcinoma. Clin Cancer Res (2013) 19(4):920–8. doi:10.1158/1078-0432.CCR-12-2616
80. Sawada Y, Yoshikawa T, Nobuoka D, Shirakawa H, Kuronuma T, Motomura Y, et al. Phase I trial of a glypican-3-derived peptide vaccine for advanced hepatocellular carcinoma: immunologic evidence and potential for improving overall survival. Clin Cancer Res (2012) 18(13):3686–96. doi:10.1158/1078-0432.CCR-11-3044
81. Ikeda M, Ohkawa S, Okusaka T, Mitsunaga S, Kobayashi S, Morizane C, et al. Japanese phase I study of GC33, a humanized antibody against glypican-3 for advanced hepatocellular carcinoma. Cancer Sci (2014) 105(4):455–62. doi:10.1111/cas.12368
82. Sawada Y, Yoshikawa T, Shimomura M, Iwama T, Endo I, Nakatsura T. Programmed death-1 blockade enhances the antitumor effects of peptide vaccine-induced peptide-specific cytotoxic T lymphocytes. Int J Oncol (2015) 46(1):28–36. doi:10.3892/ijo.2014.2737
Keywords: proteoglycans, cancer biology, biomarkers, molecular signature, cytokine signaling
Citation: Nikitovic D, Berdiaki A, Spyridaki I, Krasanakis T, Tsatsakis A and Tzanakakis GN (2018) Proteoglycans—Biomarkers and Targets in Cancer Therapy. Front. Endocrinol. 9:69. doi: 10.3389/fendo.2018.00069
Received: 19 December 2017; Accepted: 16 February 2018;
Published: 06 March 2018
Edited by:
Haim Werner, Tel Aviv University, IsraelReviewed by:
Eva Surmacz, Temple University, United StatesCopyright: © 2018 Nikitovic, Berdiaki, Spyridaki, Krasanakis, Tsatsakis and Tzanakakis. This is an open-access article distributed under the terms of the Creative Commons Attribution License (CC BY). The use, distribution or reproduction in other forums is permitted, provided the original author(s) and the copyright owner are credited and that the original publication in this journal is cited, in accordance with accepted academic practice. No use, distribution or reproduction is permitted which does not comply with these terms.
*Correspondence: Dragana Nikitovic, bmlraXRvdmljQHVvYy5ncg==
Disclaimer: All claims expressed in this article are solely those of the authors and do not necessarily represent those of their affiliated organizations, or those of the publisher, the editors and the reviewers. Any product that may be evaluated in this article or claim that may be made by its manufacturer is not guaranteed or endorsed by the publisher.
Research integrity at Frontiers
Learn more about the work of our research integrity team to safeguard the quality of each article we publish.