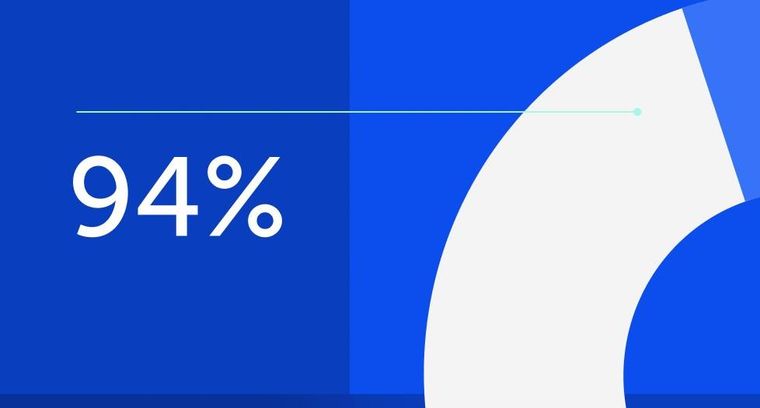
94% of researchers rate our articles as excellent or good
Learn more about the work of our research integrity team to safeguard the quality of each article we publish.
Find out more
HYPOTHESIS AND THEORY article
Front. Ecol. Evol., 14 April 2025
Sec. Evolutionary Developmental Biology
Volume 13 - 2025 | https://doi.org/10.3389/fevo.2025.1578563
Life on islands and cancer evolves under unique environmental pressures and acquire distinctive features. This manuscript is an attempt to find analogies between carcinogenesis and island biota colonization, evolution, and extinction. Hopefully this analogy will provide hints how extinct cancer. Both island biota and malignancy are initially scarce in number and genetic variation, and both experience rapid increase in genetic variability without mandatory environmental pressures. Island evolution follows two main pathways: anagenesis-linear speciation that fits the course of benign tumors, and cladogenesis-branching speciation that match malignant progression. Both island biota and most cancer types develop giantism compared to their mainland equivalents and normal cells of origin. Island biota is prone to extinction, but not all species are equally sensitive. Susceptible species exhibit low genetic diversity and long breeding cycles. Cancer cells habitually exhibit opposite features. Prolonging cancer cell cycle (by hormonal, pharmacological, or genetic manipulations) can potentially make cancer cells more prone to extinction by other interventions. Further studies of the similarities between island evolution and carcinogenesis may reveal additional intervention points.
It is difficult to see what is not, or at any time has not been an island (Maberley, 1979).
Islands are natural laboratories of evolution (Mayr, 1963).
Islands rise from the sea by volcanic eruptions (oceanic islands) or separate from mainland (continental islands). Oceanic islands start empty, whereas continental island biota is initially similar to that of mainland. Nevertheless, a secluded ecosystem is formed and is shaped by evolutionary forces. The colonization and evolution of oceanic islands are often accompanied by marked morphological and behavioral changes of arriving animals and not seldom by extinction. This occurs when a habitat is lost, fragmented, invaded by species that compete for resources, or when diseases are introduced to the island.
Cancer is an uncontrolled cellular growth that can invade and spread to other parts of the body. Chroni and Kumar suggested that cancer dynamics can be considered as island-like ecosystem, and that this view can facilitate understanding of spatial and longitudinal variations in cancer (Chroni and Kumar, 2021). Primary cancer, and more so metastases create local isolated microenvironments that can be viewed as islands. These ecosystems include locally modulated blood supply, metabolism, immune response and extracellular matrix.
In this manuscript the similarities between island habitat evolution and carcinogenesis and their potential clinical implications will be further explored.
Island colonization is an extremely complex process. Besides the physical distance from mainland, arriving biota often faces limits in food and water resources, differences in habitat (shelter, vegetation, etc.), exposure to predation, competition from existing species, and exposure to infectious agents unfamiliar to their immune system. The first colonization of oceanic islands is by organisms and plants whose seeds can be transported by wind. Flying insects and birds follow. In addition to their airborne abilities, colonizing organisms must thrive and reproduce in the foreign land. Similarly, primary tumors develop in normal tissues (occasionally modified by carcinogens or oncogenic viruses), and their survival depends on gaining multiple capabilities including self-sufficiency of growth signals, insensitivity to anti-growth factors, tissue invasion, limitless replicative potential, sustained angiogenesis, evasion of the immune system, and evasion of apoptosis (Hanahan and Weinberg, 2012). The similarity between metastatic dissemination and the habitation of an island is even greater. While primary tumors develop inside their own ecosystems (e.g., bladder cancer develops in the bladder), metastases flourish in organs far from their original habitat (Figure 1).
Figure 1. Metastases as “islands” on coronal CT images. Lung metastases (yellow arrows) in patients with melanoma (a) and colon cancer (b). Liver metastases (blue arrows) in patients with pancreatic cancer (c) and colon cancer (d).
During the initial phases of island colonization, the number of the inhabitants is small, and it may be difficult for them to find a mate. At this stage, hermaphroditic and parthenogenic species have survival advantages (Baker’s rule) (Cheptou, 2012). Likewise, both primary and metastatic tumors benefit from their “parthenogenic” ability during their initial survival period. As discussed in the next chapter, this situation changes rapidly for both insular biota and cancer.
Due to similar climatic conditions, island colonization is easier at similar latitudes (i.e., east to west or west to east) and less so across longitudes (i.e., south to north or north to south). Similarly, some tumors tend to spread horizontally inside the organs before invading deeper. Examples include superficial spreading melanoma, that spreads within the epidermis for months before invading the dermis; gastric linitis plastica, which spreads in the stomach wall, and bladder cancer, which often infiltrates the bladder mucosa and underlying submucosa months before invading the detrusor muscle. The analogies between insular and cancerous colonization are summarized in Table 1.
Relatively few founders inhabit a new island, which results in a marked decrease in genetic heterogeneity (the founder principle). The consequence of this genetic bottleneck is vulnerability to early extinction and limited ability for further dispersion (Whittaker et al., 2017; Abdelkrim et al., 2005). However, soon after colonization, and in an absence of environmental pressure, sexually reproducing species show a remarkably rapid increase in genetic variability. This phenomenon explains the relatively high percentage of dioecious species (with distinct male and female individuals) on islands (28% of Hawaiian plants compared with 9% on the tropical mainland). This diversity is believed to stem from genetic recombination (Carson, 1992). One may also speculate that some of the high genetic variability observed on the islands results from the extinction of species that failed to do so.
In a near-perfect analogy, cancer most probably stems from a single transformed (founder) cell, which is genetically pure, has a high potential for extinction and low potential for dispersion. However, variability develops rapidly in the absence of stringent selection (the Big Bang model of cancer growth) (Sun et al., 2018). This contrasts with the former concept of the stepwise acquisition of somatic mutations and expansion of clones with selective growth advantages. Mechanisms underlying this process include high mutation rate, the presence of transposable elements, chromosomal rearrangements, aneuploidy, reactivation of atavistic attractors and other genetic derangements (Hasty and Montagna, 2014; Kasperski, 2022). This feature, often termed evolvability, assists tumors in surviving catastrophes, such as fluctuations in oxygen availability, redistribution of angiogenesis, build-up of toxic metabolites, immune system endeavors, and focal necrosis (Pienta et al., 2020). Metastases follow the same sequence of events and can arise from a few cells (or even from a single cell). Early in their evolution, they have narrow genetic pool and high vulnerability to extinction, but shortly (if they survive) they evolve and gain resilience and capacity for stepwise metastasis.
Additionally, both island colonization and cancer spread can be viewed as following the vertical and the horizontal developmental models. The vertical (classical) model assumes development through sequential adjustments of island biota to new conditions and accumulation of genetic and epigenetic changes in a single cancer cellular lineage. The horizontal model assumes parallel and independent changes occurring across multiple populations (Kasperski, 2022). Nevertheless, it must be remembered the difference between island biota and cancer. While island biota has intrinsically stable genetics, the hallmark of cancer is chromosomal instability and gene mutations that promote “selfish” behavior and loss of the multicellular layer functionality (Kasperski and Heng, 2024).
In analogy to early the extinction of island inhabitants that failed to increase their genetic variability, stands the immune surveillance theory (Ribatti, 2017). Three phases of surveillance are assumed. In the first phase, natural killer, CD4, CD8, and other immune cells eliminate tumor cells. This probably occurs on a daily basis. In the second phase, there is an equilibrium between the tumor and the immune system. Escapes occur in the third phase when the tumor (armed with genetic evolvability) overcomes the immune system and spreads. This is the clinical phase that corresponds to island inhabitants that have managed to survive, flourish, and even spread to neighboring islands.
Insular biota evolution can follow one of several pathways. Benign and malignant tumors can be described using these passageways (Table 2).
This is a linear speciation that does not involve branching. This common type of evolution consists of genetic drifts shaped by the biotic and abiotic environments of the island. Anagenesis is typically observed on remote islands with relatively stable environment (Whittaker et al., 2017). This pathway perfectly fits the evolution of benign tumors. Their growth shows stable expansion without genetic branching or attempts to metastasize. Benign thyroid nodules are typical examples of this speciation (Ye et al., 2017).
This refers to the evolutionary divergence of an ancestral form to two or more lines, while the original form often fails to survive. Galápagos finches with their different beaks that have adapted to exploit different food sources, representing a classic example of this type of evolution. These birds played a significant role in shaping Darwin’s ideas of evolution. This evolutionary pathway explains like the progression of colonic adenoma to carcinoma (Cross et al., 2018). Cladogenesis benefits cancer cells by enabling them to invade and flourish on different grounds (Davis et al., 2017).
This is the evolutionary development that makes a species distinct from its ancestor. It is often the result of cladogenesis and is exemplified by the adaptation of Hawaii honeycreepers. Adaptive radiation is an evolutionary process that occurs in metastatic cells after landing at a new site.
This occurs when different species develop the same traits independently without a common ancestor. The penguins of the Southern Hemisphere and the extinct great auks of the Northern Hemisphere are examples of convergent evolution. Both birds abandoned flight and transformed their wings into flippers independently. Urothelial cancer exhibits convergent evolution. It often arises either synchronously or metachronously in the upper urinary tract and in the bladder. Both transformations have common risk factors (cigarette smoking, exposure to aromatic amines, etc.), mutated genes (FGFR3, TP53, and HRA), and epigenetic changes (KDM6A and KMT2A-C). Yet upper urothelial cancer more commonly show a luminal molecular sub-type while bladder cancer are more commonly of the basal sub-type. Additionally, T-cell depletion is more common in upper urothelial cancer (Sfakianos et al., 2021). These differences impact on responses to treatment.
Neutral evolution assumes a random and steady rate of mutations resulting in genetic drift. Most mutations do not influence cellular fitness but do contribute to tumor diversity. Punctuated evolution assumes periods of relative stability interrupted by bursts of rapid changes. While the evolution of island biota follows both pathways, the evolution of cancer remains broadly unknown (Lomolino and Brown, 2009). Recent studies using single-cell lineage tracing suggest that primary tumors more often follow the neutral, and metastases the punctuated evolutionary pathways (Butler et al., 2025).
One of the most fascinating features of island biota is their size differences and behavioral changes compared to their mainland counterparts. Foster’s rule states that upon insular isolation, small animals become larger and large animals become smaller. No single factor can explain this phenomenon. Possible explanations for giantism include immigrant selection, intensified intraspecific competition, and ecological release from large competitors and predators. Possible explanations for dwarfism include resource limitations and specialization in insular niches. Small size offers the advantages of a short gestational period and early sexual maturation. Changes in body size are mediated by island climate, size, and isolation (Benítez-López et al., 2021; Rozzi et al., 2023). The more isolated and different the islands are from the mainland, the more pronounced is this phenomenon. High seasonality (unpredictable environments) also supports this trend. The dodo bird is a well-known example of insular giantism and behavioral changes. When the volcanic Mauritius islands rose above the Indian ocean waves approximately 10 million years ago, a new empty and fertile soil became available for population. Plants arrived first, followed by birds. DNA sequencing revealed that the closest living relative of dodo, and probably its ancestor, is the Nicobar pigeon. When these birds landed in Mauritius, they found a habitat with no predators and ample supply. Flying abilities require significant resources, which have now become a waste. These conditions send the Nicobar pigeons into an evolutionary trajectory that made them larger and flightless. They also lost their defensive instincts and territoriality. A similar course of events created the giant, flightless Moa of New Zealand. Examples of dwarfism include the dwarf hippos of Madagascar and the dwarf elephants of Sicily and Malta.
Cancer cells loss control not only of proliferation but also of the tightly controlled cellular volume (Lloyd, 2013). They typically exhibit giantism, while benign tumors usually do not show this phenomenon (Tables 3, 4). Almost all cancer cells show cellular giantism, mostly by X 2–3 folds. Exceptions include melanoma with a volume similar to their melanocyte counterparts, high-grade astrocytoma cells that are X 6–7 the volume of a normal astrocyte, and lymphoma cells that are X 10–20 times the volume of a normal lymphocyte (Perry and Wesseling, 2016). In lymphomas, high cellular volume is associated with higher grade (Hauser et al., 1986). Cellular dwarfism is observed only in liposarcomas (by a factor of 0.008).
At the microecological level, cancer cell giantism is the result of increased amount of cytoplasm and organelles, constant cellular stress, altered metabolism, genetic instability, abnormal mitoses, and aneuploidy. High cellular volume makes energy production and conservation more efficient at the cost of a smaller surface-area-to-volume ratio, which makes nutrient absorption and waste excretion (including heat) more difficult. This is one reason why both primary and secondary tumors are hotter than their surrounding tissues (other reasons include increased metabolic rate, angiogenesis, and accompanying inflammation). Difficulties in heat dispersion explain the vulnerability of cancer cells to hyperthermia (Wust et al., 2002). Except for meningioma, cellular giantism was not found in benign tumors.
Cancer cells often lose their normal cellular functions, and this often leads to a diagnosis. For example, bladder cancer cells do not protect the underlying lamina propria from the urine. Exposure of blood vessels and nerve endings to urine produces the typical symptoms of bladder cancer, namely hematuria and irritative urinary symptoms.
Island biota has another important characteristic: vulnerability to extinction. It is estimated that over the lifetime of earth, more than 99.9% of all species that ever lived have become extinct. On islands this figure is probably higher. There are two types of extinction: mass and background. Mass extinctions are primarily caused by climate change, volcanic activity, asteroid impaction, geological events, or combination of these reasons. Thus far, there have been five mass extinctions (scientists believe that we are currently experiencing the sixth). Background extinction has other causes. On Islands these including direct predation, habitat loss and fragmentation, entrance of invasive species, competition for resources, and the introduction of diseases, or pollution. Island population is more susceptible to extinction than mainland population because of its limited biodiversity, small population, fragile ecosystems, and limited resources.
However, not all island species are equally sensitive to extinction. Extinction-prone species exhibit low genetic diversity, long breeding cycle, and late sexual maturity (Furlan et al., 2012). Cancer cells habitually exhibit opposite features. They have high genetic diversity, short generation cycle, and genetic instability, which allows them to adapt to changing conditions, to develop resistance to interventions and to become more aggressive. For example, most prostate cancers are androgen-dependent at diagnosis and androgenic deprivation causes dramatic disease regression. Unfortunately, castrate-resistant clones with increased androgen receptor expression or mutated receptors often develop after 1–3 years of androgenic deprivation.
Nevertheless, the analogy of cancer to island biota exposes at least one possible intervention point: cell cycle interval. Prolonging cell cycle can render cancer cells more prone to extinction by the immune system or by other interventions. There are several ways of prolonging cell cycle. These include hormonal manipulation (estrogens, androgens, or thyroxine), pharmacological manipulation with inhibitors of microtubule formation (vinca alkaloids or Taxanes at sublethal doses), gene therapy with inhibitors of the G1 to S phase transition (p21, p27, and p16) or cyclin-dependent kinases, or by increasing the expression of p53 or other cell cycle checkpoint inhibitors (ATM/ATR) (Guarducci et al., 2024; Wang et al., 2011; Athar et al., 2011). Trials targeting p53 are going on. For example, nutlin-3 and NSC-66811 are inhibitors of MDM2, the negative regulator p53. Both were shown to trigger cell death in BCR::ABL1-independent imatinib-resistant CML cells (Kimura et al., 2025). Integrating these therapies with other anticancer treatments may improve the therapeutic results without adding significant toxicity.
In conclusion, this manuscript shows that the events occurring during insular habitation, evolution and extinction offer potentially useful terminology and tools in oncology research. The colonization of islands with its mandatory genetic bottleneck and the rapid switch to high genetic diversity, match the events occurring during the primary and secondary tumors development. Cladogenesis and adaptive radiation perfectly describe the morphology and genetics of malignant tumors and anagenesis that of benign tumors. Island giantism is typical of malignant (but not benign) tumors. The proneness of island biota to extinction, can potentially expose weak spots in the malignant process and provide hints for cancer diagnosis and treatment.
The original contributions presented in the study are included in the article/supplementary material. Further inquiries can be directed to the corresponding author.
OG: Conceptualization, Data curation, Funding acquisition, Investigation, Methodology, Supervision, Validation, Writing – original draft, Writing – review & editing.
The author(s) declare that no financial support was received for the research and/or publication of this article.
The author declares that the research was conducted in the absence of any commercial or financial relationships that could be construed as a potential conflict of interest.
The author(s) declare that no Generative AI was used in the creation of this manuscript.
All claims expressed in this article are solely those of the authors and do not necessarily represent those of their affiliated organizations, or those of the publisher, the editors and the reviewers. Any product that may be evaluated in this article, or claim that may be made by its manufacturer, is not guaranteed or endorsed by the publisher.
Abdelkrim J., Pascal M., Samadi S. (2005). Island colonization and founder effects: the invasion of the Guadeloupe islands by ship rats (Rattus rattus). Mol. Ecol. 14, 2923–2931. doi: 10.1111/j.1365-294X.2005.02604.x
Athar M., Elmets C. A., Kopelovich L. (2011). Pharmacological activation of p53 in cancer cells. Curr. Pharm. Des. 17, 631–639. doi: 10.2174/138161211795222595
Benítez-López A., Santini L., Gallego-Zamorano J., Milá B. (2021). The island rule explains consistent patterns of body size evolution in terrestrial vertebrates. Nat. Ecol. Evol. 5, 768–786. doi: 10.1038/s41559-021-01426-y
Butler G., Amend S. R., Venditti C., Pienta K. J. (2025). Punctuational evolution is pervasive in distal site metastatic colonization. Proc. Biol. Sci. 292, 20242850. doi: 10.1098/rspb.2024.2850
Chroni A., Kumar S. (2021). Tumors are evolutionary island-like ecosystems. Genome Biol. Evol. 13 (12), evab276. doi: 10.1093/gbe/evab276
Cross W., Kovac M., Mustonen V., Temko D., Davis H., Baker A. M., et al. (2018). The evolutionary landscape of colorectal tumorigenesis. Nat. Ecol. Evol. 2, 1661–1672. doi: 10.1038/s41559-018-0642-z
Davis A., Gao R., Navin N. (2017). Tumor evolution: Linear, branching, neutral or punctuated? Biochim. Biophys. Acta Rev. Cancer 1867, 151–161. doi: 10.1016/j.bbcan.2017.01.003
Furlan E., Stoklosa J., Griffiths J., Gust N., Ellis R., Huggins R. M., et al (2012). Small population size and extremely low levels of genetic diversity in island populations of the platypus, Ornithorhynchus anatinus. Ecol Evol. 2 (4), 844–57. doi: 10.1002/ece3.195
Guarducci C., Nardone A., Russo D., Nagy Z., Heraud C., Grinshpun A., et al. (2024). Selective CDK7 inhibition suppresses cell cycle progression and MYC signaling while enhancing apoptosis in therapy-resistant estrogen receptor-positive breast cancer. Clin. Cancer Res. 30, 1889–1905. doi: 10.1158/1078-0432.CCR-23-2975
Hanahan D., Weinberg R. A. (2012). The hallmarks of cancer. Cell 2000 100, 57–70. doi: 10.1016/s0092-8674(00)81683-9
Hasty P., Montagna C. (2014). Chromosomal Rearrangements in Cancer: Detection and potential causal mechanisms. Mol. Cell Oncol. 1, e29904. doi: 10.4161/mco.29904
Hauser L., Kurec A., Goldberg J., Dubowy R. L., Davey F. R. (1986). Prognostic significance of mean cell volume in non-Hodgkin’s lymphomas. Cancer 57, 2363–2367. doi: 10.1002/1097-0142(19860615)57:12<2363::aid-cncr2820571221>3.0.co;2-v
Kasperski A. (2022). Life entrapped in a network of atavistic attractors: how to find a rescue. Int. J. Mol. Sci. 23, 4017. doi: 10.3390/ijms23074017
Kasperski A., Heng H. H. (2024). The spiral model of evolution: stable life forms of organisms and unstable life forms of cancers. Int. J. Mol. Sci. 25, 9163. doi: 10.3390/ijms25179163
Kimura A., Tsubaki M., Obana T., Matsuo T., Komori R., Nagai N., et al. (2025). MDM2 inhibitors induce apoptosis by suppressing MDM2 and enhancing p53, Bax, Puma and Noxa expression levels in imatinib-resistant chronic myeloid leukemia cells. BioMed. Rep. 22, 65. doi: 10.3892/br.2025.1943
Lloyd A. C. (2013). The regulation of cell size. Cell. 154, 1194–1205. doi: 10.1016/j.cell.2013.08.053
Lomolino M. V., Brown J. H. (2009). The reticulating phylogeny of island biogeography theory. Q Rev. Biol. 84, 357–390. doi: 10.1086/648123
Maberley D. J. (1979). “Pachycaul plants and islands,” in Plant and islands. Ed. Bramwell D. (Academic Press London).
Perry A., Wesseling P. (2016). Histologic classification of gliomas. Handb. Clin. Neurol. 134, 71–95. doi: 10.1016/B978-0-12-802997-8.00005-0
Pienta K. J., Hammarlund E. U., Axelrod R., Amend S. R., Brown J. S. (2020). Convergent evolution, evolving evolvability, and the origins of lethal cancer. Mol. Cancer Res. 18, 801–810. doi: 10.1158/1541-7786.MCR-19-1158
Ribatti D. (2017). The concept of immune surveillance against tumors. The first theories. Oncotarget. 8, 7175–7180. doi: 10.1158/1541-7786.MCR-19-1158
Rozzi R., Lomolino M. V., van der Geer A. A. E., Silvestro D., Lyons S. K., Bover P., et al. (2023). Dwarfism and gigantism drive human-mediated extinctions on islands. Science 379, 1054–1059. doi: 10.1126/science.add8606
Sfakianos J. P., Gul Z., Shariat S. F., Matin S. F., Daneshmand S., et al. (2021). Genetic differences between bladder and upper urinary tract carcinoma: implications for therapy. Eur. Urol Oncol. 4, 170–179. doi: 10.1016/j.euo.2020.12.007
Sun R., Hu Z., Curtis C. (2018). Big bang tumor growth and clonal evolution. Cold Spring Harb. Perspect. Med. 8 (5), a028381. doi: 10.1101/cshperspect.a028381
Wang X., Gao P., Long M., Lin F., Wei J. X., Ren J. H., et al. (2011). Essential role of cell cycle regulatory genes p21 and p27 expression in inhibition of breast cancer cells by arsenic trioxide. Med. Oncol. 28, 1225–1254. doi: 10.1007/s12032-010-9552-x
Whittaker R. J., Fernández-Palacios J. M., Matthews T. J., Borregaard M. K., Triantis K. A. (2017). Island biogeography: taking the long view of nature’s laboratories. Science 357, eaam8326. doi: 10.1126/science.aam8326
Wust P., Hildebrandt B., Sreenivasa G., Rau B., Gellermann J., Riess H., et al. (2002). Hyperthermia in combined treatment of cancer. Lancet Oncol. 3, 487–497. doi: 10.1016/s1470-2045(02)00818-5
Keywords: island biota, malignant tumors, benign tumors, colonization, evolution, extinction
Citation: Gofrit ON (2025) Island biogeography: colonization, evolution, and excitation; analogy and potential implication to oncology. Front. Ecol. Evol. 13:1578563. doi: 10.3389/fevo.2025.1578563
Received: 17 February 2025; Accepted: 24 March 2025;
Published: 14 April 2025.
Edited by:
Paola Gazzaniga, Sapienza University of Rome, ItalyReviewed by:
Andrzej Kasperski, University of Zielona Góra, PolandCopyright © 2025 Gofrit. This is an open-access article distributed under the terms of the Creative Commons Attribution License (CC BY). The use, distribution or reproduction in other forums is permitted, provided the original author(s) and the copyright owner(s) are credited and that the original publication in this journal is cited, in accordance with accepted academic practice. No use, distribution or reproduction is permitted which does not comply with these terms.
*Correspondence: Ofer N. Gofrit, b2dvZnJpdEBnbWFpbC5jb20=
†ORCID: Ofer N. Gofrit, orcid.org/0000-0001-8815-6488
Disclaimer: All claims expressed in this article are solely those of the authors and do not necessarily represent those of their affiliated organizations, or those of the publisher, the editors and the reviewers. Any product that may be evaluated in this article or claim that may be made by its manufacturer is not guaranteed or endorsed by the publisher.
Research integrity at Frontiers
Learn more about the work of our research integrity team to safeguard the quality of each article we publish.