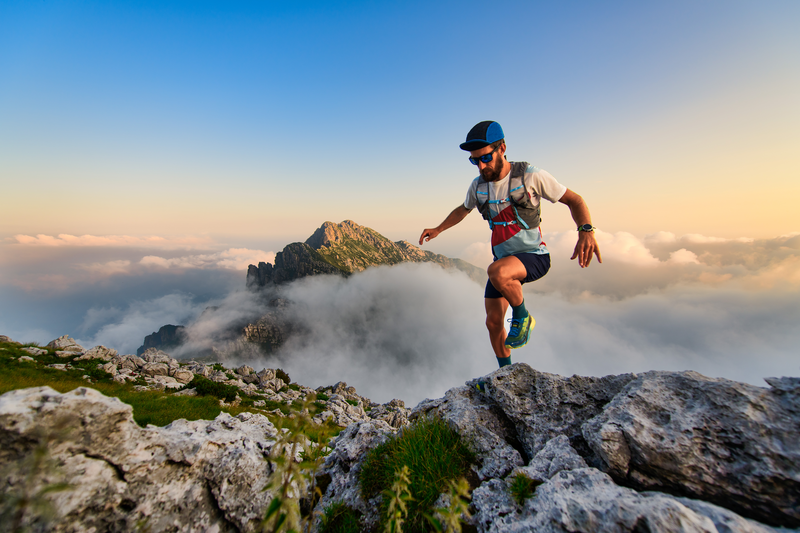
94% of researchers rate our articles as excellent or good
Learn more about the work of our research integrity team to safeguard the quality of each article we publish.
Find out more
REVIEW article
Front. Endocrinol. , 20 February 2018
Sec. Molecular and Structural Endocrinology
Volume 9 - 2018 | https://doi.org/10.3389/fendo.2018.00048
This article is part of the Research Topic Endocrine and Paracrine Role of FGF23 and Klotho in Health and Disease View all 13 articles
Fibroblast growth factor 23 (FGF23) is a phosphotropic hormone mainly produced by bone. FGF23 reduces serum phosphate by suppressing intestinal phosphate absorption through reducing 1,25-dihydroxyvitamin D and proximal tubular phosphate reabsorption. Excessive actions of FG23 result in several kinds of hypophosphatemic rickets/osteomalacia including X-linked hypophosphatemic rickets (XLH) and tumor-induced osteomalacia. While neutral phosphate and active vitamin D are standard therapies for child patients with XLH, these medications have several limitations both in their effects and adverse events. Several approaches that inhibit FGF23 actions including anti-FGF23 antibodies and inhibitors of FGF signaling have been shown to improve phenotypes of model mice for FG23-related hypophosphatemic diseases. In addition, clinical trials indicated that a humanized anti-FGF23 antibody increased serum phosphate and improved quality of life in patients with XLH. Furthermore, circulatory FGF23 is high in patients with chronic kidney disease (CKD). Many epidemiological studies indicated the association between high FGF23 levels and various adverse events especially in patients with CKD. However, it is not known whether the inhibition of FGF23 activities in patients with CKD is beneficial for these patients. In this review, recent findings concerning the modulation of FGF23 activities are discussed.
Fibroblast growth factor 23 (FGF23) was identified as a responsible gene for autosomal dominant hypophosphatemic rickets (ADHR) in 2000 by positional cloning (1). FGF23 was also cloned as a responsible humoral factor for tumor-induced osteomalacia (TIO), a rare paraneoplastic syndrome (2). ADHR and TIO are diseases characterized by hypophosphatemia associated with impaired renal tubular phosphate reabsorption. Subsequent studies established that FGF23 is a hormone mainly produced by bone and regulates phosphate and vitamin D metabolism by binding to Klotho–FGF receptor complex (3–7). Klotho is a single membrane spanning protein. While there is also soluble Klotho, its role in FGF23 signaling is not clear (8). FGF23 was shown to transduce signals only in tissues which express membrane form Klotho (6). FGF23 reduces serum phosphate by inhibiting intestinal phosphate absorption through decreasing serum 1,25-dihydroxyvitamin D [1,25(OH)2D] level and proximal tubular phosphate reabsorption (3). Therefore, ADHR and TIO have been considered to be caused by excessive actions of FGF23. These results lead to develop a concept that the inhibition of FGF23 activities may be beneficial for hypophosphatemic patients caused by excessive actions of FGF23. Furthermore, it has been shown that FGF23 is high in patients with chronic kidney disease (CKD) and can be extremely high in some subjects with end-stage renal disease (ESRD) (9, 10). Circulatory FGF23 levels have been shown to be associated with various adverse events such as higher mortality, cardiovascular events, and left ventricular hypertrophy, especially in subjects with CKD. However, it is not known whether these events are direct consequences of FGF23 activities (11). In this review, the significance of inhibiting FGF23 activities in various diseases is discussed.
Chronic hypophosphatemia is an important cause for rickets and osteomalacia characterized by impaired mineralization of bone matrix. Rickets develops in children before the closure of epiphyseal plate and results in growth retardation and bone deformities. In contrast, osteomalacia in adults can cause severe muscle weakness and bone pain. Serum phosphate level is maintained by intestinal phosphate absorption, renal tubular phosphate handling and equilibrium between extracellular phosphate and phosphate in bone or intracellular fluid. Of these, renal phosphate handling is the main determinant of chronic serum phosphate level. Most phosphate filtered through glomeruli is reabsorbed in proximal tubules by type 2a and 2c sodium-phosphate cotransporters. FGF23 reduces the expression of these sodium-phosphate cotransporters and suppresses phosphate reabsorption (3). At the same time, FGF23 reduces serum 1,25(OH)2D by modulating the expression of vitamin D-metabolizing enzymes (3). FGF23 suppresses the expression of CYP27B1 that encodes a protein responsible for the production of 1,25(OH)2D. FGF23 also enhances CYP24A1 expression that encodes an enzyme that works to reduce 1,25(OH)2D level.
After the identification of FGF23, several kinds of enzyme-linked immunosorbent assay for FGF23 have been established (12, 13). A part of FGF23 protein is proteolytically cleaved into inactive N-terminal and C-terminal fragments before or during the process of secretion. FGF23 level can be regulated by both FGF23 transcription and this posttranslational processing of FGF23 protein. For example, iron deficiency seems to enhance FGF23 production and also the processing of FGF23 protein (14). Therefore, FGF23 level does not always reflect the amount of FGF23 transcription. Intact assay using two kinds of antibodies that recognize N-terminal and C-terminal portions of the processing site of FGF23 detects only full-length biologically active FGF23 (12). In contrast, C-terminal assay using antibodies against the C-terminal part of FGF23 measures both full-length and processed inactive C-terminal fragment of FGF23 (13). FGF23 level measured by C-terminal assay seems to correlate with the amount of FGF23 transcription. Intravenous iron preparations inhibit gene expression of FGF23. While iron dextrane does not affect the cleavage of FGF23, several iron preparations such as iron polymaltose and iron carboxymaltose may block the cleavage, resulting in paradoxical hypophosphatemia (14). This two step regulation of FGF23 needs to be kept in mind when interpreting results of FGF23 levels by intact and C-terminal FGF23 assays. Measurement of FGF23 levels in patients with chronic hypophosphatemia indicated that FGF23 levels are high in hypophosphatemic patients with ADHR and TIO by both assays (12, 13, 15). In contrast, FGF23 levels are low in patients with chronic hypophosphatemia from other causes such as vitamin D deficiency and Fanconi syndrome indicating that circulatory FGF23 level is suppressed in these patients by hypophosphatemia or other accompanying metabolic changes (16). From these results, high FGF23 in patients with chronic hypophosphatemia seemed to indicate that this hypophosphatemia is caused by excessive actions of FGF23.
In addition to patients with ADHR and TIO, FGF23 levels have been shown to be high in several kinds of hypophosphatemic diseases (Table 1). Of these, X-linked hypophosphatemic rickets (XLH) caused by inactivating mutations of phosphate-regulating gene with homologies to endopeptidases on the X chromosome (PHEX) is the most prevalent cause of genetic hypophosphatemic disease. More than three hundred kinds of mutations in PHEX have been assembled in a database.1 PHEX is a single membrane spanning protein mainly expressed in bone and teeth (17). There is a murine model of XLH called Hyp. Hyp mice show similar biochemical features to those of patients with XLH. Genetic analysis indicated that there is a deletion in 3′ region of Phex gene in Hyp mice (18). It has been shown that Fgf23 is overexpressed in bone and circulatory Fgf23 is high in Hyp mice (19). Therefore, it is believed that inactivating mutations in PHEX somehow induce enhanced expression of FGF23 in bone and cause excessive actions of FGF23 in patients with XLH. Signals from FGF receptor was reported to be involved in the overproduction of FGF23 production in Hyp mice (7). However, the precise detailed role of PHEX in the regulation of FGF23 expression needs to be established.
Mutations in dentin matrix protein 1 (DMP1) and ectonucleotide pyrophosphatase/phosphodiesterase 1 (ENPP1) results in autosomal recessive hypophosphatemic rickets 1 and 2, respectively (21–24). Furthermore, mutations in several other genes have been shown to cause hypophosphatemic diseases with high FGF23 levels (31). Inactivating mutations in FAM20C was reported in Raine syndrome, a usually lethal osteosclerotic disease (32). However, hypophosphatemia with high FGF23 was later reported in some surviving patients (27). Osteoglophonic dysplasia is caused activating mutations in FGF receptor 1. Hypophosphatemia with high FGF23 in this disease again suggests the involvement of signals from FGF receptor in FGF23 production (25). Epidermal nevus syndrome is caused by somatic mutations in RAS oncogenes and is characterized by sebaceous nevi and skeletal defects (29). These oncogene products can transduce signals from receptor tyrosine kinases including FGF receptor. Jansen-type metaphyseal chodrodysplasia and McCune-Albright syndrome are caused by activating mutations in parathyroid hormone (PTH) 1 receptor and GNAS1, respectively (26, 28). These results suggest that cyclic AMP pathway is involved in FGF23 production. In some patients with TIO, FN (fibronectin)-FGF receptor 1 or FN-FGF1 fusion gene was reported in responsible tumors (30). It is likely that these genes activate some intracellular signaling pathway to enhance FGF23 production.
In addition to diseases with known genetic causes, hypophosphatemia with high FGF23 has been reported in patients receiving some intravenous iron preparations (33, 34). Recently, it has been reported that biliary atresia can be associated with hypophosphatemia with high FGF23 (35). In most of these FGF23-related hypophosphatemic diseases, FGF23 is considered to be overexpressed in bone while the detailed mechanism of this overproduction is not clear. On the contrary, in patients with TIO, the responsible tumors produce FGF23 and FGF23 is shown to be expressed in liver in a patient with biliary atresia. Collectively, these results indicate that excessive production and actions of FGF23 can cause several kinds of hypophosphatemic diseases.
Tumor-induced osteomalacia is a paraneoplastic syndrome and can be cured by complete resection of the responsible tumors. However, it is sometimes difficult to find the responsible tumors in patients with TIO. Even when the responsible tumors can be found, it is not always possible to completely remove the lesions. For those patients with TIO whose responsible tumors cannot be removed, neutral phosphate and active vitamin D are usually prescribed. For patients with most other FGF23-related hypophosphatemic diseases including XLH, the same drugs are also used. However, these medications are not drugs based on the pathophysiology of these diseases. In addition, these medications may not able to correct impaired growth completely in patients with hypophosphatemic rickets (36). Furthermore, administration of phosphate and active vitamin D can be associated with several adverse events such as hypercalcemia, hypercalciuria, nephrocalcinosis, and gastrointestinal symptoms (37).
It has been shown that excessive activities of FGF23 underlie the pathogenesis of FGF23-related hypophosphatemic diseases as mentioned above. Therefore, the suppression of FGF23 activities has been considered as a novel therapy for patients with these diseases. Murine monoclonal antibodies against N-terminal and C-terminal portions of human FGF23 were obtained. These antibodies inhibited in vitro FGF23 activity and synergistically increased serum phosphate and 1,25(OH)2D levels in normal mice (38). These results as well as phenotypes of hyperphosphatemia and high 1,25(OH)2D in Fgf23 null mice confirmed that FGF23 is a physiological regulator of phosphate and vitamin D metabolism (4, 38). Then, these antibodies were tested in Hyp mice and were shown to increase serum phosphate and 1,25(OH)2D (39). In addition, repeated administration of these antibodies enhanced longitudinal growth of long bones, increased bone mineral density and corrected impaired mineralization of Hyp mice (39). Furthermore, these antibodies increased grip power and spontaneous movement of Hyp mice (40). These results suggested that the inhibition of FGF23 activities by FGF23 antibodies can ameliorate biochemical, morphological, histological and clinical abnormalities of patients with FGF23-related hypophosphatemic rickets/osteomalacia.
Based on the results of these preclinical studies, humanized anti-FGF23 monoclonal antibody (burosumab) was developed and tested in several clinical trials. The initial phase I study involving 38 adult patients with XLH showed that burosumab increased serum phosphate, 1,25(OH)2D and tubular maximum transport of phosphate per glomerular filtration rate (TmP/GFR), an index of proximal tubular phosphate reabsorption, in a dose-dependent manner (41). Subsequent study with 28 adult patients with XLH showed that subcutaneous injections of burosumab every 4 weeks increased serum phosphate, 1,25(OH)2D and TmP/GFR after each administration (42). Furthermore, burosumab was shown to significantly improve patient perception of physical functioning and stiffness (43). These results indicated that burosumab can improve biochemical abnormalities of adult patients with XLH and improve quality of life. However, it was unclear from these studies whether burosumab can also work in children and ameliorate rickets/osteomalacia.
While the results have not yet been published as articles so far, several clinical trials with burosumab are ongoing. Results presented in several scientific meetings and available on the web suggest that burosumab can improve biochemical abnormalities in child patients with XLH and also in patients with TIO.2,3 In addition, burosumab seems to improve roentgenological signs of rickets in children. Based on these results, the US Food and Drug Administration granted a Breakthrough Therapy Designation for burosumab in 2016 (see footnote 2). However, there are several questions to be answered in the future studies. Long-term safety should be carefully monitored. In addition, it has not yet been shown whether burosumab can heal rickets/osteomalacia and normalize height in child patients with XLH. Furthermore, it has not been established how long burosumab should be used for child patients with XLH. Theoretically, burosumab seems to be effective in patients with other FGF23-related hypophosphatemic diseases than XLH and TIO. This also needs to be proved in the future trials. Collectively, burosumab has been shown to inhibit FGF23 actions in human and seems to be promising as a novel therapy for patients with FGF23-related hypophosphatemic diseases.
In addition to anti-FGF23 antibody, a computationally identified compound binding to FGF23 was also shown to increase serum phosphate and 1,25(OH)2D in a model mouse of FGF23-related hypophosphatemic diseases (44). These compounds can be cost-effective compared to the antibody. However, this small molecule has not been used in clinical trials.
Another approach is to inhibit the binding of FGF23 to Klotho–FGF receptor complex. Purified C-terminal fragment of FGF23 protein was shown to compete with full-length FGF23 for the binding to Klotho–FGF receptor complex (45). Administration of this C-terminal fragment of FGF23 temporally increased serum phosphate of Hyp mice (45). The C-terminal portion of FGF23 protein was fused to Fc portion of IgG1 to increase the stability of the C-terminal fragment (46). This FGF23 c-tail-Fc fusion molecule also increased serum phosphate and improved mineralization of Hyp mice when repeatedly injected (46). In addition to this C-terminal fragment of FGF23, several small compounds were shown to impair the interaction between FGF23 and Klotho–FGF receptor complex (47).
In addition, the inhibition of FGF receptor was shown to inhibit FGF23 activities (48, 49). Several reports suggest that signals from FGF receptor stimulate FGF23 production in bone (7, 48). Therefore, it is possible that the inhibition of FGF receptor suppresses both the production and the actions of FGF23. NVP-BGJ398, a pan FGF receptor inhibitor, increased serum phosphate, enhanced bone growth, increased mineralization, and corrected the disturbed growth plate structure in Hyp mice (49).
The inhibition of mitogen-activated protein kinase (MAPK) pathway was also shown to impair FGF23 actions while FGF receptor can activate several signal transduction pathways. PD0325901, an inhibitor of MAPK pathway, was shown to increase serum phosphate and 1,25(OH)2D, and correct impaired mineralization in Hyp mice (50). These preclinical studies indicated that there are several ways to inhibit excessive FGF23 activities causing FGF23-related hypophosphatemic diseases.
However, FGF receptor-MAPK pathway is involved in numerous biological processes in addition to mediating FGF23 actions suggesting that the inhibition of this pathway can be associated with various adverse events. NVP-BGJ398 and PD0325901 have been tested in several clinical trials mainly for patients with solid cancers.4 NVP-BGJ398 was shown to inhibit FGF23 secretion in patients with TIO caused by malignant tumors (51). However, these inhibitors have not yet tested for patients with genetic FGF23-related hypophosphatemic diseases or TIO caused by benign tumors. FGF23 c-tail-Fc fusion molecule and other small compounds that impair the binding of FGF23 to Klotho–FGF receptor complex have not been tested in clinical trials, either.
Circulatory FGF23 level is high in patients with CKD. Several studies indicated that FGF23 starts to increase in the early phase of CKD (10, 52). This increase of FGF23 precedes those of PTH and phosphate. While the detailed regulatory mechanisms of FGF23 production and circulatory FGF23 levels remain to be clarified, 1,25(OH)2D and high phosphate diet were shown to enhance FGF23 levels (53). However, in patients with early CKD with high FGF23 level, 1,25(OH)2D and phosphate are not high. Recent studies suggest that inflammation and iron deficiency are involved in this high FGF23 in patients with CKD (54).
In a model rat of early CKD, the inhibition of Fgf23 activity by anti-FGF23 antibodies were shown to enhance proximal tubular phosphate reabsorption and increase serum phosphate and 1,25(OH)2D (55). These results suggest that high FGF23 in patients with early CKD suppresses phosphate reabsorption and works to prevent the development of hyperphosphatemia. The inhibition of Fgf23 activities by anti-FGF23 antibody in a model rat of CKD was also shown to cause higher mortality by increasing serum phosphate and promoting ectopic calcification (56). Therefore, this increase of FGF23 in patients with early CKD is considered to be one of adaptive responses to maintain mineral homeostasis.
In contrast, there are now many epidemiological studies that indicate the association between high FGF23 levels and various adverse events especially in patients with CKD or ESRD (11). For example, high FGF23 levels in patients who were beginning hemodialysis were shown to be associated with higher mortality during the first year of hemodialysis (57). Adverse events associated with high FGF23 are quite diverse including cardiovascular events, left ventricular hypertrophy, progression of CKD, fractures, higher mortality, frailty, insulin resistance, and so on. These associations suggest that the inhibition of FGF23 activity may be beneficial in patients with CKD under certain conditions. Actually, secondary analysis of Evaluation Of Cinacalcet Hydrochloride Therapy to Lower CardioVascular Events indicated that cinacalcet-induced reductions in serum FGF23 were associated with lower rates of cardiovascular death and major cardiovascular events (58). However, there has been no study that examined effects of inhibiting FGF23 activities by anti-FGF23 antibody or inhibitors in patients with ESRD. In addition, the reason for the association between high FGF23 levels and various adverse events are not clear enough. While it was reported that FGF23 signals in a Klotho-independent fashion through FGFR4/nuclear factor of activated T cells/calcineurin, which may cause left ventricular hypertrophy (59, 60), it has not been shown how FGF23 can activate FGF receptor without Klotho. In addition, it is not clear either whether FGF23 induces various adverse events directly working on several tissues like heart, bone and kidney. Further studies are necessary to establish the usefulness of inhibiting FGF23 activities in patients with CKD.
Fibroblast growth factor 23 is a hormone that reduces serum phosphate and 1,25(OH)2D levels. For patients with excessive hormone actions, therapeutic measures to suppress the activities of that hormone are used. Therefore, it is reasonable to develop methods to inhibit FGF23 actions for patients with FGF23-related hypophosphatemic diseases. Patients with hypophosphatemic disorders by FGF23 excess may benefit from FGF23 blocking antibodies or inhibitors of FGF23 signaling. However, these therapies need careful monitoring because deficient actions of FGF23 result in hyperphosphatemic disease. On the contrary, FGF23 seems to be high in response to changes of mineral homeostasis or other metabolic process in patients with CKD. Patients with CKD may benefit from these novel therapies to the extent that FGF23 secretion is excessively stimulated and beyond what’s needed in compensation. However, there is not enough evidence that indicates the inhibition of FGF23 activities is useful for patients with CKD.
The author confirms being the sole contributor of this work and approved it for publication.
The department is supported by Chugai Pharmaceutical Co., Ltd., Taisho Pharmaceutical Co., Ltd., Ono Pharmaceutical Co., Ltd., and Kyowa Hakko Kirin Co., Ltd.
This work was supported in part by Grants-in-Aid for Scientific Research from Ministry of Health, Labour and Welfare.
1. ADHR Consortium. Autosomal dominant hypophosphataemic rickets is associated with mutations in FGF23. Nat Genet (2000) 26(3):345–8. doi:10.1038/81664
2. Shimada T, Mizutani S, Muto T, Yoneya T, Hino R, Takeda S, et al. Cloning and characterization of FGF23 as a causative factor of tumor-induced osteomalacia. Proc Natl Acad Sci U S A (2001) 98(11):6500–5. doi:10.1073/pnas.101545198
3. Shimada T, Hasegawa H, Yamazaki Y, Muto T, Hino R, Takeuchi Y, et al. FGF-23 is a potent regulator of vitamin D metabolism and phosphate homeostasis. J Bone Miner Res (2004) 19(3):429–35. doi:10.1359/JBMR.0301264
4. Shimada T, Kakitani M, Yamazaki Y, Hasegawa H, Takeuchi Y, Fujita T, et al. Targeted ablation of Fgf23 demonstrates an essential physiological role of FGF-23 in phosphate and vitamin D metabolism. J Clin Invest (2004) 113(4):561–8. doi:10.1172/JCI19081
5. Kurosu H, Ogawa Y, Miyoshi M, Yamamoto M, Nandi A, Rosenblatt KP, et al. Regulation of fibroblast growth factor-23 signaling by klotho. J Biol Chem (2006) 281(10):6120–3. doi:10.1074/jbc.C500457200
6. Urakawa I, Yamazaki Y, Shimada T, Iijima K, Hasegawa H, Okawa K, et al. Klotho converts canonical FGF receptor into a specific receptor for FGF23. Nature (2006) 444(7120):770–4. doi:10.1038/nature05315
7. Martin A, Liu S, David V, Li H, Karydis A, Feng JQ, et al. Bone proteins PHEX and DMP1 regulate fibroblastic growth factor Fgf23 expression in osteocytes through a common pathway involving FGF receptor (FGFR) signaling. FASEB J (2011) 25(8):2551–62. doi:10.1096/fj.10-177816
8. Dalton GD, Xie J, An SW, Huang CL. New insights into the mechanisms of action of soluble Klotho. Front Endocrinol (2017) 8:323. doi:10.3389/fendo.2017.00323
9. Weber TJ, Liu S, Indridason OS, Quarles LD. Serum FGF23 levels in normal and disordered phosphorus homeostasis. J Bone Miner Res (2003) 18(7):1227–34. doi:10.1359/jbmr.2003.18.7.1227
10. Gutierrez O, Isakova T, Rhee E, Shah A, Holmes J, Collerone G, et al. Fibroblast growth factor-23 mitigates hyperphosphatemia but accentuates calcitriol deficiency in chronic kidney disease. J Am Soc Nephrol (2005) 16(7):2205–15. doi:10.1681/ASN.2005010052
11. Fukumoto S, Shimizu Y. Fibroblast growth factor 23 as a phosphotropic hormone and beyond. J Bone Miner Metab (2011) 29(5):507–14. doi:10.1007/s00774-011-0298-0
12. Yamazaki Y, Okazaki R, Shibata M, Hasegawa Y, Satoh K, Tajima T, et al. Increased circulatory level of biologically active full-length FGF-23 in patients with hypophosphatemic rickets/osteomalacia. J Clin Endocrinol Metab (2002) 87(11):4957–60. doi:10.1210/jc.2002-021105
13. Jonsson KB, Zahradnik R, Larsson T, White KE, Sugimoto T, Imanishi Y, et al. Fibroblast growth factor 23 in oncogenic osteomalacia and X-linked hypophosphatemia. N Engl J Med (2003) 348(17):1656–63. doi:10.1056/NEJMoa020881
14. Wolf M, Koch TA, Bregman DB. Effects of iron deficiency anemia and its treatment on fibroblast growth factor 23 and phosphate homeostasis in women. J Bone Miner Res (2013) 28(8):1793–803. doi:10.1002/jbmr.1923
15. Imel EA, Hui SL, Econs MJ. FGF23 concentrations vary with disease status in autosomal dominant hypophosphatemic rickets. J Bone Miner Res (2007) 22(4):520–6. doi:10.1359/jbmr.070107
16. Endo I, Fukumoto S, Ozono K, Namba N, Tanaka H, Inoue D, et al. Clinical usefulness of measurement of fibroblast growth factor 23 (FGF23) in hypophosphatemic patients: proposal of diagnostic criteria using FGF23 measurement. Bone (2008) 42(6):1235–9. doi:10.1016/j.bone.2008.02.014
17. Ruchon AF, Marcinkiewicz M, Siegfried G, Tenenhouse HS, DesGroseillers L, Crine P, et al. Pex mRNA is localized in developing mouse osteoblasts and odontoblasts. J Histochem Cytochem (1998) 46(4):459–68. doi:10.1177/002215549804600405
18. Beck L, Soumounou Y, Martel J, Krishnamurthy G, Gauthier C, Goodyer CG, et al. Pex/PEX tissue distribution and evidence for a deletion in the 3’ region of the pex gene in X-linked hypophosphatemic mice. J Clin Invest (1997) 99(6):1200–9. doi:10.1172/JCI119276
19. Liu S, Guo R, Simpson LG, Xiao ZS, Burnham CE, Quarles LD. Regulation of fibroblastic growth factor 23 expression but not degradation by PHEX. J Biol Chem (2003) 278(39):37419–26. doi:10.1074/jbc.M304544200
20. The HYP Consortium. A gene (PEX) with homologies to endopeptidases is mutated in patients with X-linked hypophosphatemic rickets. Nat Genet (1995) 11(2):130–6. doi:10.1038/ng1095-130
21. Feng JQ, Ward LM, Liu S, Lu Y, Xie Y, Yuan B, et al. Loss of DMP1 causes rickets and osteomalacia and identifies a role for osteocytes in mineral metabolism. Nat Genet (2006) 38(11):1310–5. doi:10.1038/ng1905
22. Lorenz-Depiereux B, Bastepe M, Benet-Pages A, Amyere M, Wagenstaller J, Muller-Barth U, et al. DMP1 mutations in autosomal recessive hypophosphatemia implicate a bone matrix protein in the regulation of phosphate homeostasis. Nat Genet (2006) 38(11):1248–50. doi:10.1038/ng1868
23. Levy-Litan V, Hershkovitz E, Avizov L, Leventhal N, Bercovich D, Chalifa-Caspi V, et al. Autosomal-recessive hypophosphatemic rickets is associated with an inactivation mutation in the ENPP1 gene. Am J Hum Genet (2010) 86(2):273–8. doi:10.1016/j.ajhg.2010.01.010
24. Lorenz-Depiereux B, Schnabel D, Tiosano D, Hausler G, Strom TM. Loss-of-function ENPP1 mutations cause both generalized arterial calcification of infancy and autosomal-recessive hypophosphatemic rickets. Am J Hum Genet (2010) 86(2):267–72. doi:10.1016/j.ajhg.2010.01.006
25. White KE, Cabral JM, Davis SI, Fishburn T, Evans WE, Ichikawa S, et al. Mutations that cause osteoglophonic dysplasia define novel roles for FGFR1 in bone elongation. Am J Hum Genet (2005) 76(2):361–7. doi:10.1086/427956
26. Brown WW, Juppner H, Langman CB, Price H, Farrow EG, White KE, et al. Hypophosphatemia with elevations in serum fibroblast growth factor 23 in a child with Jansen’s metaphyseal chondrodysplasia. J Clin Endocrinol Metab (2009) 94(1):17–20. doi:10.1210/jcem.94.2.9988
27. Rafaelsen SH, Raeder H, Fagerheim AK, Knappskog P, Carpenter TO, Johansson S, et al. Exome sequencing reveals FAM20c mutations associated with firboblast growth factor 23-related hypophosphatemia, dental anomalies, and ectopic calcification. J Bone Miner Res (2013) 28(6):1378–85. doi:10.1002/jbmr.1850
28. Riminucci M, Collins MT, Fedarko NS, Cherman N, Corsi A, White KE, et al. FGF-23 in fibrous dysplasia of bone and its relationship to renal phosphate wasting. J Clin Invest (2003) 112(5):683–92. doi:10.1172/JCI18399
29. Lim YH, Ovejero D, Sugaman JS, Deklotz CM, Maruri A, Eichenfield LF, et al. Multilineage somatic activating mutations in HRAS and NRAS cause mosaic cutaneous and skeletal lesions, elevated FGF23 and hypophosphatemia. Hum Mol Genet (2014) 23(2):397–407. doi:10.1093/hmg/ddt429
30. Lee JC, Su SY, Changou CA, Yang RS, Tsai KS, Collins MT, et al. Characterization of FN1-FGFR1 and novel FN1-FGF1 fusion genes in a large series of phosphaturic mesenchymal tumors. Mod Pathol (2016) 29(11):1335–46. doi:10.1038/modpathol.2016.137
31. Fukumoto S. FGF23-FGF receptor/Klotho pathway as a new drug target for disorders of bone and mineral metabolism. Calcif Tissue Int (2016) 98(4):334–40. doi:10.1007/s00223-015-0029-y
32. Simpson MA, Hsu R, Keir LS, Hao J, Sivapalan G, Ernst LM, et al. Mutations in FAM20C are associated with lethal osteosclerotic bone dysplasia (Raine syndrome), highlighting a crucial molecule in bone development. Am J Hum Genet (2007) 81(5):960–912. doi:10.1086/522240
33. Schouten BJ, Doogue MP, Soule SG, Hunt PJ. Iron polymaltose-induced FGF23 elevation complicated by hypophosphataemic osteomalacia. Ann Clin Biochem (2009) 46(Pt 2):167–9. doi:10.1258/acb.2008.008151
34. Shimizu Y, Tada Y, Yamauchi M, Okamoto T, Suzuki H, Ito N, et al. Hypophosphatemia induced by intravenous administration of saccharated ferric oxide: another form of FGF23-related hypophosphatemia. Bone (2009) 45(4):814–6. doi:10.1016/j.bone.2009.06.017
35. Wasserman H, Ikomi C, Hafberg ET, Miethke AG, Bove KE, Backeljauw PF. Two case reports of FGF23-induced hypophosphatemia in childhood biliary atresia. Pediatrics (2016) 138(2):e20154453. doi:10.1542/peds.2015-4453
36. Makitie O, Doria A, Kooh SW, Cole WG, Daneman A, Sochett E. Early treatment improves growth and biochemical and radiographic outcome in X-linked hypophosphatemic rickets. J Clin Endocrinol Metab (2003) 88(8):3591–7. doi:10.1210/jc.2003-030036
37. Carpenter TO, Imel EA, Holm IA, Jan de Beur SM, Insogna KL. A clinician’s guide to X-linked hypophosphatemia. J Bone Miner Res (2011) 26(7):1381–8. doi:10.1002/jbmr.340
38. Yamazaki Y, Tamada T, Kasai N, Urakawa I, Aono Y, Hasegawa H, et al. Anti-FGF23 neutralizing antibodies show the physiological role and structural features of FGF23. J Bone Miner Res (2008) 23(9):1509–18. doi:10.1359/jbmr.080417
39. Aono Y, Yamazaki Y, Yasutake J, Kawata T, Hasegawa H, Urakawa I, et al. Therapeutic effects of anti-FGF23 antibodies in hypophosphatemic rickets/osteomalacia. J Bone Miner Res (2009) 24(11):1879–88. doi:10.1359/jbmr.090509
40. Aono Y, Hasegawa H, Yamazaki Y, Shimada T, Fujita T, Yamashita T, et al. Anti-FGF23 neutralizing antibodies ameliorate muscle weakness and decreased spontaneous movement of hyp mice. J Bone Miner Res (2011) 26(4):803–10. doi:10.1002/jbmr.275
41. Carpenter TO, Imel EA, Ruppe MD, Weber TJ, Klausner MA, Wooddell MM, et al. Randomized trial of the anti-FGF23 antibody KRN23 in X-linked hypophosphatemia. J Clin Invest (2014) 124(4):1587–97. doi:10.1172/jci72829
42. Imel EA, Zhang X, Ruppe MD, Weber TJ, Klausner MA, Ito T, et al. Prolonged correction of serum phosphorus in adults with X-linked hypophosphatemia using monthly doses of KRN23. J Clin Endocrinol Metab (2015) 100(7):2565–73. doi:10.1210/jc.2015-1551
43. Ruppe MD, Zhang X, Imel EA, Weber TJ, Klausner MA, Ito T, et al. Effect of four monthly doses of a human monoclonal anti-FGF23 antibody (KRN23) on quality of life in X-linked hypophosphatemia. Bone Rep (2016) 13(5):158–62. doi:10.1016/j.bonr.2016.05.004
44. Xiao Z, Riccardi D, Velazquez HA, Chin AL, Yates CR, Carrick JD, et al. A computationally identified compound antagonizes excess FGF-23 signaling in renal tubules and a mouse model of hypophosphatemia. Sci Signal (2016) 9(455):ra113. doi:10.1126/scisignal.aaf5034
45. Goetz R, Nakada Y, Hu MC, Kurosu H, Wang L, Nakatani T, et al. Isolated C-terminal tail of FGF23 alleviates hypophosphatemia by inhibiting FGF23-FGFR-Klotho complex formation. Proc Natl Acad Sci U S A (2010) 107(1):407–12. doi:10.1073/pnas.0902006107
46. Johnson K, Levine K, Sergi J, Chamoun J, Roach R, Vekich J, et al. Therapeutic effects of FGF23 c-tail Fc in a murine pre-clinical model of X-linked hypophosphatemia via the selective modulation of phosphate reabsorption. J Bone Miner Res (2017) 32(10):2062–73. doi:10.1002/jbmr.3197
47. Fakhar M, Rashid S. Targeted inhibition of Klotho binding to fibroblast growth factor 23 prevents hypophosphetemia. J Mol Graph Model (2017) 75:9–19. doi:10.1016/j.jmgm.2017.1004.1024
48. Wohrle S, Bonny O, Beluch N, Gaulis S, Stamm C, Scheibler M, et al. FGF receptors control vitamin D and phosphate homeostasis by mediating renal FGF-23 signaling and regulating FGF-23 expression in bone. J Bone Miner Res (2011) 26(10):2486–97. doi:10.1002/jbmr.478
49. Wohrle S, Henninger C, Bonny O, Thuery A, Beluch N, Hynes NE, et al. Pharmacological inhibition of fibroblast growth factor (FGF) receptor signaling ameliorates FGF23-mediated hypophosphatemic rickets. J Bone Miner Res (2013) 28(4):899–911. doi:10.1002/jbmr.1810
50. Zhang MY, Ranch D, Pereira RC, Armbrecht HJ, Portale AA, Perwad F. Chronic inhibition of ERK1/2 signaling improves disordered bone and mineral metabolism in hypophosphatemic (98Hyp) mice. Endocrinology (2012) 153(4):1806–16. doi:10.1210/en.2011-1831
51. Florenzano P, Gafni RI, Collins MT. Tumor-induced osteomalacia. Bone Rep (2017) 7:90–7. doi:10.1016/j.bonr.2017.09.002
52. Isakova T, Wahl P, Vargas GS, Gutierrez OM, Scialla J, Xie H, et al. Fibroblast growth factor 23 is elevated before parathyroid hormone and phosphate in chronic kidney disease. Kidney Int (2011) 79(12):1370–8. doi:10.1038/ki.2011.47
53. Saito H, Maeda A, Ohtomo S, Hirata M, Kusano K, Kato S, et al. Circulating FGF-23 is regulated by 1alpha,25-dihydroxyvitamin D3 and phosphorus in vivo. J Biol Chem (2005) 280(4):2543–9. doi:10.1074/jbc.M408903200
54. David V, Francis C, Babitt JL. Ironing out the cross talk between FGF23 and inflammation. Am J Physiol Renal Physiol (2017) 312(1):F1–8. doi:10.1152/ajprenal.00359.2016
55. Hasegawa H, Nagano N, Urakawa I, Yamazaki Y, Iijima K, Fujita T, et al. Direct evidence for a causative role of FGF23 in the abnormal renal phosphate handling and vitamin D metabolism in rats with early-stage chronic kidney disease. Kidney Int (2010) 78(10):975–80. doi:10.1038/ki.2010.313
56. Shalhoub V, Shatzen EM, Ward SC, Davis J, Stevens J, Bi V, et al. FGF23 neutralization improves chronic kidney disease-associated hyperparathyroidism yet increases mortality. J Clin Invest (2012) 122(7):2543–53. doi:10.1172/jci61405
57. Gutierrez OM, Mannstadt M, Isakova T, Rauh-Hain JA, Tamez H, Shah A, et al. Fibroblast growth factor 23 and mortality among patients undergoing hemodialysis. N Engl J Med (2008) 359(6):584–92. doi:10.1056/NEJMoa0706130
58. Moe SM, Chertow GM, Parfrey PS, Kubo Y, Block GA, Correa-Rotter R, et al. Cinacalcet, fibroblast growth factor-23, and cardiovascular disease in hemodialysis: the evaluation of cinacalcet HCl therapy to lower cardiovascular events (EVOLVE) trial. Circulation (2015) 132(1):27–39. doi:10.1161/CIRCULATIONAHA.114.013876
59. Faul C, Amaral AP, Oskouei B, Hu MC, Sloan A, Isakova T, et al. FGF23 induces left ventricular hypertrophy. J Clin Invest (2011) 121(11):4393–408. doi:10.1172/JCI46122
Keywords: hypophosphatemia, rickets, osteomalacia, antibody, chronic kidney disease-mineral and bone disorder (CKD-MBD)
Citation: Fukumoto S (2018) Targeting Fibroblast Growth Factor 23 Signaling with Antibodies and Inhibitors, Is There a Rationale? Front. Endocrinol. 9:48. doi: 10.3389/fendo.2018.00048
Received: 29 November 2017; Accepted: 05 February 2018;
Published: 20 February 2018
Edited by:
Reinhold Gottfried Erben, Veterinärmedizinische Universität Wien, AustriaReviewed by:
Junichiro Sonoda, Genentech, Inc., United StatesCopyright: © 2018 Fukumoto. This is an open-access article distributed under the terms of the Creative Commons Attribution License (CC BY). The use, distribution or reproduction in other forums is permitted, provided the original author(s) and the copyright owner are credited and that the original publication in this journal is cited, in accordance with accepted academic practice. No use, distribution or reproduction is permitted which does not comply with these terms.
*Correspondence: Seiji Fukumoto, ZnVrdW1vdG8tdGt5QHVtaW4uYWMuanA=
Disclaimer: All claims expressed in this article are solely those of the authors and do not necessarily represent those of their affiliated organizations, or those of the publisher, the editors and the reviewers. Any product that may be evaluated in this article or claim that may be made by its manufacturer is not guaranteed or endorsed by the publisher.
Research integrity at Frontiers
Learn more about the work of our research integrity team to safeguard the quality of each article we publish.