- 1School of Biosciences, Cardiff University, Cardiff, United Kingdom
- 2Department of Horticulture, Sapientia Hungarian University of Transylvania, Tirgu-Mureș, Romania
Evolution ultimately proceeds via ecological specialisation. This is regardless of the exact mechanism/s involved (e.g. selection, genetic drift, allopatric, sympatric, parapatric, etc.), leading to unique, functionally adapted entities (genetically-physiologically-biochemically-chemically-morphologically-behaviorally) filling new or vacant niches, whereupon the novel population becomes reproductively isolated from the original parent population. True, some such species can occasionally hybridise/introgress with other closely related species, and occasionally may be reproductively viable, but species populations tend to show restricted genetic variation (genetic identity) over their geographical range. If specialism is the main driving force of evolution, as clear from the fossil record as well as extant life forms, this must be reflected in the habitat and dietary specialisations. All living things are constrained by the aforementioned life-style factors, e.g. morphology, etc., which maintains them within their adopted niche and thereby reduces intra- and interspecific competition. Can a species, in a biological sense, be a ‘Jack-of-all-trades and master of none’? This is the paradox to be faced if the reality of generalism is to be accepted. In addition, the recent widespread discovery, using molecular techniques, of morphologically-cryptic entities within hitherto accepted ‘good species’ suggests that the diet breadth of such species complexes may in turn be more complex than imagined.
“Species are constantly evolving and changing, making speciation more of a continuum than an abrupt boundary. It can sometimes be difficult to know where to set the species threshold.” Arlo Hinckley, cited in Tamisiea (2024)
Overview
The term generalism, more especially as it refers to diet breadth in animals, which this article primarily concerns, is still widely used in the scientific literature (Fox and Morrow, 1981; Loxdale et al., 2011; 2019; Bonsall and Wright, 2012; Leonard and Lancaster, 2022; Morley et al., 2024). It is often coupled with the extreme opposite condition, specialism, although others suggest that a spectrum of states exist between the two extremes (Loxdale and Harvey, 2016; Dehling et al., 2021; Morley et al., 2024), especially in terms of functional roles, such ‘that both specialists and generalists fulfil important roles in ecological processes’ (cf. Dehling et al., 2021 for further details). Certainly, some animals appear to be highly polyphagous, even omnivorous (e.g. American black bear, Ursus americanus Pallas), but nevertheless these animals are highly specialised in relation to their physiology and behaviour, morphology/anatomy. Black bears have existed as a separate species in North America for around 5 million years (Krause et al., 2008) and despite the fact that other animals such as Grey wolves, Canis lupus L. compete with them for certain food items (e.g. deer fawns and fish), they have not been outcompeted or displaced from their unique ecological niche and habitat, and continue to thrive. And that to us is the main point: an animal evolves to fill a given ecological niche, probably involving the development of specialised character traits, i.e. anatomical/morphological, chemical/physiological, chemical ecological and importantly, behavioural, and that may be taken to be the fundamental functional specialism/s of a particular species or sub-specific species population in maintaining itself within its niche, i.e. its narrow ecological or physiological niche compared to the available functional space. It is also important to state early on that an animal may be a generalist for one trait but specialised in another, e.g. the aardvark, Orycteropus afer (Pallas) (Mammalia: Tubulidentata: Orycteropodidae) is a dietary specialist feeding almost exclusively on ants and termites (myrmecophagy), while being a climatic-habitat generalist (Nowak, 1991). As far as diet is concerned, such a niche may be a unique, hitherto largely unexploited (e.g. bats with their aerial nocturnal foraging), or the animal in question may inhabit a previously occupied but now vacant niche (e.g. albatrosses vs. sea-going pterodactyls). Either way, once the basic animal species has managed to exploit a new habitat with associated resources, it may then adaptively radiate to perhaps produce a wide range of further, even more specialised entities, exploiting new niches, as seen in the case of both bats (e.g. Sampaio et al., 2003) and pterosaurs (e.g. Chang-Fu et al., 2017; Martill and Smith, 2024).
Of course, no animal exists within its niche in isolation. Rather, it invariably survives in an integrated association with other organisms. In effect, as part of a food web, be these organisms intra- or interspecific competitors for resources (e.g. food, shelter, mates, etc.), with predators (be they feeding on animal or plant prey), parasites, pathogens, whilst in the case of plants, not only are these predated by animals, i.e. herbivores, but are parasitized by them, both externally and internally. Sometimes, the associations between living organisms are co-evolved and mutualistic, perhaps over millions of years (Thompson, 1994). Lastly, and very importantly, some animals such as bees and flies, birds and bat species also provide functional ecological services, notably the pollination of flowers, an arrangement that is also mutually beneficial (Darwin, 1862; Coyne and Orr, 2004). Plants cannot resist attacks by animals by fleeing, as animals themselves often do, so instead have evolved an array of defence mechanisms, principally chemical defences, and anatomical-morphological ones, including thickened epidermal layers, spines and sticky hairs, as mentioned below.
Plants can also recruit predatory and parasitic insects to aid them in their defence against herbivorous insects using volatile semiochemicals (Aljbory and Chen, 2018). In turn, some animals, e.g. insects and birds, have used the toxins they imbibe, especially from plants, in their own defence, and involving aposematic warning colours to advertise themselves as noxious (or apparently so in the case of dishonest signalling), either in terms of Müllerian or Batesian mimicry (Cott, 1940; Benedek et al., 2019; Loxdale, 2023). Hence, an array of specialist and generalist functional activities are seen in animals within ecological niches and their role within such niches is by no means necessarily a simple one, e.g. as in the case of Large blue butterflies, Maculinea spp. (Lepidoptera: Rhopalocera: Lycaenidae) and their predatory-parasitic association with red ants, Myrmica spp. (Thomas and Lewington, 1991; Als et al., 2004).
It is now widely accepted that living organisms evolve principally by a process of ecological specialisation, and involving selection, genetic drift and chromosomal/karyotypic changes (White, 1978; Monti et al., 2012), including inversion polymorphisms (Kapun and Flatt, 2019), epigenetic inheritance (Stajic and Jansen, 2021) and introgression and hybridisation (see below, p. 5). Sometimes such evolution is relatively rapid occurring over historical time scales (Loxdale, 2010), as in the case of the adaptive radiation of aphids belonging to the pea aphid species complex (Insecta: Hemiptera: Aphididae), feeding on leguminous hosts (Peccoud and Simon, 2010) and is ‘favoured under divergent selection imposed by increased environmental heterogeneity’ as suggested by Nery et al. (2023) in relation to plants and Leonard and Lancaster (2022) in animals.
It is thus difficult to perceive how any species, for example an animal species, can ever be a generalist sensu stricto. In fact, since the process of evolution is governed by natural selection and adaptation, then if generalism were actually to exist, evolution as we understand it would surely cease. Such a generalist animal species could in theory compete with other animals within its habitat, perhaps driving these to extinction. In other words, the theoretical contour lines on the adaptive landscape around the ‘adaptive peaks’ of competing specialist organisms would be blurred in relation to optimal foraging (Stuart Reynolds, pers. comm.; cf. also Futuyma and Moreno, 1988 and Mallet, 2007, his Figure 1). In this light, there are clearly cost-benefit considerations: a specialist must, one assumes, be at an advantage in terms of exploiting a given resource so long as this resource is available, whereas a generalist has an advantage when a given resource, hitherto abundant, becomes scarce … assuming it is able to catch/forage on that resource, and has the wherewithal in terms of behaviour, morphology, and physiology/biochemistry to tackle a small number of novel resources or less likely, a wider range of resources (i.e. optimal foraging theory; Futuyma and Moreno, 1988; Mikkelsen et al., 2024).
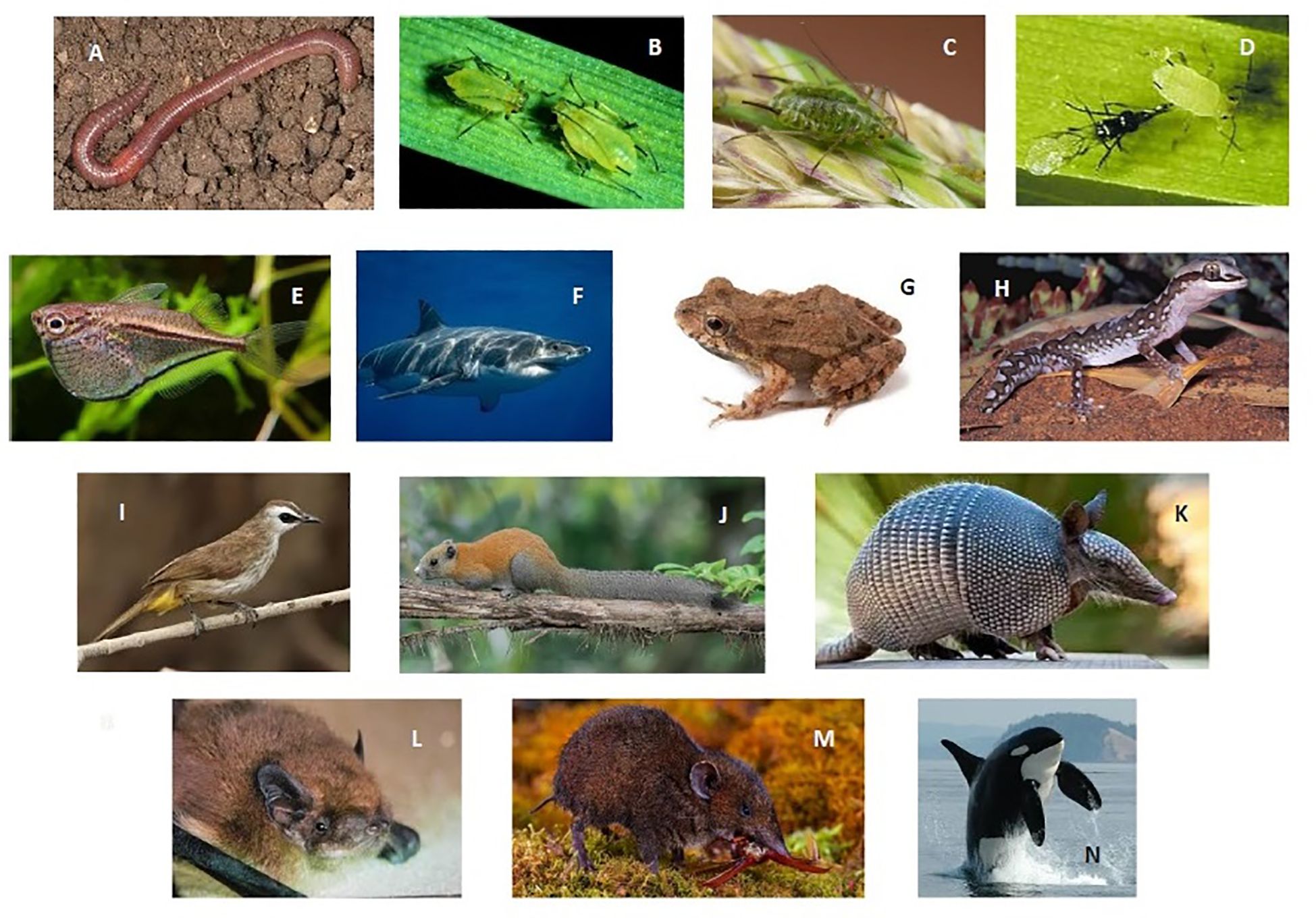
Figure 1. Range of animals hitherto considered to be ‘good’ species in terms of their population spatial genetic variability, but now shown, using molecular techniques, to be cryptic species complexes, or as in the case of the killer whale and great white shark, ecotypes/subspecies with different geographic ranges and diet preference/breadth. In this light, it is possible that all the various cryptic entities discovered in recent years, including those displayed above, have different feeding preferences and hence diet breadth. According to Oliver et al. (2009), “Available evidence suggests that hundreds of amphibian, reptile, mammal and fish species remain unrecognized.” (cf. references cited therein). With invertebrates, especially including insects, representing around 75% of all animal species recorded (Grimaldi and Engel, 2005), this value may be much higher, possibly thousands or tens of thousands of species as yet undetected. (A) Earthworm, Lumbricus rubellus Hoffmeister; (B) English Grain aphid (apterous forms), Sitobion avenae (F.); (C) Rose-grain aphid, S. fragariae (Walker); (D) Adult wasp parasitoid, Aphdius ervi Haliday attacking and laying an egg inside an apterous S. avenae; (E) Black-winged Hatchetfish, Carnegiella marthae G.S. Myers,; (F) Great white shark, Carcharodon carcharias (L.); (G) Amazonian Foam frog, Engystomops sp. Jiménez de la Espada; (H) Eastern stone gecko, Diplodactylus vittatus Gray; (I) Yellow-vented bulbul, Pycnonotus goiavier (Scopoli); (J) Grey-bellied squirrel, Callosciuris caniceps (J.E. Gray), subsp. nov. caniceps (Hinkley); (K) Nine-banded armadillo, Dasypus novemcinctus L.; (L) Pipistrelle bat, Pipistrellus pipistrellus (Schreber); (M) Bornean short-tailed gymnure, Hylomys dorsalis Thomas, sp. nov. (Hinckley); (N) Killer whale, Orcinus orca (L.). Source: © Photo credits: (A) Malcolm Storey, www.bioimages.org.uk; (B) Oklahoma State University, USA; (C) jessica-joachim.com/; (D) Author, ex-Rothamsted Research, Harpenden, Herts., UK; (E) tropical-fish-keeping.com; (F) Herbert Futterknecht, sharkproject.org; (G) Santiago Ron, amphibiaweb.org; (H) Matt Clancy, Melbourne, Australia, CC BY 2.0 ; (I) cokesmithwildlife.com; (J) Natthaphat Chotjuckdikul, Bangkok, Thailand, www.inaturalist.org/observations/50531679; (K) nwf.org; (L) David Pye (Pye, 2021), Royal Meteorological Society; (M) Quentin Martinez, www.quentinmartinez.fr; (N) victoriawhalewatching.com.
Examples of how dietary specialists and so-called generalists fare when faced with novel food resources include a recent study on the polyphagous black-bean aphid, Aphis fabae Scopoli reared in laboratory culture on five vascular host plants invasive to Europe (Ameline et al., 2025). The results revealed a gradient of suitability in terms of aphid probing behaviour, short-term survival and fecundity in clip cages, and their ability to settle and form colonies over a five week period. So as this study shows, there is not a clear ‘all or nothing’ response to the host plants on offer (cf. Ameline et al., 2025, for further details). Another paper on this broad topic area, concerning study of the dietary specialisation of frugivorous birds in south-east Peru (Bender et al., 2017), found that ‘Functional specialisation of bird species varied more among species than across seasons, and phylogenetically similar bird species showed similar degrees of functional niche breadth and functional originality.’ Furthermore ‘that birds with high functional flexibility across seasons had narrow functional niche breadth and high functional originality per season, suggesting that birds that are seasonally specialised on particular resources are most flexible in switching to other fruit resources across seasons.’ The authors conclude that ‘The high flexibility of functionally specialised bird species to switch seasonally to other resources challenges the view that consumer species rely on functionally similar resources throughout the year.’
All well and good, but as these two studies both clearly demonstrate: (1) generalism, if it exists at all, is a ‘moving feast’, and is not an exact behavioural trait in terms of what is eaten, excluding preferred resources, and 2) if animals feed on seasonally-available resources, they show a temporally-based specialism. In this light, the term generalism is clearly an unsatisfactory one and levels of phagy – mono- oligo- and polyphagy − are more accurate to describe what we see in nature and as such, are surely to be preferred.
Certainly, assumed generalism is typically uncommon compared with specialism and specialist species (Leonard and Lancaster, 2022), and in the case of hymenopterous parasitoids (Insecta) “The generalist vs. specialist strategy for a parasite consists of increasing or reducing host range, where host range is defined as the number of host species infected by a population of a single parasite species (Lymbery, 1989). Host range reflects a reciprocal relationship between host and parasite (Ward, 1992).” (cited in Sasal et al., 2001). Specialists have a much more restricted diet and often geographical range compared with so-called generalists, which in contrast, show a wider range of dietary items and often a larger geographical range, probably involving a much wide range of topography too, e.g. American Black bear (Jacoby et al., 1999; Baldwin and Bender, 2009), Racoon, Procyon lotor (L.) (Harman and Stains, 1979) and Brown rat, Rattus norvegicus Berkenhout (Munshi-South et al., 2024), and hence climatic conditions. But, as aforementioned, there are doubtless constraining costs of being a generalist, and our belief is that ‘generalism’ is most often confused with opportunism, as seen for example in brown bears and black bears and red foxes and other carnivores with omnivorous dietary intake.
The specialist has, so long as the resource lasts, little overt interspecific competition for that resource, e.g. Koala, Phascolarctos cinereus (Goldfuss) feeding on eucalyptus (cf. Moore and Foley, 2000, including in relation to Koala host plant feeding preferences). In contrast, the ‘generalist’ is always likely to have to endure competition from rivals for one or more resources, either intra- or interspecific; such resources are in turn likely to be limiting in amount and variety. If the resource of the specialist becomes limiting for whatever reason, e.g. severe drought or bush fires in the case of eucalypts and Koalas, this then could prove catastrophic, leading to local or complete population extinction. In contrast, assuming the competition is not extreme, the oligo- or polyphagous/omnivorous animal can withstand short-term reduction in resource availability … that is, if one or a few particular resource items were to become scare and thereby limiting. Clearly, there are pros and cons of either extreme dietary scenario, and hence, trade-offs (Futuyma and Moreno, 1988). In the case of insect herbivores especially, and probably many other herbivorous animals too, their diet breadth is constrained by toxic plant secondary compounds (Schoonhoven, 1982; Barik, 2021), thus limiting the number and variety of plants attacked (cf. also Fox and Morrow, 1981 and Jaenike, 1990)….unless that is, they have co-evolved (Thompson, 1994), perhaps over huge swathes of time, to combat these in various ways, e.g. by detoxification, degradation, excretion, or sequestration (War et al., 2020). There may also be other, anatomical co-evolved antifeedant mechanisms at work, e.g. sticky hairs, spikes and spines (War et al., 2020).
If generalism was such a successful approach to maintaining species individuals and by extension, populations, why is it not more common in the natural world? From a casual perspective, it seems a highly reasonable and sustainable practice. But there are clearly problems associated with this lifestyle.
From examination of extinct fauna, it is often evident from skeletal and/or fossil remains what the lifestyle of the said animal probably was, especially upon examination of the skull, i.e. jaws and teeth, placing of the eye sockets (e.g. forward or side facing), and nature of the limbs and feet (e.g. hooves or claws). Certainly the fossil evidence, following examination of a large number of extinct taxa, suggests that these animals were predominantly specialist in terms of their lifestyle and hence diet, e.g. birds (Fuller, 1987), dinosaurs (Barrett, 2024) and pterosaurs (Witton, 2018; Chang-Fu et al., 2017; Bestwick et al., 2018; Cooper et al., 2024).
Having said that, there is of course always likely to be some degree of guess work attached to the assignment of dietary habits and preferences of extinct animals, more especially long extinct ones with no living descendants or close relatives whatsoever (‘Utterly extinct’ taxa to use the term Darwin, 1859 used, p. 126), the behaviour of which is conjectural. Even so, it is surely clear from the fossilised remains of Mesozoic animals such as allosaurs and raptors that these were rapacious predators (Barrett, 2024), and filter-feeding pterosaurs were undoubtedly harvesting small water-borne creatures such as small fish and crustaceans (Chang-Fu et al., 2017) (but cf. also Seilacher, 2007).
Predatory animals may be opportunist, for example European Pine Marten, Martes martes (L.) and Red Fox, Vulpes vulpes (L.) when they come across supplementary food items, including bird’s eggs or berries. But it is obvious from their anatomy that they are essentially highly adapted predatory creatures. In that case, their diet breadth probably consists of a range of prey species they are capable of tackling, i.e. not too big or ferocious, or too small, thereby eliciting problems associated with cost-benefit. There are also cost benefit considerations in relation to how much energy is required to capture a particular species individual. In the case of the Pine Marten in the UK, they have co-existed with the Red Squirrel, Sciurus vulgaris L. for thousands of years, whereas the Grey Squirrel, Sciurus carolinensis Gmelin, originally introduced from North America into various sites in Britain, notably Woburn Abbey in Bedfordshire at the turn of the 20th century (Okubo et al., 1989), is apparently easier to capture and thus populations of Reds and Greys are attacked disproportionately, the net effect being that reds are thriving at the expense of the less adapted (to Pine Marten predation) greys (Sheehy et al., 2018).
An animal species, be it predator or herbivore, may have a broad diet breadth, but they usually evolve to feed on one or a few preferred species or food items that they have become especially adapted to finding, catching/collecting, devouring and digesting, and these prey or items may constitute a very high percentage of the diet, as found with wild cats, Felis silvestris Schreber in south-east Spain, where mice and voles account for around 80% of the diet (cf. Moleón and Gil-Sanchez, 2003 for further details). In this way, as the authors describe it, this predator is a facultative specialist, despite its broad diet breadth.
If it is essentially true that generalist animals have costs in terms of their lifestyle, yet so, there are good examples of animals such as omnivorous Racoons or bears which seemingly ‘buck the trend’ of evolution….apparently. But we suggest that bears like Black bears and Brown bears, Ursus arctos L., are highly adapted in terms of their physiology (they are the only large animals to hibernate during the winter time and are the only large predators capable of standing in freezing torrents of water to catch salmon in their mouths). They tend to be seasonal specialists, depending on what main food is abundant at any point in the year (e.g. Mikkelsen et al., 2024). Their forward facing eyes and carnassial dentition (flattened and less developed than in other carnivores) support their predominantly predatory-omnivorous life style (Sacco and Van Valkenburg, 2004).
Besides a seasonal-temporal aspect to so-called generalism, or in reality, seasonal specialism, as aforementioned, some generalist animals seems to show a regional/geographic aspect to their diet breadth (Loxdale and Harvey, 2023). But as well as this, as mentioned by Nery et al. (2023), ecological specialisation can lead to a restriction in the distribution of some less vagile species, leading to endemism. Thus the concept of generalism again is a somewhat flexible one, depending on the regional and seasonal availability of food items.
Of course, as is well known, biodiversity increases at the equator and declines towards the poles. In this sense, animals living in more polar regions are likely to have less choice in what they eat, e.g. arctic fox, Vulpes lagopus (L.) and caribou, Rangifer tarandus (L.), which in turn is likely to drive dietary specialisms (Latitudinal diversity gradient hypothesis) (cf. Dyer and Forister, 2019 and references therein). The same can be said for animals with a wide geographical range and perhaps living at the extremes of this, e.g., Montagu’s harrier, Circus pygargus (L.) (Terraube and Arroyo, 2011).
If the driving mechanism of evolution is via ecological specialism, yet some animals appear to be generalist in terms of their diet breadth, this is indeed a paradox. Can the ‘circle ever be squared’ and a rational and reasonable explanation be proffered as to why such animals have taken to this ultimately risky lifestyle? Indeed, can a generalist animal evolve and speciate into new, more specialist forms (which is what is assumed, cf. Futuyma and Moreno, 1988), or is it ultimately a victim of its own opportunistic gamble in the great scheme of life and as such inevitably doomed to extinction?
Another very important aspect of the whole specialism vs. generalism debate is the topic of cryptic species (Bickford et al., 2007, cf. their Figure 1; Hending, 2024) or morphologically similar-identical lower levels of evolutionary divergence, including genetically divergent species populations, e.g. De Barro et al. (1995) and Sunnucks et al. (1997) in the case of cereal aphids. Thus in these particular insects, the predominantly asexual English grain aphid, Sitobion avenae (F.), which feeds preferentially – but not exclusively – on cultivated wheat, Triticum aestivum L (Loxdale and Brookes, 1990; Blackman, 2010), shows introgression with its sister species, the rose-grain aphid, S. fragariae (Walker), which has an autumnal sexual phase, host alternating between a range of summer (or secondary) gramineous hosts and a woody overwintering host, especially bramble, Rubus fruticosus L. agg., on which mated sexual females (oviparae) lay cold hardy eggs (Blackman and Eastop, 2000; Blackman, 2010). In contrast, S. avenue sensu stricto when it does produce sexual forms, as a result of low light and temperature conditions, lays its eggs on the secondary, gramineous host/s (Helden and Dixon, 2002).
It is now known that many species populations undergo introgression/hybridisation. According to Mallet (2005, 2007), “The fraction of species that hybridize is variable, but on average around 10% of animal and 25% of plant species are known to hybridize with at least one other species (Mallet, 2005). Hybridization is especially prevalent in rapidly radiating groups: 75% of British ducks (Anatidae), for example.” (Mallet, 2005). Most such hybrids have maladaptive traits and die out, but some hybrid species are known to exist (Mallet, 2007). An example is Schwartz’s fruit-eating bat, Artibeus schwartzi Jones, found in the Lesser Antilles of the West Indies, and apparently originating from three precursor species, two still extant (Larsen et al., 2010).
In addition to this, within the last few decades, numerous examples of cryptic species have been discovered globally, often suspected – but now proven – using allozymes markers and high resolution molecular (DNA) markers, including microsatellites, mitochondrial DNA markers, and DNA sequencing (Oliver et al., 2009). These include animals (Figure 1) as diverse as earthworms (King et al., 2008), insects, e.g. aphids (e.g. Foottit et al., 2008; Rebijith et al., 2013; Lee et al., 2015; Li et al., 2023), hymenopterous parasitic wasps (e.g. Atanassova et al., 1998; Heraty et al., 2007; Derocles et al., 2016); arachnids (e.g. Loria et al., 2025); crustacea (Patoka et al., 2025); molluscs (Wesselingh et al., 2019); fish (e.g. Kon et al., 2007; Piggott et al., 2011), amphibians (e.g. Funk et al., 2012; Marr et al., 2024), reptiles (e.g. Oliver et al., 2007, 2009; Vasconcelos et al., 2016; Agarwal et al., 2017), birds (e.g. Lohman et al., 2010; Sands et al., 2024), and mammals (e.g. Brown et al., 2014; Fennessy et al., 2016; Barthe et al., 2024; Hinckley et al., 2024a, 2024b; Zeng et al., 2024; cf. also Videvall et al., 2025), including bats (e.g. Mayer and von Helversen, 2001; Clare, 2011) and even killer whales (Filatova et al., 2023). It is known that some of these cryptic entities are formed by chromosomal inversion polymorphisms or other small or large scale mutational changes e.g. translocations or mitochondrial DNA, which differentiate the new population genetically from the parental one and are perhaps integral in this separation, e.g. voles of the genus Microtus (e.g. Mazurok et al., 2001; Lavrenchenko et al., 2023) and mole voles, Ellobius (Romanenko et al., 2019) (cf. also Patton and Sherwood, 1983).
Furthermore, there is evidence of reproductively-isolated sub-specific forms, everything from butterflies, e.g. African Queen butterfly, Danaus chrysippus (L.) (Smith, 2014) and Heliconius species (Brower and Garzón-Orduña, 2024) to the African Lion, Panthera leo (L.) (Bertola et al., 2015). The fact that these populations are allopatrically and even sometimes sympatrically reproductively isolated, for example in the case of host plant adapted insect species (Craig et al., 1993), strongly implies that they are fulfilling slightly different ecological roles, something reflected in their diet breadth/preferences (cf. Leung and Beukeboom, 2022, in relation to the various known or theorised mechanisms of host plant adaptation in insects, including phenotypic plasticity). In the case of morphologically-similar populations (so-called ecotypes but perhaps in reality true cryptic sub-species) of Killer Whales, Orcinus orca (L.), these have different diet specialisations, including at one extreme, salmon (Vester and Hammerschmidt, 2013), at the other, the hunting and predation of seals and baleen whales (Filatova et al., 2023; cf. also Foote et al., 2011 and McInnes et al., 2024). Genetic differentiation of Great White Sharks, Carcharodon carcharias (L.), has similarly also recently been reported, representing three distinct main lineages (North Atlantic, Indo-Pacific, and North Pacific) which diverged some 100,000–200,000 years ago (Wagner et al., 2024); these ecotypes may well be related to differences in their diet. Hence, the labelling of a particular species as ‘generalist’ has to be made with extreme care. Certainly, as an example of how incorrect identification of species can lead to spurious interpretation of diet breadth, two cryptic European bat species, Pipistrellus pipistrellus (Schreber) and Pipistrellus pygmaeus (Leach), are known to have different habitats and hence somewhat different diets (cf. Mayer and von Helversen, 2001; Dick and Roche, 2017).
Basically, our lack of knowledge of many, perhaps most, species in terms of morphologically similar-identical forms acts as a break on our enthusiasm to label such and such a species as highly polyphagous, or to put less scientifically, a generalist. For example, some species of highly polyphagous aphid, often denoted as being global pests, e.g. Peach-potato aphid, Myzus persicae (Sulzer), and which reputedly attacks plants in 40 families (Blackman, 2010; Blackman and Eastop, 2000), may be an array of cryptic, host adapted species or sub-specific entities (Loxdale et al., 2011). However, recent research by Nio et al. (2025) argues against this notion, showing rather that four superclones (multilocus genotypes found in many copies) of this species studied in northern France using molecular approaches can infest plants of a wide variety of unrelated taxa, but especially sugar beet (Beta vulgaris L.) (≥ 80% on this crop). As the authors continue “Moreover, the array of characterized genotypes of M. persicae formed distinct genetic clusters, but with no clear association to specific host plants”, suggesting that the generalist characteristic of this aphid do indeed hold. Whatever, this aphid host alternates between a secondary summer herbaceous host or hosts, e.g. brassicales, and an overwintering winter host, i.e. Peach, Prunus persica (L.) Batsch on which the sexual females, after mating, lay cold hardy eggs.
So even if it is true that the aphid is generalist (polyphagous) on the secondary host, it is clearly predominantly specialist (monophagous) on the winter host (Tatchell et al., 1983). A conundrum for sure, not only in terms of semantics, but in terms of biological labelling too. As a last thought on this particular case, it could be further argued that the ability on the part of M. persicae to attack a large number of hosts, many with unique antifeedant chemical signatures, is in itself an ecological specialism related to the particular genetic-biochemical properties it has evolved (cf. Mathers et al., 2017 for details), since most aphid species do not show such adaptability, specialising on plants within the same family of plants or on single plant species (Blackman, 2010).
What we the organisers of this special issue of the journal hope is that the debate may be widened and more especially that the application of the term generalist and generalism not be taken as a given. That without firm corroboratory evidence, especially using polymorphic DNA markers and sequencing, the genetic identity of a given species population cannot be assumed to be uniform over its geographical range. And thereby, that the reality of generalism as a concept is either supported or ultimately, which we have come to believe, be denied due to the factors as briefly outlined above. To us, this is a critical issue in ecology and evolution, one that deserves to be taken more seriously and re-explored in order to uncover the truth as to what is pertaining, or thought to pertain, for the animal species deemed to be generalists.
In the end, if we biologists use factually incorrect terminology, this is likely to hold back the acquisition of new and important data and a more realistic view of what is actually occurring within the natural world. This paradox of course impinges on both invertebrates and vertebrates and ultimately, impinges on our understanding – or so we believe – of the evolutionary process occurring by genetic population divergence brought about by mutations of one form or another, natural selection/genetic drift, adaptation and ecological specialisation.
Author contributions
HDL: Conceptualization, Project administration, Writing – original draft. AB: Conceptualization, Writing – review & editing.
Funding
The author(s) declare that no financial support was received for the research and/or publication of this article.
Acknowledgments
We thank Dr. Lydia Morley, Texas A&M University, USA and Professor Stuart Reynolds, Emeritus Professor at Bath University, UK for their valuable comments on the manuscript of this paper. We also sincerely thank the reviewer, Dr Sara Gamboa, for her insightful comments which have broadened our outlook on certain topics addressed in the revision of this paper, which have undoubtedly improved the article.
Conflict of interest
The authors declare that the research was conducted in the absence of any commercial or financial relationships that could be construed as a potential conflict of interest.
Generative AI statement
The author(s) declare that no Generative AI was used in the creation of this manuscript.
Publisher’s note
All claims expressed in this article are solely those of the authors and do not necessarily represent those of their affiliated organizations, or those of the publisher, the editors and the reviewers. Any product that may be evaluated in this article, or claim that may be made by its manufacturer, is not guaranteed or endorsed by the publisher.
References
Agarwal I., Biswas S., Bauer A. M., Greenbaum E., Jackman T. R., De Silva A., et al. (2017). Cryptic species, taxonomic inflation, or a bit of both? New species phenomenon in Sri Lanka as suggested by a phylogeny of dwarf geckos (Reptilia, Squamata, Gekkonidae, Cnemaspis). Syst. Biodivers. 15, 427–439. doi: 10.1080/14772000.2017.1282553
Aljbory Z., Chen M.-S. (2018). Indirect plant defense against insect herbivores: a review. Insect Sci. 25, 2–23. doi: 10.1111/1744-7917.12436
Als T. D., Vila R., Kandul N. P., Nash D. R., Yen S.-H., Hsu Y.-F., et al. (2004). The evolution of alternative parasitic life histories in large blue butterflies. Nature 432, 386–390. doi: 10.1038/nature03020
Ameline A., Hebert M., Denoirjean T., Spicher F., Decocq G. (2025). Suitable or not? decoding generalist aphid preferences and performances for alien plants. Biol. Invasions 27 (52). doi: 10.1007/s10530-024-03509-w
Atanassova P., Brookes C. P., Loxdale H. D., Powell W. (1998). Electrophoretic study of five aphid parasitoid species of the genus Aphidius Nees (Hymenoptera: Braconidae), including evidence for reproductively isolated sympatric populations and a cryptic species. Bull. Entom. Res. 88, 3–13. doi: 10.1017/S000748530004150X
Baldwin R. A., Bender L. C. (2009). Foods and nutritional components of diets of black bear in Rocky Mountain National Park, Colorado. Can. J. Zool. 87, 1000–1008. doi: 10.1139/Z09-088
Barik A. (2021). “Phyto-antifeedants,” in Molecular Approaches for Sustainable Insect Pest Management. Ed. Omkar (Springer Nature Singapore Pte Ltd, Singapore), 283–332. doi: 10.1007/978-981-16-3591-5
Barthe M., Rancilhac L., Arteaga M. C., Feijó A., Tilak M.-K., Justy F., et al. (2024). Exon capture museomics deciphers the Nine-banded Armadillo species complex and identifies a new species endemic to the Guiana Shield. Syst. Biol., syae027. doi: 10.1093/sysbio/syae027
Bender I. M. A., Kissling W. D., Böhning-Gaese K., Hensen I., Kühn I., Wiegand T., et al. (2017). Functionally specialised birds respond flexibly to seasonal changes in fruit availability. J. Anim. Ecol. 86, 800–811. doi: 10.1111/1365-2656.12683
Benedek K., Mara G., Mehrparvar M., Bálint J., Loxdale H. D., Balog A. (2019). Near-regular distribution of adult crimson tansy aphids, Uroleucon tanaceti (L.), increases aposematic signal honesty on different tansy plant chemotypes. Biol. J. Linn. Soc 126, 315–326. doi: 10.1093/biolinnean/bly180
Bertola L. D., Tensen L., van Hooft P., White P. A., Driscoll C. A., Henschel P., et al. (2015). Autosomal and mtDNA markers affirm the distinctiveness of lions in west and central Africa. PloS One 10, e01379755. doi: 10.1371/journal.pone.0137975
Bestwick J., Unwin D. M., Butler R. J., Henderson D. M., Purnell M. A. (2018). Pterosaur dietary hypotheses: a review of ideas and approaches. Biol. Revs. 93, 2021–2048. doi: 10.1111/brv.12431
Bickford D., Lohman D. J., Sodhi N. S., Ng P. K. L., Meier R., Winker K., et al. (2007). Cryptic species as a window on diversity and conservation. Trends Ecol. Evol. 22, 148–155. doi: 10.1016/j.tree.2006.11.004
Blackman R. L. (2010). “Aphids - aphidinae (Macrosiphini),” in Handbooks for the Identification of British Insects (Volume 2, Part 7) (Royal Entomological Society-Field Studies Council, Shrewsbury).
Blackman R. L., Eastop V. F. (2000). Aphids on the World’s crops: An identification and information guide. 2nd ed (Chichester: John Wiley & Sons Ltd).
Bonsall M. B., Wright A. E. (2012). Altruism and the evolution of resource generalism and specialism. Ecol. Evol. 2, 515–524. doi: 10.1002/ece3.206
Brower A. V. Z., Garzón-Orduña I. J. (2024). Evolution of mimicry in Heliconius butterflies: Historical hypotheses meet modern models. Antenna (Royal Entomological Soc. Bulletin) 48, (4) 171–176. https://www.researchgate.net/publication/387469549 (Accessed March 14, 2025).
Brown R. M., Weghorst J. A., Olson K. V., Duya M. R. M., Barley A. J., Duya M. V., et al. (2014). Conservation genetics of the Philippine Tarsier: Cryptic genetic variation restructures conservation priorities for an island archipelago primate. PloS One 9, e104340. doi: 10.1371/journal.pone.0104340
Chang-Fu Z., Ke-Qin G., Hongyu Y., Jinzhuang X., Quanguo L. and Fox R. C. (2017). Earliest filter-feeding pterosaur from the Jurassic of China and ecological evolution of Pterodactyloidea. R. Soc Open Sci. 4, 160672. doi: 10.1098/rsos.160672
Clare E. L. (2011). Cryptic species? Patterns of maternal and paternal gene flow in eight neotropical bats. PloS One 6, e21460. doi: 10.1371/journal.pone.0021460
Cooper S. L.A., Smith R. E., Martill D. M. (2024). Dietary tendencies of the Early Jurassic pterosaurs Campylognathoides Strand 1928, and dorygnathus wagner 1860, with additional evidence for teuthophagy in Pterosauria. J. Vertebr. Paleontol. 44 (2). doi: 10.1080/02724634.2024.2403577
Cott H. B. (1940). Adaptive Coloration in Animals, (1966 reprint) (London: Methuen and Co. Ltd), 508.
Craig T. P., Itami J. K., Abrahamson W. G., Horner J. D. (1993). Behavioral evidence for host-race formation in Eurosta solidaginis. Evolution 47, 1696–1710.
Darwin C. (1859). On The Origin of Species by Means of Natural Selection, or the Preservation of Favoured Races in the Struggle for Life (London: John Murray), 502. Available at: https://darwin-online.org.uk/ (Accessed March 14, 2025).
Darwin C. (1862). The Various Contrivances by Which Orchids are Fertilised by Insects, and on the Good Effects of Intercrossing (London: John Murray), 365.
De Barro P. J., Sherratt T. N., Brookes C. P., David O., Maclean N. (1995). Spatial and temporal variation in British field populations of the grain aphid Sitobion avenae (F.) (Hemiptera: Aphididae) studied using RAPD-PCR. Proc. R. Soc B 262, 321–327. Available online at: https://www.jstor.org/stable/50112.
Dehling D. M., Bender I. M. A., Blendinger P. G., Böhning-Gaese K., Muñoz M. C., Neuschulz E. L., et al. (2021). Specialists and generalists fulfil important and complementary functional roles in ecological processes. Funct. Ecol. 35, 1810–1821. doi: 10.1111/1365-2435.13815
Derocles S. A. P., Plantegenest M., Rasplus J.-Y., Marie A., Evans D. M., Lunt D. H., et al. (2016). Are generalist Aphidiinae (Hym. Braconidae) mostly cryptic species complexes? Syst. Entomol. 41, 379–391. doi: 10.1111/syen.12160
Dick A., Roche N. (2017). Google Earth and Google Street View reveal differences in Pipistrellus pipstrellus and Pipistrellus pygmaeus roadside habitat use in Ireland. Ir. Nat. J. 35, 83–93. Available at: www.jstor.org/stable/44577825 (Accessed March 14, 2025).
Dyer L. A., Forister M. L. (2019). Challenges and advances in the study of latitudinal gradients in multitrophic interactions, with a focus on consumer specialization. Curr. Opin. Insect Sci. 32, 68–76. doi: 10.1016/j.cois.2018.11.008
Fennessy J., Bidon T., Reuss F., Vamberger M., Fritz U., Janke A. (2016). Multi-locus analyses reveal four giraffe species instead of one. Curr. Biol. 26, 2543–2549. doi: 10.1016/j.cub.2016.07.036
Filatova O. A., Fedutin I. D., Belonovich O. A., Borisova E. A., Volkova E. V., Ivkovich T. V., et al. (2023). Differences in the diet of reproductively isolated ecotypes of Killer Whales (Orcinus orca Linnaeus 1758) in the seas of the Russian Far East. Russ. J. Mar. Biol. 49, 477–487. doi: 10.1134/S1063074023060032
Foote A. D., Vilstrup J. T., De Stephanis R., Verborgh P., Abel Nielsen S. C., Deaville R., et al. (2011). Genetic differentiation among North Atlantic killer whale populations. Mol. Ecol. 20, 629–641. doi: 10.1111/j.1365-294X.2010.04957.x
Foottit R. G., Maw H. E. L., Von Dohlen C. D., Hebert P. D. N. (2008). Species identification of aphids (Insecta: Hemiptera: Aphididae) through DNA barcodes. Mol. Ecol. Res. 8, 1189–1201. doi: 10.1111/j.1755-0998.2008.02297.x
Fox L. R., Morrow P. A. (1981). Specialization: species property or local phenomenon? Science 211, 887–891. doi: 10.1126/science.211.4485.887
Funk W. C., Caminer M., Ron S. R. (2012). High levels of cryptic species diversity uncovered in Amazonian frogs. Proc. R. Soc B 279, 1806–1814. doi: 10.1098/rspb.2011.1653
Futuyma D. J., Moreno G. (1988). The evolution of ecological specialization. Ann. Rev. Ecol. Syst. 19, 207–233. doi: 10.1146/annurev.es.19.110188.001231
Harman D. M., Stains H. J. (1979). The Raccoon (Procyon lotor) on St. Catherine’s Island, Georgia. 5. Winter, spring, and summer food habits. Am. Mus. Novit. 2679, 1–24.
Helden A. J., Dixon A. F. G. (2002). Life-cycle variation in the aphid Sitobion avenae: costs and benefits of male production. Ecol. Entomol. 27, 692–701. doi: 10.1046/j.1365-2311.2002.00451.x
Hending D. (2024). Cryptic species conservation: a review. Biol. Revs. 100 (1), 258–274. doi: 10.1111/brv.13139
Heraty J. M., Woolley J. B., Hopper K. R., Hawks D. L., Kim J.-W., Buffington M. (2007). Molecular phylogenetics and reproductive incompatibility in a complex of cryptic species of aphid parasitoids. Mol. Phylogenet. Evol. 45, 480–493. doi: 10.1016/j.ympev.2007.06.021
Hinckley A., Camacho-Sanchez M., Chua M. A. H., Ruedi M., Lunde D., Maldonado J. E., et al. (2024a). An integrative taxonomic revision of lesser gymnures (Eulipotyphla: Hylomys) reveals five new species and emerging patterns of local endemism in Tropical East Asia. Zool. J. Linn. Soc 202, 1–33. doi: 10.1093/zoolinnean/zlad177
Hinckley A., Maldonado J. E., Tamura N., Leonard J. A., Hawkins M. T. R. (2024b). Lost in synonymy: Integrative species delimitation reveals two unrecognized species of Southern Asian tree squirrels (Rodentia: Sciuridae: Callosciurinae). Vertebr. Zool. 74, 683–707. doi: 10.3897/vz.74.e133467
Jacoby M. E., Hilderbrand G. V., Servheen C., Schwartz C. C., Arthur S. M., Hanley T. A., et al. (1999). Trophic relations of brown and black bears in several western North American ecosystems. J. Wildl. Manage. 63, (3) 921–929. doi: 10.2307/3802806
Jaenike J. (1990). Host specialization in phytophagous insects. Annu. Rev. Ecol. Syst. 21, 243–273. doi: 10.1146/annurev.es.21.110190.001331
Kapun M., Flatt T. (2019). The adaptive significance of chromosomal inversion polymorphisms in Drosophila melanogaster. Mol. Ecol. 28, 1263–1282. doi: 10.1111/mec.14871
King R. A., Tibble A. L., Symondson W. O. C. (2008). Opening a can of worms: unprecedented sympatric cryptic diversity within British lumbricid earthworms. Mol. Ecol. 17, (21) 4684–469. doi: 10.1111/j.1365-294X.2008.03931.x
Kon T., Yoshino T., Mukai T., Nishida M. (2007). DNA sequences identify numerous cryptic species of the vertebrate: A lesson from the gobioid fish Schindleria. Mol. Phylogenet. Evol. 44, 53–62. doi: 10.1016/j.ympev.2006.12.007
Krause J., Unger T., Noçon A., Malaspinas A., Kolokotronis S., Stiller M., et al. (2008). Mitochondrial genomes reveal an explosive radiation of extinct and extant bears near the Miocene-Pliocene boundary. BMC Evol. Biol. 8, 220. doi: 10.1186/1471-2148-8-220
Larsen P. A., Marchán-Rivadeneira M. R., Baker R. J. (2010). Natural hybridization generates mammalian lineage with species characteristics. PNAS 107, 11447–11452. doi: 10.1073/pnas.1000133107
Lavrenchenko L. A., Gromov A. R., Martynov A. A., Mironova T. A., Sycheva V. B., Kostin D. S., et al. (2023). Genetic, chromosomal and phenotypic variation across a hybrid zone between two common vole species (Microtus arvalis and M. obscurus). Hystrix Ital. J. Mammal 34, 24–32. Available online at Researchgate: https://www.researchgate.net/profile/Leonid-Lavrenchenko/publication/369661849.
Lee Y., Lee W., Lee S., Kim H. (2015). A cryptic species of Aphis gossypii (Hemiptera: Aphididae) complex revealed by genetic divergence and different host plant association. Bull. Entomol. Res. 105, 40–51. doi: 10.1017/S0007485314000704
Leonard A. M., Lancaster L. T. (2022). Evolution of resource generalism via generalized stress response confers increased reproductive thermal tolerance in a pest beetle. Biol. J. Linn. Soc 37, 374–386. doi: 10.1093/biolinnean/blac082
Leung K., Beukeboom L. W. (2022). Fundamental and specialized questions on host race formation and speciation–an introduction. Entomol. Exp. Appl. 170 (1), 2–4. doi: 10.1111/eea.13124. Special issue: Host race formation and speciation.
Li Q., Liu Q., Yu Y., Lin X., He X., Huang X. (2023). Revealing cryptic diversity and population divergence in subtropical aphids through DNA barcoding. Zool. Scr. 52, 517–530. doi: 10.1111/zsc.12613
Lohman D. J., Ingram K. K., Prawiradilaga D. M., Winker K., Sheldon F. H., Moyle R. G., et al. (2010). Cryptic genetic diversity in “widespread” Southeast Asian bird species suggests that Philippine avian endemism is gravely underestimated. Biol. Conser. 143, 1885–1890. doi: 10.1016/j.biocon.2010.04.042
Loria S. F., Frank S.-C., Dupérré N., Smith H. M., Jones B., Buzatto B. A., et al. (2025). The world’s most venomous spider is a species complex: systematics of the Sydney funnel-web spider (Atracidae: Atrax robustus). BMC Ecol. Evol. 25. doi: 10.1186/s12862-024-02332-0
Loxdale H. D. (2010). Rapid genetic changes in natural insect populations. Ecol. Ent. 35, 155–164. doi: 10.1111/j.1365-2311.2009.01141.x
Loxdale H. D. (2023). Are some brightly coloured European birds toxic? Front. Ecol. Evol. 5, 1150576. doi: 10.3389/fevo.2023.1150576
Loxdale H. D., Balog A., Harvey J. A. (2019). Generalism in nature … the great misnomer: Aphids and wasp parasitoids as examples. Insects 10, 314. doi: 10.3390/insects10100314
Loxdale H. D., Brookes C. P. (1990). Prevalence of Sitobion fragariae (Walker) over S. avenae (Fabricius) (Hemiptera: Aphididae) on wild cocksfoot grass (Dactylis glomerata) in south-east England. Bull. Entomol. Res. 80, 27–29. doi: 10.1017/S0007485300045879
Loxdale H. D., Harvey J. A. (2016). The ‘generalism’ debate: misinterpreting the term in the empirical literature focusing on dietary breadth in insects. Biol. J. Linn. Soc 119, 265–282. doi: 10.1111/bij.2016.119.issue-2
Loxdale H. D., Harvey J. A. (2023). Generalism in nature: a community ecology perspective. Community Ecol. 24, 113–125. doi: 10.1007/s42974-022-00130-6
Loxdale H. D., Lushai G., Harvey J. A. (2011). The evolutionary improbability of ‘generalism’ in nature, with special reference to insects. Biol. J. Linn. Soc 103, 1–18. doi: 10.1111/j.1095-8312.2011.01627.x
Lymbery A. J. (1989). Host specificity, host range and host preference. Parasitol. Today 5, 298. doi: 10.1016/0169-4758(89)90021-5
Mallet J. (2005). Hybridization as an invasion of the genome. Trends Ecol. Evol. 20, 229–237. doi: 10.1016/j.tree.2005.02.010
Marr M. M., Hopkins K., Tapley B., Borzée A., Liang Z., Cunningham A. A., et al. (2024). What’s in a name? Using species delimitation to inform conservation practice for Chinese giant salamanders (Andrias spp.). Evol. J. Linn. Soc 3, kzae007. doi: 10.1093/evolinnean/kzae007
Martill D. M., Smith R. E. (2024). “Cretaceous pterosaur history, diversity and extinction,” in Cretaceous Project 200 Volume 1: The Cretaceous World, vol. 544 . Eds. Hart M. B., Batenburg S. J., Huber B. T., Price G. D., Thibault N., Wagreich M., Walaszczyk I. (Geological Society of London, Special Publication, London). doi: 10.1144/SP544-2023-126
Mathers T. C., Chen Y., Kaithakottil G., Legeai F., Mugford S. T., Baa-Puyoulet P., et al. (2017). Rapid transcriptional plasticity of duplicated gene clusters enables a clonally reproducing aphid to colonise diverse plant species. Genome Biol. 18, 27. doi: 10.1186/s13059-016-1145-3
Mayer F., von Helversen O. (2001). Cryptic diversity in European bats. Proc.R. Soc B 268. doi: 10.1098/rspb.2001.1744
Mazurok N. A., Rubtsova N. V., Isaenko A. A., Pavlova M. E., Slobodyanyuk S. Y., Nesterova T. B., et al. (2001). Comparative chromosome and mitochondrial DNA analyses and phylogenetic relationships within common voles (Microtus, Arvicolidae). Chromosome Res. 9, 107–120. doi: 10.1023/A:1009226918924
McInnes J., Trites A. W., Mathieson C., Dahlheim M. E., Moore J. E., Olson P. A., et al. (2024). Evidence for an oceanic population of killer whales (Orcinus orca) in offshore waters of California and Oregon. Aquat. Mamm. 50, 93–106. doi: 10.1578/AM.50.2.2024.93
Mikkelsen A. J., Hobson K. A., Sergiel A., Hertel A. G., Selva N., Zedrosser A. (2024). Testing foraging optimization models in brown bears: Time for a paradigm shift in nutritional ecology? Ecology 105. doi: 10.1002/ecy.4228
Moleón M., Gil-Sanchez J. M. (2003). Food habits of the wildcat (Felis silvestris) in a peculiar habitat: the Mediterranean high mountain. J. Zool. 260, 17–22. doi: 10.1017/S0952836902003370
Monti V., Lombardo G., Loxdale H. D., Manicardi G. C., Mandrioli M. (2012). Continuous occurrence of intra-individual chromosome rearrangements in the peach potato aphid, Myzus persicae (Sulzer) (Hemiptera: Aphididae). Genetica 140, 90–103. doi: 10.1007/s10709-012-9661-x
Moore B. D., Foley W. J. (2000). A review of feeding and diet selection in koalas (Phascolarctos cinereus). Aust. J. Zool. 48, 317–333. doi: 10.1071/ZO99034
Morley L., Crain B. J., Krupnick G., Spalink D. (2024). Turnover importance: Operationalizing beta diversity to quantify the generalism continuum. Methods Ecol. Evol. 15, 951–964. doi: 10.1111/2041-210X.14324
Munshi-South J., Garcia J. A., Orton D., Phifer-Rixey M. (2024). The evolutionary history of wild and domestic brown rats (Rattus norvegicus). Science 385, 1292–1297. doi: 10.1126/science.adp1166
Nery E. K., Caddah M. K., Santos M. F., Nogueira A. (2023). The evolution of ecological specialization underlies plant endemism in the Atlantic Forest. Ann. Bot. 131, 921–940. doi: 10.1093/aob/mcad029
Nio Y., Buchard C., DuvaL F., Maheo F., Buzy S., Le Ralec A., et al. (2025). Host specialisation or generalism? Population genetics of the aphid Myzus persicae reveals dominance of superclones across diverse host plants. This issue.
Nowak R. M. (1991). Walker’s Mammals of the World Vol. 2 (Baltimore & London: The John Hopkins University Press), 1629.
Okubo A., Maini P. K., Williamson M. H., Murray J. D. (1989). On the spatial spread of the grey squirrel in Britain. Proc. R. Soc B. 238, 113–125. doi: 10.1098/rspb.1989.0070
Oliver P. M., Adams M., Lee M. S. Y., Hutchinson M. N., Doughty P. (2009). Cryptic diversity in vertebrates: molecular data double estimates of species diversity in a radiation of Australian lizards (Diplodactylus, Gekkota). Proc. R. Soc B 276, 2001–2007. doi: 10.1098/rspb.2008.1881
Oliver P., Hugall A., Adams M., Cooper S. J. B., Hutchinson M. (2007). Genetic elucidation of cryptic and ancient diversity in a group of Australian diplodactyline geckos: The Diplodactylus vittatus complex. Mol. Phylogenet. Evol. 44, 77–88. doi: 10.1016/j.ympev.2007.02.002
Patoka J., Akmal S. G., Bláha M., Kouba A. (2025). Cherax pulverulentus, a new freshwater crayfish (Decapoda: Parastacidae) from Southwest Papua Province, Indonesia. Zootaxa 5566, 522–534. doi: 10.11646/zootaxa.5566.3.4
Patton J. L., Sherwood S. W. (1983). Chromosome evolution and speciation in rodents. Ann. Rev. Ecol. System. 14, 39–58. doi: 10.1146/annurev.es.14.110183.001035
Peccoud J., Simon J.-C. (2010). The pea aphid complex as a model of ecological speciation. Ecol. Ent. 35, 119–130. doi: 10.1111/j.1365-2311.2009.01147.x
Piggott M. P., Chao N. L., Beheregaray L. B. (2011). Three fishes in one: cryptic species in an Amazonian floodplain forest specialist. Biol. J. Linn. Soc 102, 391–403. doi: 10.1111/j.1095-8312.2010.01571.x
Rebijith K. B., Asokan R., Kumar N. K. K., Krishna V., Chaitanya B. N., Ramamurthy V. V. (2013). DNA barcoding and elucidation of cryptic aphid species (Hemiptera: Aphididae) in India. Bull. Entomol. Res. 103, 601–610. doi: 10.1017/S0007485313000278
Romanenko S. A., Lyapunova E. A., Saidov A. S., O’Brien P. C. M., Serdyukova N. A., Ferguson-Smith M. A., et al. (2019). Chromosome translocations as a driver of diversification in Mole Voles Ellobius (Rodentia, Mammalia). Int. J. Mol. Sci. 20, 4466. doi: 10.3390/ijms20184466
Sacco T., Van Valkenburg B. (2004). Ecomorphological indicators of feeding behaviour in the bears (Carnivora: Ursidae). J. Zool. 263, 41–54. doi: 10.1017/S0952836904004856
Sampaio E. M., Kalko E. K. V., Bernard E., Rodríguez-Herrera B., Handley J. C. O. (2003). Biodiversity assessment of bats (Chiroptera) in a tropical lowland rainforest of central Amazonia, including methodological and conservation considerations. Stud. Neotrop. Fauna Environ. 38, 17–31. doi: 10.1076/snfe.38.1.17.14035
Sands A. F., Andersson A. A. L., Reid K., Hains T., Joseph L., Drew A., et al. (2024). Genomic and acoustic biogeography of the iconic sulphur-crested cockatoo clarifies species limits and patterns of intraspecific diversity. MBE 41, msae222. doi: 10.1093/molbev/msae222
Sasal P., Trouvé S., Müller-Graf C., Morand S. (2001). Specificity and host predictability: a comparative analysis among monogenean parasites of fish. J. Anim. Ecol. 68, 437–444. doi: 10.1046/j.1365-2656.1999.00313.x
Schoonhoven L. M. (1982). Biological aspects of antifeedants. Entomol. Exp. Appl. 31, 57–69. doi: 10.1111/j.1570-7458.1982.tb03119.x. Special Issue: Insect Control of Tomorrow, January 1982.
Seilacher A. (2007). Arbeitskonzept zur konstruktions-morphologie. Lethaia 3, 393–396. doi: 10.1111/j.1502-3931.1970.tb00830.x
Sheehy E., Sutherland C., O’Reilly C., Lambin X. (2018). The enemy of my enemy is my friend: Native pine marten recovery reverses the decline of the red squirrel by suppressing grey squirrel populations. Proc. R. Soc B. 285, 2017–2603. doi: 10.1098/rspb.2017.2603
Smith D. A. S. (2014). African Queens and their Kin: a Darwinian odyssey (Taunton, UK: Brambleby Books Ltd).
Stajic D., Jansen L. E. T. (2021). Empirical evidence for epigenetic inheritance driving evolutionary adaptation. Phil. Trans. R. Soc B. 376. doi: 10.1098/rstb.2020.0121
Sunnucks P., De Barro P. J., Lushai G., Maclean N., Hales D. (1997). Genetic structure of an aphid studied using microsatellites: cyclic parthenogenesis, differentiated lineages, and host specialization. Mol. Ecol. 6, 1059–1073. doi: 10.1046/j.1365-294X.1997.00280.x
Tamisiea J. (2024). Scientists at the National Museum of Natural History discover two squirrel species long obscured by mistaken identities (Smithsonian voices from the Smithsonian museums). Available at: https://www.smithsonianmag.com/blogs/national-museum-of-natural-history/2024/11/13/scientists-at-the-national-museum-of-natural-history-discover-two-squirrel-species-long-obscured-by-mistaken-identities/ (Accessed March 14, 2025).
Tatchell G. M., Parker S. J., Woiwod I. P. (1983). Synoptic monitoring of migrant insect pests in Great Britain and western Europe IV. Host plants and their distribution for pest aphids in Great Britain (Harpenden, Hertfordshire, U.K: Rothamsted Experimental Station), 45–159. Report for 1982. Part 2.
Terraube J., Arroyo B. (2011). Factors influencing diet variation in a generalist predator across its range distribution. Biodivers. Conserv. 20, 2111–2131. doi: 10.1007/s10531-011-0077-1
Thomas J., Lewington R. (1991). The Butterflies of Britain and Ireland. (London: Dorling Kindersley), 224.
Thompson J. N. (1994). The Co-evolutionary Process (Chicago, IL: The University of Chicago Press), 376.
Vasconcelos R., Montero-Mendieta S., Simó-Riudalbas M., Sindaco R., Santos X., Fasola M., et al. (2016). Unexpectedly high levels of cryptic diversity uncovered by a complete DNA barcoding of reptiles of the Socotra Archipelago. PloS One 11, e0149985. doi: 10.1371/journal.pone.0149985
Vester H., Hammerschmidt K. (2013). First record of killer whales (Orcinus orca) feeding on Atlantic salmon (Salmo salar) in northern Norway suggest a multi-prey feeding type. Mar. Biodivers. Rec. 6, e9. doi: 10.1017/S1755267212001030
Videvall E., Gill B. A., Brown M. B., Hoff H. K., Littleford-Colquhoun B. L., Lokeny P., et al (2025). Diet-microbiome covariation across three giraffe species in a close-contact zone. GECCO 58. doi: 10.1016/j.gecco.2025.e03480
Wagner I., Smolina I., Koop M. E. L., Bal T., Lizano A. M., Choo L. Q., et al. (2024). Genome analysis reveals three distinct lineages of the cosmopolitan white shark. Curr. Biol. 34, 3582–3590. doi: 10.1016/j.cub.2024.06.076
War A. R., Buhroo A. A., Hussain B., Ahmad T., Nair R. M., Sharma H. C. (2020). Plant defense and insect adaptation with reference to secondary metabolites, in Co-evolution of Secondary Metabolites. Eds. Mérillon J. M., Ramawat K.Reference Series in Phytochemistry (Springer Nature Switzerland, Switzerland), 795–822. doi: 10.1007/978-3-319-96397-6_60
Ward S. A. (1992). Assessing functional explanations of host specificity. Am. Nat. 139, 883–891. doi: 10.1086/285363
Wesselingh F. P., Neubauer T. A., Anistratenko V. V., Maxim V., Vinarski Yanina. T., Ter Poorten J. J., et al. (2019). Mollusc species from the Pontocaspian region - an expert opinion list. Zookeys 827, 124. doi: 10.3897/zookeys.827.31365
Witton M. P. (2018). Pterosaurs in Mesozoic food webs: a review of fossil evidence, in New Perspectives on Pterosaur Palaeobiology, vol. 445 .Eds. Hone D. W. E., Witton M. P., Martill D. M. (Geological Society of London special publications, London), 7–23. doi: 10.1144/SP455.3
Keywords: cryptic entities, ecological niche, evolution, generalism, genetic identity, habitat, molecular techniques, specialism
Citation: Loxdale HD and Balog A (2025) Preface: the paradox of generalism, a philosophical perspective. Front. Ecol. Evol. 13:1562456. doi: 10.3389/fevo.2025.1562456
Received: 17 January 2025; Accepted: 28 February 2025;
Published: 08 April 2025.
Edited by:
Sasha Raoul Xola Dall, University of Exeter, United KingdomReviewed by:
Sara Gamboa, University of Vigo, SpainCopyright © 2025 Loxdale and Balog. This is an open-access article distributed under the terms of the Creative Commons Attribution License (CC BY). The use, distribution or reproduction in other forums is permitted, provided the original author(s) and the copyright owner(s) are credited and that the original publication in this journal is cited, in accordance with accepted academic practice. No use, distribution or reproduction is permitted which does not comply with these terms.
*Correspondence: Hugh D. Loxdale, TG94ZGFsZUhAY2FyZGlmZi5hYy51aw==