- 1Turku PET Centre, University of Turku, Turku, Finland
- 2Department of Clinical Physiology and Nuclear Medicine, University of Turku, Turku, Finland
- 3Division of Experimental Cardiology, Thoraxcenter, Erasmus MC, University Medical Center Rotterdam, Rotterdam, Netherlands
- 4School of Kinesiology, University of British Columbia, Vancouver, BC, Canada
Adipose tissue metabolism and circulation play an important role in human health. It is well-known that adipose tissue mass is increased in response to excess caloric intake leading to obesity and further to local hypoxia and inflammatory signaling. Acute exercise increases blood supply to adipose tissue and mobilization of fat stores for energy. However, acute exercise during systemic hypoxia reduces subcutaneous blood flow in healthy young subjects, but the response in overweight or obese subjects remains to be investigated. Emerging evidence also indicates that exercise training during hypoxic exposure may provide additive benefits with respect to many traditional cardiovascular risk factors as compared to exercise performed in normoxia, but unfavorable effects of hypoxia have also been documented. These topics will be covered in this brief review dealing with hypoxia and adipose tissue physiology.
General Cardiovascular and Metabolic Responses to Hypoxia
A large body of knowledge on the physiological effects of hypoxia has been obtained over several decades from field experiments in the mountains as well as from studies in environmental chambers, where ambient air is manipulated. Hypoxia, defined as reduced or insufficient oxygen supply caused by reduced oxygen saturation of arterial blood, results in cardiovascular system adjustments to deliver more blood to tissues to compensate for reduced oxygen delivery, which is sensed by oxygen-sensing mechanisms, such as carotid bodies (1). The acute central cardiovascular response to hypoxic stress triggers an increased heart rate at an unchanged stroke volume mediated primarily by increased sympathetic neural discharge as a function of increasing hypoxic severity. At rest, lower levels of hypoxic exposure may result in some degree of systemic vasodilation, while with increasing severity of hypoxia, the peripheral vasculature constricts to redistribute oxygen delivery to the most critically dependent organs, e.g., heart (2–5), brain (6–8) needs to be ensured. This regulation is exacerbated in obstructive sleep apnea, which creates a physiological condition called chronic intermittent hypoxia, which may compromise some functions of the body. Similarly, during exercise in hypoxia, perfusion of skeletal muscle is increased to match oxygen demand, which creates circulatory competition between the locomotor skeletal muscles and other organs, and leads to decreased exercise capacity with severity of hypoxia.
In addition to cardiovascular stress, hypoxia also alters energy metabolism of the body (Figure 1). Although hypoxia might theoretically even slightly decrease the oxygen requirements at the local tissue level due to reduced oxygen supply, increased sympathetic neural activation and resulting release of various stress hormones often cause whole body metabolism to increase in response to hypoxia (9–14). It has been postulated that, particularly, glucose uptake might be favorably affected by hypoxia (13–17), which has implications for the prevention and treatment of disease states, where metabolism is deranged, such as in diabetes. Not every study, however, supports that view, as decreased skeletal muscle insulin sensitivity (18) and impaired lipid metabolism (19) have also been reported after chronic hypoxic exposures. Hypoxia also alters adipose tissue circulation, which plays an integral role in its metabolism and, therefore, has implications for obesity and diabetes.
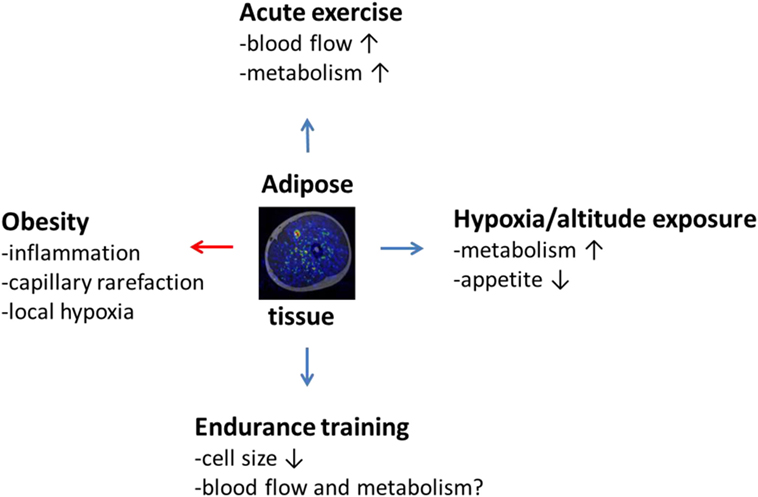
Figure 1. The effects of obesity, acute exercise, hypoxic or altitude exposure, and endurance training on adipose tissue, such as subcutaneous adipose tissue, surrounding thigh musculature as illustrated by the fusion image in the middle of the figure obtained by combining magnetic resonance and positron emission tomography imaging. Obesity induces negative (red arrow) inflammatory state in adipose tissue connected with capillary rarefaction and local hypoxia. Acute low or moderate intensity exercise is on the other hand capable of increasing adipose tissue blood flow and metabolism, which, in the long run, reduces adipose tissue cell size and inflammation. This effect may be potentiated by hypoxic exposure or altitude training, but scientific evidence is still in its infancy to prove this hypothesis correct. It also remains to be investigated to what extent classical endurance training can affect adipose tissue blood flow and metabolism in humans.
Hypoxia and Adipose Tissue Circulation and Metabolism
Adipose tissue has an important role in regulating metabolism (20–22) – a topic of growing interest as levels of obesity have increased globally over the last several decades. Adipose tissue vasculature and oxygen supply is an important determinant of its metabolism as well as endocrine function (23, 24). Despite the fact that adipose tissue has a capillary surface area less than one-third of that in skeletal muscle (24), it has long been acknowledged that also adipocytes are surrounded by an extensive network of capillaries (23). This vascular feature importantly affects the adaptability of subcutaneous adipose tissue to excess caloric overload, which is known to be associated with a hypoxic state in adipose tissue (25–27). Thus, although opposite views have also been presented (28), it is the common consensus that due to the insufficient blood supply and capillary rarefaction connected with tissue inflammation (20, 25, 29–35), chronic low oxygen levels in expanded adipose tissue is now well appreciated to contribute to metabolic derangements of the whole body.
Although there is also a noticeable extent of variability in physiological responses to hypoxia in humans (36), particularly white adipose tissue is known to respond remarkably to low levels of oxygen. This fact is well illustrated by cell culture studies, where exposure of adipocytes to low oxygen levels alters the gene expression of over 1000 genes (25). However, no change in subcutaneous adipose tissue blood flow is necessarily observed at rest in humans in response to moderate systemic hypoxia (37). Adipose tissue blood flow in humans is under the regulation of the sympathetic nervous system (38), and it is, therefore, reasonable to assume that moderate systemic hypoxia simply does not create a high enough stimulus for sympathetic neural vasoconstrictor activation to reduce blood flow in healthy human adipose tissue. On the other hand, it is also plausible that the activation of vasoconstriction by arterial chemoreceptors predominates over a local hypoxic vasodilation in adipose tissue in humans. In this regard, adipose tissue appears to be similar to bone (39). However, increased blood flow in response to systemic hypoxia has been documented in human skin (40). More studies are clearly warranted to explore whether unchanged hypoxic blood flow is also of importance to explain pathophysiological characteristics of adipose tissue under chronically low oxygen levels that is not compensated by increased blood flow (20, 25).
It is known that subcutaneous adipose tissue blood flow increases in response to low intensity exercise, but levels off when exercise intensity is further increased (41). Furthermore, at rest, but not during exercise, subcutaneous adipose blood flow is under the control of nitric oxide (42). In contrast to resting conditions, subcutaneous adipose blood flow is reduced during exercise, when subjects breathe hypoxic air (37). This novel finding is likely based on the constriction of adipose tissue vasculature by hypoxia-triggered enhanced sympathetic nervous system activity, which redistributes limb blood flow to exercising muscles, which depend more critically on adequate oxygen supply in response to exercise. In this regard, we have previously reported that blood flow in subcutaneous adipose tissue is significantly lowered by local infusion of norepinephrine, which is the principal neurotransmitter released from the sympathetic nerve endings, and that the inhibition of α-adrenergic receptors by phentolamine tends to enhance adipose tissue blood flow, both at rest and during exercise (38). It has also been previously suggested by Romijn et al. (43) that the reduction of adipose tissue blood flow is likely to be one important mechanism to explain decreased free-fatty acid release in response to high intensity exercise, which then leads to preferential utilization of glucose instead of fatty acids and contributes to the increased efficiency of ATP generation for a limited O2 availability. Additionally, it has been recently documented that the inability to increase vascular resistance in adipose tissue during exercise or to maintain mean arterial pressure during orthostatic stress in aging is largely a result of reduced α-adrenergic responsiveness of adipose tissue arterioles (44, 45). Therefore, it is concluded that reduced blood flow in adipose tissue is an acute physiological response to diminished oxygen availability during exercise, while higher blood flow in adipose tissue is needed in response to prolonged exercise that also likely associates with higher lipolysis to supply more free-fatty acids into circulation to sustain muscular work for prolonged periods (46).
In addition to the general hypoxic responses, the capacity of blood flow in human subcutaneous adipose tissue has remained largely unexplored, until recently. In this regard, a novel finding is that the vasodilatory capacity of human subcutaneous adipose tissue determined by infusion of exogenous dilator compounds approaches the physiological level reached during moderate intensity exercise (37). Furthermore, during this maximal vasodilation, vascular conductance can reach a level even higher than that induced by exercise. In terms of absolute values, the comparison of adipose tissue blood flow capacity to skeletal muscle is also of interest. In this regard, we have previously reported that blood flow in human skeletal muscle during a similar pharmacological vasodilation protocol increases to a level of 40 ml/min/100 g (47). As the absolute average value of pharmacologically induced adipose tissue blood flow was 10.5 ml/min/100 g, it only reaches ~26% of blood flow level in the muscle. Accordingly, the functional vascular capacity appears to be very closely followed by that of structural anatomy, as adipose tissue is known to have a capillary surface area that is slightly less than one-third than that in skeletal muscle (24). In relative terms, blood flow in adipose tissue increased 8-fold and blood flow in muscle 14-fold in response to pharmacological (adenosine) infusion, and, as such, the increase in adipose tissue flow is 57% of that of muscle. In contrast to human skeletal muscle (47), pharmacologically induced blood flow is not, however, positively and significantly related to subjects’ whole body maximal oxygen consumption determined in a separate fitness test, indicating that blood flow in adipose tissue and muscle do not simply parallel each other. Nevertheless, it can be concluded, based on these studies, that the functional blood flow capacity of adipose tissue is fairly large in healthy human subjects. It remains, however, to be measured if this capacity is lost in pathological states. Furthermore, it also remains to be determined if a loss of functional vascular capacity is linked to impaired fat storage in white adipose tissue which is known to contribute to metabolic and cardiovascular derangements in a human body (20).
Hypoxia as a Treatment of Obesity and Impaired Adipose Tissue Physiology?
As summarized in the beginning of the previous section, it is evident that there is a hypoxic state in adipose tissue of obese subjects, which may be caused by insufficient circulatory responses/adaptations in response to lowered oxygen supply. Despite this, chronic and/or intermittent hypoxia has also been suggested as treatment option for overweight and obesity (9, 10). This is based on findings that hypoxia alters the function of the nervous system and hormonal levels such as leptin, which lead to changes in glucose metabolism and control of appetite (9–14). These physiological responses are enhanced with increasing severity of hypoxia, such as altitude exposure. There is evidence that people living at high altitude are less likely to be overweight and/or obese, the findings which hold after adjustment for many plausible confounding factors that might also affect the association (48, 49). Protective effects of hypoxia/altitude have also been reported in regards to development of diabetes (50) and coronary heart disease, as well as stroke (51–53), meaning that hypoxia reduced the incidence of these diseases (Table 1). Furthermore, interventional trials have been conducted to test the effects of hypoxia as a treatment for weight loss and improvement of metabolic functions (Table 1). These studies demonstrated that 7 h of moderate hypoxia under resting conditions did not change postprandial glucose responses or substrate oxidation in young healthy men (54). However, when hypoxic exposure was combined with low intensity physical activity, Netzer and colleagues reported greater weight loss in obese subjects when compared to combined exercise and sham hypoxia intervention (55), although this finding could not be reproduced in their recent study (56). Beneficial effects of hypoxia regarding body weight control have also been reported in obese young adults (57). Furthermore, Haufe et al. comprehensively investigated numerous cardiovascular risk factors in response to hypoxic training and showed favorable influences on body fat content, triglycerides, fasting insulin, and insulin sensitivity, as compared to exercise training only intervention (58). These findings were confirmed in their later study in overweight and obese men with lower exercise workload, which reduces exercise burden for overweight subjects and is, thus, beneficial in terms of exercise compliance (59). Appetite regulation is not necessarily always affected, although lipid profile is improved (60). Altogether, it is concluded that training in hypoxia appears to have numerous additive and favorable effects on traditional cardiovascular risk factors, which may have important clinical implications (61, 62).
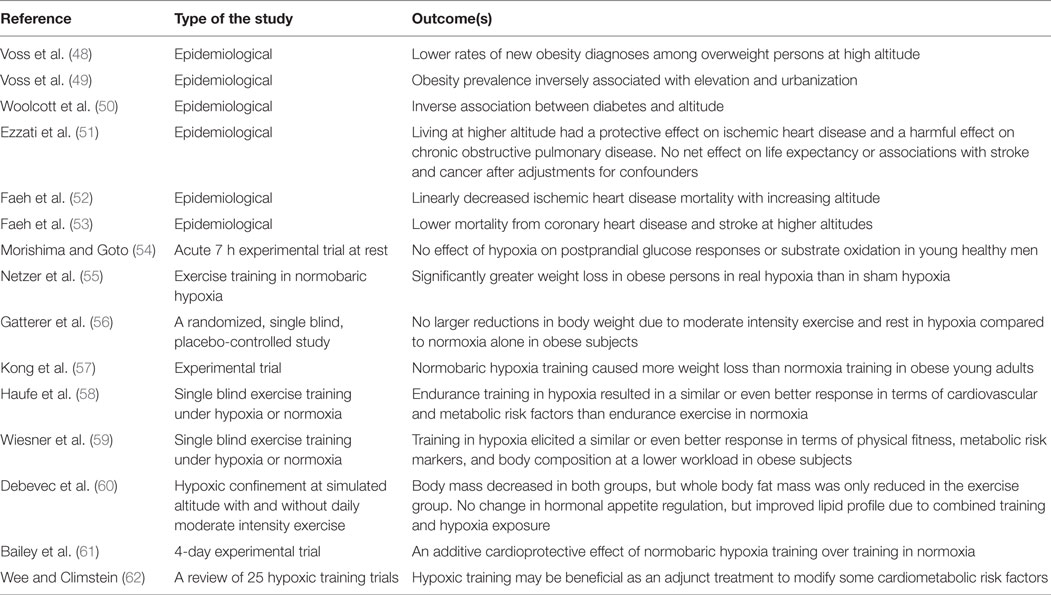
Table 1. Summary of studies investigating the effects of hypoxia on cardiovascular and metabolic health in humans.
Despite plausible physiological mechanisms and some encouraging results that hypoxia might indeed work as a potential therapeutic tool to tackle obesity, it may also have detrimental influences that need some consideration. First, hypoxia might not be well-tolerated by all subjects, as high-altitude illness is experienced in approximately 10 to 25% of unacclimatized persons above 2500 m, and the prevalence and severity of symptoms increases with increments in altitude (63). Second, hypoxia is associated with impaired cognitive performance, which may persist even after the cessation of hypoxic exposure (64). Third, hypoxic exposure is known to impair human immune system function (65), which may be detrimental in fighting against pathogens and other triggers of communicable diseases. Fourth, hypoxia is capable of inducing fibrosis in cardiac muscle (66), which increases the stiffness of the heart. Many obese individuals already have cardiac stiffness (67, 68), which may be exaggerated by hypoxic exposure. Finally, as mentioned in the previous section, hypoxia triggers an inflammatory response in adipose tissue of obese subjects, which may be further exacerbated by hypoxia creating a vicious-cycle. Thus, hypoxia not only alters human energy metabolism, which may lead to weight loss if not compensated for by increased energy intake, but is also capable of inducing several physiologically detrimental effects on bodily functions. It is likely that the balance of all these determine the overall outcome and health effects of hypoxia in humans.
Finally, as hypoxia is indeed a common feature of adipose tissue in particular, and potentially other tissues in obese subjects, it has been suggested that hyperoxia might be an option to overcome the hypoxic state. However, as oxygen is known to be toxic in high concentrations, this treatment may not be healthy in terms of circulatory and metabolic function. Hyperoxia is known to decrease adipose cell viability, increase both intra- and extracellular oxidative stress, provoke inflammation, and decrease glucose uptake of adipocytes (69). Hence, based on this information on hyperoxia and reviewed knowledge regarding hypoxia, it is concluded that there is a delicate balance of healthy oxygen supply and demand in adipose tissue that determines its overall function. While mild hypoxia over a sufficient duration of exposure may provide some additional benefits, the most feasible approach to address obesity and individual weight loss appears to reside in more traditional methods proven to be efficient in reducing adipose tissue size: physical activity and diet rich in fruits and vegetables, but low in caloric energy (Figure 1).
Author Contributions
IH drafted the manuscript and all authors contributed to its revision and intellectual content.
Conflict of Interest Statement
The authors declare that the research was conducted in the absence of any commercial or financial relationships that could be construed as a potential conflict of interest.
The reviewer MH and handling Editor declared their shared affiliation, and the handling Editor states that the process nevertheless met the standards of a fair and objective review.
Funding
The present review was financially supported by The Ministry of Education of State of Finland, Academy of Finland, The Finnish Cardiovascular Foundation, and The Finnish Diabetes Research Foundation.
References
1. Weir EK, Lopez-Barneo J, Buckler KJ, Archer SL. Acute oxygen-sensing mechanisms. N Engl J Med (2005) 353:2042–55. doi:10.1056/NEJMra050002
2. Kaijser L, Grubbstrom J, Berglund B. Coronary circulation in acute hypoxia. Clin Physiol (1990) 10:259–63. doi:10.1111/j.1475-097X.1990.tb00094.x
3. Heinonen I, Luotolahti M, Vuolteenaho O, Nikinmaa M, Saraste A, Hartiala J, et al. Circulating N-terminal brain natriuretic peptide and cardiac function in response to acute systemic hypoxia in healthy humans. J Transl Med (2014) 12:189. doi:10.1186/1479-5876-12-189
4. Tune JD. Control of coronary blood flow during hypoxemia. Adv Exp Med Biol (2007) 618:25–39. doi:10.1007/978-0-387-75434-5_3
5. Kaufmann PA, Schirlo C, Pavlicek V, Berthold T, Burger C, von Schulthess GK, et al. Increased myocardial blood flow during acute exposure to simulated altitudes. J Nucl Cardiol (2001) 8:158–64. doi:10.1067/mnc.2001.112537
6. Verges S, Rupp T, Jubeau M, Wuyam B, Esteve F, Levy P, et al. Cerebral perturbations during exercise in hypoxia. Am J Physiol Regul Integr Comp Physiol (2012) 302:R903–16. doi:10.1152/ajpregu.00555.2011
7. Buck A, Schirlo C, Jasinksy V, Weber B, Burger C, von Schulthess GK, et al. Changes of cerebral blood flow during short-term exposure to normobaric hypoxia. J Cereb Blood Flow Metab (1998) 18:906–10. doi:10.1097/00004647-199808000-00011
8. Norcliffe LJ, Rivera-Ch M, Claydon VE, Moore JP, Leon-Velarde F, Appenzeller O, et al. Cerebrovascular responses to hypoxia and hypocapnia in high-altitude dwellers. J Physiol (2005) 566:287–94. doi:10.1113/jphysiol.2005.086629
9. Kayser B, Verges S. Hypoxia, energy balance and obesity: from pathophysiological mechanisms to new treatment strategies. Obes Rev (2013) 14:579–92. doi:10.1111/obr.12034
10. Urdampilleta A, Gonzalez-Muniesa P, Portillo MP, Martinez JA. Usefulness of combining intermittent hypoxia and physical exercise in the treatment of obesity. J Physiol Biochem (2012) 68:289–304. doi:10.1007/s13105-011-0115-1
11. Yingzhong Y, Droma Y, Rili G, Kubo K. Regulation of body weight by leptin, with special reference to hypoxia-induced regulation. Intern Med (2006) 45:941–6. doi:10.2169/internalmedicine.45.1733
12. Rose MS, Houston CS, Fulco CS, Coates G, Sutton JR, Cymerman A. Operation everest. II: nutrition and body composition. J Appl Physiol (1985) (1988) 65:2545–51.
13. Woolcott OO, Ader M, Bergman RN. Glucose homeostasis during short-term and prolonged exposure to high altitudes. Endocr Rev (2015) 36:149–73. doi:10.1210/er.2014-1063
14. Ichiki T, Sunagawa K. Novel roles of hypoxia response system in glucose metabolism and obesity. Trends Cardiovasc Med (2014) 24:197–201. doi:10.1016/j.tcm.2014.03.004
15. Heinonen I, Kemppainen J, Kaskinoro K, Peltonen JE, Sipila HT, Nuutila P, et al. Effects of adenosine, exercise, and moderate acute hypoxia on energy substrate utilization of human skeletal muscle. Am J Physiol Regul Integr Comp Physiol (2012) 302:R385–90. doi:10.1152/ajpregu.00245.2011
16. Rafacho A, Goncalves-Neto LM, Ferreira FB, Protzek AO, Boschero AC, Nunes EA, et al. Glucose homoeostasis in rats exposed to acute intermittent hypoxia. Acta Physiol (Oxf) (2013) 209:77–89. doi:10.1111/apha.12118
17. Marquez JL, Rubinstein S, Fattor JA, Shah O, Hoffman AR, Friedlander AL. Cyclic hypobaric hypoxia improves markers of glucose metabolism in middle-aged men. High Alt Med Biol (2013) 14:263–72. doi:10.1089/ham.2012.1057
18. Yu J, Shi L, Wang H, Bilan PJ, Yao Z, Samaan MC, et al. Conditioned medium from hypoxia-treated adipocytes renders muscle cells insulin resistant. Eur J Cell Biol (2011) 90:1000–15. doi:10.1016/j.ejcb.2011.06.004
19. Siques P, Brito J, Naveas N, Pulido R, De la Cruz JJ, Mamani M, et al. Plasma and liver lipid profiles in rats exposed to chronic hypobaric hypoxia: changes in metabolic pathways. High Alt Med Biol (2014) 15:388–95. doi:10.1089/ham.2013.1134
20. Sun K, Kusminski CM, Scherer PE. Adipose tissue remodeling and obesity. J Clin Invest (2011) 121:2094–101. doi:10.1172/JCI45887
21. Tchernof A, Despres JP. Pathophysiology of human visceral obesity: an update. Physiol Rev (2013) 93:359–404. doi:10.1152/physrev.00033.2011
22. Feng B, Zhang T, Xu H. Human adipose dynamics and metabolic health. Ann N Y Acad Sci (2013) 1281:160–77. doi:10.1111/nyas.12009
24. Roddie IC. Circulation to skin and adipose tissue. In: Shepherd JT, Abboud FM, editors. Handbook of Physiology. Bethesda, MD: American Physiological Society (1983). p. 285–317.
25. Trayhurn P. Hypoxia and adipose tissue function and dysfunction in obesity. Physiol Rev (2013) 93:1–21. doi:10.1152/physrev.00017.2012
26. Goossens GH, Blaak EE. Adipose tissue dysfunction and impaired metabolic health in human obesity: a matter of oxygen? Front Endocrinol (2015) 6:55. doi:10.3389/fendo.2015.00055
27. Lin Q, Yun Z. The hypoxia-inducible factor pathway in adipocytes: the role of HIF-2 in adipose inflammation and hypertrophic cardiomyopathy. Front Endocrinol (2015) 6:39. doi:10.3389/fendo.2015.00039
28. Goossens GH, Bizzarri A, Venteclef N, Essers Y, Cleutjens JP, Konings E, et al. Increased adipose tissue oxygen tension in obese compared with lean men is accompanied by insulin resistance, impaired adipose tissue capillarization, and inflammation. Circulation (2011) 124:67–76. doi:10.1161/CIRCULATIONAHA.111.027813
29. Wood IS, de Heredia FP, Wang B, Trayhurn P. Cellular hypoxia and adipose tissue dysfunction in obesity. Proc Nutr Soc (2009) 68:370–7. doi:10.1017/S0029665109990206
30. Lawler HM, Underkofler CM, Kern PA, Erickson C, Bredbeck B, Rasouli N. Adipose tissue hypoxia, inflammation and fibrosis in obese insulin sensitive and obese insulin resistant subjects. J Clin Endocrinol Metab (2016) 101(4):1422–8. doi:10.1210/jc.2015-4125
31. Spencer M, Unal R, Zhu B, Rasouli N, McGehee RE Jr, Peterson CA, et al. Adipose tissue extracellular matrix and vascular abnormalities in obesity and insulin resistance. J Clin Endocrinol Metab (2011) 96:E1990–8. doi:10.1210/jc.2011-1567
32. Ye J. Adipose tissue vascularization: its role in chronic inflammation. Curr Diab Rep (2011) 11:203–10. doi:10.1007/s11892-011-0183-1
33. Gealekman O, Guseva N, Hartigan C, Apotheker S, Gorgoglione M, Gurav K, et al. Depot-specific differences and insufficient subcutaneous adipose tissue angiogenesis in human obesity. Circulation (2011) 123:186–94. doi:10.1161/CIRCULATIONAHA.110.970145
34. Pasarica M, Sereda OR, Redman LM, Albarado DC, Hymel DT, Roan LE, et al. Reduced adipose tissue oxygenation in human obesity: evidence for rarefaction, macrophage chemotaxis, and inflammation without an angiogenic response. Diabetes (2009) 58:718–25. doi:10.2337/db08-1098
35. Trayhurn P, Alomar SY. Oxygen deprivation and the cellular response to hypoxia in adipocytes – perspectives on white and brown adipose tissues in obesity. Front Endocrinol (2015) 6:19. doi:10.3389/fendo.2015.00019
36. Brooks JT, Elvidge GP, Glenny L, Gleadle JM, Liu C, Ragoussis J, et al. Variations within oxygen-regulated gene expression in humans. J Appl Physiol (1985) (2009) 106:212–20. doi:10.1152/japplphysiol.90578.2008
37. Heinonen I, Kemppainen J, Kaskinoro K, Knuuti J, Boushel R, Kalliokoski KK. Capacity and hypoxic response of subcutaneous adipose tissue blood flow in humans. Circ J (2014) 78:1501–6. doi:10.1253/circj.CJ-13-1273
38. Heinonen I, Wendelin-Saarenhovi M, Kaskinoro K, Knuuti J, Scheinin M, Kalliokoski KK. Inhibition of alpha-adrenergic tone disturbs the distribution of blood flow in the exercising human limb. Am J Physiol Heart Circ Physiol (2013) 305(2):H163–72. doi:10.1152/ajpheart.00925.2012
39. Heinonen I, Kemppainen J, Kaskinoro K, Langberg H, Knuuti J, Boushel R, et al. Bone blood flow and metabolism in humans: effect of muscular exercise and other physiological perturbations. J Bone Miner Res (2013) 28:1068–74. doi:10.1002/jbmr.1833
40. Simmons GH, Minson CT, Cracowski JL, Halliwill JR. Systemic hypoxia causes cutaneous vasodilation in healthy humans. J Appl Physiol (2007) 103:608–15. doi:10.1152/japplphysiol.01443.2006
41. Heinonen I, Bucci M, Kemppainen J, Knuuti J, Nuutila P, Boushel R, et al. Regulation of subcutaneous adipose tissue blood flow during exercise in humans. J Appl Physiol (1985) (2012) 112:1059–63. doi:10.1152/japplphysiol.00732.2011
42. Heinonen I, Saltin B, Kemppainen J, Sipila HT, Oikonen V, Nuutila P, et al. Skeletal muscle blood flow and oxygen uptake at rest and during exercise in humans: a pet study with nitric oxide and cyclooxygenase inhibition. Am J Physiol Heart Circ Physiol (2011) 300:H1510–7. doi:10.1152/ajpheart.00996.2010
43. Romijn JA, Coyle EF, Sidossis LS, Gastaldelli A, Horowitz JF, Endert E, et al. Regulation of endogenous fat and carbohydrate metabolism in relation to exercise intensity and duration. Am J Physiol (1993) 265:E380–91.
44. Davis RT III, Stabley JN, Dominguez JM, Ramsey MW, McCullough DJ, Lesniewski LA, et al. Differential effects of aging and exercise on intra-abdominal adipose arteriolar function and blood flow regulation. J Appl Physiol (2013) 114:808–15. doi:10.1152/japplphysiol.01358.2012
45. Ramsey MW, Behnke BJ, Prisby RD, Delp MD. Effects of aging on adipose resistance artery vasoconstriction: possible implications for orthostatic blood pressure regulation. J Appl Physiol (2007) 103:1636–43. doi:10.1152/japplphysiol.00637.2007
46. Stallknecht B, Lorentsen J, Enevoldsen LH, Bulow J, Biering-Sorensen F, Galbo H, et al. Role of the sympathoadrenergic system in adipose tissue metabolism during exercise in humans. J Physiol (2001) 536:283–94. doi:10.1111/j.1469-7793.2001.00283.x
47. Heinonen I, Kemppainen J, Kaskinoro K, Peltonen JE, Borra R, Lindroos MM, et al. Comparison of exogenous adenosine and voluntary exercise on human skeletal muscle perfusion and perfusion heterogeneity. J Appl Physiol (2010) 108:378–86. doi:10.1152/japplphysiol.00745.2009
48. Voss JD, Allison DB, Webber BJ, Otto JL, Clark LL. Lower obesity rate during residence at high altitude among a military population with frequent migration: a quasi experimental model for investigating spatial causation. PLoS One (2014) 9:e93493. doi:10.1371/journal.pone.0093493
49. Voss JD, Masuoka P, Webber BJ, Scher AI, Atkinson RL. Association of elevation, urbanization and ambient temperature with obesity prevalence in the United States. Int J Obes (Lond) (2013) 37:1407–12. doi:10.1038/ijo.2013.5
50. Woolcott OO, Castillo OA, Gutierrez C, Elashoff RM, Stefanovski D, Bergman RN. Inverse association between diabetes and altitude: a cross-sectional study in the adult population of the United States. Obesity (Silver Spring) (2014) 22:2080–90. doi:10.1002/oby.20800
51. Ezzati M, Horwitz ME, Thomas DS, Friedman AB, Roach R, Clark T, et al. Altitude, life expectancy and mortality from ischaemic heart disease, stroke, COPD and cancers: national population-based analysis of US counties. J Epidemiol Community Health (2012) 66:e17. doi:10.1136/jech.2010.112938
52. Faeh D, Moser A, Panczak R, Bopp M, Roosli M, Spoerri A, et al. Independent at heart: persistent association of altitude with ischaemic heart disease mortality after consideration of climate, topography and built environment. J Epidemiol Community Health (2016) 70(8):798–806. doi:10.1136/jech-2015-206210
53. Faeh D, Gutzwiller F, Bopp M; Swiss National Cohort Study Group. Lower mortality from coronary heart disease and stroke at higher altitudes in Switzerland. Circulation (2009) 120:495–501. doi:10.1161/CIRCULATIONAHA.108.819250
54. Morishima T, Goto K. Successive exposure to moderate hypoxia does not affect glucose metabolism and substrate oxidation in young healthy men. Springerplus (2014) 3:370. doi:10.1186/2193-1801-3-370
55. Netzer NC, Chytra R, Kupper T. Low intense physical exercise in normobaric hypoxia leads to more weight loss in obese people than low intense physical exercise in normobaric sham hypoxia. Sleep Breath (2008) 12:129–34. doi:10.1007/s11325-007-0149-3
56. Gatterer H, Haacke S, Burtscher M, Faulhaber M, Melmer A, Ebenbichler C, et al. Normobaric intermittent hypoxia over 8 months does not reduce body weight and metabolic risk factors – a randomized, single blind, placebo-controlled study in normobaric hypoxia and normobaric sham hypoxia. Obes Facts (2015) 8:200–9. doi:10.1159/000431157
57. Kong Z, Zang Y, Hu Y. Normobaric hypoxia training causes more weight loss than normoxia training after a 4-week residential camp for obese young adults. Sleep Breath (2014) 18:591–7. doi:10.1007/s11325-013-0922-4
58. Haufe S, Wiesner S, Engeli S, Luft FC, Jordan J. Influences of normobaric hypoxia training on metabolic risk markers in human subjects. Med Sci Sports Exerc (2008) 40:1939–44. doi:10.1249/MSS.0b013e31817f1988
59. Wiesner S, Haufe S, Engeli S, Mutschler H, Haas U, Luft FC, et al. Influences of normobaric hypoxia training on physical fitness and metabolic risk markers in overweight to obese subjects. Obesity (Silver Spring) (2010) 18:116–20. doi:10.1038/oby.2009.193
60. Debevec T, Simpson EJ, MacDonald IA, Eiken O, Mekjavic IB. Exercise training during normobaric hypoxic confinement does not alter hormonal appetite regulation. PLoS One (2014) 9:e98874. doi:10.1371/journal.pone.0098874
61. Bailey DM, Davies B, Baker J. Training in hypoxia: modulation of metabolic and cardiovascular risk factors in men. Med Sci Sports Exerc (2000) 32:1058–66. doi:10.1097/00005768-200006000-00004
62. Wee J, Climstein M. Hypoxic training: clinical benefits on cardiometabolic risk factors. J Sci Med Sport (2015) 18:56–61. doi:10.1016/j.jsams.2013.10.247
63. Bartsch P, Swenson ER. Acute high-altitude illnesses. N Engl J Med (2013) 368:2294–302. doi:10.1056/NEJMcp1214870
64. Yan X. Cognitive impairments at high altitudes and adaptation. High Alt Med Biol (2014) 15:141–5. doi:10.1089/ham.2014.1009
65. Oliver SJ, Macdonald JH, Harper Smith AD, Lawley JS, Gallagher CA, Di FU, et al. High altitude impairs in vivo immunity in humans. High Alt Med Biol (2013) 14:144–9. doi:10.1089/ham.2012.1070
66. Watson CJ, Collier P, Tea I, Neary R, Watson JA, Robinson C, et al. Hypoxia-induced epigenetic modifications are associated with cardiac tissue fibrosis and the development of a myofibroblast-like phenotype. Hum Mol Genet (2014) 23:2176–88. doi:10.1093/hmg/ddt614
67. Goncalves N, Silva AF, Rodrigues PG, Correia E, Moura C, Eloy C, et al. Early cardiac changes induced by a hypercaloric diet in “subclinical” obesity. Am J Physiol Heart Circ Physiol (2016) 15:H655–66. doi:10.1152/ajpheart.00684.2015
68. Leopoldo AS, Sugizaki MM, Lima-Leopoldo AP, do Nascimento AF, Luvizotto RA, de Campos DH, et al. Cardiac remodeling in a rat model of diet-induced obesity. Can J Cardiol (2010) 26:423–9. doi:10.1016/S0828-282X(10)70440-2
Keywords: hypoxia, humans, blood flow, metabolism, adipose tissue
Citation: Heinonen IHA, Boushel R and Kalliokoski KK (2016) The Circulatory and Metabolic Responses to Hypoxia in Humans – With Special Reference to Adipose Tissue Physiology and Obesity. Front. Endocrinol. 7:116. doi: 10.3389/fendo.2016.00116
Received: 08 March 2016; Accepted: 10 August 2016;
Published: 29 August 2016
Edited by:
Daniela Patrizia Foti, Magna Graecia University, ItalyReviewed by:
Marta Letizia Hribal, Magna Graecia University, ItalySinan Tanyolac, Istanbul University, Turkey
Copyright: © 2016 Heinonen, Boushel and Kalliokoski. This is an open-access article distributed under the terms of the Creative Commons Attribution License (CC BY). The use, distribution or reproduction in other forums is permitted, provided the original author(s) or licensor are credited and that the original publication in this journal is cited, in accordance with accepted academic practice. No use, distribution or reproduction is permitted which does not comply with these terms.
*Correspondence: Ilkka H. A. Heinonen, aWxra2EuaGVpbm9uZW5AdXR1LmZp