- Brain Research Institute (BRIMS), Jeffrey Cheah School of Medicine and Health Sciences, Monash University Malaysia, Petaling Jaya, Malaysia
Reproduction is associated with the circadian system, primarily as a result of the connectivity between the biological clock in the suprachiasmatic nucleus (SCN) and reproduction-regulating brain regions, such as preoptic area (POA), anteroventral periventricular nucleus (AVPV), and arcuate nucleus (ARC). Networking of the central pacemaker to these hypothalamic brain regions is partly represented by close fiber appositions to specialized neurons, such as kisspeptin and gonadotropin-releasing hormone (GnRH) neurons; accounting for rhythmic release of gonadotropins and sex steroids. Numerous studies have attempted to dissect the neurochemical properties of GnRH neurons, which possess intrinsic oscillatory features through the presence of clock genes to regulate the pulsatile and circadian secretion. However, less attention has been given to kisspeptin, the upstream regulator of GnRH and a potent mediator of reproductive functions including puberty. Kisspeptin exerts its stimulatory effects on GnRH secretion via its cognate Kiss-1R receptor that is co-expressed on GnRH neurons. Emerging studies have found that kisspeptin neurons oscillate on a circadian basis and that these neurons also express clock genes that are thought to regulate its rhythmic activities. Based on the fiber networks between the SCN and reproductive nuclei such as the POA, AVPV, and ARC, it is suggested that interactions among the central biological clock and reproductive neurons ensure optimal reproductive functionality. Within this neuronal circuitry, kisspeptin neuronal system is likely to “time” reproduction in a long term during development and aging, in a medium term to regulate circadian or estrus cycle, and in a short term to regulate pulsatile GnRH secretion.
Introduction
Reproduction, a central feature of life, requires synergistic actions of cellular processes at the brain and the reproductive organs to achieve normal sexual functionalities. Several neuronal populations in the hypothalamus including preoptic area (POA), anteroventral periventricular nucleus (AVPV), and arcuate nucleus (ARC) play critical roles in the hypothalamic–pituitary–gonadal (HPG) axis (1–4). Importantly, POA contains gonadotropin-releasing hormone (GnRH) neurons and AVPV and ARC contain a distinct neuronal population termed kisspeptin neurons; which are fundamental in the feedback system of the HPG axis (5–9). When kisspeptin is bound to its cognate G-protein-coupled receptor (GPR) 54, commonly known as Kiss1-R, to stimulate GnRH secretion, the release of luteinizing hormone (LH) and follicle-stimulating hormone (FSH) from gonadotropes occurs (6). This process culminates in the synthesis of sex steroids at the gonads and, in concert with the action of gonadotropins, stimulates gametogenesis (10–12) (Figure 1).
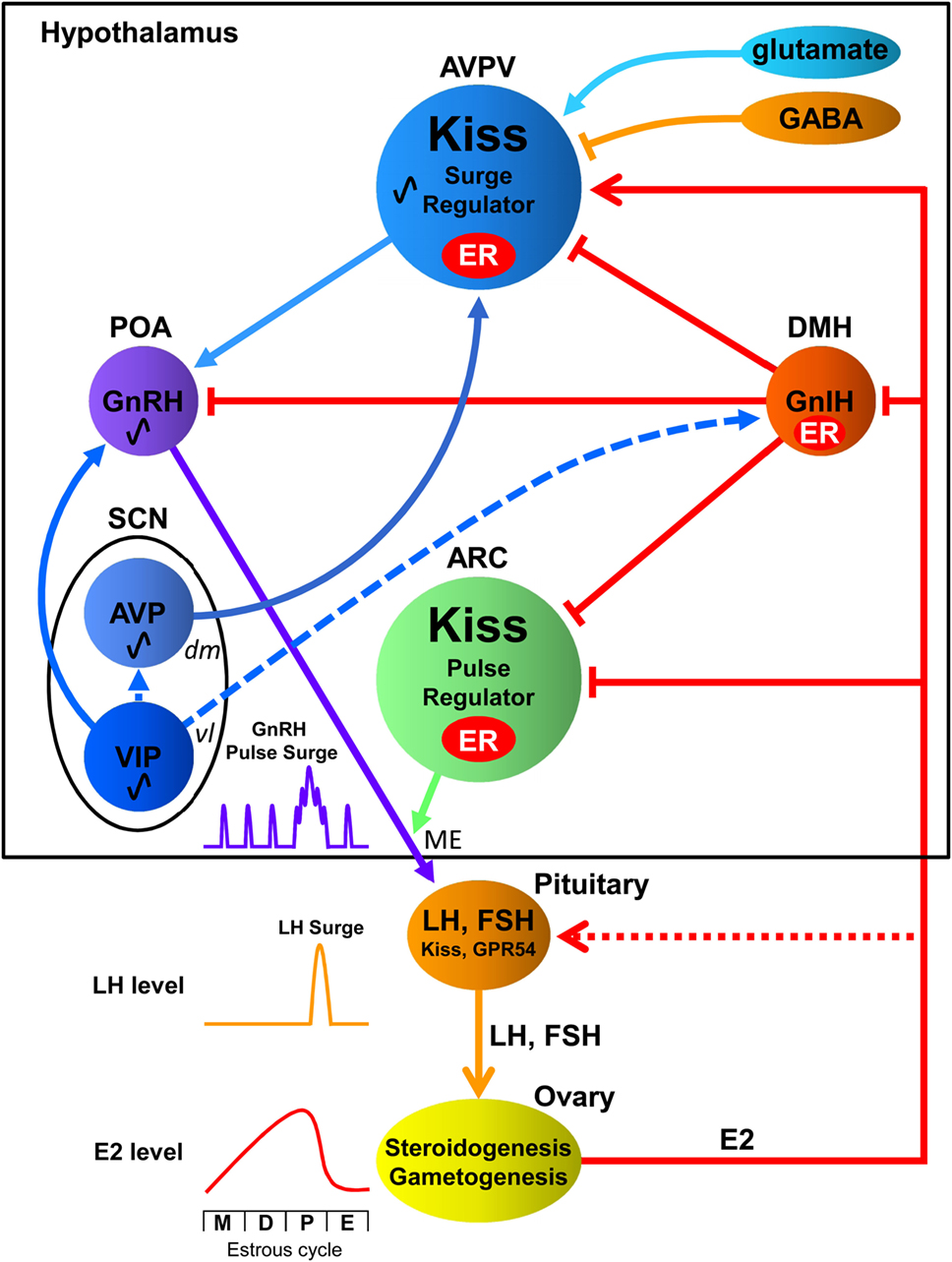
Figure 1. Interactions among the central biological clock and reproductive neurons in the hypothalamic–pituitary–gonadal axis of females. The suprachiasmatic nucleus (SCN), the central biological clock, can be divided into two major subdivisions known as the ventrolateral (vl) SCN, the core, and the dorsomedial (dm) SCN, the shell. The former contains cell bodies of vasoactive intestinal polypeptide (VIP) neurons and the latter contains cell bodies of arginine vasopressin (AVP) neurons. The vlSCN acts as the conductor of rhythmicity and transmits synchronizing cues to the dmSCN. VIP neurons project to gonadotropin-releasing hormone (GnRH) neurons in the preoptic area (POA), whereas AVP neurons project to kisspeptin (Kiss) neurons in the anteroventral periventricular nucleus (AVPV). Gonadotropin-inhibitory hormone (GnIH) neurons in the dorsomedial hypothalamus (DMH) inhibit the activity of GnRH neurons as well as kisspeptin neurons in the AVPV and arcuate nucleus (ARC). AVPV kisspeptin neuron is also regulated by stimulatory and inhibitory neurotransmitters glutamate and GABA, respectively. GnRH is released at the median eminence (ME) to stimulate luteinizing hormone (LH) and follicle-stimulating hormone (FSH) secretion from the pituitary, which stimulate steroidogenesis and gametogenesis in the ovary. Estradiol (E2) secreted from the ovary gradually increases and rapidly decreases during the estrous cycle [metestrous (M), diestrus (D), proestrus (P), and estrus (E)] in rodents. GnIH and Kiss neurons express estrogen receptor (ER) to convey hormonal information to the reproductive neuronal network. ARC Kiss neurons may function as part of the negative feedback mechanism of E2 on pulsatile GnRH release at the ME. On the other hand, AVPV Kiss neurons may function as the positive feedback mechanism of high E2 concentration on GnRH/LH surge. E2 inhibits GnIH gene expression in the DMH and Kiss gene expression in the ARC but stimulates Kiss gene expression in the AVPV. Kiss and GPR54 are reported to be expressed in gonadotropes, and they are thought to exert synergic effects with GnRH and E2 on LH release (13). Solid lines indicate direct regulation by receptors of signaling molecules, whereas dotted lines indicate possible indirect regulation.
Gonadotropin-releasing hormone neurons exhibit pulsatile and surge secretory patterns in females, with peaks occurring prior to the LH surge ensuring the temporal regulation of the HPG axis. Rhythmicity of GnRH neuronal activity and reproductive hormonal fluctuation are studied well in female rodents. During their estrous cycle (metestrous, diestrus, proestrus, and estrus), which normally cycles in 4–5 days in rats and mice, estradiol secreted from the ovary gradually increases until proestrus stage and rapidly decreases at estrus stage. Relatively low concentration of estradiol during metestrous and diestrus stages inhibits GnRH pulsatility. However, high concentration of estradiol in the afternoon of proestrus stage increases the frequency and amplitude of GnRH pulsatility resulting in GnRH/LH surge that induces ovulation from the ovary (14, 15) (Figure 1).
The HPG axis does not operate independently and is gated by multiple neurocircuitries, one of which is the circadian clock system. The term “circadian” is derived from the Latin words “circa” and “dies” referring to “around a day”; hence, the circadian clock system ensures the timely regulation of physiological and molecular processes over a 24-h cycle. The cyclic features of reproduction are the result of synchrony to the circadian system. The importance of the circadian system in the regulation of the HPG axis was clearly shown by GnRH neuron firing activity of ovariectomized mice treated with high concentration of estradiol implants (OVX + E). Increased GnRH neuron firing activity and LH surge only occurred in the late afternoon or early night in OVE + E mice (16). Reproductive behavior is also regulated by the circadian system, such as increased sexual desire evident in men during the morning phase triggered by high testosterone levels (17–20). Similarly, in rodent species, female mice appear to be more sexually receptive during the early night, a behavior associated with the circadian profile of gonadotropins and sex steroid release (21, 22).
Gonadotropin-releasing hormone neurons are webbed intricately with the biological clock system and have been studied extensively for their participation in the circadian release of gonadotropins and sex steroids (23–25). There has been a growing interest in kisspeptin neurons, the upstream regulators of GnRH neurons, which are thought to possess similar circadian components (26, 27), achieving a synchronized operating mechanism in the HPG axis. Kisspeptin neurons are not inherently localized in high numbers in reproduction-related areas of the hypothalamus; they undergo a process of developmental maturation to attain their adult numbers and activity profile (28–30). Kisspeptin neuronal system is likely to “time” reproduction during development and aging by regulating circadian or estrus reproductive cycle as well as pulsatile GnRH/LH secretion.
Neurochemical Properties of Kisspeptin
Kisspeptin belongs to the RF-amide peptide family. The propeptide, consisting of 145 amino acids is encoded by the Kiss1 gene (31), which upon cleavage by the convertase enzyme, furin, generates the active form of kisspeptin, Kp54 (32). Shorter peptides such as Kp10, Kp13, and Kp14 are found in circulating levels in the placenta and result from the fragmentation of the unstable Kp54 (33, 34). Kisspeptin neurons adopt different functional roles in the AVPV and ARC as depicted by their opposing estrogenic response to ensure the inclusion of both a positive and negative feedback loop within the HPG axis, respectively (7, 35) (Figure 1).
Kisspeptin neurons in the AVPV are estrogen-sensitive, co-expressing high percentage of ERα type receptors (approx. 99% in rodents) and lower percentage of ERβ type receptors (approx. 31%) (7). Steroid-dependent activation of kisspeptin via ERα are imperative for the positive feedback response as depicted by the increased number of cells expressing Kiss1 mRNA following estradiol treatment in intact female mice, and the lack of shift in firing pattern by estradiol in ERα knockout mice (36–38) (Figure 1). Peptides such as arginine vasopressin (AVP) (39) and gonadotropin-inhibitory hormone (GnIH) (40) and neurotransmitters including glutamate and gamma-aminobutyric acid (GABA) (41, 42) also regulate AVPV kisspeptin neurons (Figure 1). It was also reported that AVPV kisspeptin neurons co-express galanin (43–45) and dopamine (46).
As opposed to the AVPV, kisspeptin neurons in the ARC are sexually differentiated during the prepubertal stages and achieve stability both in terms of Kiss1 expression and cell number during adulthood; latter attributed to the prevailing steroidal environment (47, 48). Furthermore, they co-express ERα, ERβ, and androgen receptors (7, 8), such that castrated/ovariectomized rodents exhibit increased Kiss1 expression levels, which are suppressed upon exposure to testosterone and estrogen (7, 8) (Figure 1). Kisspeptin neurons also respond to GnIH (49) via GPR147 receptors (50, 51) (Figure 1).
The Role of Kisspeptin in Reproduction
Parhar et al. were the first to elucidate the association between kisspeptin and GnRH, and the role of kisspeptin within the reproductive systems of non-mammalian vertebrates (52). Subsequently, similar findings were reported in mammalian species, including mice (53) and rats (6). Distribution studies demonstrated the presence of Kiss1-R in hypothalamic regions engaged in reproduction, such as the POA, ARC, and dorsomedial hypothalamus (DMH) (54, 55). The concentration of Kiss1-R in the POA was found to colocalize with GnRH neurons, with kisspeptin fiber projections evident in 40 and 10% of GnRH neurons in the POA of adult female and male mice, respectively (28, 52). Furthermore, the in vivo administration of kisspeptin triggers an acute release of GnRH, an effect that is abrogated in Kiss1-R knockout mice (56).
Loss of function of kisspeptin is detrimental to the HPG axis. Kiss1/Kiss1-R gene mutations are characterized by general infertility and cause abnormal gonadal development, delayed sexual maturation, and aberrant hormone secretion (57–59). Similar sexual irregularities and decreased serum gonadotropin levels are evident in GnRH-specific Kiss1-R knockout mice (60), endorsing kisspeptin as the prime effector of GnRH.
Developmental Timing of Kisspeptin – A Checkpoint for Reproductive Functionality
The kisspeptinergic system develops dynamically throughout the mammalian lifespan until the start of the aging process. The maturation of the kisspeptin system begins with an increase in kisspeptin neurons from peripubertal stages in C57BL/6J mice (28) (Table 1). Kisspeptin cells are absent on postnatal day 10 (P10) and rapidly increase in number from P25 (28, 29). This increase in the neuronal population during development occurs concomitantly with both the formation of kisspeptin fiber appositions to GnRH neurons and Kiss1-R mRNA expression in GnRH neurons (28, 61) (Table 1). Connectivity between kisspeptin and GnRH neurons is barely noticeable before PN25 and slowly increases until puberty (28). The small number of kisspeptin cells evident during the prepubertal stage suggests that the kisspeptinergic system is “not yet-functional” and immature, an idea supported by the fact that during the same period GnRH neuronal activity is regulated by glutamatergic and GABAergic inputs (62).
Puberty is a fundamental stage, during which the kisspeptin system achieves its full functionality. Kisspeptin expression increases exponentially from the time of puberty until adulthood (30). A sevenfold increase in the number of Kiss1 mRNA-expressing cells in AVPV of male mice is evident during the transition from puberty to adulthood, a pattern consistent with a fivefold increase in the number of kisspeptin expressing cells from PN25 to adulthood (64). However, these changes are exclusive to AVPV: the number of Kiss1 mRNA-expressing cells remains unchanged in ARC (64). Evidence also exists to support the increased responsiveness of GnRH neurons to kisspeptin: 44% GnRH neuronal activity upon exposure to kisspeptin is evident during puberty, whereas 90% is evident during adulthood in male mice (28, 64). Interestingly, the pulsatile secretion of GnRH changes from one pulse every 90 min to one pulse every 30 min from the early postnatal to pubertal stages in male rats (65). The stability in the frequency of GnRH release marks the maturation of the HPG axis and suggests the onset of an active kisspeptin–GnRH stimulatory mechanism, which is required for a preovulatory GnRH/LH surge in females (66, 67).
Aging is a process that entails numerous degenerative processes and affects reproductive traits. In female mice, although the number of kisspeptin neurons remains unaffected during the aging process, specific cellular changes occur, including:
• decreased numbers of ERα-positive kisspeptin neurons,
• decreased kisspeptin neuronal activity at the time of LH surge, and
• morphological cellular changes in kisspeptin neurons featuring irregular shapes and atypical nucleus/cytoplasm (63) (Table 1).
A study by Ishii et al. showed that Kiss1 mRNA and peptide expression in AVPV remains unchanged in middle-aged rats compared with young rats (68). The findings suggest that age-related reproductive neuroendocrine deficiencies originate from a loss of response of kisspeptin neurons in AVPV to estrogenic signals, therefore altering its secretory pattern and disrupting stimulation of the HPG axis (69). Aged rats (18–21 months old) exhibit reduced LH and FSH secretion, which coincides with decreased GnRH mRNA expression in the POA and reduced GnRH fiber projections to the median eminence (ME) (70–72).
Integration of Rhythmicity within the Hypothalamic–Pituitary–Gonadal Axis – “Cycling” Reproduction
Several brain circuits converge to maintain the timely activation of the HPG axis: this maintenance is achieved by rhythmic cues originating mainly from the central biological clock located in the suprachiasmatic nucleus (SCN) (73, 74). The SCN is anatomically structured into a core and a shell, termed the ventrolateral (vl) and dorsomedial (dm) SCN, demarcated by neuropeptide composition (75). Vasoactive intestinal peptide (VIP) is the main neuropeptide released by most neurons of the vlSCN, which account for 10% of the total SCN, whereas neurons synthesizing AVP are localized in the dmSCN (76, 77). The vlSCN acts as the conductor of rhythmicity and transmits synchronizing cues to the dmSCN, which in turn amplifies the signal and conveys it to slave oscillators present in other brain regions and cellular entities (78) (Figure 1).
Among different hypothalamic nuclei of the HPG axis, the POA is densely innervated by VIP-ir fibers, with a subpopulation of GnRH neurons (±40%) expressing the cognate receptors for VIP, VPAC2/VIP2 (79) (Figure 1). This connectivity is further supported by the decrease in VIP-ergic innervations to the POA following lesions of VIP-positive neurons in the SCN (80). Importantly, the connectivity between the SCN to GnRH neurons is strengthened with an increase in VIP contacts to GnRH cells from prepubertal stages to adulthood, suggesting a circadian clock-aided maturation of the reproductive axis with the generation of estrous cyclicity and hormonal rhythms (81). Signals from the SCN are relayed to the AVPV through AVP fiber projections, as substantiated by anterograde tracing from the central clock (82). Studies have demonstrated expression of AVP-specific receptor, V1a, in kisspeptin neurons, and manipulation of AVP content in the brain elicits a time-dependent response of kisspeptin neurons (39) (Figure 1).
The DMH is also an important brain region for reproductive functionality, given its high content of GnIH neurons and co-expression of GPR147 receptors, specific to the GnIH peptide, in both kisspeptin and GnRH neurons (40, 50, 83–85) (Figure 1). The DMH receives extensive fiber projections from both regions of the SCN, although the majority of the fibers originate from the vlSCN (86). Exogenous administration of VIP triggers a decrease in GnIH cellular activity that is confined to the evening, mediating its time-specific modulation (87). Nonetheless, GnIH neurons do not co-express VIP receptors (87), suggesting that its neuronal activity is regulated by the circadian clock via alternate pathways, such as interneurons (Figure 1). On the other hand, it was recently demonstrated that GnIH inhibits VIP signaling in GnRH neuronal cell line, GT1-7, and inhibits VIP induced GnRH release from hypothalamic culture of female mice (88). Accordingly, GnIH neurons may modulate the activity of GnRH neurons in parallel with VIP, possibly to translate endogenous hormonal signals [estradiol: (89, 90) (Figure 1); melatonin: (84); glucocorticoid: (91, 92)]. Based on the fiber networks between the SCN and reproductive nuclei such as the POA, AVPV, ARC, and DMH, it is suggested that interactions among the central biological clock and reproductive neurons ensure optimal reproductive functionality (Figure 1).
Disruption of the Circadian System and Its Effects on Reproductive Functionality
It is generally accepted that ablation of the SCN leads to reproductive incompetency by affecting subparts of the HPG axis such as interfering with diurnal variation of reproductive hormones (93). Females are more prone to this ablation as reported by a loss of ovulation, disruption of estrous cyclicity with desynchronized LH surge, and vaginal cornification induced by acyclic prolactin levels (94–96).
Circadian rhythmicity of SCN neurons is maintained by the transcriptional auto-regulatory loop between the clock genes and their products. Clock genes, such as Period 1 (Per1) and Period 2 (Per2), are transcriptionally activated by photic signals (97–99) and regulate the expression of Brain and muscle ARNT-like 1 (Bmal1), which dimerizes with Circadian locomotor output cycle kaput (Clock) to enhance circadian transcriptional activity (100, 101). Additionally, Per and cryptochrome genes (Cry) are involved in the negative limb of the clock system by repressing CLOCK:BMAL1-induced transcriptional activity (102–104). Repression of the circadian machinery is also undertaken by genes such as Rev-Erbα (also known as Nuclear receptor subfamily 1, group D, member 1) (105, 106) and Glycogen synthase kinase 3β (107).
Global mutations, as listed in Table 2, generate stronger reproductive deficiencies as opposed to site- or neuron-specific gene alterations. Clock mutant female mice do not exhibit LH surges and normal estrous cyclicity (96). Bmal1 knockout male mice exhibit lowered testosterone levels, accompanied by high serum LH concentrations (108). These results also indicate that the effects of clock-gene mutations on the HPG axis differ between the sexes, as suggested by gonadal and sex chromosome-dependent differences in the circadian system (109).
Rhythmicity of GnRH Neuronal Activity
Clock genes, such as Bmal1, Per1 and Per2, exhibit rhythmic mRNA expression synchronous with oscillations in GnRH levels in GT1-7 cell line (117, 118). The intrinsic circadian molecular machinery of GnRH neurons is responsible for the mode of GnRH secretion, because a mutation in the Clock gene results in significant decrease of GnRH pulse frequency. On the other hand, overexpression of Cry gene increases GnRH pulse amplitude without changing pulse frequency (117). An in vivo study showed the sub-fertile attributes of GnRH-specific Bmal1 knockout mice, characterized by irregular LH secretion while retaining normal reproductive processes, including estrous cycle (119). These results suggest that although GnRH neurons have intrinsic molecular timing machinery, GnRH neurons have to be properly regulated by other neurons to achieve regular GnRH/LH secretion.
The circadian regulation of GnRH neurons is two-tiered, because they also receive VIP-ergic afferents from the vlSCN and possess VPAC2 receptor (79). GnRH neuronal firing is activated in OVX + E mice by VIP during surge onset, but not in OVX mice. Administration of VIP receptor antagonist during surge peak decreases GnRH neuronal activity (120). An in vitro study using GT1-7 cells showed that GnRH neurons exhibit daily changes in GnRH levels and secretory patterns following stimulatory cues from VIP and kisspeptin (121). These results suggest that VIP can directly regulate circadian rhythm of GnRH neurons (Figure 1).
Gonadotropin-releasing hormone/luteinizing hormone release is also regulated by AVP. The circadian GnRH release coincided with circadian AVP release in coculture experiment of female rat POA and SCN (122). Administration of AVP into the POA of SCN lesioned female rat induced surge-like LH release (123). Interestingly, the effect of AVP administration to the POA in SCN-intact female rat was time-dependent. When AVP was administered during the second half of the light period, LH surge was induced in 30% of the animals; however, AVP had no effect when it was administered during the first half of the light period, indicating that AVP is part of the circadian regulatory machinery of LH surge (124). Intracerebroventricular (icv) injection of AVP on the afternoon of proestrus also induced LH surge in Clock mutant mice (125). Because AVP has no direct projection on GnRH neurons, these effect of AVP on GnRH/LH release may be mediated by AVPV kisspeptin neurons because SCN sends AVP fiber projections to AVPV and AVPV kisspeptin neurons express AVP-specific receptor, V1a (39, 82) (Figure 1). Effect of AVP on GnRH or LH release shown in in vitro (122) and in vivo (123) may also have been mediated by AVPV kisspeptin neurons because AVPV and POA regions are located closely in the brain.
Rhythmicity of AVPV Kisspeptin Neuronal Activity
Kisspeptin mRNA in the AVPV peaks during the evening of proestrus in female rats, whereas kisspeptin mRNA in the ARC does not (126). AVPV kisspeptin neurons display rhythmic characteristics in ovariectomized female mice administered with constant estradiol, with peak expression occurring during late subjective day coincident with LH release. Kiss1 rhythmicity only occurs in the presence of steroidal milieu: gonadectomised females are devoid of Kiss1 rhythms, paralleled by a lack of LH rhythmicity (26). The circadian increase in Kiss1 expression in the AVPV and the activation of GnRH cells were further shown to be dependent on ipsilateral neural input from the SCN (127).
A recent study showed the presence of circadian expression of Per1 in AVPV kisspeptin neurons. Interestingly, Per1 rhythm in the AVPV was estradiol-dependent (27). Noradrenaline (NA) is one of the modulators of GnRH release, and NA fiber terminals exist in close apposition to AVPV kisspeptin neurons. The use of Prazosin, an α1-adrenergic blocker, altered Kiss1 mRNA expression and Kiss1 contents, associated with the disruption in Clock and Bmal1 expression in the POA (128), providing further evidence to the circadian regulation of kisspeptin signaling by clock genes.
Another trait endorsing rhythmicity of the kisspeptinergic system is the cyclic expression of Kiss1-R by GnRH neurons, which is dependent on an elevated steroidal environment, explaining its oscillating levels prior to the LH surge (129). This receptor expression pattern occupies a prime role in regulating the sensitivity of GnRH neurons to kisspeptin (39, 129), contributing to its cyclic secretion profile.
Rhythmicity of ARC Kisspeptin Neuronal Activity
Ovariectomized rats with subcutaneous estradiol capsules were administered with kisspeptin or kisspeptin antagonist via bilateral intra-ARC or intra-POA cannulae or icv cannulae, and blood samples were collected for LH measurement via intravenous catheters. Administration of kisspeptin resulted in a dose-dependent increase in LH release. Although icv and intra-ARC administration of kisspeptin antagonist profoundly attenuated LH pulse frequency, intra-POA administration of kisspeptin antagonist did not affect pulsatile LH secretion (130). Dense kisspeptin fibers from ARC terminate at GnRH axons in the ME (44, 131, 132) (Figure 1). Accordingly, ARC kisspeptin may stimulate the frequency of pulsatile release of GnRH in the ME.
Kisspeptin neurons of ARC are referred to as KNDy neurons, because of their unique co-expression with neurokinin B (NKB) and dynorphin (Dyn) (133). The cellular activity of KNDy neurons is induced and repressed by NKB and Dyn, respectively, and ARC also possesses a subpopulation of NKB neurons that are not kisspeptin related and mediate direct actions on GnRH secretion (134). Central administration of Dyn inhibited multiple-unit activity (MUA) in the medial basal hypothalamus and pulsatile LH secretion, whereas NKB induced MUA and pulsatile LH secretion (135). These results suggest that ARC kisspeptin neurons regulate pulsatile GnRH/LH secretion acting with NKB and Dyn in the ARC.
Concluding Remarks
It is thought that the generation of oscillations within cellular entities requires coordinated inputs from the SCN as well as an intrinsic circadian machinery (136, 137). As reviewed above, the cyclic reproductive functions also rely on regulatory cues originating from the SCN. Kisspeptin neurons exhibit their “timed” actions from their maturation to regulate GnRH/LH pulse and surge. These characteristic features of kisspeptin neuronal activity are in line with the influence of the central biological clock in imparting rhythmic cues to slave oscillators present in individual cells, and its coordinated entrainment of other elements of the HPG axis to ensure normal reproductive functions. It was shown that disruption of clock genes in GnRH neurons modifies the frequency and amplitude of GnRH pulse. Given the dearth of information on the role of circadian genes in the regulation of kisspeptin neurons, site-directed clock gene mutation study is imperative to understand the role of its intrinsic oscillatory mechanism in reproduction. It is also important to study how estradiol drives the oscillatory mechanism of kisspeptin neurons in females.
Author Contributions
All authors listed, have made substantial, direct and intellectual contribution to the work, and approved it for publication.
Conflict of Interest Statement
The authors declare that the research was conducted in the absence of any commercial or financial relationships that could be construed as a potential conflict of interest.
References
1. Seeburg PH, Mason AJ, Stewart TA, Nikolics K. The mammalian GnRH gene and its pivotal role in reproduction. Recent Prog Horm Res (1987) 43:69–98.
2. Gore AC. Gonadotropin-releasing hormone (GnRH) neurons: gene expression and neuroanatomical studies. In: Ishwar SP, editor. Progress in Brain Research. Amsterdam: Elsevier (2002). p. 193–208.
3. Simerly RB. Wired for reproduction: organization and development of sexually dimorphic circuits in the mammalian forebrain. Annu Rev Neurosci (2002) 25:507–36. doi:10.1146/annurev.neuro.25.112701.142745
4. Gill JC, Wang O, Kakar S, Martinelli E, Carroll RS, Kaiser UB. Reproductive hormone-dependent and -independent contributions to developmental changes in kisspeptin in GnRH-deficient hypogonadal mice. PLoS One (2010) 5:e11911. doi:10.1371/journal.pone.0011911
5. Gottsch ML, Cunningham MJ, Smith JT, Popa SM, Acohido BV, Crowley WF, et al. A role for kisspeptins in the regulation of gonadotropin secretion in the mouse. Endocrinology (2004) 145:4073–7. doi:10.1210/en.2004-0431
6. Irwig MS, Fraley GS, Smith JT, Acohido BV, Popa SM, Cunningham MJ, et al. Kisspeptin activation of gonadotropin releasing hormone neurons and regulation of KiSS-1 mRNA in the male rat. Neuroendocrinology (2004) 80:264–72. doi:10.1159/000083140
7. Smith JT, Cunningham MJ, Rissman EF, Clifton DK, Steiner RA. Regulation of Kiss1 gene expression in the brain of the female mouse. Endocrinology (2005) 146:3686–92. doi:10.1210/en.2005-0488
8. Smith JT, Dungan HM, Stoll EA, Gottsch ML, Braun RE, Eacker SM, et al. Differential regulation of KiSS-1 mRNA expression by sex steroids in the brain of the male mouse. Endocrinology (2005) 146:2976–84. doi:10.1210/en.2005-0323
9. Smith JT, Clifton DK, Steiner RA. Regulation of the neuroendocrine reproductive axis by kisspeptin-GPR54 signaling. Reproduction (2006) 131:623–30. doi:10.1530/rep.1.00368
10. Amory JK, Bremner W. Endocrine regulation of testicular function in men: implications for contraceptive development. Mol Cell Endocrinol (2001) 182:175–9. doi:10.1016/S0303-7207(01)00562-7
11. Counis R, Laverriere JN, Garrel G, Bleux C, Cohen-Tannoudji J, Lerrant Y, et al. Gonadotropin-releasing hormone and the control of gonadotrope function. Reprod Nutr Dev (2005) 45:243–54. doi:10.1051/rnd:2005017
12. Kaiser UB. Chapter 7 – gonadotropin hormones. In: Shlomo M, editor. The Pituitary. Third ed. San Diego: Academic Press (2011). p. 205–60.
13. Richard N, Corvaisier S, Camacho E, Kottler ML. KiSS-1 and GPR54 at the pituitary level: overview and recent insights. Peptides (2009) 30:123–9. doi:10.1016/j.peptides.2008.09.015
14. Herbison AE. Multimodal influence of estrogen upon gonadotropin-releasing hormone neurons. Endocr Rev (1998) 19:302–30. doi:10.1210/edrv.19.3.0332
15. Miller BH, Takahashi JS. Central circadian control of female reproductive function. Front Endocrinol (2014) 4:195. doi:10.3389/fendo.2013.00195
16. Christian CA, Mobley JL, Moenter SM. Diurnal and estradiol-dependent changes in gonadotropin-releasing hormone neuron firing activity. Proc Natl Acad Sci U S A (2005) 102:15682–7. doi:10.1073/pnas.0504270102
17. Resko JA, Eik-nes KB. Diurnal testosterone levels in peripheral plasma of human male subjects. J Clin Endocrinol Metab (1966) 26:573–6. doi:10.1210/jcem-26-5-573
18. Bremner WJ, Vitiello MV, Prinz PN. Loss of circadian rhythmicity in blood testosterone levels with aging in normal men. J Clin Endocrinol Metab (1983) 56:1278–81. doi:10.1210/jcem-56-6-1278
19. Andersen ML, Alvarenga TF, Mazaro-Costa R, Hachul HC, Tufik S. The association of testosterone, sleep, and sexual function in men and women. Brain Res (2011) 1416:80–104. doi:10.1016/j.brainres.2011.07.060
20. Wilson MJ, McMillin JM, Seal US, Ahmed K. Circadian variation of serum testosterone in the adult male rat with a late morning acrophase. Experientia (1976) 32:944–5. doi:10.1007/BF02003784
22. Colvin GB, Whitmoyer DI, Lisk RD, Walter DO, Sawyer CH. Changes in sleep-wakefulness in female rats during circadian and estrous cycles. Brain Res (1968) 7:173–81. doi:10.1016/0006-8993(68)90095-4
23. Carmel PW, Araki S, Ferin M. Pituitary stalk portal blood collection in rhesus monkeys: evidence for pulsatile release of gonadotropin-releasing hormone (GnRH). Endocrinology (1976) 99:243–8. doi:10.1210/endo-99-1-243
24. Belchetz PE, Plant TM, Nakai Y, Keogh EJ, Knobil E. Hypophysial responses to continuous and intermittent delivery of hypopthalamic gonadotropin-releasing hormone. Science (1978) 202:631–3. doi:10.1126/science.100883
25. Funabashi T, Mitsushima D, Nakamura TJ, Uemura T, Hirahara F, Shinohara K, et al. Gonadotropin-releasing hormone (GnRH) surge generator in female rats. Prog Brain Res (2002) 141:165–73. doi:10.1016/S0079-6123(02)41091-6
26. Robertson JL, Clifton DK, de la Iglesia HO, Steiner RA, Kauffman AS. Circadian regulation of Kiss1 neurons: implications for timing the preovulatory gonadotropin-releasing hormone/luteinizing hormone surge. Endocrinology (2009) 150:3664–71. doi:10.1210/en.2009-0247
27. Chassard D, Bur I, Poirel VJ, Mendoza J, Simonneaux V. Evidence for a putative circadian kiss-clock in the hypothalamic AVPV in female mice. Endocrinology (2015) 156(8):2999–3011. doi:10.1210/en.2014-1769
28. Clarkson J, Herbison AE. Postnatal development of kisspeptin neurons in mouse hypothalamus; sexual dimorphism and projections to gonadotropin-releasing hormone neurons. Endocrinology (2006) 147:5817–25. doi:10.1210/en.2006-0787
29. Clarkson J, Boon WC, Simpson ER, Herbison AE. Postnatal development of an estradiol-kisspeptin positive feedback mechanism implicated in puberty onset. Endocrinology (2009) 150:3214–20. doi:10.1210/en.2008-1733
30. Clarkson J, Han SK, Liu X, Lee K, Herbison AE. Neurobiological mechanisms underlying kisspeptin activation of gonadotropin-releasing hormone (GnRH) neurons at puberty. Mol Cell Endocrinol (2010) 324:45–50. doi:10.1016/j.mce.2010.01.026
31. Kotani M, Detheux M, Vandenbogaerde A, Communi D, Vanderwinden J-M, Le Poul E, et al. The metastasis suppressor gene KiSS-1 encodes kisspeptins, the natural ligands of the orphan G protein-coupled receptor GPR54. J Biol Chem (2001) 276:34631–6. doi:10.1074/jbc.M104847200
32. Harihar S, Pounds KM, Iwakuma T, Seidah NG, Welch DR. Furin is the major proprotein convertase required for KISS1-to-kisspeptin processing. PLoS One (2014) 9:e84958. doi:10.1371/journal.pone.0084958
33. Bilban M, Ghaffari-Tabrizi N, Hintermann E, Bauer S, Molzer S, Zoratti C, et al. Kisspeptin-10, a KiSS-1/metastin-derived decapeptide, is a physiological invasion inhibitor of primary human trophoblasts. J Cell Sci (2004) 117:1319–28. doi:10.1242/jcs.00971
34. Oakley AE, Clifton DK, Steiner RA. Kisspeptin signaling in the brain. Endocr Rev (2009) 30:713–43. doi:10.1210/er.2009-0005
35. Tomikawa J, Uenoyama Y, Ozawa M, Fukanuma T, Takase K, Goto T, et al. Epigenetic regulation of Kiss1 gene expression mediating estrogen-positive feedback action in the mouse brain. Proc Natl Acad Sci U S A (2012) 109:E1294–301. doi:10.1073/pnas.1114245109
36. Gottsch ML, Navarro VM, Zhao Z, Glidewell-Kenney C, Weiss J, Jameson JL, et al. Regulation of Kiss1 and dynorphin gene expression in the murine brain by classical and nonclassical estrogen receptor pathways. J Neurosci (2009) 29:9390–5. doi:10.1523/JNEUROSCI.0763-09.2009
37. Frazão R, Cravo RM, Donato J, Ratra DV, Clegg DJ, Elmquist JK, et al. Shift in Kiss1 cell activity requires estrogen receptor α. J Neurosci (2013) 33:2807–20. doi:10.1523/JNEUROSCI.1610-12.2013
38. Dubois SL, Acosta-Martínez M, DeJoseph MR, Wolfe A, Radovick S, Boehm U, et al. Positive, but not negative feedback actions of estradiol in adult female mice require estrogen receptor α in kisspeptin neurons. Endocrinology (2015) 156:1111–20. doi:10.1210/en.2014-1851
39. Williams WP III, Jarjisian SG, Mikkelsen JD, Kriegsfeld LJ. Circadian control of kisspeptin and a gated GnRH response mediate the preovulatory luteinizing hormone surge. Endocrinology (2011) 152:595–606. doi:10.1210/en.2010-0943
40. Rizwan MZ, Poling MC, Corr M, Cornes PA, Augustine RA, Quennell JH, et al. RFamide-related peptide-3 receptor gene expression in GnRH and kisspeptin neurons and GnRH-dependent mechanism of action. Endocrinology (2012) 153:3770–9. doi:10.1210/en.2012-1133
41. Ducret E, Gaidamaka G, Herbison AE. Electrical and morphological characteristics of anteroventral periventricular nucleus kisspeptin and other neurons in the female mouse. Endocrinology (2010) 151:2223–32. doi:10.1210/en.2009-1480
42. Ottem EN, Godwin JG, Krishnan S, Petersen SL. Dual-phenotype GABA/glutamate neurons in adult preoptic area: sexual dimorphism and function. J Neurosci (2004) 24:8097–105. doi:10.1523/JNEUROSCI.2267-04.2004
43. Porteous R, Petersen SL, Yeo SH, Bhattarai JP, Ciofi P, D’anglemont de Tassigny X, et al. Kisspeptin neurons co-express met-enkephalin and galanin in the rostral periventricular region of the female mouse hypothalamus. J Comp Neurol (2011) 519:3456–69. doi:10.1002/cne.22716
44. Kallo I, Vida B, Deli L, Molnar C, Hrabovszky E, Caraty A, et al. Co-localisation of kisspeptin with galanin or neurokinin B in afferents to mouse GnRH neurones. J Neuroendocrinol (2012) 24:464–76. doi:10.1111/j.1365-2826.2011.02262.x
45. Skrapits K, Borsay BA, Herczeg L, Ciofi P, Liposits Z, Hrabovszky E. Neuropeptide co-expression in hypothalamic kisspeptin neurons of laboratory animals and the human. Front Neurosci (2015) 9:29. doi:10.3389/fnins.2015.00029
46. Clarkson J, Herbison A. Dual phenotype kisspeptin-dopamine neurones of the rostral periventricular area of the third ventricle project to gonadotrophin-releasing hormone neurones. J Neuroendocrinol (2011) 23:293–301. doi:10.1111/j.1365-2826.2011.02107.x
47. Kauffman AS, Gottsch ML, Roa J, Byquist AC, Crown A, Clifton DK, et al. Sexual differentiation of Kiss1 gene expression in the brain of the rat. Endocrinology (2007) 148:1774–83. doi:10.1210/en.2006-1540
48. Kauffman AS, Navarro VM, Kim J, Clifton DK, Steiner RA. Sex differences in the regulation of Kiss1/NKB neurons in juvenile mice: implications for the timing of puberty. Am J Physiol Endocrinol Metab (2009) 297:E1212–21. doi:10.1152/ajpendo.00461.2009
49. Ancel C, Bentsen AH, Sebert ME, Tena-Sempere M, Mikkelsen JD, Simonneaux V. Stimulatory effect of RFRP-3 on the gonadotrophic axis in the male Syrian hamster: the exception proves the rule. Endocrinology (2012) 153:1352–63. doi:10.1210/en.2011-1622
50. Poling MC, Quennell JH, Anderson GM, Kauffman AS. Kisspeptin neurones do not directly signal to RFRP-3 neurones but RFRP-3 may directly modulate a subset of hypothalamic kisspeptin cells in mice. J Neuroendocrinol (2013) 25:876–86. doi:10.1111/jne.12084
51. Simonneaux V, Ancel C, Poirel VJ, Gauer F. Kisspeptins and RFRP-3 act in concert to synchronize rodent reproduction with seasons. Front Neurosci (2013) 7:22. doi:10.3389/fnins.2013.00022
52. Parhar IS, Ogawa S, Sakuma Y. Laser-captured single digoxigenin-labeled neurons of gonadotropin-releasing hormone types reveal a novel G protein-coupled receptor (Gpr54) during maturation in cichlid fish. Endocrinology (2004) 145:3613–8. doi:10.1210/en.2004-0395
53. Dungan HM, Gottsch ML, Zeng H, Gragerov A, Bergmann JE, Vassilatis DK, et al. The role of kisspeptin-GPR54 signaling in the tonic regulation and surge release of gonadotropin-releasing hormone/luteinizing hormone. J Neurosci (2007) 27:12088–95. doi:10.1523/JNEUROSCI.2748-07.2007
54. Lee DK, Nguyen T, O’Neill GP, Cheng R, Liu Y, Howard AD, et al. Discovery of a receptor related to the galanin receptors. FEBS Lett (1999) 446:103–7. doi:10.1016/S0014-5793(99)00009-5
55. Mikkelsen JD, Simonneaux V. The neuroanatomy of the kisspeptin system in the mammalian brain. Peptides (2009) 30:26–33. doi:10.1016/j.peptides.2008.09.004
56. Messager S, Chatzidaki EE, Ma D, Hendrick AG, Zahn D, Dixon J, et al. Kisspeptin directly stimulates gonadotropin-releasing hormone release via G protein-coupled receptor 54. Proc Natl Acad Sci U S A (2005) 102:1761–6. doi:10.1073/pnas.0409330102
57. Seminara SB, Messager S, Chatzidaki EE, Thresher RR, Acierno JS Jr, Shagoury JK, et al. The GPR54 gene as a regulator of puberty. N Engl J Med (2003) 349:1614–27. doi:10.1056/NEJMoa035322
58. d’Anglemont de Tassigny X, Fagg LA, Dixon JP, Day K, Leitch HG, Hendrick AG, et al. Hypogonadotropic hypogonadism in mice lacking a functional Kiss1 gene. Proc Natl Acad Sci U S A (2007) 104:10714–9. doi:10.1073/pnas.0704114104
59. Lapatto R, Pallais JC, Zhang D, Chan Y-M, Mahan A, Cerrato F, et al. Kiss1−/− mice exhibit more variable hypogonadism than Gpr54−/− mice. Endocrinology (2007) 148:4927–36. doi:10.1210/en.2007-0078
60. Novaira HJ, Sonko ML, Hoffman G, Koo Y, Ko C, Wolfe A, et al. Disrupted kisspeptin signaling in GnRH neurons leads to hypogonadotrophic hypogonadism. Mol Endocrinol (2014) 28:225–38. doi:10.1210/me.2013-1319
61. Herbison AE, de Tassigny X, Doran J, Colledge WH. Distribution and postnatal development of Gpr54 gene expression in mouse brain and gonadotropin-releasing hormone neurons. Endocrinology (2010) 151:312–21. doi:10.1210/en.2009-0552
62. Tobet SA, Bless EP, Schwarting GA. Developmental aspect of the gonadotropin-releasing hormone system. Mol Cell Endocrinol (2001) 185:173–84. doi:10.1016/S0303-7207(01)00616-5
63. Zhang J, Yang L, Lin N, Pan X, Zhu Y, Chen X. Aging-related changes in RP3V kisspeptin neurons predate the reduced activation of GnRH neurons during the early reproductive decline in female mice. Neurobiol Aging (2014) 35:655–68. doi:10.1016/j.neurobiolaging.2013.08.038
64. Han SK, Gottsch ML, Lee KJ, Popa SM, Smith JT, Jakawich SK, et al. Activation of gonadotropin-releasing hormone neurons by kisspeptin as a neuroendocrine switch for the onset of puberty. J Neurosci (2005) 25:11349–56. doi:10.1523/JNEUROSCI.3328-05.2005
65. Bourguignon JP, Franchimont P. Puberty-related increase in episodic LHRH release from rat hypothalamus in vitro. Endocrinology (1984) 114:1941–3. doi:10.1210/endo-114-5-1941
66. White SS, Ojeda SR. Changes in ovarian luteinizing hormone and follicle-stimulating hormone receptor content and in gonadotropin-induced ornithine decarboxylase activity during prepubertal and pubertal development of the female rat. Endocrinology (1981) 109:152–61. doi:10.1210/endo-109-1-152
67. Matagne V, Rasier G, Lebrethon MC, Gerard A, Bourguignon JP. Estradiol stimulation of pulsatile gonadotropin-releasing hormone secretion in vitro: correlation with perinatal exposure to sex steroids and induction of sexual precocity in vivo. Endocrinology (2004) 145:2775–83. doi:10.1210/en.2003-1259
68. Ishii MN, Matsumoto K, Matsui H, Seki N, Matsumoto H, Ishikawa K, et al. Reduced responsiveness of kisspeptin neurons to estrogenic positive feedback associated with age-related disappearance of LH surge in middle-age female rats. Gen Comp Endocrinol (2013) 193:121–9. doi:10.1016/j.ygcen.2013.06.024
69. Lederman MA, Lebesgue D, Gonzalez VV, Shu J, Merhi ZO, Etgen AM, et al. Age-related LH surge dysfunction correlates with reduced responsiveness of hypothalamic anteroventral periventricular nucleus kisspeptin neurons to estradiol positive feedback in middle-aged rats. Neuropharmacology (2010) 58:314–20. doi:10.1016/j.neuropharm.2009.06.015
70. Bruni JF, Huang HH, Marshall S, Meites J. Effects of single and multiple injections of synthetic GnRH on serum LH, FSH and testosterone in young and old male rats. Biol Reprod (1977) 17:309–12. doi:10.1095/biolreprod17.3.309
71. Hoffman GE, Sladek JR Jr. Age-related changes in dopamine, LHRH and somatostatin in the rat hypothalamus. Neurobiol Aging (1980) 1:27–37. doi:10.1016/0197-4580(80)90021-4
72. Li S, Givalois L, Pelletier G. Effects of aging and melatonin administration on gonadotropin-releasing hormones (GnRH) gene expression in the male and female rat. Peptides (1997) 18:1023–8. doi:10.1016/S0196-9781(97)00076-4
73. Urs A. Circadian rhythms: a fine c(l)ocktail! Curr Biol (2001) 11:R517–9. doi:10.1016/S0960-9822(01)00308-6
74. Gooley JJ, Saper CB. Chapter 33 – anatomy of the mammalian circadian system. In: Kryger MH, Roth T, Dement WC, editors. Fifth ed. Principles and Practice of Sleep Medicine. Philadelphia: W.B. Saunders (2011). p. 376–89.
75. Moore RY, Speh JC, Leak RK. Suprachiasmatic nucleus organization. Cell Tissue Res (2002) 309:89–98. doi:10.1007/s00441-002-0575-2
76. van Esseveldt KE, Lehman MN, Boer GJ. The suprachiasmatic nucleus and the circadian time-keeping system revisited. Brain Res Brain Res Rev (2000) 33:34–77. doi:10.1016/S0165-0173(00)00025-4
77. Welsh DK, Takahashi JS, Kay SA. Suprachiasmatic nucleus: cell autonomy and network properties. Annu Rev Physiol (2010) 72:551–77. doi:10.1146/annurev-physiol-021909-135919
78. Golombek DA, Rosenstein RE. Physiology of circadian entrainment. Physiol Rev (2010) 90:1063–102. doi:10.1152/physrev.00009.2009
79. Smith MJ, Jennes L, Wise PM. Localization of the VIP2 receptor protein on GnRH neurons in the female rat. Endocrinology (2000) 141:4317–20. doi:10.1210/en.141.11.4317
80. van der Beek EM, Wiegant VM, van der Donk HA, van den Hurk R, Buijs RM. Lesions of the suprachiasmatic nucleus indicate the presence of a direct vasoactive intestinal polypeptide-containing projection to gonadotrophin-releasing hormone neurons in the female rat. J Neuroendocrinol (1993) 5:137–44. doi:10.1111/j.1365-2826.1993.tb00373.x
81. Kriegsfeld LJ, Silver R, Gore AC, Crews D. Vasoactive intestinal polypeptide contacts on gonadotropin-releasing hormone neurones increase following puberty in female rats. J Neuroendocrinol (2002) 14:685–90. doi:10.1046/j.1365-2826.2002.00818.x
82. Kriegsfeld LJ, Leak RK, Yackulic CB, LeSauter J, Silver R. Organization of suprachiasmatic nucleus projections in Syrian hamsters (Mesocricetus auratus): an anterograde and retrograde analysis. J Comp Neurol (2004) 468:361–79. doi:10.1002/cne.10995
83. Ducret E, Anderson GM, Herbison AE. RFamide-related peptide-3, a mammalian gonadotropin-inhibitory hormone ortholog, regulates gonadotropin-releasing hormone neuron firing in the mouse. Endocrinology (2009) 150:2799–804. doi:10.1210/en.2008-1623
84. Ubuka T, Inoue K, Fukuda Y, Mizuno T, Ukena K, Kriegsfeld LJ, et al. Identification, expression, and physiological functions of Siberian hamster gonadotropin-inhibitory hormone. Endocrinology (2012) 153:373–85. doi:10.1210/en.2011-1110
85. Ubuka T, Son YL, Tsutsui K. Molecular, cellular, morphological, physiological and behavioral aspects of gonadotropin-inhibitory hormone. Gen Comp Endocrinol (2016) 227:27–50. doi:10.1016/j.ygcen.2015.09.009
86. Gibson EM, Humber SA, Jain S, Williams WP III, Zhao S, Bentley GE, et al. Alterations in RFamide-related peptide expression are coordinated with the preovulatory luteinizing hormone surge. Endocrinology (2008) 149:4958–69. doi:10.1210/en.2008-0316
87. Russo KA, La JL, Stephens SB, Poling MC, Padgaonkar NA, Jennings KJ, et al. Circadian control of the female reproductive axis through gated responsiveness of the RFRP-3 system to VIP signaling. Endocrinology (2015) 156:2608–18. doi:10.1210/en.2014-1762
88. Son YL, Ubuka T, Soga T, Yamamoto K, Bentley GE, Tsutsui K. Inhibitory action of gonadotropin-inhibitory hormone on the signaling pathways induced by kisspeptin and vasoactive intestinal polypeptide in GnRH neuronal cell line, GT1-7. FASEB J (2016) 30(6):2198–210. doi:10.1096/fj.201500055
89. Kriegsfeld LJ, Mei DF, Bentley GE, Ubuka T, Mason AO, Inoue K, et al. Identification and characterization of a gonadotropin-inhibitory system in the brains of mammals. Proc Natl Acad Sci U S A (2006) 103:2410–5. doi:10.1073/pnas.0511003103
90. Molnár CS, Kalló I, Liposits Z, Hrabovszky E. Estradiol down-regulates RF-amide-related peptide (RFRP) expression in the mouse hypothalamus. Endocrinology (2011) 152:1684–90. doi:10.1210/en.2010-1418
91. Kirby ED, Geraghty AC, Ubuka T, Bentley GE, Kaufer D. Stress increases putative gonadotropin inhibitory hormone and decreases luteinizing hormone in male rats. Proc Natl Acad Sci U S A (2009) 106:11324–9. doi:10.1073/pnas.0901176106
92. Son YL, Ubuka T, Narihiro M, Fukuda Y, Hasunuma I, Yamamoto K, et al. Molecular basis for the activation of gonadotropin-inhibitory hormone gene transcription by corticosterone. Endocrinology (2014) 155:1817–26. doi:10.1210/en.2013-2076
93. Brown-Grant K, Raisman G. Abnormalities in reproductive function associated with the destruction of the suprachiasmatic nuclei in female rats. Proc R Soc Lond B Biol Sci (1977) 198:279–96. doi:10.1098/rspb.1977.0098
94. Bethea CL, Neill JD. Lesions of the suprachiasmatic nuclei abolish the cervically stimulated prolactin surges in the rat. Endocrinology (1980) 107:1–5. doi:10.1210/endo-107-1-1
95. Dunn JD, Johnson DC, Castro AJ, Swenson R. Twenty-four hour pattern of prolactin levels in female rats subjected to transection of the mesencephalic raphe or ablation of the suprachiasmatic nuclei. Neuroendocrinology (1980) 31:85–91. doi:10.1159/000123055
96. Miller BH, Olson SL, Turek FW, Levine JE, Horton TH, Takahashi JS. Circadian clock mutation disrupts estrous cyclicity and maintenance of pregnancy. Curr Biol (2004) 14:1367–73. doi:10.1016/j.cub.2004.07.055
97. Balsalobre A, Damiola F, Schibler U. A serum shock induces circadian gene expression in mammalian tissue culture cells. Cell (1998) 93:929–37. doi:10.1016/S0092-8674(00)81199-X
98. Travnickova-Bendova Z, Cermakian N, Reppert SM, Sassone-Corsi P. Bimodal regulation of mPeriod promoters by CREB-dependent signaling and CLOCK/BMAL1 activity. Proc Natl Acad Sci U S A (2002) 99:7728–33. doi:10.1073/pnas.102075599
99. Mateju K, Bendova Z, El-Hennamy R, Sladek M, Sosniyenko S, Sumova A. Development of the light sensitivity of the clock genes Period1 and Period2, and immediate-early gene c-fos within the rat suprachiasmatic nucleus. Eur J Neurosci (2009) 29:490–501. doi:10.1111/j.1460-9568.2009.06609.x
100. Panda S, Antoch MP, Miller BH, Su AI, Schook AB, Straume M, et al. Coordinated transcription of key pathways in the mouse by the circadian clock. Cell (2002) 109:307–20. doi:10.1016/S0092-8674(02)00722-5
101. Yu W, Nomura M, Ikeda M. Interactivating feedback loops within the mammalian clock: BMAL1 is negatively autoregulated and upregulated by CRY1, CRY2, and PER2. Biochem Biophys Res Commun (2002) 290:933–41. doi:10.1006/bbrc.2001.6300
102. Yagita K, Tamanini F, Yasuda M, Hoeijmakers JH, van der Horst GT, Okamura H. Nucleocytoplasmic shuttling and mCRY-dependent inhibition of ubiquitylation of the mPER2 clock protein. EMBO J (2002) 21:1301–14. doi:10.1093/emboj/21.6.1301
103. Hennig S, Strauss HM, Vanselow K, Yildiz O, Schulze S, Arens J, et al. Structural and functional analyses of PAS domain interactions of the clock proteins Drosophila PERIOD and mouse PERIOD2. PLoS Biol (2009) 7:e94. doi:10.1371/journal.pbio.1000094
104. Kucera N, Schmalen I, Hennig S, Ollinger R, Strauss HM, Grudziecki A, et al. Unwinding the differences of the mammalian PERIOD clock proteins from crystal structure to cellular function. Proc Natl Acad Sci U S A (2012) 109:3311–6. doi:10.1073/pnas.1113280109
105. Ripperger JA, Schmutz I, Albrecht U. PERsuading nuclear receptors to dance the circadian rhythm. Cell Cycle (2010) 9:2515–21. doi:10.4161/cc.9.13.12075
106. Schmutz I, Ripperger JA, Baeriswyl-Aebischer S, Albrecht U. The mammalian clock component PERIOD2 coordinates circadian output by interaction with nuclear receptors. Genes Dev (2010) 24:345–57. doi:10.1101/gad.564110
107. Sahar S, Zocchi L, Kinoshita C, Borrelli E, Sassone-Corsi P. Regulation of BMAL1 protein stability and circadian function by GSK3beta-mediated phosphorylation. PLoS One (2010) 5:e8561. doi:10.1371/journal.pone.0008561
108. Alvarez JD, Hansen A, Ord T, Bebas P, Chappell PE, Giebultowicz JM, et al. The circadian clock protein BMAL1 is necessary for fertility and proper testosterone production in mice. J Biol Rhythms (2008) 23:26–36. doi:10.1177/0748730407311254
109. Kuljis DA, Loh DH, Truong D, Vosko AM, Ong ML, McClusky R, et al. Gonadal- and sex-chromosome-dependent sex differences in the circadian system. Endocrinology (2013) 154:1501–12. doi:10.1210/en.2012-1921
110. Dolatshad H, Campbell EA, O’Hara L, Maywood ES, Hastings MH, Johnson MH. Developmental and reproductive performance in circadian mutant mice. Hum Reprod (2006) 21:68–79. doi:10.1093/humrep/dei313
111. Hoshino K, Wakatsuki Y, Iigo M, Shibata S. Circadian clock mutation in dams disrupts nursing behavior and growth of pups. Endocrinology (2006) 147:1916–23. doi:10.1210/en.2005-1343
112. Kennaway DJ. The role of circadian rhythmicity in reproduction. Hum Reprod Update (2005) 11:91–101. doi:10.1093/humupd/dmh054
113. Boden MJ, Kennaway DJ. Reproduction in the arrhythmic Bmal1 knockout mouse. Reprod Fertil Dev (2005) 17:126–126. doi:10.1071/SRB05Abs297
114. Kondratov RV, Kondratova AA, Gorbacheva VY, Vykhovanets OV, Antoch MP. Early aging and age-related pathologies in mice deficient in BMAL1, the core componentof the circadian clock. Genes Dev (2006) 20:1868–73. doi:10.1101/gad.1432206
115. Ratajczak CK, Boehle KL, Muglia LJ. Impaired steroidogenesis and implantation failure in Bmal1-/- mice. Endocrinology (2009) 150:1879–85. doi:10.1210/en.2008-1021
116. Pilorz V, Steinlechner S. Low reproductive success in Per1 and Per2 mutant mouse females due to accelerated ageing? Reproduction (2008) 135:559–68. doi:10.1530/REP-07-0434
117. Chappell PE, White RS, Mellon PL. Circadian gene expression regulates pulsatile gonadotropin-releasing hormone (GnRH) secretory patterns in the hypothalamic GnRH-secreting GT1-7 cell line. J Neurosci (2003) 23:11202–13.
118. Gillespie JM, Chan BP, Roy D, Cai F, Belsham DD. Expression of circadian rhythm genes in gonadotropin-releasing hormone-secreting GT1-7 neurons. Endocrinology (2003) 144:5285–92. doi:10.1210/en.2003-0802
119. Tolson KP, Tonsfeldt KJ, Goodall CP, Chappell PE. Disruption of endogenous clocks in GnRH neurons impairs reproduction in vivo. OR12-5. The Endocrine Society’s 94th Annual Meeting and Expo. Houston, TX (2012).
120. Christian CA, Moenter SM. Vasoactive intestinal polypeptide can excite gonadotropin-releasing hormone neurons in a manner dependent on estradiol and gated by time of day. Endocrinology (2008) 149:3130–6. doi:10.1210/en.2007-1098
121. Zhao S, Kriegsfeld LJ. Daily changes in GT1-7 cell sensitivity to GnRH secretagogues that trigger ovulation. Neuroendocrinology (2009) 89:448–57. doi:10.1159/000192370
122. Funabashi T, Shinohara K, Mitsushima D, Kimura F. Gonadotropin-releasing hormone exhibits circadian rhythm in phase with arginine-vasopressin in co-cultures of the female rat preoptic area and suprachiasmatic nucleus. J Neuroendocrinol (2000) 12:521–8. doi:10.1046/j.1365-2826.2000.00481.x
123. Palm IF, Van Der Beek EM, Wiegant VM, Buijs RM, Kalsbeek A. Vasopressin induces a luteinizing hormone surge in ovariectomized, estradiol-treated rats with lesions of the suprachiasmatic nucleus. Neuroscience (1999) 93:659–66. doi:10.1016/S0306-4522(99)00106-2
124. Palm IF, van der Beek EM, Wiegant VM, Buijs RM, Kalsbeek A. The stimulatory effect of vasopressin on the luteinizing hormone surge in ovariectomized, estradiol-treated rats is time-dependent. Brain Res (2001) 901:109–16. doi:10.1016/S0006-8993(01)02309-5
125. Miller BH, Olson SL, Levine JE, Turek FW, Horton TH, Takahashi JS. Vasopressin regulation of the proestrous luteinizing hormone surge in wild-type and clock mutant mice. Biol Reprod (2006) 75:778–84. doi:10.1095/biolreprod.106.052845
126. Smith JT, Popa SM, Clifton DK, Hoffman GE, Steiner RA. Kiss1 neurons in the forebrain as central processors for generating the preovulatory luteinizing hormone surge. J Neurosci (2006) 26:6687–94. doi:10.1523/JNEUROSCI.1618-06.2006
127. Smarr BL, Morris E, de la Iglesia HO. The dorsomedial suprachiasmatic nucleus times circadian expression of kiss1 and the luteinizing hormone surge. Endocrinology (2012) 153:2839–50. doi:10.1210/en.2011-1857
128. Kalil B, Ribeiro AB, Leite CM, Uchoa ET, Carolino RO, Cardoso TS, et al. The increase in signaling by kisspeptin neurons in the preoptic area and associated changes in clock gene expression that trigger the LH surge in female rats are dependent on the facilitatory action of a noradrenaline input. Endocrinology (2016) 157:323–35. doi:10.1210/en.2015-1323
129. Tonsfeldt KJ, Goodall CP, Latham KL, Chappell PE. Oestrogen induces rhythmic expression of the Kisspeptin-1 receptor GPR54 in hypothalamic gonadotrophin-releasing hormone-secreting GT1-7 cells. J Neuroendocrinol (2011) 23:823–30. doi:10.1111/j.1365-2826.2011.02188.x
130. Li XF, Kinsey-Jones JS, Cheng Y, Knox AM, Lin Y, Petrou NA, et al. Kisspeptin signalling in the hypothalamic arcuate nucleus regulates GnRH pulse generator frequency in the rat. PLoS One (2009) 4:e8334. doi:10.1371/journal.pone.0008334
131. Burke MC, Letts PA, Krajewski SJ, Rance NE. Coexpression of dynorphin and neurokinin B immunoreactivity in the rat hypothalamus: morphologic evidence of interrelated function within the arcuate nucleus. J Comp Neurol (2006) 498:712–26. doi:10.1002/cne.21086
132. True C, Kirigiti M, Ciofi P, Grove KL, Smith MS. Characterisation of arcuate nucleus kisspeptin/neurokinin B neuronal projections and regulation during lactation in the rat. J Neuroendocrinol (2011) 23:52–64. doi:10.1111/j.1365-2826.2010.02076.x
133. Cheng G, Coolen LM, Padmanabhan V, Goodman RL, Lehman MN. The kisspeptin/neurokinin B/dynorphin (KNDy) cell population of the arcuate nucleus: sex differences and effects of prenatal testosterone in sheep. Endocrinology (2010) 151:301–11. doi:10.1210/en.2009-0541
134. Navarro VM, Gottsch ML, Wu M, Garcia-Galiano D, Hobbs SJ, Bosch MA, et al. Regulation of NKB pathways and their roles in the control of Kiss1 neurons in the arcuate nucleus of the male mouse. Endocrinology (2011) 152:4265–75. doi:10.1210/en.2011-1143
135. Wakabayashi Y, Nakada T, Murata K, Ohkura S, Mogi K, Navarro VM, et al. Neurokinin B and dynorphin A in kisspeptin neurons of the arcuate nucleus participate in generation of periodic oscillation of neural activity driving pulsatile gonadotropin-releasing hormone secretion in the goat. J Neurosci (2010) 30:3124–32. doi:10.1523/JNEUROSCI.5848-09.2010
136. Sahar S, Sassone-Corsi P. Metabolism and cancer: the circadian clock connection. Nat Rev Cancer (2009) 9:886–96. doi:10.1038/nrc2747
Keywords: kisspeptin, reproduction, circadian rhythms, clock genes, GnRH, AVPV
Citation: Putteeraj M, Soga T, Ubuka T and Parhar IS (2016) A “Timed” Kiss Is Essential for Reproduction: Lessons from Mammalian Studies. Front. Endocrinol. 7:121. doi: 10.3389/fendo.2016.00121
Received: 09 June 2016; Accepted: 19 August 2016;
Published: 31 August 2016
Edited by:
Honoo Satake, Suntory Foundation for Life Sciences, JapanReviewed by:
Pierre J. Deviche, Arizona State University, USAShinji Kanda, University of Tokyo, Japan
Copyright: © 2016 Putteeraj, Soga, Ubuka and Parhar. This is an open-access article distributed under the terms of the Creative Commons Attribution License (CC BY). The use, distribution or reproduction in other forums is permitted, provided the original author(s) or licensor are credited and that the original publication in this journal is cited, in accordance with accepted academic practice. No use, distribution or reproduction is permitted which does not comply with these terms.
*Correspondence: Ishwar S. Parhar, ishwar@monash.edu