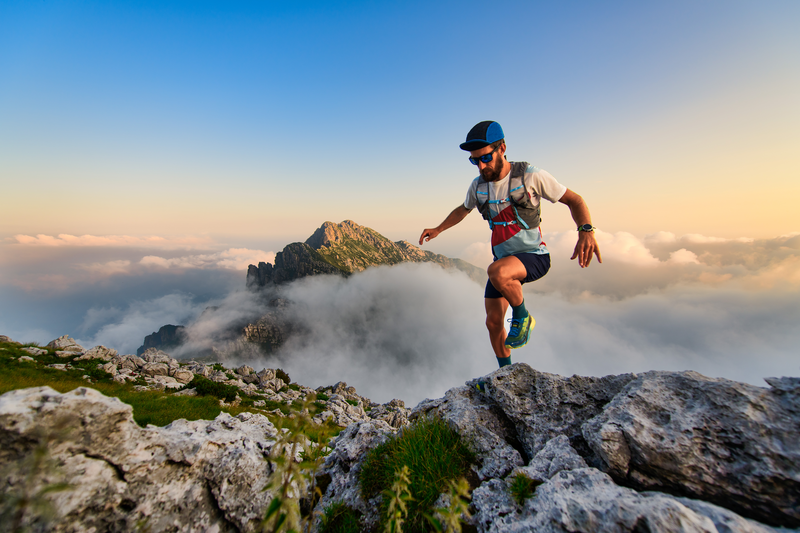
95% of researchers rate our articles as excellent or good
Learn more about the work of our research integrity team to safeguard the quality of each article we publish.
Find out more
ORIGINAL RESEARCH article
Front. Ecol. Evol. , 31 March 2025
Sec. Phylogenetics, Phylogenomics, and Systematics
Volume 13 - 2025 | https://doi.org/10.3389/fevo.2025.1547300
High-density Diopatra populations were recorded in the South Brazilian Bight in the 1970s and 1980s. However, by the 1990s, intertidal populations had declined sharply. The decline and partial recovery raise questions about the factors driving long-term changes in abundance. To better understand these shifts, patches of Diopatra species from Brazilian sandy beaches were followed for 50 years. Data were accessed from papers, gray literature, images, and collections to verify time changes in the South Brazilian Bight (SBB) from 1974 to 2023. We modeled maximum density over time at 15 beaches, observing very high densities (>100 ind.m−2) in 1974 followed by a decrease (~10 ind.m−2) of three species of Diopatra until 1995 and a strong decline (1996–2002) when populations were almost regionally extinct (0–2ind.m−2). A recovery (3–20 ind.m−2) occurred after 2006 for D. victoriae and D. marinae, the latter associated with warmer northern waters, suggesting a range shift. This pattern was associated with heatwaves linked to an El-Niño event (1988) and a gradual SST surface warming of ca. 1°C since 1974. The usage of Diopatra spp. as fishing bait could also be associated with such a reduction. After 2016, D. neapolitana, a likely alien species, was established in the SBB in high densities. Projections based on species distribution modeling (SDM) suggest a potential of invasion in the same range of the known species of D. cuprea complex along the Brazilian coast despite that there are no signs of competition between both species.
Species of the polychaete genus Diopatra are easily noticeable, not only to trained marine biologists but also to any person wandering onto sandy beaches during low tide. Of course, this is not different in the South Atlantic coast, including the extensive 7,500-km coastline of Brazil. Scientific records of Diopatra species in Brazil date back to the 19th century (D. brasiliensis Kinberg, 1865, D. longicornis Kinberg, 1865, D. agave Grube, 1869, and D. variegata Hansen, 1882). By the year 2000, the total number of reported Diopatra species in Brazil increased to 11. However, taxonomic uncertainties have persisted, with some species being only known from their original descriptions.
For decades, D. cuprea (Bosc, 1802) was believed to be the dominant intertidal Diopatra species in Brazil, particularly in the South Brazilian Bight (SBB). However, its classification has been debated, and D. viridis Kinberg, 1865, was proposed to occur in southeastern Brazil and Argentina instead of D. cuprea (Lana, 1991; Orensanz, 1974). Similarly, Paiva (1990) suggested the possible presence of multiple species or subspecies within the region. Molecular and morphological investigations revealed the presence of at least nine Diopatra species in the region (Garcia, 2003; Steiner, 2005), which resulted in the description of four previously unrecognized intertidal species (Seixas et al., 2021 – D. marinae Steiner and Amaral 2021, D. victoriae Steiner and Amaral 2021, D. hannelorae Steiner and Amaral 2021, and D. pectiniconicum Steiner and Amaral 2021). Notably, despite being the historically dominant species, D. cuprea was absent from both morphological and molecular analyses, reinforcing the hypothesis that previous records were actually a cryptic species complex (Sotka et al., 2023; Ziegler et al., 2024).
High-density Diopatra populations were recorded in the SBB in the 1970s and 1980s, with patches reaching 20–130 ind.m2, mainly on fine sand beaches (Amaral, 1979; Corbisier, 1991) and image records from 1974. However, by the 1990s, intertidal populations had declined sharply, leading to the inclusion of D. cuprea on Brazil’s Red List of Threatened Species (Steiner and Amaral, 2008), thus legally restricting collection of this worms. Nevertheless, some intertidal populations in SBB have shown signs of recovery in recent years, with densities ranging from 2 to 20 individuals per square meter. The decline and partial recovery of these populations raise important questions about the factors driving long-term changes in abundance. To better understand these shifts, we examined museum and collection specimens from 1970 to 1990 and compared them with more recent records (both published and unpublished).
One possible factor contributing to these population fluctuations is the harvesting of Diopatra specimens for use as fishing bait. While this practice has been documented in several regions worldwide (Cunha et al., 2005; Arias and Paxton, 2015; Berke, 2022), few publications refer to South America, and as pointed out by Cole et al. (2018), researchers from South American countries report no evidence that polychaetes are abundantly used as bait. Only two references were found in small regions of the southern coast of Brazil, one in Rio Grande do Sul State and another in Santa Catarina State (Lewis and Fontoura, 2005; Alves et al., 2009). Nevertheless, there is no quantitative data on the number of specimens collected or the potential impact on local populations. In addition to harvesting, climate change, particularly seawater warming in recent decades, may also affect these intertidal organisms (Pinsky et al., 2020). This pattern has been well documented for other intertidal species along the southeastern Atlantic coast (Gianelli et al., 2023) and has already been observed in Diopatra species along the French/Spanish Atlantic coasts (Berke et al., 2010).
Out of the native species of Diopatra complex, a newcomer has emerged in recent decades: D. neapolitana Delle Chiaje, 1841, an introduced species originally described from the Mediterranean Sea. This species was first collected in 1992 in intertidal zones of beaches from São Sebastião Island in São Paulo State. The specimens were deposited in Museu de Diversidade Biológica – MDBio – UNICAMP (ZUEC-POL Scientific Collection), but records were not formally published. Diopatra neapolitana only became widespread in SBB after 2016, when large and dense tube banks were reported along the southeastern Brazilian coast (Bergamo et al., 2019). Its identification was confirmed through both morphological and molecular analyses, with genetic comparisons to specimens from its type locality (Bergamo et al., 2019; Seixas et al., 2021). The potential for D. neapolitana to expand its range has been suggested based on its reproductive pattern, distribution, and low molecular divergence (Bergamo et al., 2019; Hektoen et al., 2022). Recent reports further north, such as along Rio de Janeiro coast, suggest it may continue spreading along the Brazilian coast, raising concerns about its impact on native species and ecosystems.
Considering the complex pattern of distribution of the Diopatra cuprea complex and the newcomer, D. neapolitana, herein, we evaluate: (1) temporal density shifts of Diopatra sp. (cuprea group) in SBB sandy beaches over the past 50 years; (2) which species of Diopatra were responsible for such shifts, i.e., is the same species recovering or has this species been replaced over time?; (3) the invasion potential of D. neapolitana through species distribution modeling.
By integrating historical records, field sampling, and ecological modeling, we seek to better understand the factors shaping Diopatra beach populations along the Brazilian coast and the potential consequences of species introductions.
Population densities were obtained from publications (Morgado et al., 1990; Rizzo and Amaral, 2001), grey literature (Gonçalves, 2019; Shimizu, 1991), photographic records (Figure 1), and personal/expert observations. Even though Diopatra spp. from D. cuprea complex were distributed throughout the Brazilian coast from the extreme south (32°S) to the northern Amazonian coast (1°S) (Santos and Aviz, 2019; Seixas et al., 2021), populational information was restricted to the South Brazilian Bight (SBB, Figure 2). SBB is an area of great biogeographical concern because it is a transitional zone where several closely related intertidal polychaete species have distribution overlap, including Diopatra (Silva et al., 2017; Seixas et al., 2021).
Figure 1. Patches of Diopatra spp. in the region of Ubatuba (São Paulo State) over time: (A) high density (1974, Praia Grande, Anchieta Island, Ubatuba); (B) low density (2021, Ubatumirim Beach, Ubatuba); (C) high density (2023, Embaré Beach, Santos).
Figure 2. Brazilian Coast and Southern Brazilian Bight with localities from where density estimations were obtained.
Density data from beaches of SBB, especially in São Paulo State, were estimated or measured whenever past and present density records were available. Seven beaches in this region were sampled more than once over 20–40 years, since they are common in some specific beaches they were visited along time and their no-occurrences were considered in the analysis. Nevertheless, data were grouped by localities (Figure 2; Supplementary Table S1), sometimes with estimates from different beaches since information of maximum density shifts can vary among beaches of the same locality. Thus, beaches could be considered as random samples of Diopatra densities for each locality.
As the size of Diopatra patches depends on the sediment type and beach conditions (tidal range, slope, etc.), population densities were estimated only for the main area of occurrence on the intertidal region. Two measurements were obtained, mean density in the occurrence area and maximum density, both per square meter. Maximum densities were measured on a square meter scale and not extrapolated from lower measured scales, since many patches were smaller than 1 m−2. Since there is a dependence in time-series data, we fitted a local polynomial regression with the best span parameter selected by a Generalized Cross-Validation using the “fANCOVA” package (Wang, 2020).
Historical data of surface sea-water temperature (SST) were obtained from NOAA (NOAA NCDC ERSST version5) (Huang et al., 2017) through R package “rerddap” (Chamberlain, 2021). From these data, we extracted mean annual temperatures, mean of the warmest and coldest months, and thermal anomalies from 1970 to 2021 along the Brazilian coast. The intensities of El Niño events were accessed through the Multivariate and El Niño/Southern Oscillation (ENSO) Index (MEI v.2), obtained from the NOAA Physical Sciences Laboratory (Multivariate ENSO Index Version 2 - MEI.v2, 2024).
D. neapolitana is closely related to D. aciculata Knox and Cameron, 1972 (Zanol et al., 2021), a species found in the Indo-Pacific. Despite their high morphological similarity, Paxton (1993) maintained both species as valid. However, an integrative taxonomic study (Elgetany et al., 2020) suggested that these species exist in a “gray zone” of speciation, where clear molecular and morphological distinctions are difficult to define. Given these uncertainties, we consider both species as a single ecological unit for species distribution modeling in this study. Thus, we combine worldwide distributions of both species in order to obtain their occurrences for modeling. Data from a total of 593 occurrences obtained from GBIF and two more references from our collected material from Rio de Janeiro State were used. Data were filtered, leaving only those with lat/long and thinned to a 5-km distance to avoid oversampling in close areas. Thus, the remaining 290 occurrences were used for modeling. Environmental data were obtained through the “sdmpredictors” package (Bosch and Fernandez, 2022) from Bio-Oracle (Assis et al., 2018), for marine data, and WorldClim (Fick and Hijmans, 2017) for terrestrial data of 5 arcmin.
Modeling was performed using the “biomod2” package (Thuiller et. al., 2021) using 70% of the data for calibration and 30% for evaluation, in four runs. Since we have only presence data, pseudo-absences (=background) data were generated 10 times. The number of pseudo-absences (PAs) was similar to the number of presence data, as suggested by Barbet-Massin et al. (2012) and Čengić et al. (2020). Performance of the combination of models, runs, and PAs was assessed by True Skill Statistics (TSS) with values higher than 0.7 selected for building an ensemble model. The following is the combination of several algorithms: General Linear Model (GLM), General Additive Model (GAM), Boosted Regression Trees (GBM), Random Forest (RF), Multiple Adaptative Regression Splines (MARS), Flexible Discriminant Analysis (FDA), and Maximum Entropy (MaxEnt). Despite the worldwide modeling, forecast of habitat suitability was projected only for the area of interest, the Brazilian coast.
A total of 28 density estimates were obtained from 15 beaches over 50 years (1974–2023) in the SBB Coast (22.7–23.9°S), seven of which were sampled more than once in this period. Since maximum density was strongly correlated with mean density (r=0.88), analysis was performed only for the former. Densities were very high (>100 ind.m−2) in 1974 followed by a decrease (~10 ind.m−2) until 1995 (Supplementary Table S1). After that, there was a sharp decline (1996–2002) when populations were almost regionally extinct (0–2 ind.m−2) and the species (named as D. cuprea) was included in the Red List of Threatened Species of Brazil (Steiner and Amaral, 2008). However, this reduction was followed by a slight recovery (2008–2021) with maximum patches of 3–5 ind.m−2 and a recovery from 2022 to 2023 with densities around 25–30 ind.m−2 in some localities. The local polynomial regression (LOESS) model fitted to the data showed that there were strong long-term population changes (Figure 3).
Figure 3. Density variation of Diopatra cuprea complex species over time (1974–2023) from beaches of the South Brazilian Bight (22–25°S). Species not identified or reported as Diopatra cuprea are plotted as nonidentified (open circles).
Data from older surveys (1974–1990) are mainly based on images or density estimates in the field, referring mainly to D. cuprea or only to the generic level and with rare vouchers. Thus, we are not sure to be working with the same species, considering the taxonomic knowledge update for the region that does not consider D. cuprea as an inhabitant of the Brazilian coast. Nevertheless, we were able to retrieve some vouchers from the same beaches and surveys in museum collections and identified them at a specific level. According to this analysis, the oldest samples (1988) from Lázaro Beach (Ubatuba)—higher densities=10 ind.m−2—were identified as D. hannelorae. In the period of lowest densities (1996–2002), the rare specimens found were D. hannelorae and D. victoriae. Those found on the recent slight recovery (2010–2021) from the beaches of Santos, Guarujá, and Ubatuba were identified as D. marinae and D. victoriae, the latter forming the more recent (2023) higher densities patches in Santos and Armação de Búzios. A collection voucher from Santos in 1958, not included in the plot since there is no quantitative density information, was also an individual of D. hannelorae. Nevertheless, anecdotal information obtained from older observations indicated that high-density patches were common in the region.
Thus, it seems what was considered the sign of populational recovery of a threatened species was due to shift in species dominance, at least in this region. The surveyed region (SE Brazil) is the one where more species of Diopatra were found (Seixas et al., 2021). The distribution range of the species, showing an overlap of at least four species in the SBB, does not mean that they are still sympatric in their distribution since they were sampled at different periods, except for those recently collected in Armação de Búzios (all reported as D. marinae or D. victoriae).
The observed temporal dynamics, characterized by a pronounced decline (~1996–2002) followed by a subsequent recovery (post-2010) in Diopatra, appear to be linked to fluctuations in surface sea-water temperature (SST) within the region, as indicated by temperature anomalies (Figure 4; Supplementary Figures S1, S2). The speciation of D. victoriae, closely related to D. marinae, is suggestive of parapatric processes, considering the paraphyletic state of D. marinae (Seixas et al., 2021) and its distribution range extending to the southern limit of D. marinae (Supplementary Figure S3). Consequently, the expansion of the D. victoriae/D. marinae clade and the disappearance of the colder/southern D. hannelorae in the same geographic area may be attributed to an approximate 1°C mean monthly increase over the past 50 years, implying a thermal threshold exceeding 27°C. Notably, within the SBB region (approximately 12–22°S), where D. hannelorae is infrequently encountered, the mean temperature of warmer months (MTWM) exceeding 28°C was only observed post-2000 (Figure 4; Supplementary Figure S1).
Figure 4. Sea-surface temperature (SST) variation over time (1970–2020) on the Brazilian Coast. South Brazilian Bight is shown below shaded line.
The mean annual temperature increased in the SBB from 22°C to 23°C before 2000 (23°C in 1970) to 23°C to 24°C after 2000 (24°C in 2021) (Figure 4; Supplementary Figure S1). The SBB warming, especially after 2000, gave rise to peaks of temperature anomalies (monthly mean) of ca. 1.8°C in contrast to ca. 1°C before (Supplementary Figure S2) with a significant (p < 0.005) increase in mean anomaly of 0.53°C between 1970–2000 and 2000–2021 intervals. Thus, southward seawater warming in recent years would allow D. victoriae, to expand its population to be the dominant intertidal species in SBB in more recent years.
Despite the restricted area of occurrence of D. neapolitana (=D. aciculata) on the Southeastern Brazilian coast, its higher densities in some beaches (Araça Bay, São Sebastião, São Paulo State), Engenhoca Beach (Governador Island, Rio de Janeiro State), and Japonês Island (Cabo Frio, Rio de Janeiro State) with around 20 ind.m−2 (Figure 5) in the same region where the D. cuprea complex once dominated triggered the alarm for its potential invasion on the Brazilian coast.
Figure 5. Tubes of Diopatra neapolitana in Engenhoca Beach (Governador Island, Rio de Janeiro State, 2017).
Since intertidal animal distribution and tolerance depend on both water and air conditions, we modeled the suitability of D. neapolitana (=D. aciculata) habitat for both marine and terrestrial environmental drivers. After removing collinear and correlated factors, we modeled with just four drivers: sea-surface temperature range, mean salinity, and maximum air-temperature and precipitation of the wettest month. TSS values (threshold of 0.7) and all algorithms (GLM, GAM, GBM, MARS, RF, FDA, and Maxent) were included for at least one run and PA. The final ensemble model with the four environmental variables, salinity, maximum air temperature (MAT), precipitation of the wettest month (PWM), and range of surface sea-water temperature (rSST), were very informative, with high evaluations (TTS = 0.941, Supplementary Figure S4). Importance of each variable was rather different among them with salinity being the most important (median >0.75), all others having lower medians (<0.20) but with some higher values for specific models and runs (Supplementary Figure S5).
Ensemble results projecting the habitat suitability of the species are shown in Figure 6. For the current distribution, which is in the short latitudinal range of 22.8 to 27.7°S, the habitat suitability for the establishment of the species is much longer, ranging from 9 to almost 30°S. This suggests that, in relation to current conditions mainly of salinity and temperature (air and water), the species could increase its distribution, especially northward, wherever sandy beaches and tidal flats occur. The range is the same of intertidal species of the D. cuprea complex, except for the Amazon River mouth (around 0–1°S) where patches of D. cuprea were reported (Santos and Aviz, 2021), but it seems to be not a suitable habitat for D. neapolitana, mainly due to lower salinity (Supplementary Figure S4). Likewise, the medium suitability areas within high suitability areas between 10°S and 21°S, for instance, are located close to two river mouths (Doce and São Francisco rivers). Despite its worldwide distribution, D. neapolitana presented low tolerance to lower salinities (<21 PSU) in lab experiments (Pires et al., 2015). Our modeling based on actual occurrences indicated a higher probability in salinities greater than 34 PSU, which in association with lower levels of precipitation (<250 mm in the wettest month) suggests a strong limitation to areas of brackish waters. Despite being a tube dweller, and thus not likely to be strongly affected by temperature variation (Newell, 1976), mainly air temperature, a short range between 22°C and 33°C was predicted for its distribution.
Figure 6. Ensemble model (TSS weighted mean) of current habitat suitability for D. neapolitana/D. aciculata along the Brazilian coast. Current occurrences are shown as blackspots.
Data on thermal requirements of Diopatra species are difficult to obtain since many of them were based on D. cuprea and other species of the genus that seem to belong to species complexes and not a unique evolutionary unit. Thus, observed patterns are generally not species-specific requirements. Experiments performed with D. cuprea from the type locality, in North Carolina (the “real” D. cuprea), showed a wide range of thermal tolerance surviving in warm waters up to 42.5°C and, for specimens from the Gulf of Mexico, 40.1°C during summer (Kenny, 1969; Berke, 2022). However, for field data of another species, D. biscayensis from Biscay Bay (western European coast) in similar latitudes and thermal conditions, the tolerance limits ranged from 16°C to 24°C (Berke et al., 2010). In SBB, where there seems to be an expansion of the D. marinae/D. victoriae clade, lower monthly mean SST temperatures varied from 19.5°C to 27.8°C (08/2000 and 02/2019, respectively) with a range shift from 19.5°C to 26.9°C in the period of 1970–2000 to 20.1°C to 27.8°C in 2001–2021, suggesting a thermal lower tolerance of both species around 20°C. The mean SST of the coldest month was always greater than 20°C after 2000 in the northern part of the SBB (Figure 4), a clear sign of warming in the last 20 years. Conversely, the warming of coastal waters after 2000 was likely to be responsible for the retraction of D. hannelorae to southern areas, disappearing from the SBB as well as the expansion of the northern clade (D. marinae/D. victoriae).
Shifts in the range of distribution of the Diopatra species were already recorded for the northeastern Atlantic coast (European beaches) with range shifts of D. biscayensis and D. neapolitana related to an increase in SST (surface sea-water temperatures and anomalies) over time (Wethey and Woodin, 2008; Berke et al., 2010). However, this process was related to expansion in the time scale of decades in a gradual process of tropicalization, while, in the SBB, the unusual and acute reduction was before the main gradual global warming, more intense in the last decades (2000–2020).
While increase in SST seems to be the main factor related to shifts in species distribution and sensitivity of coastal species (Gianelli et al., 2023), one cannot disregard the possible role of marine heatwaves, i.e., a wave of extreme and prolonged continuous warming for several days or even months (Holbrook et al., 2020). They are likely to have sudden devastating impact in coastal environments such as coral bleaching and high mortality/local extinction of marine species (Holbrook, et al., 2020). Heatwaves were associated with a retreat in the distribution of the lugworm Arenicola marina, a species with habitats similar to those of Diopatra, forecasting its complete disappearance in Mediterranean in the future (Wethey and Woodin, 2022). Intense and frequent heatwaves were associated with an El Niño—Southern Oscillation (ENSO) event in 1997/1998 (Oliver et al., 2018), one of the three most intense ENSO events since MEI started to be measured (Supplementary Figure S2). This event affected all oceans but, particularly in Southwestern Atlantic, the SBB (Tanaka and Houtan, 2022). It could be responsible for the above cited sudden fall of Diopatra species densities in the SBB followed by the maintenance of the warmer water-associated D. marinae/D. victoriae typical from the eastern and northern coasts of Brazil owing to the gradual SST increase after 2000 (Supplementary Figure S3). Their maintenance after 1998 events (heatwaves) was followed by increased population densities in more recent years. Furthermore, the fact that SST warming is a long-term process that would be causing its spatial expansion, as suggested by molecular analysis in the area (Seixas et al., 2021), made us hypothesize that they are expanding their distribution, a historical process in course that was enhanced more recently.
This SST-driven dominance shift could indicate a process of tropicalization and deborealization (McLean et al., 2021) with a warm-water species expansion and cold-water species retreat of the intertidal species from the D. cuprea complex in SBB. Range shifts of marine species, although less immediately apparent than the impact of introduced species, are likely to affect biological systems at the community level with similar magnitude as those from introduced ones (Sorte et al., 2010). Range shifts of intertidal species distribution seem to be a complex process, with a combination of gradual long-term changes punctuated by shifts caused by extreme events such as heatwaves (Wethey et al., 2011). Besides range shifts, SST increase is also related to strong mass mortality in sandy beach species, such as the yellow clam, Mesoderma mactroides Reeve (1854) (Ortega et al., 2016; Gianelli et al., 2021). Furthermore, other human impacts, like collecting specimens for fishing bait, are likely to affect population density and interact with climate change, especially in areas at the range limit of the species. Nevertheless, quantitative data about the amount of worms harvested and the impact of such activities on population resilience are not yet available, so we cannot assess the actual impact of these activities on the fall and rise of the D. cuprea complex in the region. However, climate change is likely to be a key factor responsible for social-ecological shifts and collapses of beach species along southern Atlantic (Defeo et al., 2021), including strong impacts on fisheries and harvesting of commercial species (Franco et al., 2020).
There is still some uncertainty about the worldwide distribution of D. neapolitana (Elgetany et al., 2020; Berke, 2022) considering a more restricted Mediterranean, Atlantic Iberian, and French coast distribution with all other occurrences (e.g., Southeastern Atlantic, Pacific, and Indian Oceans) as being from related similar species (Arias et al., 2016). However, recent surveys validated some of these doubtful records that show a wider distribution for the species (Bergamo et al., 2019; Paxton and Arias, 2017). Data on genetic distance indicate that the species in the Brazilian Coast is the same evolutionary unit than those from Mediterranean Sea (Seixas et al., 2021) and western European coast (Bergamo et al., 2019). Likewise, reproductive patterns, with a typical sequential protandric hermaphroditism, were also similar in populations from both sides of the Atlantic, despite some subtle morphological differences that could indicate populational variation (Arias et al., 2016; Bergamo et al., 2019)
Thus, it seems that D. neapolitana is an alien species that spreads out owing to a wide range of environmental conditions. Furthermore, the species shows some plasticity regarding its reproductive cycle, with a single annual spawning in temperate regions and a continuous cycle in tropical ones (Escobar-Ortega et al., 2022), with reproductive plasticity being a precondition for the success of marine invasive species providing competitive advantages over native species (Smith, 2009).
Diopatra neapolitana and D. marinae are sympatric in southern Brazil and are seldom syntopic (coexisting in only one or two beaches), probably because D. neapolitana appears to occupy lower-energy, muddier habitats than D. cuprea (Berke, 2022). Thus, negative interactions between them seem not to be an issue, and competition for resources in soft sediments, mainly intertidal tube dwellers, is not common (Wilson, 1990). Nevertheless, projections of habitat suitability for D. neapolitana are rather similar, except for the estuarine and brackish waters in the eastern and northern Brazilian coast. However, considering the struggle for survival in sandy beaches with all human-mediated impacts in such an environment (e.g., nourishment, fishing bait, trampling, and cleaning; see Defeo et al., 2009), we cannot disregard the new risk for the native species coming from a more closely related congeneric species. Despite taxonomic problems regarding correct identification of old records of Diopatra in the South Brazilian Bight, global warming and heatwaves are likely to be involved in the fall and rise of species of the Diopatra cuprea complex over the last 50 years through retraction, extinction, and expansion of ranges of different species of the group. Also, an exotic species, D. neapolitana, just arrived and seems to have a potential for dispersing to the same beaches that native species inhabit. Human impact is also likely to act on populational changes, even though its relative role is still to be evaluated.
The data presented in this study were partially sourced from the following literature: Shimizu (1991), Morgado et al. (1990), Rizzo and Amaral (2001), Garcia (2003), and Gonçalves (2019). Collected specimens are available at the Museu de Diversidade Biológica (MDBio) – UNICAMP (ZUEC-POL Scientific Collection) and can be accessed via the following link: https://specieslink.net/search/records/col/166, accession numbers ZUEC POL 2510, 2643, 2693, 3170, 3172, 3173, 3177, 3178, 3331, 3335, 3337, 3339, 4622, 4868, 8283, 10738, 10739, and 19778.
The manuscript presents research on animals that do not require ethical approval for their study.
PP: Conceptualization, Formal Analysis, Funding acquisition, Investigation, Methodology, Project administration, Resources, Software, Validation, Visualization, Writing – original draft, Writing – review & editing. AA: Conceptualization, Data curation, Formal Analysis, Funding acquisition, Investigation, Project administration, Resources, Supervision, Validation, Writing – review & editing. VS: Conceptualization, Formal Analysis, Investigation, Methodology, Validation, Writing – review & editing. MP: Conceptualization, Data curation, Investigation, Resources, Validation, Writing – original draft, Writing – review & editing. TS: Conceptualization, Data curation, Formal Analysis, Investigation, Validation, Writing – review & editing.
The author(s) declare that financial support was received for the research and/or publication of this article. This research was funded by FAPESP (Fundação de Amparo à Pesquisa do Estado de São Paulo, grant number 2018/10313-0), FAPERJ (Fundação Carlos Chagas Filho de Amparo à Pesquisa do Estado do Rio de Janeiro, grant number E-26/200.375/2023), and CNPq (Conselho Nacional de Desenvolvimento Científico e Tecnológico, grants number 306788/2021-7, 428447/2018-0 and 301551/2019-7).
Specimens of Diopatra examined were loaned by Marcelo V. Fukuda (MZUSP), Joana Zanol (MN-RJ), Tatiana M. Steiner (MDBio), and Monica A. V. Petti (ColBIO). We also thank Dr. Natalia Venturini and Dr. Omar Defeo (Universidad de la República, Montevideo, Uruguay) for their valuable suggestions.
The authors declare that the research was conducted in the absence of any commercial or financial relationships that could be construed as a potential conflict of interest.
The author(s) declared that they were an editorial board member of Frontiers, at the time of submission. This had no impact on the peer review process and the final decision.
The author(s) declare that Generative AI was used in the creation of this manuscript. For correcting and improving the abstract and some paragraphs of the Introduction, after Generative AI was used, the authors reviewed it again.
All claims expressed in this article are solely those of the authors and do not necessarily represent those of their affiliated organizations, or those of the publisher, the editors and the reviewers. Any product that may be evaluated in this article, or claim that may be made by its manufacturer, is not guaranteed or endorsed by the publisher.
The Supplementary Material for this article can be found online at: https://www.frontiersin.org/articles/10.3389/fevo.2025.1547300/full#supplementary-material
Alves G., Dalben A., Hanazaki N. (2009). “Gênero Diopatra: dados etnobiológicos da baía da Ilha de Santa Catarina,” in Ecologia de Campo na Lagoa do Peri. Eds. Cantor M., de Macedo-Soares L. C. P., Hanazaki N. (UFSC, Florianópolis, Brazil), 181–192.
Amaral A. C. Z. (1979). Ecologia e contribuição dos anelídeos poliquetos para a biomassa bêntica da zona das marés, no litoral norte do estado de São Paulo. Bolm Inst. Oceanogr S Paulo 28, 1–52. doi: 10.1590/S0373-55241979000100001
Arias A., Paxton H. (2015). The cryptogenic bait worm Diopatra biscayensis Fauchald et al., 2012 (Annelida: Onuphidae) - Revisiting its history, biology and ecology. Estuar. Coast. Shelf Sci. 163, 22–36. doi: 10.1016/j.ecss.2015.05.033
Arias A., Paxton H., Budaeva N. (2016). Redescription and biology of Diopatra neapolitana(Annelida: Onuphidae), a protandric hermaphrodite with external spermaducal papillae. Estuar. Coast. Shelf Sci. 174, 1–17. doi: 10.1111/geb.12693
Assis J., Tyberghein L., Bosch S., Verbruggen H., Serrão E. A., De Clerck O. (2018). Bio-ORACLE v2.0: Extending marine 373 data layers for bioclimatic modelling. Glob. Ecol. Biogeogr. 27 (3), 277–284. doi: 10.1111/geb.12693
Barbet-Massin M., Jiguet F., Albert C. H., Thuiller W. (2012). Selecting pseudo-absences for species distribution models: how, where and how many? Methods Ecol. Evol. 3, 327–338. doi: 10.1111/j.2041-210X.2011.00172.x
Bergamo G., Carrerette O., Nogueira J. M. M. (2019). Continuous and non-seasonal reproductive cycle of the alien species Diopatra neapolitana (Onuphidae, Annelida) in a tropical bay of SW Atlantic. Estuar. Coast. Shelf Sci. 231, 106479. doi: 10.1016/j.ecss.2019.106479
Berke S. K. (2022). A review of Diopatra ecology: current knowledge, open questions, and future threats for an ecosystem engineering polychaete. Biology 11, 1485. doi: 10.3390/biology11101485
Berke S. K., Mahon A. R., Lima F. P., Halanych K. M., Wethey D. S., Woodin S. A. (2010). Range shifts and species diversity in marine ecosystem engineers: Patterns and predictions for European sedimentary habitats. Glob. Ecol. Biogeogr 19, 223–232. doi: 10.1111/j.1466-8238.2009.00509.x
Bosch S., Fernandez S. (2022). sdmpredictors: Species Distribution Modelling Predictor Datasets. R package version 0.2.11. Available online at: https://CRAN.R-project.org/package=sdmpredictors (Accessed December 21 2022).
Čengić M., Rost J., Remenska D., Janse J. H., Huijbregts M. A. J., Schipper A. M. (2020). On the importance of predictor choice, modeling technique, and number of pseudo-absences for bioclimatic envelope model performance. Ecol. Evol. 10, 12307–12317. doi: 10.1002/ece3.6859
Chamberlain S. (2021). rerddap: General Purpose Client for ‘ERDDAP’ Servers. R package version 0.7.6. Available online at: https://CRAN.Rproject.org/package=rerddap (Accessed December 2, 2024).
Cole V. J., Chick R. C., Hutchings P. A. (2018). A review of global fisheries for polychaete worms as a resource for recreational fishers: diversity, sustainability and research needs. Rev. Fish Biol. Fisheries 28, 543–565. doi: 10.1007/s11160-018-9523-4
Corbisier T. N. (1991). Benthic macrofauna of sandy intertidal zone at Santos estuarine system, São Paulo, Brazil. Bolm Inst. oceanogr S Paulo 39, 1–13. doi: 10.1590/S0373-55241991000100001
Cunha T., Hall A., Queiroga H. (2005). Estimation of the Diopatra neapolitana annual harvest resulting from digging activity in Canal de Mira, Ria de Aveiro. Fish Res. 76, 56–66. doi: 10.1016/j.fishres
Defeo O., McLachlan A., Schoeman D. S., Schlacher T. A., Dugan J., Jones A., et al. (2009). Threats to sandy beach ecosystems: a review. Estuar. Coast. Shelf Sci. 81, 1–12. doi: 10.1016/j.ecss.2008.09.022
Defeo O., McvLachlan A., Armitage D., Elliot M., Pittman J. (2021). Sandy beach social-ecological systems at risk: regime shifts, collapses, and governance challenges. Front. Ecol. Environ. 19, 564–573. doi: 10.1002/fee.2406
Elgetany A. H., Van Rensburg H., Hektoen M., Matthee C., Budaeva N., Simon C. A., et al. (2020). Species delineation in the speciation grey zone - The case of Diopatra (Annelida, Onuphidae). Zool. Scr 49, 516–534. doi: 10.1111/zsc.12421
Escobar-Ortega D., Couceiro L., Muíño R., No E., Fernández N. (2022). Insights into environmental drivers on the reproductive cycle of Diopatra napolitana (Polychaeta: Onuphidae). Biology. 11, 1504. doi: 10.3390/biology11101504
Fick S. E., Hijmans R. J. (2017). WorldClim 2: new 1-km spatial resolution climate surfaces for global land areas. Int. J. Climatol. 37, 4302–4315. doi: 10.1002/joc.5086
Franco B. C., Defeo O., Piola A. R., Barreiro M., Yang H., Ortega L., et al. (2020). Climate change impacts on the atmospheric circulation, ocean, and fisheries in the southwest South Atlantic Ocean: a review. Clim. Change 162, 2359–2377. doi: 10.1007/s10584-020-02783-6
Garcia P. M. (2003). Sistemática morfológica e molecular do complexo específico Diopatra cuprea (Bosc 1802) do litoral brasileiro. MSc. Thesis (Rio de Janeiro (Brazil: Universidade Federal do Rio de Janeiro, Rio de Janeiro).
Gianelli I., Orlando L., Cardoso L. G., Carranza A., Celentano E., Correa P., et al. (2023). Sensitivity of fishery resources to climate change in the warm-temperate Southwest Atlantic Ocean. Reg. Environ. Change 23, 49. doi: 10.1007/s10113-023-02049-8
Gianelli I., Ortega L., Pittman J., Vasconcellos M., Defeo O. (2021). Harnessing scientific and local knowledge to face climate change in small-scale fisheries. Glob. Env Change 68, 102253. doi: 10.1016/j.gloenvcha.2021.102253
Gonçalves K. R. (2019). Análise da efetividade do uso de indicadores de qualidade ambiental para gestão de praias arenosas. Estudo de caso na Baixada Santista, São Paulo, Brasil. MSc Thesis (Santos (Brazil: Universidade Federal de São Paulo, São Paulo).
Hektoen M. M., Willassen E., Budaeva N. (2022). Phylogeny and cryptic diversity of Diopatra (Onuphidae, Annelida) in the East Atlantic. Biology 11, 327. doi: 10.3390/biology11020327
Holbrook N. J., Sen Gupta A., Oliver E. C. J., Hobday A. J., Benthuysen J. A., Scannell H. A., et al. (2020). Keeping pace with marine heatwaves. Nat. Rev. Earth Environ. 1, 482–493. doi: 10.1038/s43017-020-0068-4
Huang B., Thorne P. W., Banzon V. F., Boyer T., Chepurin G., Lawrimore J. H., et al. (2017). Extended reconstructed sea surface temperature, version 5 (ERSSTv5): upgrades, validations, and intercomparisons. J. Clim. 30, 8179–8205. doi: 10.1175/JCLI-D-16-0836.1
Kenny R. (1969). Temperature tolerance of the polychaete worms Diopatra cuprea and Clymenella torquata. Mar. Biol. 4, 219–223. doi: 10.1007/BF00393896
Lana P. C. (1991). Onuphidae (Annelida: polychaeta) from southeastern Brazil, (1991). Bull. Mar. Sci. 48, 280–295.
Lewis D., dos S., Fontoura N. F. (2005). Maturity and growth of Paralonchurus brasiliensis females in southern Brazil (Teleostei, Perciformes, Sciaenidae). J. Appl. Ichthyol 21, 94–100. doi: 10.1111/j.1439-0426.2004.00637.x
McLean M., Mouillot D., Maureaud A. A., Hattab T., MacNeil M. A., Goberville E., et al. (2021). Disentangling tropicalization and deborealization in marine ecosystems under climate change. Curr. Biol. 31, 4817–4823. doi: 10.1016/j.cub.2021.08.034
Morgado E. H., Amaral A. C. Z., Belucio L. F., Lopes P. P., Ferreira C. P., Leite F. P. P. (1990). ““The intertidal macrofauna of São Francisco complex beaches (São Sebastião, SP)”,” in Anais II Simpósio de Ecossistemas da Costa Sul e Sudeste Brasileira-Estrutura, Função e Manejo. Águas de Lindóia, Brazil, 4-11 April 1990, vol. 3. (Publ. ACIESP, São Paulo, Brazil), 314–325.
Multivariate ENSO Index Version 2 - MEI.v2 (2024). Available online at: https://psl.noaa.gov/enso/mei/ (Accessed November 5, 2024).
Museu de Diversidade Biológica – MDBio – UNICAMP Specimen, ZUEC-POL 4622, polychaete collection, campinas (São Paulo, Brasil). Available online at: https://specieslink.net/search/records/col/166 (Accessed February 19, 2025).
Newell R. C. (1976). Adaptation to environment: essays on the physiology of marine animals (London: Butterworth & Co).
Oliver E. C. J., Donat M. G., Burrows M. T., Moore P. J., Smale D. A., Alexander L. V., et al. (2018). Longer and more frequent marine heatwaves over the past century. Nat. Commun. 9, 1324. doi: 10.1038/s41467-018-03732-9
Orensanz J. M. (1974). Los anelidos poliquetos de la Provincia Biogeografica Argentina. V. Onuphidae. Physis Sec A. 33, 75–122.
Ortega L., Celentano E., Delgado E., Defeo O. (2016). Climate change influences on abundance, individual size and body abnormalities in a sandy beach clam. Mar. Ecol. Prog. Ser. 545, 203–213. doi: 10.3354/meps11643
Paiva P. C. (1990). Padrões de distribuição e estrutura trófica dos anelídeos poliquetas da plataforma continental do litoral norte do Estado de São Paulo. MSc Thesis, Oceanography (São Paulo (Brazil: Universidade de São Paulo).
Paxton H. (1993). Diopatra Audouin and Milne Edwards (Polychaeta: Onuphidae) from Australia, with a discussion of developmental patterns in the genus. Beagle Rec N. Territ Mus Arts Sci. 10, 115–154. doi: 10.5962/p.271283
Paxton H., Arias A. (2017). Unveiling a surprising diversity of the genus Diopatra Audouin & Milne Edward (Annelida: Onuphidae) in the Macaronesian region (eastern North Atlantic) with the description of four new species. Zootaxa. 4300, 505–535. doi: 10.11646/zootaxa.4300.4.3
Pinsky M. L., Selden R. L., Kitchel Z. J. (2020). Climate-driven shifts in marine species ranges: scaling from organisms to communities. Ann. Ver Ecol. Syst. 12, 153–179. doi: 10.1146/annurev-marine-010419-010916
Pires A., Figueira E., Moreira A., Soares A. M. V. M., Freitas R. (2015). The effects of water acidification, temperature and salinity on the regenerative capacity of the polychaete Diopatra neapolitana. Mar. Environ. Res. 106, 30–41. doi: 10.1016/j.marenvres.2015.03.002
Rizzo A. E., Amaral A. C. Z. (2001). Spatial distribution of annelids in the intertidal zone in Sao Sebastiao Channel, Brazil. Sci. Mar. 65, 323–331. doi: 10.3989/scimar.2001.65n4323
Santos T. M. T., Aviz D. (2019). Macrobenthic fauna associated with Diopatra cuprea (Onuphidae: Polychaeta) tubes on a macrotidal sandy beach of the Brazilian Amazon Coast. J. Mar. Biol. Assoc. U.K. 99, 751–759. doi: 10.1017/S0025315418000711
Seixas V. C., Steiner T. M., Solé-Cava A. M., Amaral A. C. Z., Paiva P. C. (2021). Hidden diversity within the Diopatra cuprea complex (Annelida: Onuphidae): Morphological and genetics analyses reveal four new species in the south-west Atlantic. Zool. J. Linn. Soc 191, 637–671. doi: 10.1093/zoolinnean/zlaa032
Shimizu R. M. (1991). A Comunidade de Macroinvertebrados da Região Entremarés da Praia de Barequeçaba, São Sebastião, SP. MSc Thesis (São Paulo, Brazil: Universidade de São Paulo).
Silva C. F., Seixas V. C., Barroso R., Di Domenico M., Amaral A. C. Z., Paiva P. C. (2017). Demystifying the Capitella capitata complex (Annelida, Capitellidae) diversity by morphological and molecular data along the Brazilian coast. PloS One 12, e0177760. doi: 10.1371/journal.pone.0177760
Smith L. D. (2009). “The role of phenotypic plasticity in marine biological invasion,” in Biological invasions in marine ecosystems - ecological studies. Eds. Rilov G., Crooks J. A. (Springer, Berlin/Heidelberg, Germany). doi: 10.1007/978-3-540-79236-9_10
Sorte C. J. B., Williams S. L., Carlton J. T. (2010). Marine range shifts and species introductions: comparative spread rates and community impacts. Global Ecol. Biogeogr. 19, 303–316. doi: 10.1111/j.1466-8238.2009.00519.x
Sotka E. E., Bell T., Berke S. (2023). Cryptic mtDNA Diversity of Diopatra cuprea (Onuphidae, Annelida) in the Northwestern Atlantic Ocean. Biology. 12, 521. doi: 10.3390/biology12040521
Steiner T. M. (2005). Estudo taxonômico da família Onuphidae (Annelida, Polychaeta) das regiões Sudeste e Sul do Brasil (PhD Thesis. Campinas (Brazil: Universidade de Campinas).
Steiner T. M., Amaral A. C. Z. (2008). “Diopatra cuprea bos,” in Livro Vermelho da Fauna Brasileira Ameaçada de Extinção, vol. 1 . Eds. MaChado A. B. M., Drummond G. M., Paglia A. P. (Fundação Biodiversitas/Ministério do Meio Ambiente, Brasília, Brazil), 283–285.
Tanaka K. R., Van Houtan K. S. (2022). The recent normalization of historical marine heat extremes. PloS Clim. 1, e0000007. doi: 10.1371/journal.pclm.0000007
Thuiller W., Georges D., Gueguen M., Engler R., Breiner F. (2021). Biomod2: Ensemble platform for species distribution modeling. R package version 3.5.1. Available online at: https://cran.r-projetc.org/package=biomod2 (Accessed December 21, 2022).
Wang X. (2020). fANCOVA: Nonparametric analysis of covariance. R package version 0.6-1. Available online at: https://CRAN.R-project.org/package=fANCOVA (Accessed March 18, 2023).
Wethey D. S., Woodin S. A. (2008). “Ecological hindcasting of biogeographic responses to climate change in the European intertidal zone,” in Challenges to marine ecosystems - developments in hydrobiology, vol. 202 . Eds. Davenport J., Burnell G., Croos T., Emmerson M., McAllen R., Ramsay R., Rogan E. (Springer, Dordrecht, Germany), 139–151. doi: 10.1007/978-1-4020-8808-7_13
Wethey D. S., Woodin S. A. (2022). Climate change and Arenicola marina: Heat waves and the southern limit of an ecosystem engineer. Estuar. Coast. Shelf Sci. 276, 10801. doi: 10.1016/j.ecss.2022.108015
Wethey D. S., Woodin S. A., Hilbish T. J., Jones S. J., Lima F. P., Brannock P. M. (2011). Response of intertidal populations to climate: Effects of extreme events versus long term change. J. Exp. Mar. Biol. Ecol. 400, 132–144. doi: 10.1016/j.jembe.2011.02.008
Wilson W. H. (1990). Competition and predation in marine soft-sediment communities. Annu. Rev. Ecol. Evol. Syst. 21, 221–241. doi: 10.1146/annurev.es.21.110190.001253
Zanol J., Carrera-Parra L. F., Steiner T. M., Amaral A. C. Z., Wiklund H., Ravara A., et al. (2021). The current state of Eunicida (Annelida) systematics and biodiversity. Diversity. 13, 74. doi: 10.3390/d13020074
Ziegler A. J., Bell T. M., Berke S. K., et al. (2024). Multiple cryptic lineages and restricted gene flow in the decorator worm Diopatra cuprea. Research Square. Available online at: https://doi.org/10.21203/rs.3.rs-4536533/v1 (Accessed July 3, 2024).
Keywords: South Brazilian Bight, biogeography, heatwaves, global warming, range-shifts, alien species
Citation: Paiva PC, Amaral ACZ, Seixas VC, Petti MAV and Steiner TM (2025) The fall and rise of Diopatra in Southern Brazilian sandy beaches. Front. Ecol. Evol. 13:1547300. doi: 10.3389/fevo.2025.1547300
Received: 18 December 2024; Accepted: 04 March 2025;
Published: 31 March 2025.
Edited by:
Andrés Arias, University of Oviedo, SpainReviewed by:
Adília Pires, University of Aveiro, PortugalCopyright © 2025 Paiva, Amaral, Seixas, Petti and Steiner. This is an open-access article distributed under the terms of the Creative Commons Attribution License (CC BY). The use, distribution or reproduction in other forums is permitted, provided the original author(s) and the copyright owner(s) are credited and that the original publication in this journal is cited, in accordance with accepted academic practice. No use, distribution or reproduction is permitted which does not comply with these terms.
*Correspondence: Paulo Cesar Paiva, cGF1bG8ucGFpdmFAZ21haWwuY29t
Disclaimer: All claims expressed in this article are solely those of the authors and do not necessarily represent those of their affiliated organizations, or those of the publisher, the editors and the reviewers. Any product that may be evaluated in this article or claim that may be made by its manufacturer is not guaranteed or endorsed by the publisher.
Research integrity at Frontiers
Learn more about the work of our research integrity team to safeguard the quality of each article we publish.