- Laboratory for Cellular and Molecular Thyroid Research, Division of Endocrinology and Metabolism, The Johns Hopkins University School of Medicine, Baltimore, MD, USA
Aberrant gene methylation is often seen in thyroid cancer, a common endocrine malignancy. Tobacco smoking has been shown to be associated with aberrant gene methylation in several cancers, but its relationship with gene methylation in thyroid cancer has not been examined. In the present study, we investigated the relationship between smoking of patients and aberrant methylation of tumor suppressor genes for TIMP3, SLC5A8, death-associated protein kinase, and retinoic acid receptor β2 (RARβ2) in papillary thyroid cancer (PTC), the most common type of thyroid cancer. The promoter methylation status of these genes was analyzed using quantitative real-time methylation-specific PCR on bisulfite-treated genomic DNA isolated from tumor tissues and correlated with smoking history of the patients. Among the four genes, methylation of the RARβ2 gene was significantly associated with smoking and other three genes showed a trend of association. Specifically, among the 138 patients investigated, 13/42 (31.0%) ever smokers vs. 10/96 (10.4%) never smokers harbored methylation of the RARβ2 gene (P = 0.003). This association was highly significant also in the subset of conventional variant PTC (P = 0.005) and marginally significant in follicular variant PTC (P = 0.06). The results demonstrate that smoking-associated aberrant methylation of the RARβ2 gene is a specific molecular event that may represent an important mechanism in thyroid tumorigenesis in smokers.
Introduction
Thyroid cancer is a common endocrine malignancy with a rapidly rising incidence in recent decades and at least 458,403 patients with thyroid cancer are currently living in the United States (Howlader et al., 2011). The most common histological type of this cancer is papillary thyroid cancer (PTC), which accounts for >80% of all thyroid malignancies (Hundahl et al., 1998; Howlader et al., 2011). PTC can be further divided into several subtypes, including mainly, in the order of decreasing prevalences, conventional, follicular variant and tall-cell PTC. Among the molecular derangements that drive thyroid tumorigenesis are epigenetic alterations, particularly aberrant gene methylation (Xing, 2007). Gene methylation is the covalent addition of a methyl group to the fifth carbon position of the cytosine residue in a CpG dinucleotide, which is usually associated with gene silencing and is a common mechanism for functional loss of key genes in human cancers (Esteller, 2008). Aberrant gene methylation in thyroid tumorigenesis often involves major tumor suppressor genes, such as those for the tissue inhibitor of metalloproteinase-3 (TIMP3), SLC5A8, death-associated protein kinase (DAPK), and retinoic acid receptor β2 (RARβ2), particularly in PTC (Hoque et al., 2005; Hu et al., 2006). The tumor suppressor functions of these genes have been well characterized and they are frequently methylated in cancers. For example, the anti-tumor role of the RARβ2 gene, which is often silenced by aberrant methylation in cancers, is mediated through inhibition of cancer cell proliferation and metastasis and induction of apoptosis (Raffo et al., 2000; Widschwendter et al., 2001; Treuting et al., 2002). Conversely, re-expression of RARβ2 is associated with significant reduction of cell growth in thyroid cancer cells (Miasaki et al., 2008).
Few environmental risk factors for thyroid cancer are known. Ionizing radiation exposure, the best-established risk factor for thyroid cancer, can induce RET/PTC rearrangements and consequent development of PTC, particularly in the pediatric population (Nikiforov, 2006). No environmental risk factor is known to cause epigenetic alterations in thyroid cancer. Smoking is a well known risk factor in many cancers. Smoking has also been widely shown to be a significant risk factor for the development of thyroid nodule/goiter, particularly in women (Lio et al., 1989; Ericsson and Lindgarde, 1991; Galanti et al., 2005). Thyroid nodule/goiter is, in turn, a strong risk factor for thyroid cancer (Preston-Martin et al., 1993; Franceschi et al., 1999). Although some studies did not show an increased risk associated with smoking for thyroid cancer (Ron et al., 1987; Kreiger and Parkes, 2000; Bandurska-Stankiewicz et al., 2011), others did show an association of smoking with occurrence of thyroid cancer (Sokic et al., 1994). Interestingly, smoking has been found to be associated with aberrant gene methylation in some cancers, such as the RARβ2 gene methylation in human lung cancer (Tomizawa et al., 2004) and animal lung cancer models (Vuillemenot et al., 2004). The effect of smoking on gene methylation has not been explored in thyroid cancer. In the present study, we investigated the relationship of smoking with methylation of several major tumor suppressor genes in PTC.
Materials and Methods
Patients
With Institutional Review Board approval and, where required, informed patient consent, we included 138 patients (99 female and 39 male, age 15–85 years), who were operated for PTC between 1990 and 2006 and had recorded history of smoking status, in this study. These included 96 patients as never smokers and 42 patients as ever smokers. The latter included current and former smokers. The smoking history of a patient was obtained through a retrospective review of the patient’s records. Smoking histories of the ever smokers varied from 2 to 35 pack years of cigarette smoking. A pack-year is defined as equivalent to smoking one pack of cigarettes daily for a period of 1 year.
Methylation Analysis
Paraffin-embedded PTC tumors were microdissected and processed and genomic DNA was isolated as described previously (Hu et al., 2006). Bisulfite-treated DNA was subjected to methylation analysis for the promoters of the tumor suppressor genes TIMP3, SLC5A8, DAPK, and RARβ2 using real-time quantitative methylation-specific PCR. The primers for these genes and PCR reaction conditions were as described previously (Hu et al., 2006). Any detectable level of methylation for the indicated genes defined as a positive methylation result and zero value on the current detection system was defined as a negative methylation result.
Statistical Analysis
Categorical data were summarized with frequencies and percentages, and continuous data with medians and ranges. Age and gender distribution were analyzed utilizing Mann–Whitney rank sum test and Fisher’s exact test, respectively. Smoking status groups were compared using Fisher’s exact test for categorical data, and the non-parametric Wilcoxon rank sum test for continuous measures. All reported P values are two-sided. A P-value of <0.05 was considered to be statistically significant. Analysis was performed using SAS version 9.1.3 software (SAS Institute Inc., Cary, NC, USA).
Results
We analyzed 138 PTC for the methylation status of the tumor suppressor genes TIMP3, SLC5A8, DAPK, and RARβ2. These genes were chosen for analysis because they are important human tumor suppressor genes and their methylation was frequently found in thyroid cancer (Hoque et al., 2005; Hu et al., 2006). Overall, methylation of these genes were detected in 67 (48.5%), 36 (26.1%), 40 (29.0%), and 23 (16.7%) of the 138 cases, respectively. Age and gender were potential confounding variables that could affect gene methylation. As shown in Table 1, except for the age of the DAPK methylation-positive patients that was significantly older in the ever-smoker group than the never-smoker group, there was no significant difference in the age of the methylation-positive patients for the remaining three genes between the ever-smoker and never-smoker groups. Similarly, as shown in Table 2, there was no significant difference in gender distribution of methylation-positive patients for the four genes examined in the present study. Also, smoking did not affect the relationship of gene methylation with tumor size and other tumor characteristics (data not shown).
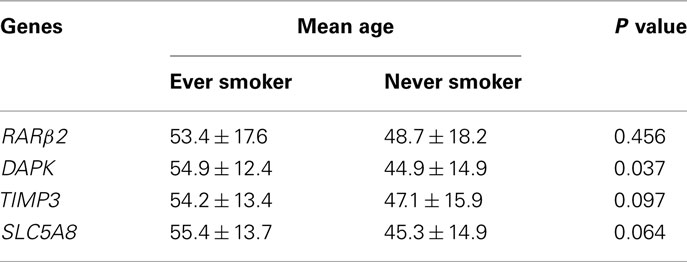
Table 1. Comparison of mean ages of methylation-positive patients for the indicated genes between the ever-smoker and never-smoker groups.
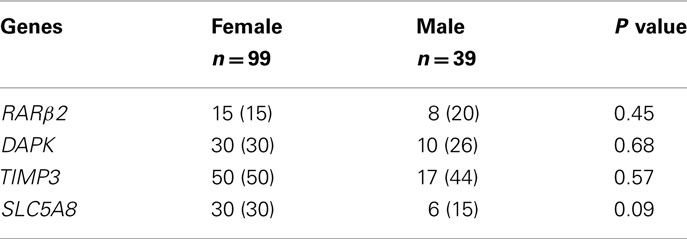
Table 2. Gender distribution of methylation-positive patients for the indicated genes [n (%)] (% = n/N).
We next analyzed the relationship of gene methylation in PTC with cigarette smoking history in 138 patients. As summarized in Table 3 and Figure 1, among the four tumor suppressor genes analyzed, methylation of the RARβ2 gene was significantly associated with a history of smoking. Specifically, 13/42 (31.0%) ever smokers vs. 10/96 (10.4%) never smokers harbored methylation in the promoter of the RARβ2 gene (P = 0.003). Although there was no statistically significant difference in the methylation of the TIMP3, SLC5A8, and DAPK genes between the never and ever smokers, there was a tendency of higher prevalences of methylation in these genes in the smokers (Figure 1; Table 3).
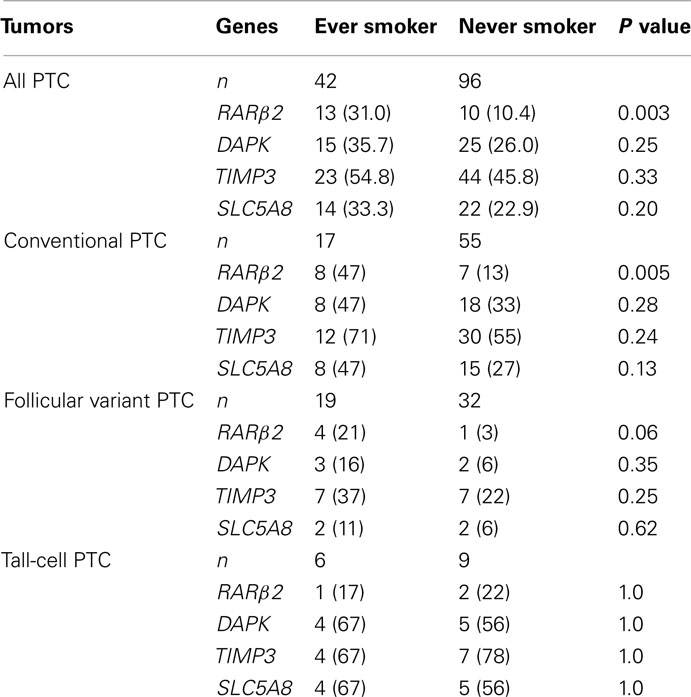
Table 3. Relationship of methylation of the RARβ 2, DAPK, TIMP3, and SLC5A78 genes in papillary thyroid cancer (PTC) with smoking of patients [n (%)].
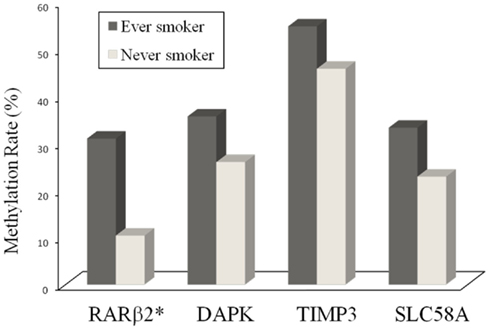
Figure 1. Aberrant gene methylation in papillary thyroid cancer in ever and never smokers. Shown on y-axis is the methylation rate – percentage of the methylation-positive cases (%) – for each of the four genes indicated on the x-axis. *The methylation rate of the RARβ2 gene is significantly higher (P = 0.003) in the ever smoker group than the never smoker group. The methylation rate for the remaining three genes shows a higher trend for ever smokers but does not reach statistical significance.
The 138 cases of PTC included 72 conventional PTC, 51 follicular variant PTC, and 15 tall-cell PTC. We also analyzed the relationship of smoking with gene methylation in each of the subtype groups of PTC. As shown in Table 3, similar to the results on the overall analysis of all the cases of PTC, RARβ2 methylation was significantly associated with smoking [8/17 (47%) of ever smokers vs. 7/55 (13%) of never smokers, P = 0.005] in conventional PTC. In the smaller number of cases of follicular variant PTC, marginally significant association of smoking with RARβ2 methylation was demonstrated (P = 0.06). This relationship was not seen in patients with tall-cell PTC, probably due to the small number of cases. A trend of association of smoking with the TIMP3, SLC5A8, and DAPK methylation was observed in these subtypes of PTC. The average methylation levels for each gene in the ever and never smokers were not significantly different (Table 4). However, this result was likely limited by the inability of the methylation PCR approach used in the present study to accurately provide quantitative measurements.
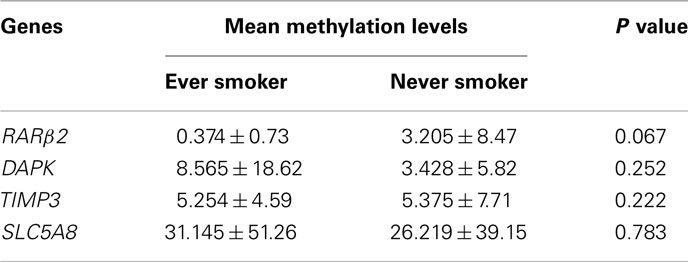
Table 4. Comparison of methylation levels for the indicated genes in papillary thyroid cancer between ever and never smokers.
Discussion
Tobacco smoking has long been known to be a significant risk factor for human cancers. This has prompted numerous studies on its role in molecular derangements in cancers, including genetic and epigenetic alterations, such as aberrant gene methylation. Smoking has also been shown to be associated with the development of thyroid nodules in many studies (Lio et al., 1989; Ericsson and Lindgarde, 1991; Galanti et al., 2005), a strong risk factor for thyroid cancer (Preston-Martin et al., 1993; Franceschi et al., 1999), and occurrence of thyroid cancer in some studies (Sokic et al., 1994). However, little is known about molecular derangements associated with smoking in thyroid cancer. In the present study, we for the first time investigated the relationship between patient smoking and aberrant gene methylation in PTC, the most common type of thyroid cancer. We found a significant association of smoking with methylation of the RARβ2 gene in PTC, both in overall PTC and in its major subtypes. This association represented a specific epigenetic event in PTC as the association of smoking with the methylation of other genes (TIMP3, SLC5A8, and DAPK) was not significant. Some of the relationship patterns of smoking with methylation of tumor suppressor genes in PTC observed in the present study were similar to the findings in other cancers. For example, as in the present study which failed to show a significant association of DAPK methylation with smoking in PTC, such an association was also not found in non-small lung cancer patients (Liu et al., 2007). Similar to our findings in PTC, previous studies demonstrated a significant association of smoking with RARβ2 methylation in lung cancers in humans (Tomizawa et al., 2004) and in animal models (Vuillemenot et al., 2004). Interestingly, even in normal non-cancerous epithelium of upper aerodigestive tract, methylation of the RARβ2 gene and other genes was more frequently seen in smokers (Zochbauer-Muller et al., 2003), supporting a direct effect of smoking on gene aberrant methylation. Frequent methylation of the RARβ2 gene has also been recently detected in laryngeal squamous cell carcinomas (Paluszczak et al., 2011), a malignancy often associated with smoking.
We previously demonstrated that methylation of the tumor suppressor gene RARβ2 was associated with its silencing and, conversely, un-methylation was associated with expression of this gene in thyroid cancer cells (Hu et al., 2006). Re-expression of RARβ2 using demethylating agent 5-aza-2′-deoxycytidine caused a significant inhibition of thyroid cancer cell growth (Miasaki et al., 2008). The association of smoking with RARβ2 methylation in PTC suggests that this gene may more commonly loose function in PTC of smokers. RARβ2 is a major type of retinoic acid (RA) receptors, which are transcription factors that bind with the biologically active metabolites of vitamin A to regulate cell growth and proliferation (Pfahl and Chytil, 1996). Therapeutic potential of vitamin A and retinoic acid has been demonstrated in various human cancers. RA has been shown to be able to re-differentiate thyroid cancer cell lines and increase radioiodine uptake in some patients who lost radioiodine avidity (Haugen et al., 2004; Coelho et al., 2005). However, the overall treatment efficacy is poor. One cause for the failure of RA therapy for thyroid cancer is the loss of RA receptor expression in this cancer. In vitro studies showed that RA could only inhibit the growth of thyroid cancer cell lines that expressed RARβ and some other RA receptors (Haugen et al., 2004; Elisei et al., 2005). Expression of RA receptors is often decreased or lost in thyroid cancers, particularly in PTC in which expression of the RARβ gene was most frequently decreased or silenced (Tang et al., 2003; Elisei et al., 2005). Our previous demonstration of aberrant RARβ2 gene methylation in thyroid cancer provided a molecular explanation (Hoque et al., 2005; Hu et al., 2006). Given these data and the results in the present study, it is clear that aberrant methylation of RA receptor genes is an important molecular event in PTC associated with smoking. How this aberrant methylation of RARβ2 gene specifically contributes to the tumorigenesis of PTC in smokers warrants further studies to elucidate.
Conflict of Interest Statement
The authors declare that the research was conducted in the absence of any commercial or financial relationships that could be construed as a potential conflict of interest.
Acknowledgments
This work was supported by NIH grant R01 CA134225 (to Mingzhao Xing). We thank the authors of the Hu et al., 2006 study who contributed to specimens and DNA methylation analysis in that publication, which were partially used in the present study.
References
Bandurska-Stankiewicz, E., Aksamit-Bialoszewska, E., Rutkowska, J., Stankiewicz, A., and Shafie, D. (2011). The effect of nutritional habits and addictions on the incidence of thyroid carcinoma in the Olsztyn province of Poland. Endokrynol. Pol. 62, 145–150.
Coelho, S. M., Vaisman, M., and Carvalho, D. P. (2005). Tumour re-differentiation effect of retinoic acid: a novel therapeutic approach for advanced thyroid cancer. Curr. Pharm. Des. 11, 2525–2531.
Elisei, R., Vivaldi, A., Agate, L., Ciampi, R., Molinaro, E., Piampiani, P., Romei, C., Faviana, P., Basolo, F., Miccoli, P., Capodanno, A., Collecchi, P., Pacini, F., and Pinchera, A. (2005). All-trans-retinoic acid treatment inhibits the growth of retinoic acid receptor beta messenger ribonucleic acid expressing thyroid cancer cell lines but does not reinduce the expression of thyroid-specific genes. J. Clin. Endocrinol. Metab. 90, 2403–2411.
Ericsson, U. B., and Lindgarde, F. (1991). Effects of cigarette smoking on thyroid function and the prevalence of goitre, thyrotoxicosis and autoimmune thyroiditis. J. Intern. Med. 229, 67–71.
Franceschi, S., Preston-Martin, S., Dal Maso, L., Negri, E., La Vecchia, C., Mack, W. J., Mctiernan, A., Kolonel, L., Mark, S. D., Mabuchi, K., Jin, F., Wingren, G., Galanti, R., Hallquist, A., Glattre, E., Lund, E., Levi, F., Linos, D., and Ron, E. (1999). A pooled analysis of case-control studies of thyroid cancer. IV. Benign thyroid diseases. Cancer Causes Control 10, 583–595.
Galanti, M. R., Granath, F., Cnattingius, S., Ekbom-Schnell, A., and Ekbom, A. (2005). Cigarette smoking and the risk of goitre and thyroid nodules amongst parous women. J. Intern. Med. 258, 257–264.
Haugen, B. R., Larson, L. L., Pugazhenthi, U., Hays, W. R., Klopper, J. P., Kramer, C. A., and Sharma, V. (2004). Retinoic acid and retinoid X receptors are differentially expressed in thyroid cancer and thyroid carcinoma cell lines and predict response to treatment with retinoids. J. Clin. Endocrinol. Metab. 89, 272–280.
Hoque, M. O., Rosenbaum, E., Westra, W. H., Xing, M., Ladenson, P., Zeiger, M. A., Sidransky, D., and Umbricht, C. B. (2005). Quantitative assessment of promoter methylation profiles in thyroid neoplasms. J. Clin. Endocrinol. Metab. 90, 4011–4018.
Howlader, N., Noone, A. M., Krapcho, M., Neyman, N., Aminou, R., Waldron, W., Altekruse, S. F., Kosary, C. L., Ruhl, J., Tatalovich, Z., Cho, H., Mariotto, A., Eisner, M. P., Lewis, D. R., Chen, H. S., Feuer, E. J., Cronin, K. A., and Edwards, B. K. (eds). (2011). SEER Cancer Statistics Review 1975-2008. Bethesda, MD: National Cancer Institute.
Hu, S., Liu, D., Tufano, R. P., Carson, K. A., Rosenbaum, E., Cohen, Y., Holt, E. H., Kiseljak-Vassiliades, K., Rhoden, K. J., Tolaney, S., Condouris, S., Tallini, G., Westra, W. H., Umbricht, C. B., Zeiger, M. A., Califano, J. A., Vasko, V., and Xing, M. (2006). Association of aberrant methylation of tumor suppressor genes with tumor aggressiveness and BRAF mutation in papillary thyroid cancer. Int. J. Cancer 119, 2322–2329.
Hundahl, S. A., Fleming, I. D., Fremgen, A. M., and Menck, H. R. (1998). A National Cancer Data Base report on 53,856 cases of thyroid carcinoma treated in the U.S., 1985–1995. Cancer 83, 2638–2648. [See comments].
Kreiger, N., and Parkes, R. (2000). Cigarette smoking and the risk of thyroid cancer. Eur. J. Cancer 36, 1969–1973.
Lio, S., Napolitano, G., Marinuzzi, G., and Monaco, F. (1989). Role of smoking in goiter morphology and thyrotropin response to TRH in untreated goitrous women. J. Endocrinol. Invest. 12, 93–97.
Liu, Y., Gao, W., Siegfried, J. M., Weissfeld, J. L., Luketich, J. D., and Keohavong, P. (2007). Promoter methylation of RASSF1A and DAPK and mutations of K-ras, p53, and EGFR in lung tumors from smokers and never-smokers. BMC Cancer 7, 74. doi: 10.1186/1471-2407-7-74
Miasaki, F. Y., Vivaldi, A., Ciampi, R., Agate, L., Collecchi, P., Capodanno, A., Pinchera, A., and Elisei, R. (2008). Retinoic acid receptor beta2 re-expression and growth inhibition in thyroid carcinoma cell lines after 5-aza-2′-deoxycytidine treatment. J. Endocrinol. Invest. 31, 724–730.
Nikiforov, Y. E. (2006). Radiation-induced thyroid cancer: what we have learned from chernobyl. Endocr. Pathol. 17, 307–317.
Paluszczak, J., Misiak, P., Wierzbicka, M., Wozniak, A., and Baer-Dubowska, W. (2011). Frequent hypermethylation of DAPK, RARbeta, MGMT, RASSF1A and FHIT in laryngeal squamous cell carcinomas and adjacent normal mucosa. Oral Oncol. 47, 104–107.
Pfahl, M., and Chytil, F. (1996). Regulation of metabolism by retinoic acid and its nuclear receptors. Annu. Rev. Nutr. 16, 257–283.
Preston-Martin, S., Jin, F., Duda, M. J., and Mack, W. J. (1993). A case-control study of thyroid cancer in women under age 55 in Shanghai (People’s Republic of China). Cancer Causes Control 4, 431–440.
Raffo, P., Emionite, L., Colucci, L., Belmondo, F., Moro, M. G., Bollag, W., and Toma, S. (2000). Retinoid receptors: pathways of proliferation inhibition and apoptosis induction in breast cancer cell lines. Anticancer Res. 20, 1535–1543.
Ron, E., Kleinerman, R. A., Boice, J. D. Jr., Livolsi, V. A., Flannery, J. T., and Fraumeni, J. F. Jr. (1987). A population-based case-control study of thyroid cancer. J. Natl. Cancer Inst. 79, 1–12.
Sokic, S. I., Adanja, B. J., Vlajinac, H. D., Jankovic, R. R., Marinkovic, J. P., and Zivaljevic, V. R. (1994). Risk factors for thyroid cancer. Neoplasma 41, 371–374.
Tang, W., Nakamura, Y., Zuo, H., Yasuoka, H., Yang, Q., Wang, X., Nakamura, M., Mori, I., Miyauchi, A., and Kakudo, K. (2003). Differentiation, proliferation and retinoid receptor status of papillary carcinoma of the thyroid. Pathol. Int. 53, 204–213.
Tomizawa, Y., Iijima, H., Nomoto, T., Iwasaki, Y., Otani, Y., Tsuchiya, S., Saito, R., Dobashi, K., Nakajima, T., and Mori, M. (2004). Clinicopathological significance of aberrant methylation of RARbeta2 at 3p24, RASSF1A at 3p21.3, and FHIT at 3p14.2 in patients with non-small cell lung cancer. Lung Cancer 46, 305–312.
Treuting, P. M., Chen, L. I., Buetow, B. S., Zeng, W., Birkebak, T. A., Seewaldt, V. L., Sommer, K. M., Emond, M., Maggio-Price, L., and Swisshelm, K. (2002). Retinoic acid receptor beta2 inhibition of metastasis in mouse mammary gland xenografts. Breast Cancer Res. Treat. 72, 79–88.
Vuillemenot, B. R., Pulling, L. C., Palmisano, W. A., Hutt, J. A., and Belinsky, S. A. (2004). Carcinogen exposure differentially modulates RAR-beta promoter hypermethylation, an early and frequent event in mouse lung carcinogenesis. Carcinogenesis 25, 623–629.
Widschwendter, M., Berger, J., Muller, H. M., Zeimet, A. G., and Marth, C. (2001). Epigenetic downregulation of the retinoic acid receptor-beta2 gene in breast cancer. J. Mammary Gland Biol. Neoplasia 6, 193–201.
Keywords: thyroid cancer, tobacco smoking, methylation, tumor suppressor gene, RARβ gene
Citation: Kiseljak-Vassiliades K and Xing M (2011) Association of cigarette smoking with aberrant methylation of the tumor suppressor gene RARβ2 in papillary thyroid cancer. Front. Endocrin. 2:99. doi: 10.3389/fendo.2011.00099
Received: 06 September 2011; Paper pending published: 28 September 2011;
Accepted: 22 November 2011; Published online: 08 December 2011.
Edited by:
Carmelo Nucera, Beth Israel Deaconess Medical Center/Harvard Medical School, USAReviewed by:
Oliver Gimm, University of Linköping, SwedenErzsebet Regan, Beth Israel Deaconess Medical Center, USA
Mario Vitale, University of Salerno, Italy
Copyright: © 2011 Kiseljak-Vassiliades and Xing. This is an open-access article distributed under the terms of the Creative Commons Attribution Non Commercial License, which permits non-commercial use, distribution, and reproduction in other forums, provided the original authors and source are credited.
*Correspondence: Mingzhao Xing, Division of Endocrinology and Metabolism, The Johns Hopkins University School of Medicine, 1830 East Monument Street, Suite 333, Baltimore, MD 21287, USA. e-mail: mxing1@jhmi.edu