- 1Department of Exercise Science, Concordia University, Montreal, QC, Canada
- 2Nutrition, Obesity, and Metabolism Laboratory, PERFORM Centre, Montreal, QC, Canada
- 3Endocrine Research Unit, Mayo Clinic, Rochester, MN, USA
In addition to the obvious differences in body shape, there are substantial differences in lipid metabolism between men and women. These differences include how dietary fatty acids are handled, the secretion and clearance of very low-density lipoprotein-triglycerides, the release rates of free fatty acids (FFA) from adipose tissue relative to energy needs, and the removal of FFA from the circulation, including the storage of FFA into adipose tissue via the direct uptake process. We will review what is known about these processes and how they may contribute to the sexual dimorphism of body fat distribution.
Introduction
Where we store fat in our bodies is a significant predictor of metabolic disease risk. Metabolic diseases predominantly affect people with an upper body/android fat distribution compared to those with a lower body/gynoid distribution (1). Jean Vague was the first to report the relationship between “android” obesity and diabetes (2). Since that report, numerous studies have confirmed the relationship between central adiposity and risk of diabetes (3, 4), cardiovascular disease risk and events (5, 6), hypertension (7), sleep apnea (8), cancer (9), and overall mortality rates (10); men and women in the highest quintile of measured waist circumference (WC) are reported to have almost twice the mortality rates than those in the lower quintiles. Though we do not fully understand why people are shaped differently, one of the most obvious factors determining body shape is sex hormone status.
Healthy, modestly active/sedentary women with BMIs of 20–25 kg/m2 often have 25–35% body fat, whereas men of the same BMI have 10–20% body fat; highly athletic women and men can have less body fat (11). Typical non-obese adult males have little leg fat relative to upper body fat and most non-obese, premenopausal adult females have ample lower body fat stores and limited visceral adipose tissue (VAT). In contrast, men have greater amounts of VAT, even controlling for body fat mass, and VAT constitutes a greater proportion of total fat mass in men than women, especially at higher BMIs (12). The mechanisms underlying the sexual dimorphism in body fat and its distribution between men and women are not well understood. Studies that investigate the contribution of lipid metabolism to body shape indicate a staunch effect of sex steroids. The objective of this review is to examine how lipid metabolism contributes to differences in body fat distribution and the role of sex hormones in modulating these differences.
Fatty Acid Metabolism
The principle function of adipose tissue is to store and release FA in response to changes in energy balance. Following consumption of a fat containing meal, a significant proportion of circulating chylomicron-triacylglycerol (TAG) fatty acids (FA) is stored via lipoprotein lipase (LPL) into adipose tissue. In this pathway, endothelial LPL hydrolyzes FA from the glycerol backbone of chylomicron-TAG, allowing them to enter the adjacent adipose tissue. Some of the FAs from chylomicron-TAG are not taken up locally, but instead “spillover” into systemic circulation. A less appreciated pathway of FA storage occurs via the direct re-uptake of free fatty acids (FFA) from the systemic circulation independent of the LPL mechanism. Although we typically envision that the FFA released as a result of adipose tissue lipolysis are destined to supply fuel to lean tissues for fat oxidation, a portion is taken up and stored in distant adipocytes. We have referred to this process as direct FFA storage.
In addition to LPL, there are a number of other proteins and enzymes that are involved in adipose tissue FA metabolism. When it comes to FA entry into cells, FA can be taken up by passive (flip–flop) diffusion or via membrane protein facilitated diffusion (13). One of the more closely examined proteins involved in facilitated diffusion is the FA transport protein CD36. CD36 is a cell surface glycoprotein that is particularly important when extracellular FA concentrations are reduced (13, 14). CD36 appears to play a main role in the localization of long chain FA to the adipocyte membrane through FA binding (13). Recent studies indicate that CD36 facilitates muscle and adipose tissue FFA uptake under conditions of reduced FFA availability, whereas hepatic FFA uptake appears to be CD36-independent and myocardial uptake is partially CD36-dependent across a wider range of FFA concentrations (14). Other proteins that have been investigated in their role of FA storage are acyl-CoA synthetase (ACS) and diacylglycerol acyl transferase (DGAT). ACS catalyzes the intracellular activation of the FA to their CoA form (15). DGAT, the final step of storage of FA as TAG, is suggested to be a rate limiting enzyme in FA storage (16). There are three enzymes involved in triglyceride hydrolysis: adipose triglyceride lipase (ATGL), hormone-sensitive lipase (HSL), and monoglyceride lipase (MGL) (17). Of these three enzymes, HSL has been the most studied and has been linked to aberrations in lipolysis associated with obesity (18). HSL hydrolyzes an assortment of substrates including TAG, diacylglycerol, and monoacylglycerol (17). We have postulated that the activity of proteins involved in FA storage and lipolysis play key roles in modulating regional fat distribution.
The relative and absolute size of the different adipose tissue depots is ultimately determined by the balance between lipolysis on one hand and storage of FA from chylomicrons, very low-density lipoprotein (VLDL), and direct FFA uptake on the other. Although other factors, such as the gain or loss of lean tissue and changes in endocrine signaling, impact body composition, this review will focus on studies that examine the contributions of FA metabolism in determining regional body composition. Several studies have been conducted to expand our understanding of how the variation in body fat distribution in men and women relate to systemic and regional fat metabolism.
Lipid Metabolism in Men and Women
Figure 1 summarizes the findings in lean men vs. age- and BMI-matched premenopausal women for each aspect of FA metabolism discussed below.
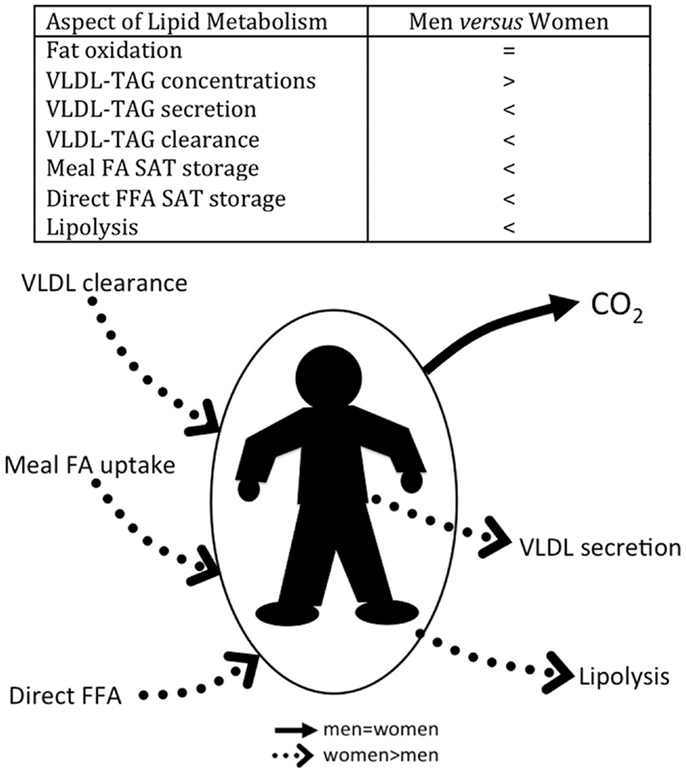
Figure 1. Summary of relative FFA metabolism in young, lean, fasted men, and age- and BMI-matched fasted premenopausal women.
Fatty Acid Oxidation
In the postprandial and postabsorptive state, FA oxidation does not appear to be different between men and women whereas during exercise fat oxidation is higher in women. This is because there are several factors other than sex that are potential independent contributors toward the variations in fat oxidation between individuals including energy balance, age, heredity, and body composition (19). Because fat oxidation has been shown to relate to fat free mass (FFM), and because men and women of the same weight often differ with regards to body composition, comparing fat oxidation in milligrams per minute or milligram per kilogram per minute may be misleading. In determining whether there are differences in fat oxidation between sexes, FFM can be accounted for by statistical adjustment or fat oxidation can be expressed in the context of total energy expenditure (as RQ or a percent). Unless men and women are matched for FFM, we prefer not to express substrate oxidation divided by FFM per unit time. This is because the relationship between energy expenditure and FFM is not ratio-standard; that is, the intercept is not zero and the slope is not 1. On average at a given energy expenditure (substrate oxidation), individuals with lower FFM will de facto have greater energy expenditure per kilogram FFM than those with greater amounts of FFM.
When the results of tracer studies were integrated, it was suggested that over a day that includes 2 h of exercise, 14 h in the postabsorptive state, and 8 h in the postprandial state, fat oxidation was similar in men and women (20). This conclusion was supported by a study that measured 24 h RQ in a whole room calorimeter that included spontaneous physical activity, which found no differences between men and women (19). The lack of a difference in fat oxidation between men and women remains after adjusting for FFM during rest (21) or during a 24 h period with the incorporation of light–moderate physical activity (22). However, it should be noted that it is difficult to draw conclusions based on these and the following studies discussed herein for lack of appropriate statistical adjustment for FFM. In contrast, a whole room calorimeter study by Melanson et al. (23) found that women had 24–56% greater 24 h fat oxidation (milligram fat oxidation per kilogram FFM) than men, regardless of physical activity levels. Women in the study also had an enzymatic profile that favored β-oxidation in muscle (23). A review by Henderson (24) concluded that men oxidize more fat than women in the post-exercise state provided that they remain fasting. However, if food is ingested, this difference is no longer present. Additionally, with aging, changes in sex steroid concentrations may impact fat oxidation, as fat oxidation (rather than glucose oxidation) was observed to decrease in proportion to energy expenditure (21). Hober et al. (25) showed that with increasing age in males, resting fat oxidation (gram per kilogram lean mass) decreases whereas in females, no relationship was observed between resting fat oxidation and age. Thus, fluctuations in sex hormones as a result of age may be partially responsible for differences in fat oxidation between men and women.
Very Low-Density Lipoprotein TAG Kinetics
In the fasting state, most circulating plasma TAG are in VLDL, which are synthesized and secreted by the liver (26). Similar to chylomicron-TAG, VLDL-TAG are hydrolyzed by LPL residing on the capillary endothelium, making FA available to the underlying tissue for energy or storage. It is well established that men have higher circulating VLDL-TAG than age-matched women (27), but the etiology of this sex difference is not completely understood. Lean women have both greater VLDL-TAG secretion and clearance compared with lean men (28), which causes us to favor greater relative removal of VLDL-TAG as the primary explanation. In obesity, increased rates of VLDL-TAG secretion and decreased rates of VLDL-TAG clearance in men vs. women have been reported (28, 29).
Meal Fatty Acid Storage
Patterns of meal FA storage only somewhat mirror the patterns of regional fat distribution. We initially found no differences in patterns of meal FA storage in men and women; more meal FAs were stored (per gram lipid) in upper body SAT than in leg SAT in both groups (30–32). However, following consumption of a high-fat meal, women stored a greater proportion of meal fat in lower body SAT whereas men did not (33). Moreover, when meal FA storage was compared in men and women with different obesity phenotypes, variations in regional meal fat storage were found (34). In women with lower body obesity, meal FA storage was greater in gluteal than abdominal fat (34), whereas storage was similar in these two depots in upper body obese men and women. Furthermore, upper body obese men stored a lesser percent of meal fat in subcutaneous fat than either group of women (34). This suggests that meal FA storage may play a role in determining the sex differences in regional adiposity.
Direct FFA Storage
Even more so than meal FA storage, patterns of circulating FFA storage (termed, “direct FFA storage”) correspond to the sexual dimorphism in fat distribution. When the direct FFA storage pathway was first reported, FFA storage into SAT was consistent with body shape in men and women. More specifically, direct FFA storage in SAT was less in men than women, and men stored more FFA in abdominal vs. femoral SAT depots whereas women did not (35). Follow-up studies confirmed these results and noted that FFA storage rates were greater in femoral than abdominal SAT in women (36). In women with varying regional fat distribution, direct storage of FFA also followed regional fat distribution (37). These studies support a role of direct FFA storage as a means of redistributing FAs in a manner that appears to influence body shape.
Lipolysis
If rates of lipolysis were a major contributor toward sex differences in AT deposition, we would expect sex differences in the relative rates of total and regional lipolysis in men compared to women. For example, since women have greater lower body fat mass, lower rates of leg adipose tissue lipolysis would be observed in women compared with men. However, we found that the opposite is true. We found that leg adipose tissue lipolysis rates were 44 μmol/min in lean and 107 μmol/min in obese men, and 61 μmol/min in lean and 107 μmol/min in obese women. Thus, lipolysis in leg SAT of men and women was approximately equal, and even a little higher in lean women vs. men (38). Moreover, studies collectively show that regardless of sex, upper body AT is more lipolytically active than lower body (38–42). Therefore, lipolysis does not appear to explain the sexual dimorphism seen in body fat distribution.
The Effect of Estrogen and Testosterone on Lipid Metabolism
The sexual dimorphism in adiposity along with differences in fat metabolism found in men and women allude to a compelling role of sex steroids in determining regional fat distribution. Several studies have examined the effects of sex steroid suppression and/or supplementation on fat metabolism. Table 1 summarizes what is known about the changes in lipid metabolism that occurs with sex steroid suppression in men and women.
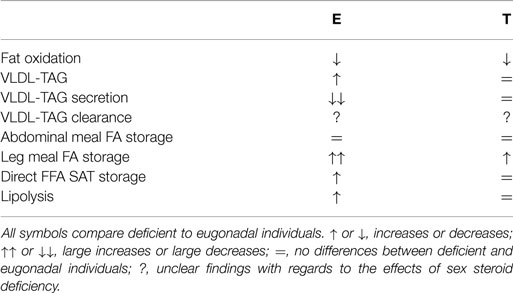
Table 1. Changes in lipid metabolism as a result of estrogen deficiency in women and testosterone deficiency in men relative to eugonadal women and men, respectively.
Fat Oxidation
Periodic 24 h measurements over a 4-year period showed that fat oxidation did not change in premenopausal women, whereas in postmenopasual women, fat oxidation decreased 32% (43). However, significant changes in body composition and diet may have affected the changes in fat oxidation in these women (43). We were able to exclude differences in body composition as the factor influencing fat oxidation in pre- vs. postmenopausal women by studying two groups of women matched for age, BMI, and body fat (44). We found that postprandial fat oxidation was indeed lower in postmenopausal women (44), confirming that hypogonadism/estrogen deficiency reduces fat oxidation, at least during a portion of the day. Whether the opposite is true, i.e. whether estrogen repletion increases fat oxidation, is unclear. When transdermal estrogen is provided to postmenopausal women over 6 months, neither changes in body composition nor changes in fat oxidation were observed (45). However, another study that supplemented estrogen transdermally over a 12-month period observed increases in lipid oxidation. These women had an increase in lean mass and no changes in fat mass over the 12-month estrogen repletion period (46). The different results in these studies could stem from the changes in body composition that occurred over the treatment period. More studies are required to tease out whether estrogen repletion in women affects fat oxidation.
We believe the balance of data suggests that testosterone increases fat oxidation in men. One study showed that acute hypogonadism did not change fat oxidation over a 3-week period compared to men replete with estrogen and/or testosterone (47). In contrast, Host et al. (48) showed that 1 month of hypogonadism decreased fat oxidation. This finding was supported by another study, which observed that hypogonadism over 10 weeks decreased rates of fat oxidation; however, decreases in fat free and increases in fat mass may partially explain these changes (49). To address the potential effect of body composition on fat oxidation, we matched chronically hypogonadal males for age and body composition and found lower fat oxidation in hypogonadal vs. eugonadal males (50). Four months of testosterone treatment in adolescent males with delayed puberty resulted in an increase in fat oxidation (51). Although 2 years of transdermal testosterone supplementation in elderly men with modestly reduced testosterone did not change body composition or meal fat oxidation, these men were not truly hypogonadal (52).
VLDL-TAG Kinetics
Studies examining the effects of sex hormones on VLDL-TAG kinetics in men and women do not appear to clarify the lower VLDL-TAG concentrations in women. VLDL-TAG secretion in premenopausal women was found to be twice that of postmenopausal women despite higher VLDL-TAG concentrations in postmenopausal women (29). In another study, women treated with estradiol vs. progesterone vs. testosterone vs. control were compared (53). VLDL-TAG kinetics did not change with placebo, or progesterone or testosterone treatment but clearance increased in the estrogen-treated group in conjunction with decreased VLDL-TAG concentrations (53). There were no differences in VLDL-TAG secretion in any of the groups of the study (53). The finding that testosterone supplementation in women did not affect VLDL kinetics was consistent with that of another study by the same group that supplemented testosterone in obese women for 3 weeks (54). In men, 1 month of hypogonadism and acute testosterone repletion to physiological levels did not affect VLDL-TAG secretion and concentrations (48).
Meal FA Storage
Few studies have been conducted to examine the effects of estrogen on meal FA storage. We found that postmenopausal had greater meal FA storage than premenopausal women (44). We also found that, compared to premenopausal women, meal FA storage in the femoral depot was two times greater in postmenopausal women (44). Another study that used LPL as a surrogate for meal FA storage observed that in postmenopausal women treated with estrogen and progesterone, LPL activity was higher in femoral vs. abdominal adipocytes (55). These studies indicate that estrogen affects meal FA storage in such a way as to promote meal fat storage in lower body fat in women. Why postmenopausal women would thus tend to gain abdominal fat is unclear.
There have been a limited number of studies on the effects of acute or chronic testosterone hormonal manipulation on meal FA metabolism. We found that chronically hypogonadal men stored more meal FA in the femoral region than eugonadal men (50). Whereas meal FA storage (milligram per gram lipid) in hypogonadal men was similar in the abdominal and femoral region, eugonadal men tended to store more meal FA in the abdominal than thigh region (50). These patterns of fat storage would be consistent with what we would expect given the regional differences in fat distribution between hypogonadal and eugonadal men. Thus, testosterone likely affects regional adiposity through its effects on regional meal FA storage. We did find that 2 years of testosterone supplementation of older men with somewhat reduced testosterone concentrations increased meal FA storage in the abdominal vs. femoral region (52). In another study, acute (5 days) of testosterone treatment decreased meal FA storage in VAT (30). In contrast, Marin et al. (31) found at baseline that more meal FA was stored in the abdominal vs. femoral depot and that after 9 months of testosterone treatment, these differences in meal FA storage no longer existed (31). This shift was likely because of a significant decrease in meal FA storage in abdominal SAT with T treatment. Potential reasons for this contradictory finding could stem from differences in ages of men between studies, differences in the testosterone treatment period, or differences in the timing of biopsies after ingestion of the experimental meal (56).
Direct FFA Storage
We matched pre- and postmenopausal women for age and body composition and found that direct FFA storage was greater in postmenopausal than premenopausal women (44). However, we found no significant regional differences in the storage of FFA between these two groups (44). A closer examination of the key factors involved in FFA storage showed that activity of ACS and DGAT were significantly upregulated in postmenopausal compared to premenopausal women indicating greater potential for FFA storage in postmenopausal women. In a second study, we found no differences in the rates of FFA storage between age and body composition match men who were chronically hypogonadal and eugonadal (50). However, activity of ACS was significantly greater in femoral adipose tissue of eugondal than hypogonadal men. The effects of sex steroids on direct FFA storage remains to be investigated before a conclusion can be drawn.
Lipolysis
Although regional differences in the relative rates of lipolysis are unlikely to be central in determining regional fat differences in men and women, there are major differences in rates of lipolysis relative to energy expenditure (38, 39). The rates of FFA release are ~40% greater in women than men relative to energy needs (38), despite similar relative rates of fat oxidation. Studies that manipulate sex steroids in humans support this conclusion. Contrary to what would be expected, decreases in sex steroids increase lipolysis whereas no differences in lipolysis occur with hormone treatment. Estrogen deficiency in women resulted in a 10–20% increase in lipolysis whereas estrogen repletion did not affect adrenergic stimulated lipolysis (57). This finding is consistent with that of another study, which showed that estrogen and progesterone treatment in postmenopausal women did not affect lipolysis (55).
Testosterone treatment has been shown to decrease total and abdominal fat mass and thus, would be expected to increase lipolysis. Testosterone treatment has been shown to stimulate lipolysis in the abdominal region (55) and in VAT (58). However, these cited studies involved shorter treatment periods. Another short-term study found that 7–10 days of hypogonadism and acute testosterone rescue did not have any effects on indicators of adipose tissue lipolysis (48). These findings are consistent with a longer 2-year period of testosterone treatment in hypogonadal men that did not detect any changes in lipolysis from upper or lower body SAT (52). Moreover, whole-body lipolysis was also found to be unchanged in adolescent males after 4 months of testosterone treatment (51).
Conclusion
The objective of this review was to describe the differences in lipid metabolism between males and females and to link, when possible, these differences to how they may cause or create the differences in body shape. Subsequently, we also sought to explore how sex steroids regulate this relationship. Fat oxidation, lipolysis, VLDL-TAG, meal FA metabolism, and direct FFA storage may collectively contribute toward total and regional adiposity. Several studies have been conducted to examine these aspects of fat distribution in the context of differences in sex and sex steroids. Regional meal FA and direct FFA storage appear to contribute toward the sexual dimorphism in fat distribution. While the extent to which fat oxidation and VLDL-TAG kinetics contribute to body composition are unclear, it is evident that lipolysis has little effect in contributing toward differences in body shape between men and women.
Conflict of Interest Statement
The authors declare that the research was conducted in the absence of any commercial or financial relationships that could be construed as a potential conflict of interest.
Acknowledgments
This work was funded by a Natural Science and Engineering Research Council of Canada Discovery Grant 418323-2012, and National Institutes of Health grants DK40484 and DK45343. SS holds a Canada Research Chair, Tier II in Clinical Nutrition.
References
1. Jensen MD. Role of body fat distribution and the metabolic complications of obesity. J Clin Endocrinol Metab (2008) 93(11 Suppl 1):S57–63. doi: 10.1210/jc.2008-1585
2. Vague J. La differenciation sexuelle facteur determinent des formes de l’obesité. Presse Med (1947) 55(30):339.
3. Bray GA, Jablonski KA, Fujimoto WY, Barrett-Connor E, Haffner S, Hanson RL, et al. Relation of central adiposity and body mass index to the development of diabetes in the diabetes prevention program. Am J Clin Nutr (2008) 87(5):1212–8.
4. Goodpaster BH, Thaete FL, Simoneau JA, Kelley DE. Subcutaneous abdominal fat and thigh muscle composition predict insulin sensitivity independently of visceral fat. Diabetes (1997) 46(10):1579–85. doi:10.2337/diabetes.46.10.1579
5. Kannel WB, Cupples LA, Ramaswami R, Stokes J III, Kreger BE, Higgins M. Regional obesity and risk of cardiovascular disease; the Framingham study. J Clin Epidemiol (1991) 44(2):183–90. doi:10.1016/0895-4356(91)90265-B
6. Donahue RP, Abbott RD. Central obesity and coronary heart disease in men. Lancet (1987) 2(8569):821–4. doi:10.1016/S0140-6736(87)91605-9
7. Cassano PA, Segal MR, Vokonas PS, Weiss ST. Body fat distribution, blood pressure, and hypertension. A prospective cohort study of men in the normative aging study. Ann Epidemiol (1990) 1(1):33–48. doi:10.1016/1047-2797(90)90017-M
8. Vgontzas AN, Papanicolaou DA, Bixler EO, Hopper K, Lotsikas A, Lin HM, et al. Sleep apnea and daytime sleepiness and fatigue: relation to visceral obesity, insulin resistance, and hypercytokinemia. J Clin Endocrinol Metab (2000) 85(3):1151–8. doi:10.1210/jcem.85.3.6484
9. Britton KA, Massaro JM, Murabito JM, Kreger BE, Hoffmann U, Fox CS. Body fat distribution, incident cardiovascular disease, cancer, and all-cause mortality. J Am Coll Cardiol (2013) 62(10):921–5. doi:10.1016/j.jacc.2013.06.027
10. Pischon T, Boeing H, Hoffmann K, Bergmann M, Schulze MB, Overvad K, et al. General and abdominal adiposity and risk of death in Europe. N Engl J Med (2008) 359(20):2105–20. doi:10.1056/NEJMoa0801891
12. Lemieux S, Prud’homme D, Bouchard C, Tremblay A, Despres JP. Sex differences in the relation of visceral adipose tissue accumulation to total body fatness. Am J Clin Nutr (1993) 58(4):463–7.
13. Hajri T, Abumrad NA. Fatty acid transport across membranes: relevance to nutrition and metabolic pathology. Annu Rev Nutr (2002) 22:383–415. doi:10.1146/annurev.nutr.22.020402.130846
14. Hames KC, Vella A, Kemp BJ, Jensen MD. Free fatty acid uptake in humans with CD36 deficiency. Diabetes (2014) 63:3606–14. doi:10.2337/db14-0369
15. Mashek DG, Li LO, Coleman RA. Long-chain acyl-CoA synthetases and fatty acid channeling. Future Lipidol (2007) 2(4):465–76. doi:10.2217/17460875.2.4.465
16. Yen CL, Stone SJ, Koliwad S, Harris C, Farese RV Jr. Thematic review series: glycerolipids. DGAT enzymes and triacylglycerol biosynthesis. J Lipid Res (2008) 49(11):2283–301. doi:10.1194/jlr.R800018-JLR200
17. Lass A, Zimmermann R, Oberer M, Zechner R. Lipolysis – a highly regulated multi-enzyme complex mediates the catabolism of cellular fat stores. Prog Lipid Res (2011) 50(1):14–27. doi:10.1016/j.plipres.2010.10.004
18. Ryden M, Jocken J, van Harmelen V, Dicker A, Hoffstedt J, Wiren M, et al. Comparative studies of the role of hormone-sensitive lipase and adipose triglyceride lipase in human fat cell lipolysis. Am J Physiol Endocrinol Metab (2007) 292(6):E1847–55. doi:10.1152/ajpendo.00040.2007
19. Weyer C, Snitker S, Rising R, Bogardus C, Ravussin E. Determinants of energy expenditure and fuel utilization in man: effects of body composition, age, sex, ethnicity and glucose tolerance in 916 subjects. Int J Obes Relat Metab Disord (1999) 23(7):715–22. doi:10.1038/sj.ijo.0800910
20. Santosa S, Jensen MD. Why are we shaped differently, and why does it matter? Am J Physiol Endocrinol Metab (2008) 295(3):E531–5. doi:10.1152/ajpendo.90357.2008
21. Levadoux E, Morio B, Montaurier C, Puissant V, Boirie Y, Fellmann N, et al. Reduced whole-body fat oxidation in women and in the elderly. Int J Obes Relat Metab Disord (2001) 25(1):39–44. doi:10.1038/sj.ijo.0801530
22. Calles-Escandon J, Driscoll P. Free fatty acid metabolism in aerobically fit individuals. J Appl Physiol (1994) 77(5):2374–9.
23. Melanson EL, Sharp TA, Seagle HM, Horton TJ, Donahoo WT, Grunwald GK, et al. Effect of exercise intensity on 24-h energy expenditure and nutrient oxidation. J Appl Physiol (2002) 92(3):1045–52. doi:10.1152/japplphysiol.00706.2001
24. Henderson GC. Sexual dimorphism in the effects of exercise on metabolism of lipids to support resting metabolism. Front Endocrinol (2014) 5:162. doi:10.3389/fendo.2014.00200
25. Horber FF, Gruber B, Thomi F, Jensen EX, Jaeger P. Effect of sex and age on bone mass, body composition and fuel metabolism in humans. Nutrition (1997) 13(6):524–34. doi:10.1016/S0899-9007(97)00031-2
26. Magkos F, Mittendorfer B. Gender differences in lipid metabolism and the effect of obesity. Obstet Gynecol Clin North Am (2009) 36(2):245–65. doi:10.1016/j.ogc.2009.03.001
27. Wang X, Magkos F, Mittendorfer B. Sex differences in lipid and lipoprotein metabolism: it’s not just about sex hormones. J Clin Endocrinol Metab (2011) 96(4):885–93. doi:10.1210/jc.2010-2061
28. Mittendorfer B, Patterson BW, Klein S. Effect of sex and obesity on basal VLDL-triacylglycerol kinetics. Am J Clin Nutr (2003) 77(3):573–9.
29. Magkos F, Fabbrini E, Mohammed BS, Patterson BW, Klein S, Mittendorfer B. Estrogen deficiency after menopause does not result in male very-low-density lipoprotein metabolism phenotype. J Clin Endocrinol Metab (2010) 95(7):3377–84. doi:10.1210/jc.2010-0341
30. Marin P, Lonn L, Andersson B, Oden B, Olbe L, Bengtsson BA, et al. Assimilation of triglycerides in subcutaneous and intraabdominal adipose tissues in vivo in men: effects of testosterone. J Clin Endocrinol Metab (1996) 81(3):1018–22. doi:10.1210/jc.81.3.1018
31. Marin P, Oden B, Bjorntorp P. Assimilation and mobilization of triglycerides in subcutaneous abdominal and femoral adipose tissue in vivo in men: effects of androgens. J Clin Endocrinol Metab (1995) 80(1):239–43. doi:10.1210/jcem.80.1.7829619
32. Votruba SB, Jensen MD. Short-term regional meal fat storage in nonobese humans is not a predictor of long-term regional fat gain. Am J Physiol Endocrinol Metab (2012) 302(9):E1078–83. doi:10.1152/ajpendo.00414.2011
33. Votruba SB, Jensen MD. Sex-specific differences in leg fat uptake are revealed with a high-fat meal. Am J Physiol Endocrinol Metab (2006) 291(5):E1115–23. doi:10.1152/ajpendo.00196.2006
34. Santosa S, Hensrud DD, Votruba SB, Jensen MD. The influence of sex and obesity phenotype on meal fatty acid metabolism before and after weight loss. Am J Clin Nutr (2008) 88(4):1134–41.
35. Shadid S, Koutsari C, Jensen MD. Direct free fatty acid uptake into human adipocytes in vivo: relation to body fat distribution. Diabetes (2007) 56(5):1369–75. doi:10.2337/db06-1680
36. Koutsari C, Ali AH, Mundi MS, Jensen MD. Storage of circulating free fatty acid in adipose tissue of postabsorptive humans: quantitative measures and implications for body fat distribution. Diabetes (2011) 60(8):2032–40. doi:10.2337/db11-0154
37. Sondergaard E, Gormsen LC, Nellemann B, Jensen MD, Nielsen S. Body composition determines direct FFA storage pattern in overweight women. Am J Physiol Endocrinol Metab (2012) 302(12):E1599–604. doi:10.1152/ajpendo.00015.2012
38. Nielsen S, Guo Z, Johnson CM, Hensrud DD, Jensen MD. Splanchnic lipolysis in human obesity. J Clin Invest (2004) 113(11):1582–8. doi:10.1172/JCI21047
39. Jensen MD. Gender differences in regional fatty acid metabolism before and after meal ingestion. J Clin Invest (1995) 96(5):2297–303. doi:10.1172/JCI118285
40. Jensen MD, Haymond MW, Rizza RA, Cryer PE, Miles JM. Influence of body fat distribution on free fatty acid metabolism in obesity. J Clin Invest (1989) 83(4):1168–73. doi:10.1172/JCI113997
41. Guo Z, Johnson CM, Jensen MD. Regional lipolytic responses to isoproterenol in women. Am J Physiol (1997) 273(1 Pt 1):E108–12.
42. Burguera B, Proctor D, Dietz N, Guo Z, Joyner M, Jensen MD. Leg free fatty acid kinetics during exercise in men and women. Am J Physiol Endocrinol Metab (2000) 278(1):E113–7.
43. Lovejoy JC, Champagne CM, de Jonge L, Xie H, Smith SR. Increased visceral fat and decreased energy expenditure during the menopausal transition. Int J Obes (Lond) (2008) 32(6):949–58. doi:10.1038/ijo.2008.25
44. Santosa S, Jensen MD. Adipocyte fatty acid storage factors enhance subcutaneous fat storage in postmenopausal women. Diabetes (2013) 62(3):775–82. doi:10.2337/db12-0912
45. O’Sullivan AJ, Crampton LJ, Freund J, Ho KK. The route of estrogen replacement therapy confers divergent effects on substrate oxidation and body composition in postmenopausal women. J Clin Invest (1998) 102(5):1035–40. doi:10.1172/JCI2773
46. dos Reis CM, de Melo NR, Meirelles ES, Vezozzo DP, Halpern A. Body composition, visceral fat distribution and fat oxidation in postmenopausal women using oral or transdermal oestrogen. Maturitas (2003) 46(1):59–68. doi:10.1016/S0378-5122(03)00159-2
47. Santosa S, Khosla S, McCready LK, Jensen MD. Effects of estrogen and testosterone on resting energy expenditure in older men. Obesity (Silver Spring) (2010) 18(12):2392–4. doi:10.1038/oby.2010.98
48. Host C, Gormsen LC, Christensen B, Jessen N, Hougaard DM, Christiansen JS, et al. Independent effects of testosterone on lipid oxidation and VLDL-TG production: a randomized, double-blind, placebo-controlled, crossover study. Diabetes (2013) 62(5):1409–16. doi:10.2337/db12-0440
49. Mauras N, Hayes V, Welch S, Rini A, Helgeson K, Dokler M, et al. Testosterone deficiency in young men: marked alterations in whole body protein kinetics, strength, and adiposity. J Clin Endocrinol Metab (1998) 83(6):1886–92. doi:10.1210/jcem.83.6.4892
50. Santosa S, Jensen MD. Effects of male hypogonadism on regional adipose tissue fatty acid storage and lipogenic proteins. PLoS One (2012) 7(2):e31473. doi:10.1371/journal.pone.0031473
51. Arslanian S, Suprasongsin C. Testosterone treatment in adolescents with delayed puberty: changes in body composition, protein, fat, and glucose metabolism. J Clin Endocrinol Metab (1997) 82(10):3213–20. doi:10.1210/jc.82.10.3213
52. Koutsari C, Ali AH, Nair KS, Rizza RA, O’Brien P, Khosla S, et al. Fatty acid metabolism in the elderly: effects of dehydroepiandrosterone and testosterone replacement in hormonally deficient men and women. J Clin Endocrinol Metab (2009) 94(9):3414–23. doi:10.1210/jc.2009-0165
53. Smith GI, Reeds DN, Okunade AL, Patterson BW, Mittendorfer B. Systemic delivery of estradiol, but not testosterone or progesterone, alters very low density lipoprotein-triglyceride kinetics in postmenopausal women. J Clin Endocrinol Metab (2014) 99(7):E1306–10. doi:10.1210/jc.2013-4470
54. Wang X, Smith GI, Patterson BW, Reeds DN, Kampelman J, Magkos F, et al. Testosterone increases the muscle protein synthesis rate but does not affect very-low-density lipoprotein metabolism in obese premenopausal women. Am J Physiol Endocrinol Metab (2012) 302(6):E740–6. doi:10.1152/ajpendo.00533.2011
55. Rebuffe-Scrive M, Lonnroth P, Marin P, Wesslau C, Bjorntorp P, Smith U. Regional adipose tissue metabolism in men and postmenopausal women. Int J Obes (1987) 11(4):347–55.
56. Jensen MD, Sarr MG, Dumesic DA, Southorn PA, Levine JA. Regional uptake of meal fatty acids in humans. Am J Physiol Endocrinol Metab (2003) 285(6):E1282–8. doi:10.1152/ajpendo.00220.2003
57. Jensen MD, Martin ML, Cryer PE, Roust LR. Effects of estrogen on free fatty acid metabolism in humans. Am J Physiol (1994) 266(6 Pt 1):E914–20.
Keywords: sex steroids, estrogen, testosterone, sex hormone, metabolism, fatty acid, lipids
Citation: Santosa S and Jensen MD (2015) The sexual dimorphism of lipid kinetics in humans. Front. Endocrinol. 6:103. doi: 10.3389/fendo.2015.00103
Received: 02 January 2015; Paper pending published: 23 February 2015;
Accepted: 13 June 2015; Published: 02 July 2015
Edited by:
Janne Lebeck, Danish Diabetes Academy, DenmarkReviewed by:
Marek Bolanowski, Wroclaw Medical University, PolandGordon Smith, Washington University, USA
Copyright: © 2015 Santosa and Jensen. This is an open-access article distributed under the terms of the Creative Commons Attribution License (CC BY). The use, distribution or reproduction in other forums is permitted, provided the original author(s) or licensor are credited and that the original publication in this journal is cited, in accordance with accepted academic practice. No use, distribution or reproduction is permitted which does not comply with these terms.
*Correspondence: Sylvia Santosa, Concordia University, Loyola Campus, 7141 Sherbrooke Street West, SP165.06, Montreal, QC H4B 1R6, Canada, s.santosa@concordia.ca