- 1Institute of Anatomy II, University of Frankfurt, Frankfurt am Main, Germany
- 2Dr. Senckenbergisches Chronomedizinisches Institut, University of Frankfurt, Frankfurt am Main, Germany
The overall staining by GS clearly reveals astrocytes, including all cells of the astroglial family (1), i.e., Bergmann glia, Müller cells (2), tanycytes (3), and ependymal cells. The star shaped morphology from classical silver impregnations relates to cortical and hippocampal astrocytes, which display a comparable pattern in material stained for GFAP. However, the dense population of GS stained astrocytes found in all diencephalic and mesencephalic regions, known to display faint GFAP-labeling (unpublished observations) indicated that while apparently all astrocytes contain GS they have GFAP-ir filaments only in a region-dependent pattern. This is complicated by the emerging view that “astrocytes” constitute a heterogeneous population even within a given region. In the rat hippocampus, combined immunostainings have revealed that the “classical” GFAP-ir astrocyte constitutes a subpopulation of GS-ir astrocytes, which can also lack GFAP staining [direct double staining (4)]. In view of several astroglial subtypes and/or glial precursors present in the adult rodent brain, anti-GS appears to be the most general astrocyte marker, covering all subtypes. In addition, GS has been found early on to label exclusively astrocytic cells and no other glial or neuronal cell types in situ or in culture [reviewed by (5)]. GS has, thus, been applied as a reliable astrocyte marker in very many studies since.
“Complex cells” in rat hippocampus, initially assumed to be an astrocyte subtype (6) but now understood to belong to NG2 cells, a fourth glial type in the CNS (7), may display faint GS-ir in the soma but not its fine processes. Oligodendrocyte precursor cells, possibly also related to NG2 cells, were found to be devoid of GS-ir (8). The immunocytochemical profile and possible heterogeneity of NG2 cells is still under debate to date. Disputing the exclusion of non-astrocytic cells in GS staining, some authors have later reported GS+ oligodendrocytes, although this has not been investigated systematically. Reports on non-astrocytic GS will be discussed in detail here.
Oligodendrocytes
The authors observing oligodendroglial GS localization rely mostly on the non-convincing morphology of “ovoid cells” in the gray matter, and only sometimes on the unambiguous alignment of interfascicular oligodendrocytes. Only one study is based on GS mRNA in situ hybridization (GS exclusively in astrocytes), and three studies on colocalization of GS-ir with oligodendroglial markers (see below). The reports on GS-ir in oligodendrocytes by three groups (9–11) can, however, not be reconciled, and might result from the use of different antisera and/or divergent interpretations of morphology. Thus, Cammer (9), applying a proprietary anti-sheep brain GS, observed clearly intrafascicular oligodendrocytes, but only faint white matter astrocytes in rat spinal chord. A similar pattern was evident in rat forebrain white matter (proprietary GS antiserum; specimens prepared by Dr. M. Lavialle). Anti rat liver GS (9) produced the most convincing intrafascicular oligodendrocytes displaying also immunoreactivity for CNPase, an established oligodendrocyte marker. However, in gray matter, oligodendrocytes but hardly astrocytes were detectable by anti-GS. Based on a different rabbit anti-sheep brain GS antiserum in cat brain, GS localization was found in an inverse relation, i.e., only in gray matter oligodendrocytes but not interfascicular oligodendrocytes (10). These cells were identified by light microscopic morphological criteria, most of them in perineuronal position. A localization of GS in gray (but not white) matter oligodendrocytes, mostly perineuronal and perivascular was confirmed by plausible ultrastructural criteria, using another rabbit anti-sheep brain GS in the mouse brain (11). In this context, the absence of a typical light microscopical pattern distinguishing astrocytes from oligodendrocytes in perineuronal position in cortex or hippocampus, and generally in non-telencephalic regions (where astrocytes are generally non-stellate) might be relevant. In these regions, the GS-ir gray matter oligodendrocytes observed by Miyake and Kitamura (11) were particularly abundant but “astrocytes” were hardly observed. GS-ir perineuronal oligodendrocytes were present in addition to astrocytes also in the cortex but not in the hippocampus, which would imply subclasses of perineuronal oligodendrocytes. Similarly, the figures provided by (10), of GS-ir gray matter oligodendrocytes in the cortex (perineuronal) and cerebellum (around Purkinje cells,) do not allow for clear differentiation from astrocytes or Bergmann glia. This applies particularly to the cerebellum where even an “oligodendrocyte-like astrocyte” has been described (12). In contrast, the GS-ir “ovoid cells” in the lizard mesencephalon were interpreted as astrocytic, since they were in alignment with radial glial fibers, and forming perivascular end feet (13). Non-astrocytic labeling by anti-GS might also be associated with technical difficulties. Thus, Werner et al. (14), although colocalizing GS with CNPase in oligodendrocytes, depict GS-ir in the nucleus or putative perinuclear cytoplasm, but not in processes. They also find positive microglia, which has never been reported before, and would normally represent a negative control. Similarly, in the report on GS staining in over 50% of CNPase positive perineuronal oligodendrocytes (15), the GS-staining was not seen within the typical, ring-like CNPase+ cell rim (as clearly shown for several other markers in the same publication). Although applying confocal microscopy, the images show occasional 3D overlay of incongruent shapes in the two channels, which might lead to misinterpretation particularly since the authors did not consider neighboring, perineuronal astrocytes. In cell culture, where glial cell type purity and technical preparation may lead to diverging results, GS can be induced in cells that are normally not GS+, such as fibroblasts (16) or even chick brain neurons, which are GS− negative in vivo (17). GS induction in cultured oligodendrocytes, by corticoids or thyroid hormones was observed by some (18) but not others (17).
As evidence in favor of an astrocyte-restricted GS localization [reviewed by (5)], absence of oligodendrocyte labeling in white and gray matter has been reported by the group of Norenberg, in particular at the ultrastructural level (19, 20), and the group of Derouiche, who investigated vibratome sections from human (21), and rat (3), using a previously characterized anti-GS antiserum (16) or commercial GS antibodies (Chemicon-Millipore, Billerica, MA, USA: clone GS-6, MAB 302; Santa Cruz Biotechnology, CA, USA, Ab: sc-6640; unpublished observations). In particular, GS-ir in the conspicuous interfascicular oligodendrocytes would not have been overlooked in the studies mentioned, and very many others. Based on distribution and morphology, localization of GS mRNA in rat brain, although without cell identification, was in line with exclusive astrocytic labeling (22).
The clear distinction by GS-ir between astroglial cells and oligodendrocytes would be maintained in the tumors derived from these cells, since all astrocytomas and ependymomas but none of the oligodendrogliomas were GS+ (23). Another astrocytic antigen, ezrin, which labels predominantly the fine, peripheral astrocyte processes of all astrocytic cells but not oligodendrocytes (24), has a corresponding, clear-cut specificity within the range of human glial tumors (25).
Neurons
Neuronal localization of GS has been undisputedly excluded by all studies, apart from two reports on human autoptic material from normal subjects, and individuals suffering from Alzheimer’s disease (26, 27). In addition to astrocytes, the perikarya particularly of pyramidal cells were intensely labeled. Labeled neurons were observed in 2 (of 7) normal brains, and were highly variable in localization and quantity over cortical fields and layers also in all 10 cases from Alzheimer demented subjects (27). However, previous evidence and technical considerations suggest that these observations should be taken cum grano salis. Although there is no animal model for Alzheimer’s disease, neuronal localization has not been reported in any of the many experimental neuropathology studies employing GS-ir. The finding also contrasts with previous human data (21) (4 cases), (23) (15), (28) (17). The only neuronal localization of GS in situ has been reported in a proteomic analysis of squid optic lobe synaptosomes, a definitely glia-free preparation (29). However, neuronal labeling similar to that reported (26, 27) has been observed in sections from rat brain (perfusion or immersion-fixed, vibratome) or human hippocampus (vibratome, paraffin), applying various anti-GS antisera in two laboratories (author’s unpublished observations; Dr. M. Lavialle, personal communication). This neuronal labeling was regarded as spurious, since it occurred inconsistently after storage (exceeding 1 week), often without the expected glial staining, even in vibratome sections from the same block that has yielded the exclusive astroglial pattern in staining runs before. This might indicate the recognition of distinct epitopes displaying independent physicochemical properties. Interestingly, the anti-GS mAb (Chemicon) also used by (27, 28) has been found to cross reacts with a “GS-like protein” different from GS (30), however, its cellular localization in the brain has not been established.
Subcellular GS Localization
Anti-GS has been found to represent an ultrastructural marker completely “filling” astrocytic cytoplasm in situ, well suited to mark also the extremely fine glial processes (20, 31). However, it was noted that labeling might also be associated with vesicles (5), which could not be verified in the stainings based on the diffusible chromogen DAB (5). We further investigated this applying high-resolution fluorescence microscopy and deconvolution in primary astrocyte culture (32). Based on negative controls (Figures 1A–J), GS-ir using a polyclonal antibody made in rabbit (16) was concentrated in discrete structures resembling vesicles (Figures 1K–O), which were also double-labeled applying different GS antibodies simultaneously (Figures 1K,N). Note that there is no full “colocalization,” i.e., full pixel superimposition of green and red channels. With the individual organelle as the unit of observation, however, the sparse, mostly incoherent pixels of the green channel [goat anti-GS (Santa Cruz sc-6640)] are mostly associated with the discrete, vesicle-like structures of the red channel (Figures 1K,N). This finding, possibly resulting from differential labeling efficiencies of the antibodies, can be referred to as vesicular colocalization. These double-labeled vesicular structures were present throughout the cell but concentrated at limited stretches of the cell boundaries (arrows in Figures 1K,L, also in Figures 1E,H,J), they were frequently arranged in rows (arrows in Figures 1M,O), and their structure as far as could be resolved was non-uniform, pleomorph (Figures 1M,O).
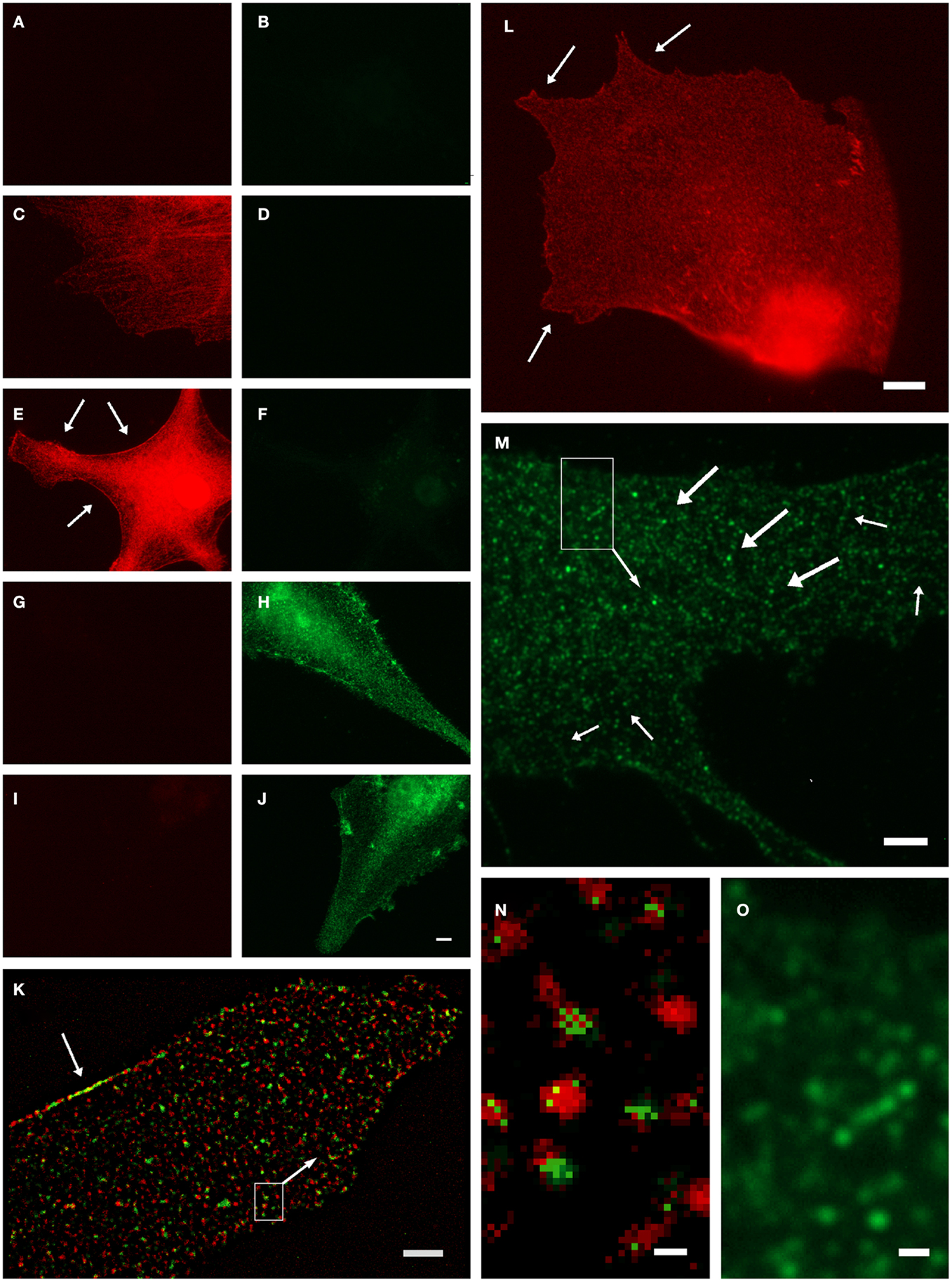
Figure 1. Organelle-bound localization of GS-immunoreactivity, in primary culture of rat cortical astrocytes using the following anti-GS antibodies: (1) polyclonal made in rabbit (15), or (2) goat (Santa Cruz sc-6640), or (3) mouse monoclonal (Chemicon-Millipore, Billerica, MA, USA; clone GS-6, MAB 302). (A–J) Controls for double-labeling (red and green) with two anti-GS antibodies, red channel always in left, green in right column. (A,B) Control for autofluorescence, no immunoreagents. (C,D) Control for fluorescence red-to-green bleed through: Single staining anti-GS (1) with secondary anti-rabbit antibody (red). (E,F) Control for detection system of green channel: Same as in (C,D), in addition secondary anti-mouse antibody (green). (G,H) Control for fluorescence green-to-red bleed through: Single staining anti-GS (3) with secondary anti-mouse antibody (green). (I,J) control for detection system of red channel: Same as in (G,H), in addition anti-rabbit antibody (red). (K) Double-labeling by antibodies (1) and (2) coincides on the same organelles, even at high magnification [(N), from inset in (K)]. Antibody (1), red channel, labels the complete outline of organelles, whereas antibody (2), green channel only yields pixels within the extent of individual red labeled structures. Optical section 100 μm thick, after deconvolution. Single labeling by antibodies (3) (L) or (2) [(M,O), from inset] yields comparable organelles. Scale 5 μm [in (J), for (A–J)], 3 μm (K,M), 4 μm (L), 0.25 μm (N), 0.5 μm (O).
While these observations do not necessarily exclude the commonly assumed cytosolic presence of GS, they clearly suggest a vesicle-bound form. GS might be indirectly linked to vesicular membranes, as is known, e.g., from the glutamic acid decarboxylase isoform GAD65, which is attached to transmitter vesicles via the vesicular GABA transporter, to support neurotransmission (33). It will be interesting to further investigate whether these findings are important in the context of metabolic compartmentation, in particular in relation to glial glutamate uptake, metabolism, and release (34). Similarly, how would the GS+ vesicles relate to those involved in vesicular exocytosis of glutamate from astrocytes?
References
1. Fedoroff S. Prenatal ontogenesis of astrocytes. In: Fedoroff S, Vernadakis A editors. Astrocytes. Development, Morphology, and Regional Specialization of Astrocytes (Vol. 1), Orlando, FL: Academic Press (1986). p. 35–74.
2. Riepe RE, Norenberg MD. Müller cell localization of glutamine synthetase in rat retina. Nature (1977) 286:654–5. doi: 10.1038/268654a0
3. Derouiche A. “Coupling of glutamate uptake and degradation in transmitter clearance: anatomical evidence.” In: Pögün S, Parnas I, editors. Neurotransmitter Release and Uptake (NATO ASI Series, Series H: Cell Biology Series) Berlin, Heidelberg, New York: Springer (1997). p. 263–83.
4. Walz W, Lang MK. Immunocytochemical evidence for a distinct GFAP-negative subpopulation of astrocytes in the adult rat hippocampus. Neurosci Lett (1998) 257:127–30. doi:10.1016/S0304-3940(98)00813-1
5. Norenberg MD. Immunohistochemistry of glutamine synthetase. In: Hertz L, Kvamme E, McGeer EG, Schousboe A, editors. Glutamine, Glutamate, and GABA in the Central Nervous System. New York, NY: Alan R. Liss (1983). p. 95–111.
6. Akopian G, Kressin K, Derouiche A, Steinhäuser C. Identified glial cells in the early postnatal mouse hippocampus display different types of Ca2+ currents. Glia (1996) 17:181–94. doi:10.1002/(SICI)1098-1136(199607)17:3<181::AID-GLIA1>3.3.CO;2-Z
7. Ong WY, Levine JM. A light and electron microscopic study of NG2 chondroitin sulfate proteoglycan-positive oligodendrocyte precursor cells in the normal and kainate-lesioned rat hippocampus. Neuroscience (1999) 92:83–95. doi:10.1016/S0306-4522(98)00751-9
8. Reynolds R, Hardy R. Oligodendroglial progenitors labeled with the O4 antibody persist in the adult rat cerebral cortex in vivo. J Neurosci Res (1997) 47:455–70. doi:10.1002/(SICI)1097-4547(19970301)47:5<455::AID-JNR1>3.0.CO;2-G
9. Cammer W. Glutamine synthetase in the central nervous system is not confined to astrocytes. J Neuroimmunol (1990) 26:173–8. doi:10.1016/0165-5728(90)90088-5
10. D’Amelio F, Eng LF, Gibbs MA. Glutamine synthetase immunoreactivity is present in ologodendroglia of various regions of the central nervous system. Glia (1990) 3:335–41. doi:10.1002/glia.440030504
11. Miyake T, Kitamura T. Glutamine synthetase immunoreactivity in two types of mouse brain glial cells. Brain Res (1992) 586:53–60. doi:10.1016/0006-8993(92)91370-T
12. Palay SL, Chan-Palay V. Cerebellar Cortex, Cytology and Organization. New York: Springer (1974).
13. Monzon-Mayor M, Yanes C, Tholey G, De Barry J, Gombos G. Immunohistochemical localozation of glutamine synthetase in mesencephalon and telencephalon of the lizard Gallotia Galloti during ontogeny. Glia (1990) 3:81–97. doi:10.1002/glia.440030202
14. Werner P, Pitt D, Raine CS. Multiple sclerosis: altered glutamate homeostasis in lesions correlates with oligodendrocyte and axonal damage. Ann Neurol (2001) 50:169–80. doi:10.1002/ana.1077
15. Takasaki C, Yamasaki M, Uchigashima M, Konno K, Yanagawa Y, Watanabe M. Cytochemical and cytological properties of perineuronal oligodendrocytes in the mouse cortex. Eur J Neurosci (2010) 32:1326–36. doi:10.1111/j.1460-9568.2010.07377.x
16. Hallermayer K, Hamprecht B. Cellular heterogeneity in primary cultures of brain cells revealed by immunocytochemical localisation of glutamine synthetase. Brain Res (1984) 295:1–11. doi:10.1016/0006-8993(84)90810-2
17. Tholey G, Ghandour MS, Bloch S, Ledig M, Mandel P. Glutamine synthetase and energy metabolism in cultivated chick neurons and astrocytes: modulation by serum and hydrocortisone. Brain Res (1987) 428:73–81.
18. Baas D, Fressinaud C, Vitkovic L, Sarlieve LL. Glutamine synthetase expression and activity are regulated by 3,5,3′-triodo-L-thyronine and hydrocortisone in rat oligodendrocyte cultures. Int J Dev Neurosci (1998) 16:333–40. doi:10.1016/S0736-5748(98)00040-9
19. Norenberg MD. The distribution of glutamine synthetase in the rat central nervous system. J Histochem Cytochem (1979) 27:756–62. doi:10.1177/27.3.39099
20. Norenberg MD, Martinez-Hernandez A. Fine structural localization of glutamine synthetase in astrocytes of rat brain. Brain Res (1979) 161:303–10. doi:10.1016/0006-8993(79)90071-4
21. Derouiche A, Ohm TG. Glutamine synthetase immunoreactivity in the human hippocampus is lamina-specific. Neurosci Lett (1994) 165:179–82. doi:10.1016/0304-3940(94)90739-0
22. Mearow KM, Mill JF, Vitkovic L. The ontogeny and localization of glutamine synthetase gene expression in rat brain. Brain Res Mol Brain Res (1989) 6:223–32. doi:10.1016/0169-328X(89)90068-5
23. Pilkington GJ, Lantos PL. The role of glutamine synthetase in the diagnosis of cerebral tumours. Neuropathol Appl Neurobiol (1982) 8:227–36. doi:10.1111/j.1365-2990.1982.tb00277.x
24. Derouiche A, Frotscher M. Peripheral astrocyte processes: monitoring by selective immunostaining for the actin-binding ERM proteins. Glia (2001) 36:330–41. doi:10.1002/glia.1120
25. Geiger K, Stoldt P, Schlote W, Derouiche A. Ezrin immunoreactivity is associated with increasing malignancy of astrocytic tumors but is absent in oligodendrogliomas. Am J Pathol (2000) 157:1785–93. doi:10.1016/S0002-9440(10)64816-X
26. Robinson SR. Neuronal expression of glutamine synthetase in Alzheimer’s disease indicates a profound impairment of metabolic interactions with astrocytes. Neurochem Int (2000) 36:471–82. doi:10.1016/S0197-0186(99)00150-3
27. Robinson SR. Changes in the cellular distribution of glutamine synthetase in Alzheimer’s disease. J Neurosci Res (2001) 66:972–80. doi:10.1002/jnr.10057
28. Smith DA, Lantos PL. Immunocytochemistry of cerebellar astrocytomas: with a special note on Rosenthal fibres. Acta Neuropathol (1985) 66:155–9. doi:10.1007/BF00688691
29. Jiménez CR, Eyman M, Lavina ZS, Gioio A, Li KW, van der Schors RC, et al. Protein synthesis in synaptosomes: a proteomics analysis. J Neurochem (2002) 81:735–44. doi:10.1046/j.1471-4159.2002.00873.x
30. Boksha I, Tereshkina EB, Burbaeva GS. Glutamine synthetase and glutamine synthetase-like protein from human brain: purification and comparative characterization. J Neurochem (2000) 75:2574–82.
31. Derouiche A, Frotscher M. Astroglial processes around identified glutamatergic synapses contain glutamine synthetase: evidence for transmitter degradation. Brain Res (1991) 552:346–50. doi:10.1016/0006-8993(91)90103-3
32. Anlauf E, Derouiche A. A practical calibration procedure for fluorescence colocalization at the single organelle level. J Microsc (2009) 233:225–33. doi:10.1111/j.1365-2818.2009.03112.x
33. Jin H, Wu H, Osterhaus G, Wei J, Davis K, Sha D, et al. Demonstration of functional coupling between gamma-aminobutyric acid (GABA) synthesis and vesicular GABA transport into synaptic vesicles. Proc Natl Acad Sci U S A (2003) 100:4293–8. doi:10.1073/pnas.0730698100
Keywords: astrocyte, oligodendrocyte, glutamate metabolism, immunocytochemistry, deconvolution
Citation: Anlauf E and Derouiche A (2013) Glutamine synthetase as an astrocytic marker: its cell type and vesicle localization. Front. Endocrinol. 4:144. doi: 10.3389/fendo.2013.00144
Received: 19 September 2013; Accepted: 25 September 2013;
Published online: 16 October 2013.
Edited by:
Leif Hertz, China Medical University, ChinaReviewed by:
Leif Hertz, China Medical University, ChinaCopyright: © 2013 Anlauf and Derouiche. This is an open-access article distributed under the terms of the Creative Commons Attribution License (CC BY). The use, distribution or reproduction in other forums is permitted, provided the original author(s) or licensor are credited and that the original publication in this journal is cited, in accordance with accepted academic practice. No use, distribution or reproduction is permitted which does not comply with these terms.
*Correspondence: derouiche@em.uni-frankfurt.de