- 1Marine Biotechnology Research Center, Korea Institute of Ocean Science & Technology (KIOST), Busan, Republic of Korea
- 2Department of Biological Sciences and Biotechnology, Hannam University, Daejeon, Republic of Korea
- 3KIOST School, University of Science and Technology, Daejeon, Republic of Korea
Introduction: Psychrophilic marine bacteria belonging to the family Colwelliaceae have garnered increasing industrial and ecological interest. However, their phylogenetic positions remain ambiguous when classified solely based on 16S rRNA gene sequences, limiting accurate taxonomic resolution.
Methods: To resolve these ambiguities, we characterized four newly isolated species using a comprehensive taxogenomic framework. We analyzed genome-based indices, including average nucleotide identity (ANI), digital DNA–DNA hybridization (dDDH), and average amino acid identity (AAI), across all publicly available Colwelliaceae genomes. Genus-level AAI thresholds were established through repetitive clustering and evaluation strategies.
Results: Our analysis revealed that genus-level AAI values within Colwelliaceae ranged from 74.07% to 75.11%. Based on these thresholds, we re-evaluated 47 species, including the four novel isolates, and proposed the establishment of 18 new genera, expanding the current taxonomy from 6 to 24 genera. The four novel species were assigned to three of these newly proposed genera.
Discussion: This study presents a revised classification of the family Colwelliaceae grounded in genome-based taxonomic metrics. Our findings highlight the limitations of traditional 16S rRNA-based phylogenies and support the use of taxogenomic approaches to achieve higher taxonomic resolution in marine microbial lineages.
1 Introduction
The family Colwelliaceae (order Alteromonadales, class Gammaproteobacteria, phylum Pseudomonadota) (Ivanova et al., 2004; Ivanova and Mikhailov, 2001) is primarily found in marine environments, such as marine sediments (Nogi et al., 2004; Xu et al., 2016), sea ice (Bowman et al., 1998; Zhang et al., 2008), seawater (Kristyanto et al., 2022; Wang et al., 2018a), and marine animals (Kim et al., 2017; Zhang et al., 2014). Genome sequencing confirmed that strains of the genus Colwellia (Deming et al., 1988) are specifically adapted to low temperatures (5°C) (Methé et al., 2005). The family Colwelliaceae is a typical marine secondary producer and plays a role in the decomposition of organic substances (hydrocarbons, lipids, proteins, and polysaccharides). They also ferment simple compounds (Bowman, 2014). In addition, ligA genes from some Colwellia species can be used to develop temperature-sensitive versions of the pathogen, which can induce an immune response while mitigating the potential for disease caused by the vaccine (such as Polio, viral disease; flu) (Duplantis et al., 2010). Like the related group Pseudoalteromonas (Gauthier et al., 1995), Colwellia can synthesize bioactive compounds (Bowman, 2007). Eicosapentaenoic acid production by members of the family Colwelliaceae has been demonstrated (Tatsumi et al., 2019), suggesting their potential application in biotechnology (Hashimoto et al., 2015). Accordingly, Colwelliaceae may be useful in various industrial and medical contexts.
The family Colwelliaceae currently consists of six genera: Colwellia (Deming et al., 1988), Thalassomonas (Tsm) (Macián et al., 2001), Litorilituus (Wang et al., 2013), Thalassotalea (Tst) (Zhang et al., 2014), Cognaticolwellia (Liu et al., 2020a), and Pseudocolwellia (Liu et al., 2020b). Initially, Colwellia psychrerythraea was assigned to the family Vibrionaceae (Deming et al., 1988), but it was later reclassified into a new genus based on 5S rRNA gene analysis (D’Aoust and Kushner, 1972). Based on DNA G + C content, the genus Thalassomonas formed a distinct clade from Colwellia (Macián et al., 2001), which led to the proposal of the family Colwelliaceae (Ivanova et al., 2004). The genus Litorilituus was introduced based on a strain isolated from an amphibian breeding area (Wang et al., 2013), while the genus Thalassotalea was established from the reclassification of several species in the genus Thalassomonas based on phylogenetic differences and DNA G + C content (Zhang et al., 2014). The taxonomic history has continued up to the present, with recent extensive reclassification based on genomic data. In this reclassification (Liu et al., 2020b), emphasized the limitations of using 16S rRNA gene for classifying this group.
The family Colwelliaceae is an important taxonomic group both industrially and academically because it produces a variety of substances, but the phylogenetic position of microorganisms belonging to the family Colwelliaceae based on the 16S rRNA gene is still unclear. While a bacterial species can be defined based on 70% genome identity, the genus definition is still ambiguous and this makes it difficult to identify position of species. Despite the 16S rRNA gene sequences have been used as a supplementary indicator, the criteria for defining genera vary across taxonomic groups (Caudill and Brayton, 2022). In particular, unlike Colwellia, which was recently reclassified based on genomic data, Thalassomonas and Thalassotalea have not yet undergone genome-based reclassification (Li et al., 2024; Rey-Velasco et al., 2024). A key limitation in the genome-wide analysis of certain genera is insufficient information on metabolic activity, hindering comprehensive genome-based analysis. Thus, both genomic and metabolic analyses are necessary to establish accurate phylogenetic relationships.
Advances in technology have made genome sequencing and in silico analysis commonplace, enabling the analysis of genomic differences and the original definition of species as well as applying it to taxa where taxonomic relationships are unclear. Recently, taxogenomics—focusing on whole-genome analyses—has gained attention as a method for addressing taxonomic ambiguities in various groups. Taxogenomics also reveals the unique characteristics of each group influenced by environmental and evolutionary factors. Therefore, the aim of this study was to perform a phylogenetic classification of all members of the family Colwelliaceae based on genomes to correct taxonomic errors, as well as to perform genome-based metabolic activity analysis.
2 Materials and methods
2.1 Isolation and cultivation
Four strains were isolated from different locations and dates. Strain MEBiC14330T was isolated from sediment on April 5, 2020, from the Gordin Guyot region in the North-West Pacific Ocean (16° 50’ 50” N, 150° 04’ 36” E) at a depth of 1,300 m. Strain MEBiC06441T was isolated on February 25, 2009, from sediment in Incheon (37° 16’ 12” N, 126° 26’ 34” E). Strain MEBiC06471T was isolated on February 10, 2009, from sediment in the Taean-gun mud flat (36° 46’ 30” N, 126° 08’ 01” E). Strain MEBiC02087T (=BS250T) was isolated from sediment collected at 680 m deep in the Barents Sea (73° 03’ N, 50° 03’ E) in Russia. The first three strains were cultured at 25°C on marine agar 2216 medium (MA; BD Biosciences, Franklin Lakes, NJ, USA) on April 5, 2020; March 2, 2009; and February 15, 2009, respectively. After one week, the strains were purified by subculturing on new MA plates. To isolate the fourth strain, 1 cm2 of sediment was diluted with sterile seawater and spread on MA (BD Biosciences). Inoculated plates were cultivated at 10°C for 1 week. Individual colonies were isolated from MA, and the morphologically distinct strain MEBiC02087T was selected. After primary isolation and purification, the strain was cultivated at 15°C on MA. All strains were routinely cultured on MA and stored at –80°C in marine broth (BD Biosciences) supplemented with 20% (v/v) glycerol.
2.2 Small subunit rRNA gene and whole-genome sequencing
For small subunit 16S rRNA gene sequencing by Sanger Sequencing, the genomic DNA of strains MEBiC14330T, MEBiC06441T, MEBiC06471T, and MEBiC02087T were extracted using a LaboPass bacterial genomic DNA isolation kit (Cosmo Gentech, Seoul, Korea) according to the manufacturer’s instructions. PCR amplification of the 16S rRNA gene was performed using TaKaRa Ex Taq mixture (Takara Bio, Shiga, Japan) and a prokaryote-universal primer set: 27F (5′–AGAGTTTGATCMTGGCTCAG–3′) and 1492R (5′–TACGGYTACCTTGTTACGACTT–3′). The PCR conditions were as follows: initial denaturation at 98°C for 30 seconds, followed by 30 cycles of denaturation at 98°C for 30 seconds, annealing at 55°C for 30 seconds, and extension at 72°C for 1 minute. A final extension was performed at 72°C for 10 minutes. The PCR product was purified using a QIAquick PCR Purification Kit (QIAGEN, Hilden, Germany) and sequenced by Macrogen Co., Ltd. (Seoul, Korea). The PCR-generated 1492R and 27F sequences were processed using EditSeq version 7.0.0 (DNAstar, Madison, WI, USA). Each sequence was saved in SEQ file format and imported into SeqMan version 7.0.0 (DNAstar) (Hall, 1999) for assembly. In SeqMan, overlapping regions were automatically identified and merged into a single contig. The resulting contig was saved in text file format and subjected to BLAST analysis for further comparison and identification. Closely related strains were identified through BLAST, and their 16S rRNA gene sequences were obtained from the GenBank database and EzBioCloud server (http://www.ezbiocloud.net). (Yoon et al., 2017a). Multiple alignments were performed using Clustal W embedded in MEGA 11 (Tamura et al., 2021) and BioEdit (Hall, 1999). The distances were calculated using the Kimura 2-parameter model (Kimura, 1980) to construct a phylogenetic tree with neighbor-joining (Saitou and Nei, 1987), maximum-likelihood (Felsenstein, 1981), and minimum-evolution (Rzhetsky and Nei, 1992) methods in MEGA 11. The outgroups downloaded from the NCBI were Idiomarina baltica OS145T (AJ440214) and Psychromonas antarctica star-1T (Y14697). The 16S rRNA gene sequences were deposited in the NCBI GenBank database under the accession numbers MEBiC14330T (OR958774), MEBiC06441T (OR958780), MEBiC06471T (OR958775), and MEBiC02087T (PQ119528).
For whole-genome sequencing, genomic DNA was extracted using the LaboPass Bacterial Genomic DNA Isolation Kit (Cosmo Gentech). The extracted DNA concentrations were confirmed using a NanoDrop and Qubit 4 (Thermo Fisher Scientific, Waltham, MA, USA) (García-Alegría et al., 2020). For whole-genome sequencing, an Illumina iSeq 100 was used (Khan et al., 2018). The quality of the sequencing data was confirmed by visualization using FastQC version 0.12.1 (FastQC Quality Control, San Diego, CA, USA) (Leggett et al., 2013). Poly-G trimming is essential to eliminate errors caused by sequencing artifacts, which commonly occur in Illumina sequencing platforms. To address this issue, Poly-G trimming was performed using Cutadapt version 4.4 (Martin, 2011). Specifically, consecutive G bases (≥10) at the start of the forward and reverse reads were removed to ensure the accuracy of the sequencing data and reduce the impact of sequencing biases. Trimming was performed using Trimmomatic version 0.39 (Bolger et al., 2014) with a quality cutoff of Q20 for both forward and reverse reads. Illumina universal adapter sequences (AGATCGGAAGAGC) were removed using the ILLUMINACLIP option, and sequences shorter than 50 bp after trimming were discarded using the MINLEN parameter. Additional parameters included LEADING:3, TRAILING:3, and SLIDINGWINDOW:4:20 to trim low-quality bases at the ends and sliding window-based quality trimming. The forward and reverse reads were processed separately and saved as paired output files. Subsequently, assembly was performed using SPAdes version 3.15.5 (Bankevich et al., 2012), with k-mer sizes of 21, 33, 55, 77, and 99, the –careful mode enabled, and the –cov-cutoff set to ‘auto’ to optimize assembly accuracy and perform error correction. The quality of the genome assembly was evaluated using the Quality Assessment Tool version 5.2.0 (Mikheenko et al., 2018) and was visually confirmed. The assessment was based on metrics such as N50, total length, and the number of contigs, with assemblies being selected based on a lower number of contigs, a higher N50 value, and a larger total genome size to ensure accuracy. Additionally, genome completeness and contamination levels were evaluated using CheckM version 1.2.3 (Parks et al., 2015) to further confirm the quality of the assembly. Annotation was performed using Prokka version 1.14.6 (Seemann, 2014), which predicts putative coding sequences and provides basic annotations of the predicted protein-coding regions. The genomes from type materials (n = 47) belonging to the family Colwelliaceae available in the NCBI database were collected for subsequent analysis. Their basic genomic information is described in Supplementary Table S1.
2.3 Genome-based analysis
The core gene phylogeny was performed using PhyloPhlAn version 3.1.68 (Asnicar et al., 2020). FAA result files were obtained from Prokka. The phylogenetic tree was constructed using RAxML version 8.2.12 (Stamatakis, 2014) with 400 core universal protein markers (Segata et al., 2013). The PROTCATLG model was applied, utilizing the LG amino acid substitution matrix with a CAT approximation to model rate heterogeneity across sites. Phylogenetic inference was conducted with 1,000 bootstrap replicates using rapid bootstrapping and a thorough maximum likelihood (ML) search (-f a), and final branch support values were computed using the ‘-f b’ option. To assess the reliability of the RAxML tree’s internal branches, further validation was performed using IQ-TREE2 version 2.3.5 (Minh et al., 2020). The optimal substitution model in IQ-TREE2 was selected using the ModelFinder feature, and the tree was constructed according to the selected Q.insect+F+R5 model. Bootstrap support for internal branches was assessed with 1,000 replicates, and approximate likelihood ratio tests (aLRT) were performed to validate the tree topology with 1,000 iterations.
The average nucleotide identity values were calculated using the OrthoANI-usearch tool (Yoon et al., 2017b), and digital DNA-DNA hybridization (dDDH) was calculated using the Genome-to-Genome Distance Calculator provided by DSMZ (Braunschweig, Germany) (Meier-Kolthoff et al., 2013). The AAI calculator by EzAAI version 1.2.3 (Kim et al., 2021) was used to compare two genomes with amino acids with a 20% minimum identity and 50% minimum query coverage threshold. The POCPs were calculated according to the method previously described (Qin et al., 2014). The POCP is a comparison of the amino acid sequences of the genomes of two strains using BLASTp. Roary 5.26.2 version (Page et al., 2015) was used to summarize the unique genes and features of the distinct genera. KofamKOALA (Aramaki et al., 2020) was used based on the Prokka numbers and protein sequences of 47 strains in the family Colwelliaceae. The codon usage bias of each group was analyzed to understand their evolutionary relationships and clustering. This was done by calculating the relative synonymous codon usage (RSCU), which reflects evolutionary processes influenced by factors such as G + C content, replication strand skew, and gene expression (Sharp et al., 2005). Codon usage was determined using the tool available at https://www.bioinformatics.org/sms2/codon_usage.html. The resulting values were then visualized using Plotly version 4.10.4 (Sievert, 2020) on the RStudio server (Carpenter et al., 2021).
2.4 Biochemical and morphological tests
The cell growth of strains MEBiC14330T, MEBiC06441T, MEBiC06471T, and MEBiC02087T were observed for 5 days on MA plates at 4, 10, 15, 20, 25, 28, 30, 35, and 45 °C. The pH tolerance range was determined at pH 3, 4, 5, 6, 7, 8, 9, and 10 in marine broth, with the pH adjusted using 10 mM MES (pH 4–6), 10 mM HEPES (pH 6–8), or 10 mM AMPSO (pH 8–10) as biological buffers. The salinity range for growth was tested in MB prepared from distilled water with 0, 0.5, and 1–10% (w/v) NaCl at intervals of 1%. The pH and NaCl growth tolerance test were observed for up to 5 days at 25°C in a 180 rpm shaking incubator and measured at OD600. Catalase and oxidase activities were determined as previously described (Cappuccino and Sherman, 2014). Growth under anaerobic conditions was investigated using the GasPak Ez anaerobic pouch system (BD Biosciences) over 7 days on MA and MA supplemented with potassium nitrate at a concentration of 0.1%. Biochemical tests were performed using API kits (API 20NE, API 50CH, and API ZYM) according to the manufacturer’s instructions (bioMérieux, Marcy l’Etoile, France). The API 20NE and 50CH kit results were observed after 24 and 48 h at 25°C. Gram staining was performed as previously described (Buck, 1982). Morphological characteristics were determined using transmission electron microscopy (Hitachi HT7800, Hitachi, Tokyo, Japan) of cells negatively stained with 2% uracil.
Fatty acid profiles were analyzed by the Korea Culture Center of Microorganisms (KCCM), using MIDI with Sherlock version 6.3 and the RTSBA6 database (Sasser, 1990). The polar lipids of the four strains were extracted and analyzed using two-dimensional ascending thin-layer chromatography (Minnikin et al., 1984). The major respiratory quinones were determined by high-performance liquid chromatography, as previously described (Collins et al., 1979). Cellular quinone analysis was performed by high-performance liquid chromatography using a reversed-phase C18 column as previously described (Minnikin et al., 1984).
3 Results
3.1 16S rRNA gene sequence-based phylogenetic analysis
To assess the taxonomic relationships of the four novel strains, 16S rRNA gene sequencing and phylogenetic analysis were conducted. The 16S rRNA gene sequence lengths obtained by Sanger sequencing were 1,415, 1,411, 1,411, and 1,431 bp, respectively. Phylogenetic analysis based on 16S rRNA gene sequences was conducted to elucidate the relationships among the four novel strains; however, low bootstrap values indicated weak support for these relationships (Supplementary Figure S1). The similarities between MEBiC14330T, MEBiC06441T, MEBiC06471T, and MEBiC02087T and other strains were as follows: strain MEBiC14330T: Colwellia asteriadis KMD 002T (96.75%), Litorilituus lipolyticus RZ04T (96.26%), and C. psychrerythraea ATCC 27364T (95.91%); strain MEBiC06441T: Thalassotalea piscium T202T (96.21%), C. hornerae ACAM 607T (95.35%), and C. piezophile Y223GT (95.13%); strain MEBiC06471T: Tst. profundi YM155T (96.01%), Tsm. viridans XOM25T (95.63%), and C. meonggei MA1-3T (95.49%); strain MEBiC02087T: Tsm. haliotis A5K-61T (95.60%), C. hornerae ACAM 607T (95.35%), and Cognaticolwellia beringensis NB097-1T (95.13%). All similarity values were calculated based on the valid names, and the top three strains with a similarity of over 95% were described.
In the phylogenetic tree, Litroilituus was located within the Colwellia clade, and some strains of Pseudocolwellia and Colwellia were located within the Cognaticolwellia clade, making the boundary between these genera ambiguous. This suggests the need for whole-genome analysis to clarify the phylogenetic relationships.
3.2 Genomic characteristics and genome-based phylogeny
The genomes of the strains MEBiC14330T, MEBiC06441T, MEBiC06471T, and MEBiC02087T were assembled de novo from 234, 136, 27, and 121 contigs, respectively. The genome size of new strains ranged from 4.19 to 4.46 Mb, concurrent with those of current Colwelliaceae members (3.30–7.95 Mb). Other genomic features are detailed in Supplementary Table S1. The 16S rRNA gene results obtained by Sanger sequencing were compared with those of the 16S rRNA gene sequences in the whole-genome assembly to verify the accuracy of the assembly. The lengths of the 16S rRNA extracted from the genome were 1,539, 1,537, 1,539, and 1,541 bp, respectively. The results were 99.01, 98.94, 98.31, and 99.72% for MEBiC14330T, MEBiC06441T, MEBiC06471T, and MEBiC02087T, respectively. The completeness of the assembled genomes was 97.81, 99.66, 99.66, and 98.94% for the four strains, respectively, and contamination was 1.32, 2.35, 1.62, and 0.52%, respectively. The six genera in the Colwelliaceae family have genome sizes of 3.72–5.72 Mb (Colwellia), 4.14–4.28 Mb (Litorilituus), 3.85–4.66 Mb (Cognaticolwellia), 4.72 Mb (Pseudocolwellia), 3.30–4.90 Mb (Thalassotalea), and 6.45–7.95 Mb (Thalassomonas). The G + C contents are 36.8% to 39.6%, 37.4% to 39.4%, 37.5% to 41.9%, 35.8%, 36.3% to 45.9%, and 47.3% to 48.9%, respectively. Genera classifications before reclassification are presented in Supplementary Table S1.
In contrast to 16S rRNA gene-based phylogeny (Supplementary Figure S1), the phylogenomic tree provided a clear relationship (Figure 1); therefore, references for the four novel strains were selected from the phylogenomic tree, and the strain designation numbers are shown in Supplementary Table S1. The closest relatives of each novel strain were as follows: MEBiC14330T: C. ponticola OISW-25T; MEBiC06441T: Tst. castellviae W431T and Tst. insulae JDTF-40T; MEBiC06471T: Tsm. viridans XOM25T; and MEBiC02087T: Tst. psychrophile SQ149T, and Tst. crassostreae LPB0090T. Therefore, relatives were selected based on Figure 1, and strains that were easily obtainable and for which direct experiments could be conducted were selected. The reference relationships for each strain are indicated by numbers in Supplementary Table S1 for all subsequent descriptions.
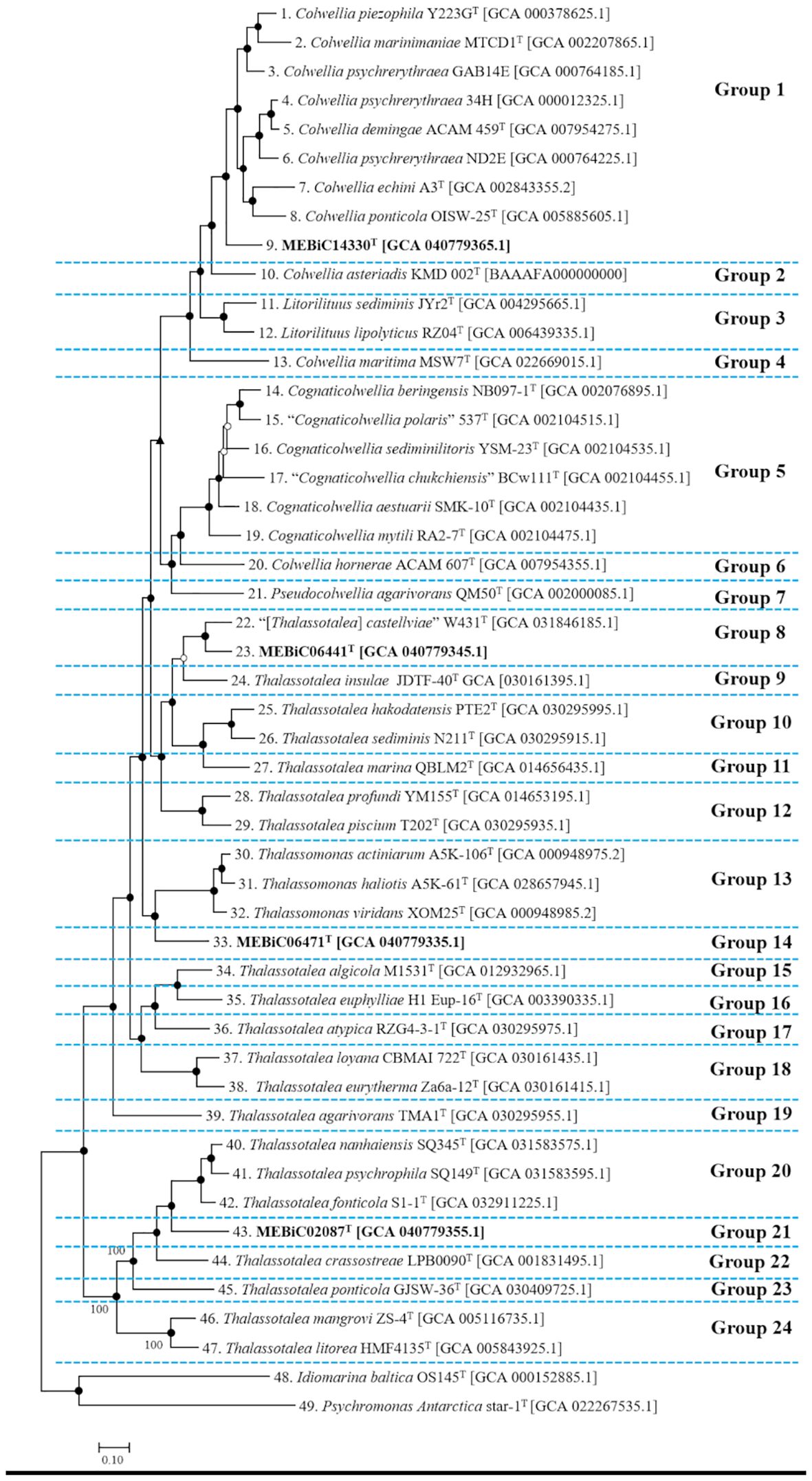
Figure 1. Phylogenomic tree of strains belonging to the family Colwelliaceae. A total of 47 strains within the family Colwelliaceae were subdivided into 24 groups based on a branch length threshold of 0.2. Two strains from the order Alteromonadales were selected as outgroups. The numbers preceding each scientific name correspond to the identical organisms in subsequent analyses. The scale bar represents 10.0% estimated sequence divergence. Node support values were determined using bootstrap values from PhyloPhlAn, approximate likelihood ratio test (aLRT) values from IQ-TREE, and additional bootstrap values. Node support is indicated as follows: ▲) for nodes with >90% support across all methods, (○) for nodes with 70–90% support, and (▲) for nodes where at least one method yielded <70% support.
Most target genomes have been isolated from marine environments. Additionally, some species have been isolated from cold environments (Arctic sea ice, Arctic marine sediments, and the deep sea). However, their genomic characteristics differed. The genome size ranged from 3.30–7.95 Mb, and the G + C content ranged from 35.8% to 48.9%. Other information regarding the genome (contig number, N50, coding DNA, rRNA, tRNA, tmRNA, and CRISPR repeat regions) is summarized with the GenBank assembly accession numbers in Supplementary Table S1.
When the amino acid sequence similarity between two genomes exceeds 80%, they can be classified into the same genus (Luo et al., 2014). This threshold has been widely applied in taxonomic studies (Liu, Zhang, Cheng, et al., 2020; On et al., 2020; Soutar and Stavrinides, 2022; Suresh et al., 2019). To reflect this classification threshold (corresponding to an estimated 20% sequence divergence), we identified groups with a phylogenetic distance of 0.2 or greater. The 47 Colwelliaceae genomes were divided into 24 groups, excluding the outgroup (Figure 1). Furthermore, strains 2, 4, and 6, numbered based on Figure 1, were not included because of the lack of genomic information. Therefore, genome-based classification was limited compared with the 16S rRNA gene-based phylogenetic tree. Groups 1, 2, 4, and 6 (Figure 1) were previously in the genus Colwellia; however, only Group 1 was retained in the genus Colwellia, and the newly reported novel strain MEBiC14330T was included in Group 1. In addition, Groups 2, 4, and 6 should be classified as different genera, and genome-based phylogenetic results revealed new genera. Groups 8, 9, 10, 11, 12, 15, 16, 17, 18, 19, 20, 22, 23, and 24 were assigned to the genus Thalassotalea; however, like Colwellia, they formed different clades, suggesting that they were different species. These results indicate that the family Colwelliaceae should be reorganized into 24 genera, including three genera comprising new strains from the existing six genera. A more detailed phylogenomic tree of these strains, including bootstrap values and aLRT, support values, is provided in Supplementary Figure S2.
3.3 Phenotypic and chemotaxonomic characterization
The four novel strains are described according to type, along with each reference strain (Figure 1). Type 1: strains 9 and 8; Type 2: strains 32 and 33; Type 3: strains 22, 23, and 24; Type 4: strains 41, 43, and 44. The reference species selected for the direct comparison were as follows: C. ponticola OISW-25T, Tst. insulae JDTF-40T (Korean Collection for Type Cultures, Jeollabuk-do, Korea), and Tst. crassostreae LPB0090T (Korean Agricultural Culture Collection). The criteria for classifying these types were selected based on a genome-based phylogenetic tree. The criteria were selected based on strains that were easy to obtain, could be directly experimented on, and were published at the time of the comparative experiment. Therefore, strain 42 was not compared to Type 4.
MEBiC14330T, MEBiC06471T, MEBiC06441T, and MEBiC02087T are aerobic, motile, gram-negative, and catalase- and oxidase-positive bacteria. Microscopic images showed that all four strains were rod–shaped. All strains also had flagella except for MEBiC02087T (Supplementary Figure S3). The optimal temperature, pH, and NaCl concentration for growth of strain MEBiC14330T was 28°C, pH 7, and 2.0%, respectively. The optimal growth values for MEBiC06471T were 25°C, pH 7, and 1.0% NaCl, respectively. The optimal growth values for MEBiC06441T were 20°C, pH 7, and 1.0% NaCl, respectively. The optimal growth values for MEBiC02087T were 9°C, pH 7–8, and 2.0–3.0% (Table 1). The optimal temperature, pH, and concentration of NaCl for Type 1 were similar to members in the genus Colwellia. Types 2 and 4 were strains belonging to different genera with large differences in their growth ranges. In Type 2, MEBiC06471T grew at a higher temperature than strain 32, and a difference in the presence or absence of nitrate reduction was observed (strain 32 is a psychrophile). In Type 3, MEBiC06441T and strain 22 were of the same genus; however, unlike strain 24, they hydrolyze starch and have yellow or brown pigments. Type 4 strains showed large differences in growth ranges, and MEBiC02087T is a psychrophilic strain with a low growth temperature.
The chemotaxonomic data showed similar basic characteristics with the exception of MEBiC02087T. All Type 1 strains showed activity toward esculin ferric citrate and inulin and used the naphthol-AS-BI-phosphohydrolase enzyme. However, MEBiC14330T showed negative activities toward arabinose, adonitol, and arabitol and did not use L-arginine and urea enzymes. MEBiC06471T was positive for nitrate reduction and negative for starch and urea hydrolysis and D-glucose use. MEBiC06441T differed from the other two strains as it was negative for casein and gelatin hydrolysis, whereas MEBiC06441T and strain 22 of the same genus did not hydrolyze starch. MEBiC02087T was motile and positive for maltose use (Table 1).
In Type 1, the major fatty acids of the two strains were the same; however, C16:0 and summed feature 3 (C16:1 ω7c and/or C16:1 ω6c) showed a difference of > 10%, and MEBiC14330T also showed a high value of 9.5% in summed feature 8 (C18:1 ω7c and/or C18:1 ω6c). Type 2 had the same C16:0 and summed feature 3 as their major fatty acids; however, C17:1 ω8c in strain 32 was 19.7%, which was >10% higher than that in MEBiC06471T. Strain 22 was excluded owing to lack of fatty acid information, and Type 3 had C15:1 ω8c, C17:1 ω8c, and summed feature 3 as major fatty acids. Additionally, MEBiC06441T had C16:0 as a major fatty acid. Type 4 showed substantially different fatty acid compositions, and MEBiC02087T showed a high proportion of C16:1 ω9c, C12:0 3OH, and summed feature 3 (Supplementary Table S2).
Except for the undocumented strains, all strains contained the same major polar lipids, namely phosphatidylethanolamine and phosphatidylglycerol. MEBiC14330T, MEBiC06471T, and MEBiC06441T contained phosphatidylethanolamine, phosphatidylglycerol, and unidentified amino lipids and lipids. Accordingly, the major polar lipid composition of Type 1 was different. Type 3 strain 24 differed because it had a glycolipid. Type 4 had the same composition, and all contained phosphatidylethanolamine and phosphatidylglycerol (Supplementary Figure S4).
All strains, except Type 4, had Q-8 as the major quinone. Type 4 strains 44 and 41 contained Q-8; however, strain 43 also contained MK-7 as the major quinone. MK-6 of MEBiC02087T is species-specific. These characteristics differed among clade members and could be used to distinguish clade members from the four novel strains. However, the classification of the four novel strains was challenging because of the complexity of Colwelliaceae; therefore, additional taxogenomics was performed.
3.4 Matrix of genome indices
The AAI value is used as a standard to distinguish genera within a certain range, which varies from 60–80% (Konstantinidis and Tiedje, 2005; Rodriguez-R and Konstantinidis, 2014). AAI of 80–85% or 79% is used to distinguish species of the genus Thalassotalea within the family Colwelliaceae (Liu et al., 2020a). However, distinguishing between the genera of the entire family Colwelliaceae was not possible, and whether this is an appropriate AAI standard must be confirmed. To avoid subjective intervention, a previously published method of determining the thresholds was followed using clustering and scoring (Park et al., 2022). In this approach, the initial threshold was set to 60.00, and clustering was performed accordingly. A stringent penalty was applied for violations of monophyly, while a milder penalty was imposed for the formation of singleton groups. The threshold was then incrementally increased by 0.01 to determine the optimal value. When calculating the AAI values, the outgroups (48 and 49) value was removed before proceeding. The EzAAI value was 2.16 ± 0.53 higher than that of the Kostas Lab AAI calculator previously used (Park et al., 2022). Applying the EzAAI values, the AAI threshold range was calculated as 74.07–75.11%. Based on the determined threshold range, the separated groups in the column were represented by one square, resulting in 24 squares (Figure 2) identical to the genome tree (Figure 1).
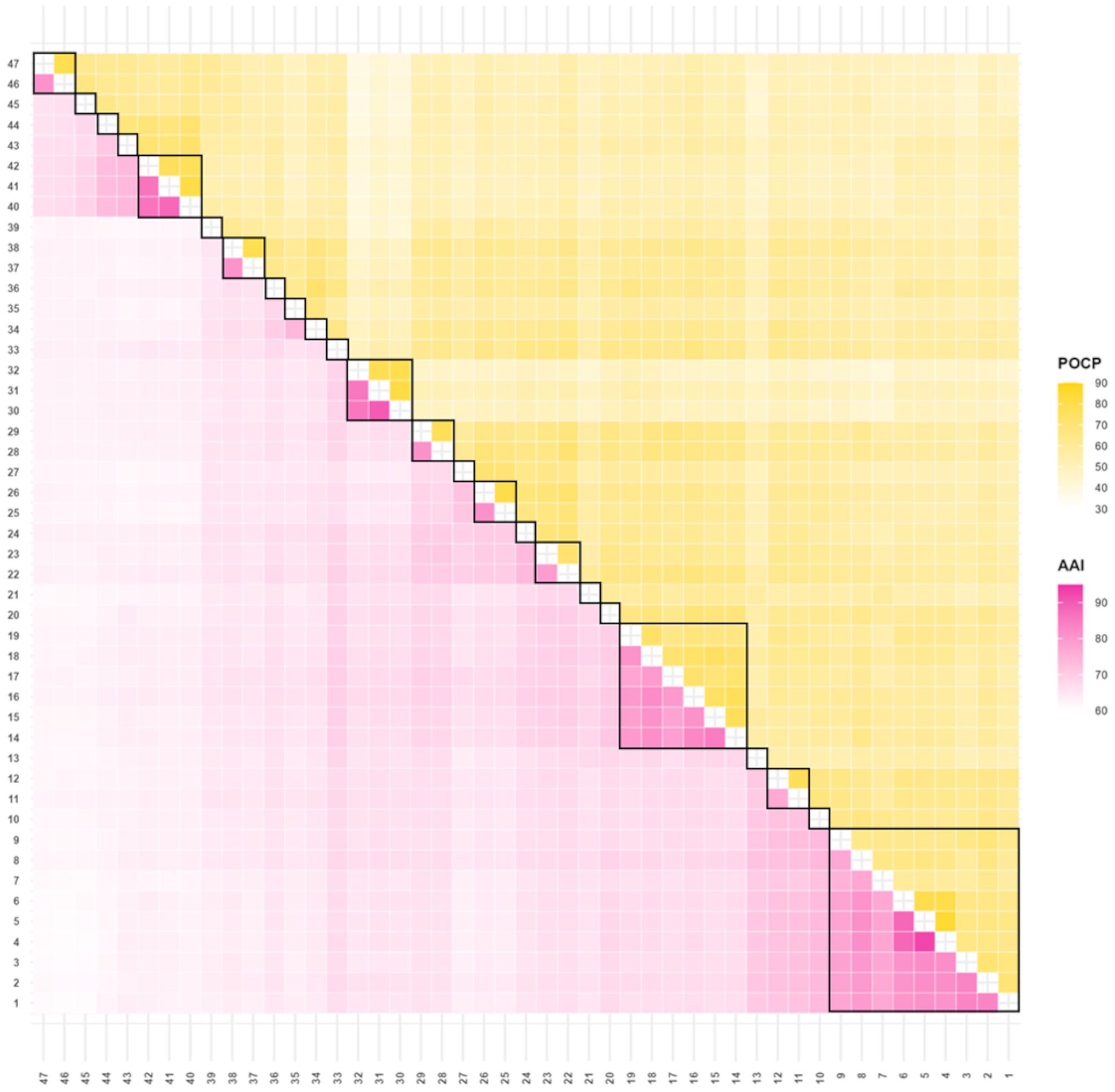
Figure 2. AAI and Percentage of Conserved Protein (POCP) from pairwise whole-genome comparisons. The AAI and POCP values are expressed as heat maps. The lower left triangle in pink indicates AAI, and POCP is indicated in yellow in the upper right triangle. The group was subdivided into 24 groups when it was divided based on the AAI value alone, which are separately surrounded by a black square on the figure.
In addition to the AAI value, POCP is also applied as an auxiliary standard, and 50% was recommended as the genus classification value for a specific group (Qin et al., 2014). However, applying a POCP threshold of 50% is not an appropriate standard for classifying species into many taxa. For example, a 65% POCP value for the genome-based classification of Geobacterales was used to separate the genus Geotalea into two lineages; however, phylogenomic analysis and AAI values did not support these results (Xu et al., 2021). The AAI and POCP results for each genome are displayed as a heat map in Figure 2, and detailed values can be found in Supplementary Tables S3, S4. The average nucleotide identity and digital DNA–DNA hybridization results, used as criteria for species differentiation between genomes, are summarized in a heatmap (Supplementary Figure S5). Detailed values are provided in Supplementary Tables S5, S6. Therefore, these results indicate that it is appropriate to reclassify Colwelliaceae into 24 genera based on their AAI values and phylogenomic results, excluding the POCP values.
3.5 Comparison of codon bias
The relative synonymous codon usage values were calculated, and the codon usage bias was measured to test the evolutionary relationships and clustering (Supplementary Table S7; Supplementary Figure S6). Groups 1, 5, and 12 were considered individual genera; however, the plot distances of each species were relatively large compared to the distances of species in other groups (Supplementary Figure S7). In particular, species 3, 4, and 6 in Group 1 were the same species, namely C. psychrerythraea; however, the plot results showed different codon usage. Among the Colwellia strains belonging to Group 1, C. psychrerythraea formed different clades, including C. psychrerythraea GAB14E, C. psychrerythraea 34HT, and C. psychrerythraea ND2E and should be separated into different strains. The three C. psychrerythraea strains differed, with values below the cutoff value (Supplementary Table S8). In addition, strains 3, 4, and 6 were classified as the same species, C. psychrerythraea. However, strains 3, 4, and 6 were separated by a distance of 0.38 and 0.22, respectively, and strains 4 and 6 were also related to each other by a distance of 0.2 (Supplementary Figures S8, S9). Additionally, the genomes of three C. psychrerythraea species show significant differences in genomic and phenotypic diversity (Techtmann et al., 2016). Group 5 was also divided into the same genus but formed a long distance. Codon usage across species can vary greatly, depending on mutations or genetic patterns. Therefore, they are limited to certain taxa and classes (Plotkin and Kudla, 2011). The average nucleotide identity (Supplementary Table S5) and digital DNA–DNA hybridization (Supplementary Table S6) values were also used to distinguish between species, and different C. psychrerythraea strains (strains 3, 4, and 6) were distinguished as independent species.
3.6 Defining phenotype and genotype characteristics for each group
Physiological and biochemical characteristics of all species within the Colwelliaceae family were collected by reviewing novel species descriptions and substrate utilization studies available in PubMed. The collected data were then organized in Table 2 according to the newly reclassified genera. Based on the summarized characteristics, a new genus name was determined, and the corresponding generic name was adopted and described for accurate distinction in the subsequent description. Most cells were rod-shaped (bacillus) and motile with flagella. However, the genera “Colwellia,” “Litorilithuus,” “Cognaticolwellia,” “Petrothalassotalea,” “Aminothalassotalea,” “Saccharothalassotalea,” and “Aquiithalassomonas” include strains with a curved shape (vibroid), suggesting that a single genus can exhibit various forms beyond bacillus. Regarding the growth conditions, the characteristics of each group became clear. Differences in optimal growth temperatures were observed depending on the isolation environment and source. Because all species in the genera were isolated from marine environments, they required at least 1.0% (w/v) NaCl. On the genome tree (Figure 3), strains in the clade formed by “Petrothalassotalea” to “Thalassotalea” hydrolyzed casein and were positive for nitrate reduction. All strains included in “Thalassomonas” require artificial seawater containing NaCl, Mg²+, Ca²+, and K+ for growth. Strains in the clade formed by “Algithalassotalea” to “Saccharothalassotalea” had a relatively high optimal growth temperature of 25 °C or higher, and all strains were rod–shaped. No flagellation was observed from “Saccharothalassotalea” onwards. They were also classified based on the presence or absence of motility. Strains were negative for casein hydrolysis and aerobic from “Psychrothalassomonas” onwards. Strains in the clade formed by “Psychrothalassomonas” and “Arcticithalassomona” had ubiquinone and menaquinone as quinones. The genus “Litorithalassotalea” requires nitrate as an essential growth factor. Unlike other groups that have Q-8, “Psychrothalassomonas” and “Arcticithalassomonas” have MK-7 and MK-6 as their major quinones, respectively. The difference in DNA G + C content shows that all species in the “Litorithalassotalea,” “Thalassomonas,” “Vitithalassotalea,” “Aminothalassotalea,” “Saccharothalassotalea,” “Aquiithalassomonas,” and “Solithalassomonas” groups have DNA G + C content of >40%.
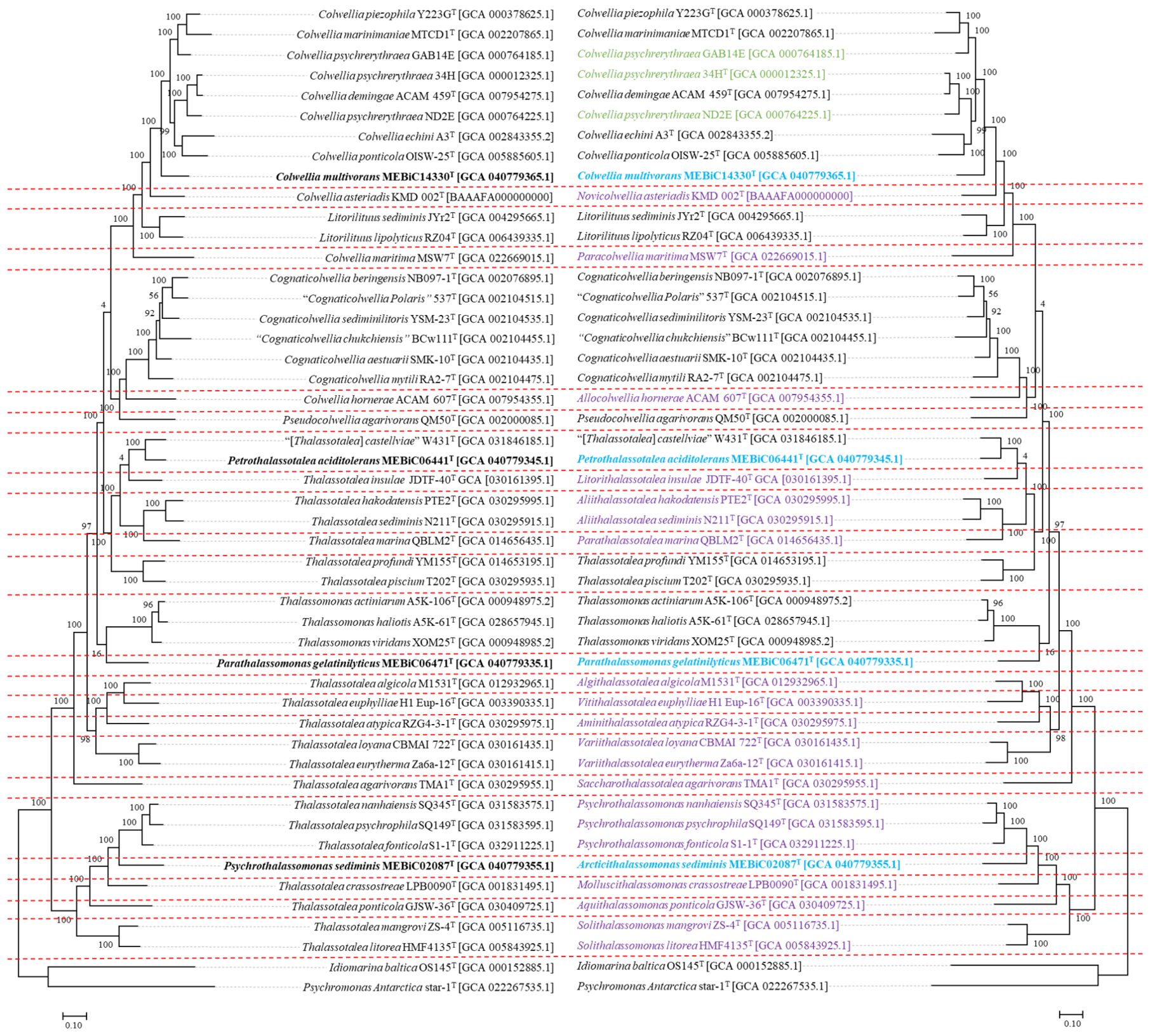
Figure 3. Reclassification of Colwelliaceae using whole-genome sequence similarity inferred by a genomic tree. This figure includes a mirror image. The left-sided tree represents before reclassifications, and the right-sided tree represents after reclassifications. Purple labels indicate that a new genus has been proposed in this study, blue labels indicate that a species or genus has been newly proposed in this study, and green labels indicate that a species is currently in need of further research as it may be classified as a different species. The tree is based on Maximum Likelihood with 1,000 bootstraps.
3.7 Potential metabolic differences between groups
Although a distinction between the genera was also observed in the phenotype, the potential metabolic differences were compared based on genome annotation to perform a more specific comparison between the genera (Figure 4). The methanogenesis, coenzyme, methane and methanol, photosystem, and nitrogen fixation pathways were absent in the family Colwelliaceae. Among the 20 amino acids, cysteine, tyrosine, and histidine were absent or were species-specific.
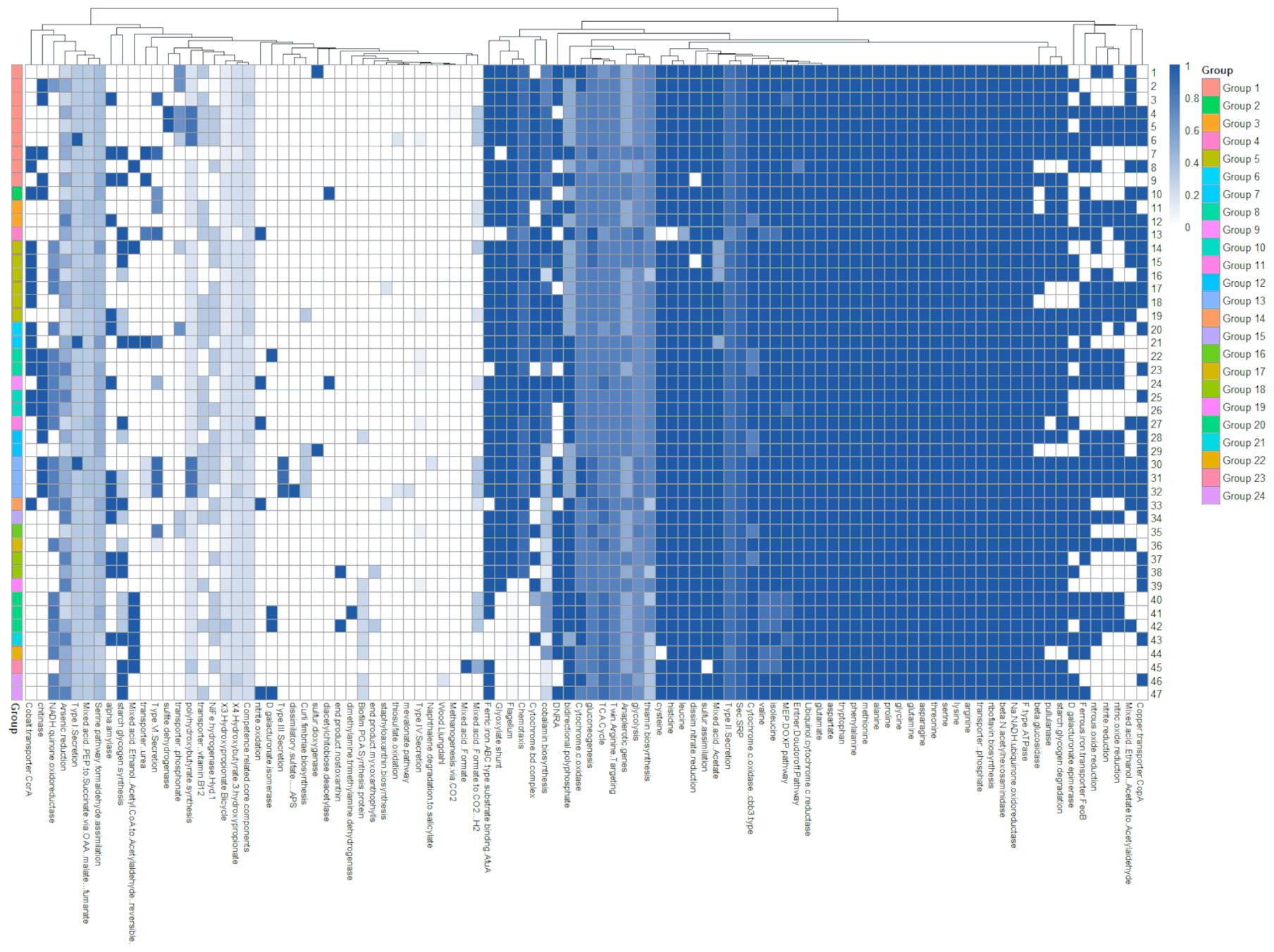
Figure 4. A heatmap based on the Kyoto Encyclopedia of Genes and Genomes metabolic pathways, created by extracting only active categories from the Kofamscan results. The same color on the left distinguishes groups of the same type. Relative abundance (%) is indicated by the blue bar.
Novicolwellia (Group 2) had complete pathways for dimethylamine, trimethylamine dehydrogenase, and chitinase, distinguishing it from other groups in the same clade. Litorilituus (Group 3) had complete pathways for all metabolic pathways related to nitrate reduction, distinguishing it from other groups in the same clade. Paracolwellia (Group 4) had complete pathways for nitrate oxidation but no pathways for the glyoxylate shunt and flagellum. Strains in Cognaticolwellia (Group 5) had complete pathways for ferric iron AGC types substrate binding to AfuA, glyoxylate shunt, flagellum, chemotaxis, cytochrome bd complex, and cobalamin biosynthesis in all six strains. Allocolwellia (Group 6) had the same pathways as Cognaticolwellia (Group 5) but had a different metabolic pathway for the transporter phosphonate. The metabolism of phosphonate transporters is a characteristic feature of Allocolwellia (Group 6) isolated from Antarctica, reflecting the high abundance of phosphonate-producing genes in Antarctic seawater (Lockwood et al., 2022). Pseudocolwellia (Group 7) had the same pathway as Cognaticolwellia (Group 5); however, starch glycogen synthesis, mixed acid—ethanol, acetyl-CoA to acetaldehyde (reversible)—transporter urea, and type VI sections were the only pathways in Pseudocolwellia (Group 7). Petrothalassotalea (Group 8) and Litorithalassotalea (Group 9) are groups within the same clade with complete chitinase and nitrate pathways; however, Petrothalassotalea had the cobalt transporter CorA, whereas Litorithalassotalea had dimethylamine trimethylamine dehydrogenase. Aliithalassotalea (Group 10) and Parathalassotalea (Group 11) were grouped within the same clade, which did not have a nitrate pathway and contained chitinase. However, Aliithalassotalea had the cobalt transporter CorA, whereas Parathalassotalea had a mixed acid-ethanol, acetyl-CoA to acetaldehyde (reversible). Thalassotalea (Group 12) completely metabolizes nitrate, and some species contain sulfur dioxygenase. Sulfur dioxygenase metabolism is associated with the common sulfide toxins found in marine animals and is a prominent feature of Thalassotalea isolated from marine organisms (Zhang et al., 2013). Thalassomonas (Group 13) had complete pathways for nitrite reduction, nitrous oxide reduction, nitric oxide reduction, cobalt transporter CorA, chitinase, curli fimbriae biosynthesis, and type II secretion. Thalassomonas is isolated from marine organisms inhabiting intertidal zones and metabolizes the cobalt transporter CorA, which is associated with osmotic stress (Guskov and Eshaghi, 2012). Parathalassomonas (Group 14) had nitrate oxidation and the cobalt transporter CorA metabolic pathways, distinguishing it from other species forming the same clade. Algithalassotalea (Group 15) to Variithalassotalea (Group 18) formed a single clade and showed complete pathways for ferric iron ABC-type substrate binding of AfuA, glyoxylate shunt, and chemotaxis. Vitithalassotalea (Group 16) did not have a pathway for nitrate but had a pathway for Type VI secretion. The Type VI secretion system is a defense mechanism against coral disease outbreaks and is a characteristic feature of strains isolated from symbiotic coral environments (Wang et al., 2024). Aminithalassotalea (group 17) was the only group in this clade with a nitrate pathway. Variithalassotalea (group 18) had complete pathways for alpha-amylase and starch glycogen synthesis. Saccharothalassotalea (Group 19) had a pathway for biofilm PGA synthesis, different from Algithalassotalea (Group 15) to Variithalassotalea (Group 18). Psychrothalassomonas (Group 20) and Solithalassomonas (Group 24) formed a separate clade and did not exhibit metabolism in the glyoxylate shunt, flagellum, or chemotaxis. In addition, they possess a pathway for biofilm PGA synthesis, unlike other groups.
These findings indicate that the groups that are phylogenetically distinguished into clades have specific metabolic pathways. Many shared characteristics exist among members in the family Colwelliaceae, yet each genus exhibits sufficiently distinctive features for clear differentiation (Supplementary Figure S10).
4 Discussion
Although a genome-based reclassification of the Colwelliaceae, which was widely distributed in marine environments, performed in 2020 (Liu et al., 2020b), it was based on only 14 publicly available genomes at the time, failing to provide clear taxonomic criteria for many newly isolated Colwelliaceae species. Consequently, determining the precise phylogenetic position of four newly isolated Colwelliaceae strains based on 16S rRNA gene sequences was also challenging. Therefore, constructing a whole-genome phylogenetic tree was necessary to clarify their phylogenetic placement. To address this, we conducted an extensive reclassification using all available Colwelliaceae genomes, following a taxogenomic approach. This approach was inspired by previous studies that resolved taxonomic discrepancies and corrected misidentifications through genome-based reclassification, preventing future errors (Liang et al., 2021). Furthermore, genome-based reclassifications of the family Desulfovibrionaceae (Park et al., 2022) have revealed clear taxonomic trends, which guided our adoption of AAI-based genus delineation thresholds. Using this approach, we incorporated the genomes of 47 available strains, expanding the previously recognized six genera and reclassifying the family Colwelliaceae into 24 genera. The 24 genera were isolated from marine environments and shared many features, such as their Gram staining properties and requirement for NaCl for growth; however, they were distinguished from each other by their DNA G + C content, range, optimal growth temperature, respiration mode, and fatty acid composition. Differences were also observed in the metabolic potential predicted by genome annotation. The two genera (Psychrothalassomonas, Arcticithalassomonas) can be distinguished from each other by their markedly different major quinones (Groups 20 and 21), essential ion requirements for growth (Groups 1, 5, 13, 17, 20, and 22), and growth temperature ranges (Group 21 is psychrophilic). However, to propose a new taxon or change an existing taxon, Rule 30-3b of the International Code of Nomenclature of Prokaryotes must be followed, which indicates that the type strain must be deposited in two publicly recognized public culture collections. The classification cannot be ideally completed because some taxa of Colwelliaceae do not meet the mentioned conditions, owing to deposits remaining in only one culture collection or the loss of resources (Allocolwellia hornerae ACAM 607 and the Variithalassotalea loyana CBMAI 722). However, based on these data, 16 new genera, four new species, namely Colwellia multivorans MEBiC14330T (=KCCM 43508T = MCCC 1K09162T), Petrothalassotalea aciditolerans MEBiC06441T (=KCCM 43506T = MCCC 1K09573T), Parathalassomonas gelatinilyticus MEBiC06471T (=KCCM 43507T = MCCC 1K09163T), and Arcticithalassomonas sediminis MEBiC02087T (=KCCM 42335T = JCM 14072T), and three additional genera, namely Petrothalassotalea, Parathalassomonas, and Arcticithalassomonas, were proposed by reclassifying members of the family Colwelliaceae. The details of this process are described below.
5 Description
5.1 Emended description of the genus Colwellia Deming et al., 1988 emend. Liu et al., 2020
Colwellia [Colwel’li’a. M. L. ending -ia. M. L. fem.n. Colwellia; named after Rita Colwell to honor her work in the systematics of marine bacteria]
This emended description is based on the original description by Deming et al. (1988) and Liu et al. (2020a), with the following additions: Cells are Gram-stain negative, curved or straight rods. Most species are motile by means of a single polar flagellum. Catalase and oxidase positive. The predominant ubiquinone is Q-8. The DNA G + C content is 36.8% to 39.6%. The most abundant fatty acids and polar lipids are summarized as summed feature 3 (C16:1 ω7c and/or C16:1 ω6c), and C16:0, and phosphatidylethanolamine and phosphatidylglycerol, respectively. The Type species is Colwellia psychrerythraea. A member of the family Colwelliaceae, the class Gammaproteobacteria, according to 16S rRNA gene and whole genome sequence analysis.
5.2 Description of Colwellia multivorans sp. nov.
Colwellia multivorans [mul.ti.vo’rans. L. masc. adj. multus many; L. part. adj. vorans devouring, eating; N.L. part. adj. multivorans devouring different kinds of carbon source]
Cells are Gram-stain-negative, aerobic, motile, and catalase- and oxidase-positive. Colonies grown on MA are smooth, convex and punctiform and the cell size was 1.2–8.0 µm × 0.4–1.0 µm. Growth is observed at 25–30°C (optimal temperature 28°C), pH 6–8 (optimal pH 7), and 1.0–4.0% (optimum 2.0%) NaCl. The major fatty acids and quinones are C16:0 and summed feature 3 (C16:1 ω7c and/or C16:1 ω6c) and Q-8, respectively. Polar lipids mainly included phosphatidylethanolamine, phosphatidylglycerol, three unidentified aminolipids, and three unidentified lipids. In the API kit result, nitrate reduction is negative. In the API 50CH and 20NE system, acid is produced from the following substrates; Glycerol, D-Glucose, Methyl-α D-Mannopyranoside, N-Acetyl Glucosamine, Esculin ferric citrate, D-Melibiose, Inulin, and D-Tagatose. But negative for Erythritol, Arabinose, D-Adonitol, Methyl-β D-Xylopyranoside, Xylose, Galactose, Inositol, Cellobiose, D-Lactose, Glycogen, Xylitol, Gentiobiose, D-Turanose, D-Lyxose, L- Fucose, L-Arabitol, Urea, Gellatine, Capric acid, Adipic acid, Malic acid, and phenylacetic acid. Enzymes that exhibited activity include Alkaline Phosphatase, Leucine Arylamidase, Valine Arylamidase, Trypsin, α-Chymotrypsin, Acid Phosphatase, Naphtol-AS-BI-Phosphohydrolase, and N-acetyl-β-Glucosaminidase. In contrast, α-Galactosidase, β-Glucuronidase, β-Glucosidase, α-Glucosidase, α-Mannosidase, and α- Fucosidase are negative in enzyme activity. The DNA G + C content is 38.2%.
The type strain is MEBiC14330T (=KCCM 43508T = MCCC 1K09162T), isolated from 1,300m depth sediment collected at Gordin Guyot (seamount). The GenBank/DDBJ/EMBL accession number for the 16S rRNA gene is OR958774 and that of the genome is JBFONR000000000.
5.3 Description of Novicolwellia gen. nov.
Novicolwellia [No.vi.col.well’i.a. L. masc. adj. novus, new; N.L. fem. n. Colwellia, a prokaryotic genus from the American microbiologist Rita R. Colwell. N.L. fem. n. Novicolwellia a new Colwellia]
Cells are Gram-stain-negative, facultative anaerobic, rod-shaped, and motile. Catalase- and oxidase-positive. Nitrate reduction is positive. The predominant ubiquinone is Q-8. The major fatty acids are summed feature 3 (C16:1 ω7c and/or C16:1 ω6c) and C16:0. The DNA G + C content of type species is 38.0%. The type species is Novicolwellia asteriadis. A member of the family Colwelliaceae, the class Gammaproteobacteria, according to 16S rRNA gene and whole genome sequence analysis.
5.4 Description of Novicolwellia asteriadis comb. nov.
Novicolwellia asteriadis [as.te′ri.adis. N.L. n. Asterias a zoological genus name; N.L. gen. n. asteriadis of Asterias, referring to the isolation of the type strain from the starfish Asterias amurensis]
Basonym: Colwellia asteriadis Choi et al., 2010
The description is identical to that of Choi et al. (2010).
The type strain KMD 002T (=KCCM 90077T = JCM 15608T) was isolated from the skin of a specimen of the starfish Asterias amurensis collected at the Im-Won port in Samcheok on the East Sea of Korea. The GenBank/EMBL/DDBJ accession number for 16S rRNA gene sequence and genome sequence of strain KMD 002T is EU599214 and NZ_BAAAFA000000000, respectively.
5.5 Description of Paracolwellia gen. nov.
Paracolwellia [Pa.ra.col.well’i.a. Gr. prep. para, next to; N.L. fem. n. Colwellia, a prokaryotic genus from the American microbiologist Rita R. Colwell. N.L. fem. n. Paracolwellia a new genus near the genus Colwellia]
Cells are Gram-stain-negative, aerobic, rod-shaped, and motile. Catalase- and oxidase-positive. Nitrate reduction is positive. The predominant ubiquinone is Q-8. The major fatty acids are C16: 0, C15:1 ω8c, C17: 1 ω8c, and summed feature 3 (C16:1 ω7c and/or C16:1 ω6c). The major polar lipids are phosphatidylethanolamine and phosphatidylglycerol. The DNA G + C content of type species is 37.3%. The type species is Paracolwellia maritima. A member of the family Colwelliaceae, the class Gammaproteobacteria, according to 16S rRNA gene and whole genome sequence analysis.
5.6 Description of Paracolwellia maritima comb. nov.
Paracolwellia maritima [ma.ri′ti.ma. L. fem. adj. maritima, of the marine environment, maritime]
Basonym: Colwellia maritima Kristyanto et al., 2022
The description is identical to that of Kristyanto et al (2022).
The type strain MSW7T (=KACC 22339T = JCM 35001T) was isolated from seawater of Yellow Sea in South Korea. The GenBank/EMBL/DDBJ accession number for 16S rRNA gene sequence and genome sequence of strain MSW7T is MZ310521 and JAKKSL000000000, respectively.
5.7 Emended description of the genus Cognaticolwellia Liu et al., 2020
Cognaticolwellia [Cog.na.ti.col.wel’li.a. L. masc. adj. cognatus relative, related, kindred; N.L. fem. n. Colwellia a bacteria generic name; N.L. fem. n. Cognaticolwellia related to Colwellia]
This emended description is based on the original description by Liu et al. (2020a), with the following additions: Some members require Mg2+ and NaCl for survival.
5.8 Description of Allocolwellia gen. nov.
Allocolwellia [Al.lo.col.well’i.a. Gr. masc. adj. allos, other; N.L. fem. n. Colwellia, a prokaryotic genus from the American microbiologist Rita R. Colwell. N.L. fem. n. Allocolwellia another Colwellia]
Cells are Gram-stain-negative, facultatively anaerobic, curved-rod, and motile. Psychrophilic and chemoheterotroph. The predominant ubiquinone is Q-8. In liquid media, Topt is approximately 12°C and Tmax is about 23–24°C. The DNA G + C content of type species is 38.1%. The type species is Allocolwellia hornerae. A member of the family Colwelliaceae, the class Gammaproteobacteria, according to 16S rRNA gene and whole genome sequence analysis.
5.9 Description of Allocolwellia hornerae comb. nov.
Allocolwellia hornerae [hor.ner′ae. L. adj. hornerae in honor of Rita Horner, an American biologist who pioneered studies on sea-ice microbiota]
Basonym: Colwellia hornerae Bowman et al., 1998
The description is identical to that of Bowman et al. (1998).
The type strain ACAM 607T (=CIP 105821T = ACAM 607T) was isolated from fast sea ice of the Prydz Bay coast, Antarctica. The GenBank/EMBL/DDBJ accession number for 16S rRNA gene sequence and genome sequence of strain ACAM 607T is JN175346 and VOLR00000000, respectively.
5.10 Description of Petrothalassotalea gen. nov.
Petrothalassotalea [Pe.tro.tha.las.so.ta’le.a. Gr. pem. n. petra, rock, stone (referring to petroleum); N.L. fem. n. Thalassotalea, a genus name meaning a rod from the sea; N.L. fem. n. Petrothalassotalea; a Thalassotalea isolated from petroleum contaminated coastal sediments]
Cells are Gram-stain-negative, aerobic, rod-shaped, and motile. Catalase- and oxidase-positive. Nitrate reduction is positive. The predominant ubiquinone is Q-8. The major fatty acids are C15:1 ω8c, C16:0, C17:1 ω8c, and summed feature 3 (C16:1 ω7c and/or C16:1 ω6c). The major polar lipids are phosphatidylethanolamine, phosphatidylglycerol, five unidentified aminolipids, and four unidentified lipids. The DNA G + C content of type species is 37.4%. The type species is Petrothalassotalea aciditolerans. A member of the family Colwelliaceae, the class Gammaproteobacteria, according to 16S rRNA gene and whole genome sequence analysis.
5.11 Description of Petrothalassotalea aciditolerans sp. nov.
Petrothalassotalea aciditolerans [a.ci.di.to’le.rans. L. neut. adj. acidum, an acid; L. pres. part. Tolerans, tolerating; N.L. neut. part. adj. aciditolerans, acid-tolerating]
In addition to the genus description, the following are added: Colonies grown on MA are rough, flat, and irregular. The cell size was 0.9–3.4 µm × 0.4–0.9 µm. Growth is observed at 10–25°C (optimal temperature 20°C), pH 5–7 (optimal pH 7), and 0.5–2.0% (optimum 1.0%) NaCl. In the API 50CH and 20NE system, acid is produced from the following substrates: D-Ribose, L-Xylose, L-Rhamnose, D-Mannitol, Esculin ferric citrate, L-Arabitol, L-arginine and Urea. L-Arabinose, Dulcitol, D-Maltose, D-Lactose, D-Melezitose, D-Raffinose, D-Tagatose, and L-Fucose are weakly positive. Glycerol, Erythritol, D-Arabinose, D-Xylose, D-Adonitol, Methyl-β D-Xylopyranoside, Glucose, Inositol, Methyl-α D-Mannopyranoside, Methyl-α D-Glucopyranoside, N-AcetylGlucosamine, Arbutin, Salicin, Cellobiose, Inulin, Starch, Glycogen, Xylitol, and Gentiobiose are negative. Alkaline phosphatase, Leucine arylamidase, and Valine arylamidase activites are positive, but Esterase, Lipase, Trypsin, α-galactosidase, β-glucuronidase, β-glucosidase, α-glucosidase, β-glucosidase, α-mannosidase, and α-fucosidase activities are negative. Cystine arylamidase, α-chymotrypsin, acid phosphatase, Naphthol-AS-BI-phosphohydrolase, and N-acetyl-β-glucosaminidase activities are weak.
The type strain is MEBiC06441T (=KCCM 43506T = MCCC 1K09573T), isolated from tidal sediment collected at Incheon City, Republic of Korea. The GenBank/DDBJ/EMBL accession number for the 16S rRNA gene is OR958780 and that of the genome is JBFONU000000000.
5.12 Description of Litorithalassotalea gen. nov.
Litorithalassotalea [Li.to.ri.tha.las.so.ta’le.a. L. neut. n. litus, shore; N.L. fem. n. Thalassotalea, a genus name meaning a rod from the sea; N.L. fem. n. Litorithalassotalea, a relative of Thalassotalea from the shore]
Cells are Gram-stain-negative, aerobic, rod-shaped, and motile. Catalase- and oxidase-positive. Nitrate reduction is positive. Some members require Mg2+ ions or Nitrate for growth. The predominant ubiquinone is Q-8. The major fatty acids are C16:0 and summed feature 3 (C16:1 ω7c and/or C16:1 ω6c). The major polar lipids are phosphatidylglycerol, phosphatidylethanolamine, one unidentified aminolipid, one unidentified glycolipid, and three unidentified lipids. The DNA G + C content of type species is 40.3%. The type species is Litorithalassotalea insulae. A member of the family Colwelliaceae, the class Gammaproteobacteria, according to 16S rRNA gene and whole genome sequence analysis.
5.13 Description of Litorithalassotalea insulae comb. nov.
Litorithalassotalea insulae [in′su.lae. L. gen. n. insulae of an island, referring to the source of isolation of the type strain]
Basonym: Thalassotalea insulae Park et al., 2018
The description is identical to that of Park et al. (2018).
The type strain JDTF-40T (=KACC 19433T = KCTC 62186T = NBRC 113040T) was isolated from a tidal flat in Jindo Island, an island of the Republic of South Korea, in the Yellow Sea. The GenBank/EMBL/DDBJ accession number for 16S rRNA gene sequence and genome sequence of strain JDTF-40T is MG592731 and BSST00000000, respectively.
5.14 Description of Aliithalassotalea gen. nov.
Aliithalassotalea [A.li.i.tha.las.so.ta’le.a. L. masc. adj. alius other, another; N.L. fem. n. Thalassotalea, a genus name meaning a rod from the sea; N.L. fem. n. Aliithalassotalea, another Thalassotalea]
Cells are Gram-stain-negative, aerobic or facultatively anaerobic, rod-shaped and motile. Catalase- and oxidase-positive. Nitrate reduction is positive. The predominant ubiquinone is Q-8. The major fatty acids are C16:0, C17:1 ω8c and summed feature 3 (C16:1 ω7c and/or C16:1 ω6c). The major polar lipids are phosphatidylglycerol and phosphatidylethanolamine. The DNA G + C content is 38.4% to 38.6%. The type species is Aliithalassotalea sediminis. A member of the family Colwelliaceae, the class Gammaproteobacteria, according to 16S rRNA gene and whole genome sequence analysis.
5.15 Description of Aliithalassotalea hakodatensis comb. nov.
Aliithalassotalea hakodatensis [ha.ko.da.ten’sis. N.L. fem. adj. hakodatensis, from Hakodate, referring to the isolation site of the strain]
Basonym: Thalassotalea hakodatensis Yamano et al., 2023
The description is identical to that of Yamano et al. (2023).
The type strain PTE2T (=JCM 34608T = KCTC 82592T) was isolated from a pentactula larvae of Apostichopus japonicus reared in a laboratory aquarium at Hokkaido University, Hokkaido, Japan. The GenBank/EMBL/DDBJ accession number for 16S rRNA gene sequence and genome sequence of strain PTE2T is LC757706 and AP027365, respectively.
5.16 Description of Aliithalassotalea sediminis comb. nov.
Aliithalassotalea sediminis [se.di’mi.nis. L. gen. n. sediminis of sediment]
Basonym: Thalassotalea sediminis Xu et al., 2017
The description is identical to that of Xu et al. (2016).
The type strain N211T (=KCTC 42588T = MCCC 1H00116T) was isolated from marine sediment collected off the coast of Weihai, China. The GenBank/EMBL/DDBJ accession number for 16S rRNA gene sequence and genome sequence of strain N211T is LC757706 and AP027361, respectively.
5.17 Description of Parathalassotalea gen. nov.
Parathalassotalea [Pa.ra.tha.las.so.ta’le.a. Gr. prep. para, next to; N.L. fem. n. Thalassotalea, a genus name meaning a rod from the sea; N.L. fem. n. Parathalassotalea, a new genus near the genus Thalassotalea]
Cells are Gram-stain-negative, facultatively anaerobic, rod-shaped, and motile. Catalase- and oxidase-positive. The predominant ubiquinone is Q-8. The major fatty acids are C17:1 ω8c and summed feature 3 (C16:1 ω7c and/or C16:1 ω6c). The major polar lipids are phosphatidylglycerol and phosphatidylethanolamine. The DNA G + C content of type species is 39.4%. The type species is Parathalassotalea marina. A member of the family Colwelliaceae, the class Gammaproteobacteria, according to 16S rRNA gene and whole genome sequence analysis.
5.18 Description of Parathalassotalea marina comb. nov.
Parathalassotalea marina [se.di’mi.nis. L. gen. n. sediminis of sediment]
Basonym: Thalassotalea marina Hou et al., 2015
The description is identical to that of Hou et al. (2015).
The type strain QBLM2T (=CGMCC 1.12814T = KCTC 42731T) was isolated from the rearing water of a marine recirculating aquaculture system for yellow grouper (Epinephelus awoara) in Tianjin, China. The GenBank/EMBL/DDBJ accession number for the 16S rRNA gene sequence and genome sequence of strain QBLM2T are KT153611 and BNCK00000000, respectively.
5.19 Emended description of the genus Thalassotalea Zhang et al., 2014
Thalassotalea [Tha.las.so.ta′le.a. Gr. n. thalasso the sea; L. fem. n. talea a rod; N.L. fem. n. Thalassotalea a rod from the sea]
This emended description is based on the original description by Zhang et al. (2014), with the following additions: Cells are Gram-stain-negative, aerobic or strictly aerobic, chemo-organotrophic rod-shaped, and motile. Catalase- and oxidase-positive. Nitrate reduction is positive. Some members require Na+ for growth. The predominant ubiquinone is Q-8. The major fatty acids are C17:1 ω8c and summed feature 3 (C16:1 ω7c and/or C16:1 ω6c). The major polar lipids are phosphatidylglycerol and phosphatidylethanolamine. The DNA G + C content is 36.3% to 37.5%. The type species is Thalassotalea piscium. A member of the family Colwelliaceae, the class Gammaproteobacteria, according to 16S rRNA gene and whole genome sequence analysis.
5.20 Emended description of the genus Thalassomonas Macián et al., 2001
Thalassomonas [Tha.las’so.mo.nas. Gr. n. Thalasso the sea; Gr. n. monas a unit; N.L. n. Thalassomonas a monad from the sea]
This emended description is based on the original description by Macián et al. (2001), with the following additions: Cells are Gram-stain-negative, aerobic or strictly aerobic, rod-shaped, and motile. Catalase- and oxidase-positive. some members require seawater components such as Mg2+ for growth in addition to the NaCl and heterotrophic. The predominant ubiquinone is Q-8. The major fatty acids are C16:0 and C17:1 ω8c. The DNA G + C content is 47.3% to 48.9%. The type species is Thalassomonas viridans. A member of the family Colwelliaceae, the class Gammaproteobacteria, according to 16S rRNA gene and whole genome sequence analysis.
5.21 Description of Parathalassomonas gen. nov.
Parathalassomonas [Pa.ra.tha.las.so.mo.nas. Gr. prep. para, next to; N.L. fem. n. Thalassomonas, a genus name meaning a rod from the sea; N.L. fem. n. Parathalassomonas; a genus adjacent to the genus Thalassomonas]
Cells are Gram-stain-negative, aerobic, rod-shaped, and motile. Catalase- and oxidase-positive. Nitrate reduction is negative. The predominant ubiquinone is Q-8. The major fatty acids are C16:0 and summed feature 3 (C16:1 ω7c and/or C16:1 ω6c). The major polar lipids are phosphatidylethanolamine, phosphatidylglycerol, four unidentified aminolipids, and four unidentified lipids. The DNA G + C content of type species is 39.6%. The type species is Petrothalassotalea aciditolerans. A member of the family Colwelliaceae, the class Gammaproteobacteria, according to 16S rRNA gene and whole genome sequence analysis.
5.22 Description of Parathalassomonas gelatinilyticus sp. nov.
Parathalassomonas gelatinilyticus [ge.la.ti.ni.ly’ti.cus. N.L. neut. n. gelatinum, gelatin; N.L. masc. adj. lyticus (from Gr. masc. adj. lytikos), able to loosen, able to dissolve; N.L. masc. adj. gelatinilyticus, gelatin-dissolving]
In addition to the genus description, the following are added: Colonies grown on MA are smooth, convex, and circular, and the cell size was 1.1–3.3 µm × 0.4–1.0 µm. Growth is observed at 10–30°C (optimal temperature 25°C), pH 5–8 (optimal pH 7), and 0.5–2.0% (optimum 1.0%) NaCl. In the API 50CH and 20NE system, acid is produced from the following substrates; Erythritol, D-Ribose, D-Adonitol, Methyl-β D-Xylopyranoside, D-Glucose, D-Mannose, Inositol, D-Mannitol, N-Acetylglucosamine, Amygdalin, Arbutin, Esculin ferric citrate, Salicin, Cellobiose, Maltose, Lactose, D-Melibiose, D-Saccharose, D-Tagatose, and Gelatin. Acid from D-Fructose, D-Sorbitol, D-Trehalose, Inulin, D-Melezitose, Xylitol, and Arabitol is weakly positive. Glycerol, Arabinose, Xylose, Galactose, L-Sorbose, L-Rhamnose, Dulcitol, Methyl-α D-Mannopyranoside, Methyl-α D-Glucopyranoside, Starch, Glycogen, Gentiobiose, D-Turanose, D-Lyxose, Fucose, Urea, Esculin, Capric acid, Adipic acid, Malic acid, and trisodium citrate are negative for acid production. Alkaline phosphatase and Leucine arylamidase enzyme activities are positive, while Esterase, Lipase, Valine arylamidase, Naphthol-AS-BI-phosphohydrolase, α-glucosidase, and N-acetyl-β-glucosaminidase are weakly positive. Cystine arylamidase, Trypsin, α-chymotrypsin, α-galactosidase, β- glucuronidase, β-glucosidase, β-glucosidase, α-mannosidase, and α-fucosidase activity is negative
The type strain is MEBiC06471T (=KCCM 43507T = MCCC 1K09163T), isolated from tidal sediment collected at Taean-gun, Republic of Korea. The GenBank/DDBJ/EMBL accession number for the 16S rRNA gene is OR958775 and that of the genome is JBFONV000000000.
5.23 Description of Algithalassotalea gen. nov.
Algithalassotalea [Al.gi.tha.las.so.ta’le.a. L. fem. n. alga, a seaweed; N.L. fem. n. Thalassotalea, a genus name meaning a rod from the sea; N.L. fem. n. Algithalassotalea; a relative of Thalassotalea from an alga]
Cells are Gram-stain-negative, facultatively anaerobic, rod-shaped, and motile by a single polar flagellum and gliding. Catalase- and oxidase-positive. Nitrate reduction is positive. The predominant ubiquinone is Q-8. The major fatty acids are summed features 3 (C16:1 ω7c and/or C16:1 ω6c), C16:0, and summed features 8 (C18:1 ω7c and/or C18:1 ω6c). The major polar lipids are phosphatidylglycerol and phosphatidylethanolamine. The DNA G + C content of type species is 39.1%. The type species is Algithalassotalea algicola. A member of the family Colwelliaceae, the class Gammaproteobacteria, according to 16S rRNA gene and whole genome sequence analysis.
5.24 Description of Algithalassotalea algicola comb. nov.
Algithalassotalea algicola [al.gi′co.la. L. n. alga -ae an alga (seaweed, dinoflagellate etc.); L. suff. -cola from L. n. incola an inhabitant; N.L. fem. n. algicola an inhabitant of algae]
Basonym: Thalassotalea algicola Lian et al., 2022
The description is identical to that of Lian et al. (2021).
The type strain M1531T (=MCCC 1H00400T = KCTC 72865T) was isolated from a red alga at coastal water in Weihai, China. The GenBank/EMBL/DDBJ accession number for the 16S rRNA gene sequence and genome sequence of strain M1531T are MN822801 and JABBXH000000000, respectively.
5.25 Description of Vitithalassotalea gen. nov.
Vitithalassotalea [Vi.ti.tha.las.so.ta’le.a. L. fem. n. vita, life; N.L. fem. n. Thalassotalea, a genus name meaning a rod from the sea; N.L. fem. n. Vitithalassotalea; a Thalassotalea from marine live]
Cells are Gram-stain-negative, aerobic, rod-shaped, and motile by means of a single polar flagellum. Catalase- and oxidase-positive. Nitrate reduction is positive. The predominant ubiquinone is Q-8. The major fatty acids are summed feature 3 (C16:1 ω7c and/or C16:1 ω6c), C18:1 ω7c, C16:0, and C17:1 ω8c. The major polar lipids are phosphatidylethanolamine, phosphatidylglycerol, one uncharacterized aminophospholipid, five uncharacterized phospholipids, three uncharacterized aminolipids, and two uncharacterized lipids. The DNA G + C content of type species is 44.3%. The type species is Vitithalassotalea euphylliae. A member of the family Colwelliaceae, the class Gammaproteobacteria, according to 16S rRNA gene and whole genome sequence analysis.
5.26 Description of Vitithalassotalea euphylliae comb. nov.
Vitithalassotalea euphylliae [eu.phyl′li.ae. N.L. gen. n. euphylliae of Euphyllia, isolated from a coral belonging to the genus Euphyllia]
Basonym: Thalassotalea euphylliae Sheu et al., 2016
The description is identical to that of Sheu et al. (2016).
The type strain Eup-16T (=BCRC 80910T = LMG 29001T = KCTC 42743T) was isolated from the torch coral Euphyllia glabrescens, collected off southern Taiwan. The GenBank/EMBL/DDBJ accession number for the 16S rRNA gene sequence and genome sequence of strain Eup-16T are LN849949 and QUOU00000000, respectively.
5.27 Description of Aminithalassotalea gen. nov.
Aminithalassotalea [A.mi.ni.tha.las.so.ta’le.a. N.L. fem. n. amina, amine; N.L. fem. n. Thalassotalea, a genus name meaning a rod from the sea; N.L. fem. n. Aminithalassotalea; a Thalassotalea loving amino acids]
Cells are Gram-stain-negative, strictly aerobic, straight or slightly curved rods, non-flagellated and non-gliding. Oxidase is positive, but catalase is negative. Nitrate reduction is positive. The predominant ubiquinone is Q-8. The major fatty acids are summed feature 3 (C16:1 ω7c and/or C16:1 ω6c) and C16:0. The major polar lipids are phosphatidylethanolamine and phosphatidylglycerol. The DNA G + C content of type species is 40.2%. The type species is Aminithalassotalea atypica. A member of the family Colwelliaceae, the class Gammaproteobacteria, according to 16S rRNA gene and whole genome sequence analysis.
5.28 Description of Aminithalassotalea atypica comb. nov.
Aminithalassotalea atypica [a.ty′pi.ca. Gr. pref. a- not; L. adj. typicus typical; N.L. fem. adj. atypica atypical]
Basonym: Thalassotalea atypica Wang et al., 2018
The description is identical to that of Wang et al. (2018).
The type strain RZG4-3-1T (=JCM 31894T = KCTC 52745T = MCCC 1K03276T) was isolated from the surface seawater of the Yellow Sea in China (35° 31’ 01” N, 119° 37’ 30” E). The GenBank/EMBL/DDBJ accession number for the 16S rRNA gene sequence and genome sequence of strain RZG4-3-1T is KY014437 and AP027364, respectively.
5.29 Description of Variithalassotalea gen. nov.
Variithalassotalea [Va.ri.i.tha.las.so.ta’le.a. L. masc. adj. varius, varying; N.L. fem. n. Thalassotalea, a genus name meaning a rod from the sea; N.L. fem. n. Variithalassotalea; a Thalassotalea from various origin]
Cells are Gram-stain-negative, aerobic, rod-shaped, and motile by a single polar flagellum. Catalase is positive. The major fatty acids are summed feature 3 (C16:1 ω7c and/or C16:1 ω6c), C14:0, and C17:1 ω8c. The DNA G + C content is 39.7% to 40.4%. The type species is Variithalassotalea loyana. A member of the family Colwelliaceae, the class Gammaproteobacteria, according to 16S rRNA gene and whole genome sequence analysis.
5.30 Description of Variithalassotalea loyana comb. nov.
Variithalassotalea loyana [loy′an.a. N.L. fem. adj. loyana named in honor of the Israeli biologist Y. Loya]
Basonym: Thalassomonas loyana Thompson et al., 2006
The description is identical to that of Thompson et al. (2006).
The type strain CBMAI 722T (=LMG 22536T = CBMAI 722T) was isolated from diseased coral in Eilat, Israel. The GenBank/EMBL/DDBJ accession number for 16S rRNA gene sequence and genome sequence of strain CBMAI 722T is AY643537 and BSSV00000000, respectively.
5.31 Description of Variithalassotalea eurytherma comb. nov.
Variithalassotalea eurytherma [eu.ry.ther′ma. Gr. adj. eurys wide; Gr. adj. thermos hot; N.L. fem. adj. eurytherma able to tolerate a wide range of temperatures]
Basonym: Thalassomonas eurytherma Sun et al., 2014
The description is identical to that of Sun et al. (2014).
The type strain Za6a-12T (= CGMCC 1.12116 T = JCM 18483T) was isolated from the Zhoushan Islands in the East China Sea. The GenBank/EMBL/DDBJ accession number for 16S rRNA gene sequence and genome sequence of strain Za6a-12T is JQ288724 and BSSU00000000, respectively.
5.32 Description of Saccharothalassotalea gen. nov.
Saccharothalassotalea [Sac.cha.ro.tha.las.so.ta’le.a. Gr. neut. n. sakchar, sugar; N.L. fem. n. Thalassotalea, a genus name meaning a rod from the sea; N.L. fem. n. Saccharothalassotalea; a Thalassotalea loving sugar]
Cells are Gram-stain-negative, aerobic, straight or curved rods, and non-motile. Catalase- and oxidase-positive. Nitrate is reduced to nitrite. The major fatty acids are C16:0, C17:1 ω8c, C17:0, C15:0 iso 2-OH/C16:1 ω7c, and C13:0. The DNA G + C content of type species is 41.9%. The type species is Saccharothalassotalea agarivorans. A member of the family Colwelliaceae, the class Gammaproteobacteria, according to 16S rRNA gene and whole genome sequence analysis.
5.33 Description of Saccharothalassotalea agarivorans comb. nov.
Saccharothalassotalea agarivorans [a.ga.ri.vo′rans. N.L. n. agarum agar; L. part. adj. vorans devouring, destroying; N.L. part. adj. agarivorans agar-devouring]
Basonym: Thalassomonas agarivorans Jean et al., 2006
The description is identical to that of Jean et al. (2006).
The type strain TMA1T (= BCRC 17492T = JCM 13379T = DSM 19706T) was isolated from shallow seawater of An-Ping Harbour, Tainan, Taiwan. The GenBank/EMBL/DDBJ accession number for 16S rRNA gene sequence and genome sequence of strain TMA1T is DQ212914 and AP027363, respectively.
5.34 Description of Psychrothalassomonas gen. nov.
Psychrothalassomonas [Psy.chro.tha.las.so.mo.nas. Gr. masc. adj. psychros, cold; N.L. fem. n. Thalassomonas, a genus name meaning a rod from the sea; N.L. fem. n. Psychrothalassomonas; a Thalassomonas living in cold water]
Cells are Gram-stain-negative, aerobic, rod-shaped, and non-motile. Oxidase is positive, but catalase is negative. The strains are psychrophilic or psychrotrophic. Nitrate reduction is variable. The predominant quinone is Q-8, but some members additionally include MK-7. The major fatty acids are summed feature 3 (C16:1 ω7c and/or C16:1 ω6c) and C16:0. The major polar lipids are phosphatidylethanolamine and phosphatidylglycerol. The DNA G + C content is 38.1% to 39.0%. The type species is Psychrothalassomonas psychrophila. A member of the family Colwelliaceae, the class Gammaproteobacteria, according to 16S rRNA gene and whole genome sequence analysis.
5.35 Description of Psychrothalassomonas nanhaiensis comb. nov.
Psychrothalassomonas nanhaiensis [nan.hai.en’sis. N.L. fem. adj. nanhaiensis, pertaining to NanHai, the Chinese name for the South China Sea, where the type strain was isolated]
Basonym: Thalassotalea nanhaiensis Li et al., 2024
The description is identical to that of Li et al. (2024).
The type strain SQ345T (= MCCC 1K04232T = JCM 33808T) was isolated from deep-sea sediment of the South China Sea. The GenBank/EMBL/DDBJ accession number for the 16S rRNA gene sequence and genome sequence of strain SQ345T is MK801284 and CP134146, respectively.
5.36 Description of Psychrothalassomonas psychrophila comb. nov.
Psychrothalassomonas psychrophile [psy.chro’phi.la. Gr. masc. adj. psychros cold; Gr. masc. adj. philos loving; N.L. fem. adj. psychrophila, referring to loving low temperatures]
Basonym: Thalassotalea psychrophila Li et al., 2024
The description is identical to that of Li et al. (2024).
The type strain SQ149T (= MCCC 1K04231T = JCM 33807T) was isolated from deep-sea sediment of the South China Sea. The GenBank/EMBL/DDBJ accession number for 16S rRNA gene sequence and genome sequence of strain SQ149T is MK801283 and CP134145, respectively.
5.37 Description of Psychrothalassomonas fonticola comb. nov.
Psychrothalassomonas fonticola [fon.ti’co.la. L. fem. n. fons, spring; L. masc./fem. suff. -cola, inhabitant, dweller; N.L. fem. n. fonticola, an inhabitant of a seep]
Basonym: Thalassotalea fonticola Li et al., 2024
The description is identical to that of Li et al. (2024).
The type strain S1-1T (= MCCC 1K06879T = JCM 34824T) was isolated from deep-sea sediment of the South China Sea. The GenBank/EMBL/DDBJ accession number for 16S rRNA gene sequence and genome sequence of strain S1-1T is OP303371 and CP136600, respectively.
5.38 Description of Arcticithalassomonas gen. nov.
Arcticithalassomonas [Arc.ti.ci.tha.las.so.mo.nas. L. masc. adj. arcticus, northern, arctic; N.L. fem. n. Thalassomonas, a genus name meaning a rod from the sea; N.L. fem. n. Arcticithalassomonas; a Thalassomonas isolated from Arctic Sea]
Cells are Gram-stain-negative, aerobic, rod-shaped and motile. Catalase- and oxidase-positive. Nitrate reduction is positive. Some members require Mg2+ and K+ ions in addition to the NaCl for growth. The predominant ubiquinone is MK-6. The major fatty acids are C16:0 and summed feature 3 (C16:1 ω7c and/or C16:1 ω6c). The major polar lipids are phosphatidylethanolamine and phosphatidylglycerol. The DNA G + C content of type species is 39.3%. The type species is Arcticithalassomonas sediminis. A member of the family Colwelliaceae, the class Gammaproteobacteria, according to 16S rRNA gene and whole genome sequence analysis.
5.39 Description of Arcticithalassomonas sediminis sp. nov.
Arcticithalssomonas sediminis [se.di.mi’ nis. L. gen. n. sediminis of sediment, the source of the type strain]
In addition to the genus description, followings are added; Colonies grown on MA are circular, opaque, convex with entire margin and the cell size was 0.5–0.7 µm × 1.1–2.5 µm. Growth is observed at 0–16°C (optimal temperature 9°C), pH 6–10 (optimal pH 7–8) and 0.0–4.0% (optimum 2.0–3.0%) NaCl. Require Mg2+ and K+ ions in addition to the NaCl for growth. β-galactosidase, and β-Glucosidase activities are present but arginine dihydrolase, protease and Urease activities are not. Indole is produced from tryptophan and nitrate reduction is negative. Alkaline phosphatase, esterase, esterase lipase, leucine arylamidase, valine arylamidase, trypsin, α-Chymotrypsin, acid phosphatase, and naphthol-AS-BI-phosphohydrolase activities and weak cystine arylamidase activities are present. Substrates utilized are include α-Cyclodextrin, dextrin, glycogen, N-acetyl-D-glucosamine, cellobiose, D-fructose, gentiobiose, α-D-glucose, α-D-lactose, maltose, D-mannitol, sucrose, D-trehalose, cis-aconitic acid, citric acid, D-glucuronic acid, D,L-lactic acid, bromosuccinic acid, L-alanine, L-alanyl-glycine, L-asparagine, L-aspartic acid, L-glutamic acid, glycyl-L-aspartic acid, glycyl-L-glutamic acid, L-proline, L-serine, L-threonine, inosine, uridine, thymidine, glycerol, D,L-α-glycerol phosphate, glucose-1-phosphate, and glucose-6-phosphate. D-galactose, β-methyl-D-glucoside, α-ketobutyric acid, α-ketoglutaric acid, and alaninamide is weakly utilize. In the API 50CH and 20NE system, acid produced from the following substrates; glycerol, glucose, fructose, mannose, esculin, cellobiose, maltose, melibiose, sucrose, trehalose, starch, and glycogen, and weakly for ribose, galactose, mannitol, and salicin. MEBiC02087T is identical to BS250T.
The type strain is MEBiC02087T (=KCCM 42335T = JCM 14072T), isolated from 680 m depth sediment collected at Barents Sea, Russia. The GenBank/DDBJ/EMBL accession number for the 16S rRNA gene is PQ119528 and that of the genome is JBFONS000000000.
5.40 Description of Molluscithalassomonas gen. nov.
Molluscithalassomonas [Mol.lus.ci.tha.las.so.mo.nas. L. pl. neut. n. Mollusca, an animal phylum; N.L. fem. n. Thalassomonas, a genus name meaning a rod from the sea; N.L. fem. n. Molluscithalassomonas; a Thalassomonas from a mollusks]
Cells are Gram-stain-negative, aerobic, rod-shaped and non-motile. Catalase- and oxidase-positive. Nitrate reduction is positive. Does not grow under microaerophilic or anaerobic conditions. The predominant ubiquinone is Q-8. The major fatty acids are C16:0, C17:1 ω8c, and summed feature 3 (C16:1 ω7c and/or C16:1 ω6c). The major polar lipids are phosphatidylethanolamine and phosphatidylglycerol. The DNA G + C content of type species is 38.8%. The type species is Molluscithalassomonas crassostreae. A member of the family Colwelliaceae, the class Gammaproteobacteria, according to 16S rRNA gene and whole genome sequence analysis.
5.41 Description of Molluscithalassomonas crassostreae comb. nov.
Molluscithalassomonas crassostreae [crass.os′tre.ae. N.L. gen. n. crassostreae of Crassostrea gigas, named after the generic name of the oyster from which the type strain was isolated]
Basonym: Thalassotalea crassostreae Choi et al., 2017
The description is identical to that of Choi et al. (2017).
The type strain LPB0090T (= KACC 18695T = JCM 31189T) was isolated from an oyster species from Yeongheung Island, Korea. The GenBank/EMBL/DDBJ accession number for the 16S rRNA gene sequence and genome sequence of strain LPB0090T is KU160140 and CP017689, respectively.
5.42 Description of Aquithalassomonas gen. nov.
Aquithalassomonas [A.qui.tha.las.so.mo.nas. L. fem. n. aqua, water; N.L. fem. n. Thalassomonas, a genus name meaning a rod from the sea; N.L. fem. n. Aquithalassomonas; a Thalassomonas live in water]
Cells are Gram-stain-negative, aerobic, rod-shaped or ovoid, non-motile, and non-flagellated. Catalase- and oxidase-positive. Nitrate reduction is positive. Some members require Mg2+ ions for growth. The predominant ubiquinone is Q-8. The major fatty acids are C16:0, C17:1 ω8c, and summed feature 3 (C16:1 ω7c and/or C16:1 ω6c). The major polar lipids are phosphatidylethanolamine and phosphatidylglycerol. The DNA G + C content of type species is 44.4%. The type species is Aquithalassomonas ponticola. A member of the family Colwelliaceae, the class Gammaproteobacteria, according to 16S rRNA gene and whole genome sequence analysis.
5.43 Description of Aquithalassomonas ponticola comb. nov.
Aquithalassomonas ponticola [pon.ti′co.la. L. n. pontus the sea; L. suff. -cola (from L. n. incola) inhabitant, dweller; N.L. fem. n. ponticola living in the sea]
Basonym: Thalassotalea ponticola Park et al., 2014
The description is identical to that of Park et al. (2014).
The type strain GJSW-36T (= KCTC 42155T = CECT 8656T) was isolated from seawater collected from Geoje Island in the South Sea, South Korea. The GenBank/EMBL/DDBJ accession number for the 16S rRNA gene sequence and genome sequence of strain GJSW-36T is KU160140 and CP017689, respectively.
5.44 Description of Solithalassomonas gen. nov.
Solithalassomonas [So.li.tha.las.so.mo.nas. L. neut. n. solum, soil; N.L. fem. n. Thalassomonas, a genus name meaning a rod from the sea; N.L. fem. n. Solithalassomonas; a Thalassomonas from a soil]
Cells are Gram-stain-negative, strictly aerobic, rod-shaped or ovoid, non-motile or motile by single polar flagellum. Oxidase is positive, but catalase varies depending on the strain. Nitrate reduction is positive. The predominant ubiquinone is Q-8. The main fatty acid in common is C16:0. The major polar lipids are phosphatidylglycerol and phosphatidylethanolamine. The DNA G + C content is 43.9% to 45.9%. The type species is Aquithalassomonas ponticola. A member of the family Colwelliaceae, the class Gammaproteobacteria, according to 16S rRNA gene and whole genome sequence analysis.
5.45 Description of Solithalassomonas mangrovi comb. nov.
Solithalassomonas mangrovi [man.gro′vi. N.L. gen. n. mangrovi of or belonging to a mangrove wetland]
Basonym: Thalassotalea mangrovi Zheng et al., 2019
The description is identical to that of Zheng et al. (2019).
The type strain ZS-4T (= KCTC 72399T = MCCC 1K03630T) was isolated from marine mangrove sediment collected at Beilun Estuary National Nature Reserve, Guangxi Province, PR China. The GenBank/EMBL/DDBJ accession number for the 16S rRNA gene sequence and genome sequence of strain ZS-4T are MK911729 and SWDB00000000, respectively.
5.46 Description of Solithalassomonas litorea comb. nov.
Solithalassomonas litorea [li.to′re.a. L. fem. adj. litorea of seashore]
Basonym: Thalassotalea litorea Kang et al., 2017
The description is identical to that of Kang et al. (2017).
The type strain HMF4135T (= KCTC 52154T = NBRC 112672T) was isolated from seashore sand in Jeju, Republic of Korea. The GenBank/EMBL/DDBJ accession number for the 16S rRNA gene sequence and genome sequence of strain HMF4135T are KU853009 and VCBC00000000, respectively.
Data availability statement
The datasets presented in this study can be found in online repositories. The names of the repository/repositories and accession number(s) can be found in the article/Supplementary Material.
Author contributions
ML: Data curation, Formal Analysis, Investigation, Methodology, Software, Visualization, Writing – original draft. S-HY: Formal Analysis, Writing – review & editing. YK: Formal Analysis, Resources, Validation, Writing – review & editing. YP: Formal Analysis, Methodology, Writing – review & editing. M-JP: Conceptualization, Data curation, Methodology, Software, Supervision, Validation, Visualization, Writing – review & editing. KK: Conceptualization, Data curation, Funding acquisition, Project administration, Supervision, Validation, Writing – review & editing.
Funding
The author(s) declare that financial support was received for the research and/or publication of this article. This work was supported by the management of Marine Fishery Bio-resources Center (2024) funded by the National Marine Biodiversity Institute of Korea (MABIK), the High Seas bioresources program of the Korea Institute of Marine Science & Technology Promotion (KIMST) funded by the Ministry of Oceans and Fisheries (KIMST-20210646), and the KIOST in-house program (PEA0212).
Acknowledgments
We are thankful to the crews and cruise members of the High Sea Cruise via R/V Isabu and special thanks to the Late Dr. KyungHo Jung and Dr. Jae Kyu Lim for their contribution to sampling at Barents Sea, Russia.
Conflict of interest
The authors declare that the research was conducted in the absence of any commercial or financial relationships that could be construed as a potential conflict of interest.
Generative AI statement
The author(s) declare that no Generative AI was used in the creation of this manuscript.
Publisher’s note
All claims expressed in this article are solely those of the authors and do not necessarily represent those of their affiliated organizations, or those of the publisher, the editors and the reviewers. Any product that may be evaluated in this article, or claim that may be made by its manufacturer, is not guaranteed or endorsed by the publisher.
Supplementary material
The Supplementary Material for this article can be found online at: https://www.frontiersin.org/articles/10.3389/fevo.2025.1532186/full#supplementary-material
References
Aramaki T., Blanc-Mathieu R., Endo H., Ohkubo K., Kanehisa M., Goto S., et al. (2020). KofamKOALA: KEGG Ortholog assignment based on profile HMM and adaptive score threshold. Bioinformatics 36 (7), 2251–2252. doi: 10.1093/bioinformatics/btz859
Asnicar F., Thomas A. M., Beghini F., Mengoni C., Manara S., Manghi P., et al. (2020). Precise phylogenetic analysis of microbial isolates and genomes from metagenomes using PhyloPhlAn 3.0. Nat. Commun. 11, 2500. doi: 10.1038/s41467-020-16366-7
Bankevich A., Nurk S., Antipov D., Gurevich A. A., Dvorkin M., Kulikov A. S., et al. (2012). SPAdes: a new genome assembly algorithm and its applications to single-cell sequencing. J. Comput. Biol. 19, 455–477. doi: 10.1089/cmb.2012.0021
Bolger A. M., Lohse M., Usadel B. (2014). Trimmomatic: a flexible trimmer for Illumina sequence data. Bioinformatics 30, 2114–2120. doi: 10.1093/bioinformatics/btu170
Bowman J. P. (2007). Bioactive compound synthetic capacity and ecological significance of marine bacterial genus Pseudoalteromonas. Mar. Drugs 5, 220–241. doi: 10.3390/md504220
Bowman J. P. (2014).The family colwelliaceae, in The prokaryotes Eds. Rosenberg E., DeLong E. F., Lory S., Stackebrandt E., Thompson F. (Springer, Berlin, Heidelberg), 179–195. doi: 10.1007/978-3-642-38922-1_230
Bowman J. P., Gosink J. J., McCammon S. A., Lewis T. E., Nichols D. S., Nichols P. D., et al. (1998). Colwellia demingiae sp. nov., Colwellia hornerae sp. nov., Colwellia rossensis sp. nov. and Colwellia psychrotropica sp. nov.: psychrophilic Antarctic species with the ability to synthesize docosahexaenoic acid (22:6ω3). Int. J. Syst. Evol. Microbiol. 48, 1171–1180. doi: 10.1099/00207713-48-4-1171
Buck J. D. (1982). Nonstaining (KOH) method for determination of gram reactions of marine bacteria. Appl. Environ. Microbiol. 44, 992–993. doi: 10.1128/aem.44.4.992-993.1982
Carpenter C. M., Frank D. N., Williamson K., Arbet J., Wagner B. D., Kechris K., et al. (2021). tidyMicro: a pipeline for microbiome data analysis and visualization using the tidyverse in R. BMC Bioinf. 22, 41. doi: 10.1186/s12859-021-03967-2
Caudill M. T., Brayton K. A. (2022). The use and limitations of the 16S rRNA sequence for species classification of anaplasma samples. Microorganisms. 10 (3), 605. doi: 10.3390/microorganisms10030605
Choi E. J., Kwon H. C., Koh H. Y., Kim Y. S., Yang H. O. (2010). Colwellia asteriadis sp. nov., a marine bacterium isolated from the starfish Asterias amurensis. Int. J. Syst. Evol. Microbiol. 60, 1952–1957. doi: 10.1099/ijs.0.016055-0
Choi S., Kim E., Shin S. K., Yi H. (2017). Thalassotalea crassostreae sp. nov., isolated from Pacific oyster. Int. J. Syst. Evol. Microbiol. 67, 2195–2198. doi: 10.1099/ijsem.0.001923
Christiansen L., Bech P. K., Schultz-Johansen M., Martens H. J., Stougaard P. (2018). Colwellia echini sp. nov., an agar- and carrageenan-solubilizing bacterium isolated from sea urchin. Int. J. Syst. Evol. Microbiol. 68, 687–691. doi: 10.1099/ijsem.0.002568
Collins M. D., Goodfellow M., Minnikin D. E. (1979). Isoprenoid quinones in the classification of coryneform and related bacteria. Microbiology 110, 127–136. doi: 10.1099/00221287-110-1-127
D’Aoust J. Y., Kushner D. J. (1972). Vibrio psychroerythrus sp. n.: classification of the psychrophilic marine bacterium, NRC 1004. J. Bacteriol 111, 340–342. doi: 10.1128/jb.111.2.340-342.1972
Deming J. W., Somers L. K., Straube W. L., Swartz D. G., Macdonell M. T. (1988). Isolation of an obligately barophilic bacterium and description of a new genus, Colwellia gen. nov. Syst. Appl. Microbiol. 10, 152–160. doi: 10.1016/S0723-2020(88)80030-4
Duplantis B. N., Osusky M., Schmerk C. L., Ross D. R., Bosio C. M., Nano F. E. (2010). Essential genes from Arctic bacteria used to construct stabl e, temperature-sensitive bacterial vaccines. Proc. Natl. Acad. Sci. U.S.A. 107, 13456–13460. doi: 10.1073/pnas.1004119107
Felsenstein J. (1981). Evolutionary trees from DNA sequences: a maximum likelihood approach. J. Mol. Evol. 17, 368–376. doi: 10.1007/bf01734359
García-Alegría A. M., Anduro-Corona I., Pérez-Martínez C. J., Guadalupe Corella-Madueño M. A., Rascón-Durán M. L., Astiazaran-Garcia H. (2020). Quantification of DNA through the nanoDrop spectrophotometer: methodological validation using standard reference material and sprague dawley rat and human DNA. Int. J. Anal. Chem. 2020, 8896738. doi: 10.1155/2020/8896738
Gauthier G., Gauthier M., Christen R. (1995). Phylogenetic analysis of the genera Alteromonas, Shewanella, and Moritella using genes coding for small-subunit rRNA sequences and division of the genus Alteromonas into two genera, Alteromonas (emended) and Pseudoalteromonas gen. nov., and proposal of twelve new species combinations. Int. J. Syst. Bacteriol 45, 755–761. doi: 10.1099/00207713-45-4-755
Guskov A., Eshaghi S. (2012). The mechanisms of Mg2+ and Co2+ transport by the CorA family of divalent cation transporters. Curr. Top. Membr. 69, 393–414. doi: 10.1016/B978-0-12-394390-3.00014-8
Hall T. A. (1999). BioEdit: a user-friendly biological sequence alignment editor and analysis program for Windows 95/98/NT. In Nucleic Acids symposium Ser. 41, 95–98.
Hashimoto M., Orikasa Y., Hayashi H., Watanabe K., Yoshida K., Okuyama H. (2015). Occurrence of trans monounsaturated and polyunsaturated fatty acids in Colwellia psychrerythraea strain 34H. J. Basic Microbiol. 55, 838–845. doi: 10.1002/jobm.201400815
Hosoya S., Adachi K., Kasai H. (2009). Thalassomonas actiniarum sp. nov. and Thalassomonas haliotis sp. nov., isolated from marine animals. Int. J. Syst. Evol. Microbiol. 59, 686–690. doi: 10.1099/ijs.0.000539-0
Hou T. T., Liu Y., Zhong Z. P., Liu H. C., Liu Z. P. (2015). Thalassotalea marina sp. nov., isolated from a marine recirculating aquaculture system, reclassification of Thalassomonas eurytherma as Thalassotalea eurytherma comb. nov. and emended description of the genus Thalassotalea. Int. J. Syst. Evol. Microbiol. 65, 4710–4715. doi: 10.1099/ijsem.0.000637
Ivanova E. P., Flavier S., Christen R. (2004). Phylogenetic relationships among marine Alteromonas-like Proteobacteria: emended description of the family Alteromonadaceae and proposal of Pseudoalteromonadaceae fam. nov., Colwelliaceae fam. nov., Shewanellaceae fam. nov., Moritellaceae fam. nov., Ferrimonadaceae fam. nov., Idiomarinaceae fam. nov. and Psychromonadaceae fam. nov. Int. J. Syst. Evol. Microbiol. 5), 1773–1788. doi: 10.1099/ijs.0.02997-0
Ivanova E. P., Mikhailov V. V. (2001). A new family of Alteromonadaceae fam. nov., including the marine Proteobacteria species Alteromonas, Pseudoalteromonas, Idiomarina i Colwellia. Mikrobiologiia 70, 15–23. (Novoe semeistvo Alteromonadaceae fam. nov., ob’’ediniaiushchee morskie proteobakterii rodov Alteromonas, Pseudoalteromonas, Idiomarina i Colwellia.). doi: 10.1023/A:1004876301036
Jean W. D., Shieh W. Y., Liu T. Y. (2006). Thalassomonas agarivorans sp. nov., a marine agarolytic bacterium isolated from shallow coastal water of An-Ping Harbour, Taiwan, and emended description of the genus Thalassomonas. Int. J. Syst. Evol. Microbiol. 56, 1245–1250. doi: 10.1099/ijs.0.64130-0
Jung S. Y., Oh T. K., Yoon J. H. (2006). Colwellia aestuarii sp. nov., isolated from a tidal flat sediment in Korea. Int. J. Syst. Evol. Microbiol. 56, 33–37. doi: 10.1099/ijs.0.63920-0
Kang H., Kim H., Nam I. Y., Joung Y., Jang T. Y., Joh K. (2017). Thalassotalea litorea sp. nov., isolated from seashore sand. Int. J. Syst. Evol. Microbiol. 67, 2268–2273. doi: 10.1099/ijsem.0.001938
Khan A. R., Pervez M. T., Babar M. E., Naveed N., Shoaib M. (2018). A comprehensive study of de novo genome assemblers: current challenges and future prospective. Evol. Bioinform. Online 14, 1176934318758650. doi: 10.1177/1176934318758650
Kim D., Park S., Chun J. (2021). Introducing EzAAI: a pipeline for high throughput calculations of prokaryotic average amino acid identity. J. Microbiol. 59, 476–480. doi: 10.1007/s12275-021-1154-0
Kim Y. O., Park I. S., Park S., Nam B. H., Jung Y. T., Kim D. G., et al. (2017). Colwellia mytili sp. nov., isolated from mussel Mytilus edulis. Int. J. Syst. Evol. Microbiol. 67, 31–36. doi: 10.1099/ijsem.0.001564
Kimura M. (1980). A simple method for estimating evolutionary rates of base substitutions through comparative studies of nucleotide sequences. J. Mol. Evol. 16, 111–120. doi: 10.1007/BF01731581
Konstantinidis K. T., Tiedje J. M. (2005). Genomic insights that advance the species definition for prokaryotes. Proc. Natl. Acad. Sci. U.S.A. 102, 2567–2572. doi: 10.1073/pnas.0409727102
Kristyanto S., Jung J., Kim J. M., Kim K., Lee M. H., Hao L., et al. (2022). Colwellia maritima marinus sp. nov., isolated from seawater Polaribacter. Int. J. Syst. Evol. Microbiol. 72 (11), 1–9. doi: 10.1099/ijsem.0.005620
Kusube M., Kyaw T. S., Tanikawa K., Chastain R. A., Hardy K. M., Cameron J., et al. (2017). Colwellia marinimaniae sp. nov., a hyperpiezophilic species isolated from an amphipod within the Challenger Deep, Mariana Trench. Int. J. Syst. Evol. Microbiol. 67, 824–831. doi: 10.1099/ijsem.0.001671
Leggett R. M., Ramirez-Gonzalez R. H., Clavijo B. J., Waite D., Davey R. P. (2013). Sequencing quality assessment tools to enable data-driven informatics for high throughput genomics. Front. Genet. 4. doi: 10.3389/fgene.2013.00288
Li A. Q., Qi X. Q., Zhang C., Huang X. G., Wen D. Y., Li X. G., et al. (2024). Thalassotalea psychrophila sp. nov., Thalassotalea nanhaiensis sp. nov. and Thalassotalea fonticola sp. nov., three psychrophilic bacteria isolated from deep-sea sediment. Int. J. Syst. Evol. Microbiol. 74 (5), 1–10. doi: 10.1099/ijsem.0.006399
Lian F. B., Jiang S., Ren T. Y., Zhou B. J., Du Z. J. (2021). Thalassotalea algicola sp. nov., an alginate-utilizing bacterium isolated from a red alga. Antonie Van Leeuwenhoek 114, 835–844. doi: 10.1007/s10482-021-01562-2
Liang K. Y., Orata F. D., Boucher Y. F., Case R. J. (2021). Roseobacters in a sea of poly-and paraphyly: whole genome-based taxonomy of the family Rhodobacteraceae and the proposal for the split of the “Roseobacter clade” into a novel family, Roseobacteraceae fam. nov. Front. Microbiol. 12. doi: 10.3389/fmicb.2021.683109
Liu J., Sun Y.-W., Li S.-N., Zhang D.-C. (2017). Thalassotalea profundi sp. nov. isolated from a deep-sea seamount. Int. J. Syst. Evol. Microbiol. 67, 3739–3743. doi: 10.1099/ijsem.0.002180
Liu A., Zhang Y. J., Cheng P., Peng Y. J., Blom J., Xue Q. J. (2020a). Whole genome analysis calls for a taxonomic rearrangement of the genus Colwellia. Antonie Van Leeuwenhoek 113, 919–931. doi: 10.1007/s10482-020-01405-6
Liu A., Zhang Y. J., Xue Q. J., Wang H., Yang Y. Y., Du F., et al. (2020b). Litorilituus lipolyticus sp. nov., isolated from intertidal sand of the Yellow Sea in China, and emended description of Colwellia asteriadis. Antonie Van Leeuwenhoek 113, 449–458. doi: 10.1007/s10482-019-01355-8
Lockwood S., Greening C., Baltar F., Morales S. E. (2022). Global and seasonal variation of marine phosphonate metabolism. ISME J. 16, 2198–2212. doi: 10.1038/s41396-022-01266-z
Luo C., Rodriguez R. L., Konstantinidis K. T. (2014). MyTaxa: an advanced taxonomic classifier for genomic and metagenomic sequences. Nucleic Acids Res. 42 (8), e73. doi: 10.1093/nar/gku169
Macián M. C., Ludwig W., Schleifer K. H., Garay E., Pujalte M. J. (2001). Thalassomonas viridans gen. nov., sp. nov., a novel marine Gamma-Proteobacterium. Int. J. Syst. Evol. Microbiol. 4), 1283–1289. doi: 10.1099/00207713-51-4-1283
Martin M. (2011). Cutadapt removes adapter sequences from high-throughput sequencing reads. EMBnet. J. 17, 10–12. doi: 10.14806/ej.17.1.200
Meier-Kolthoff J. P., Auch A. F., Klenk H. P., Göker M. (2013). Genome sequence-based species delimitation with confidence intervals and improved distance functions. BMC Bioinf. 14, 60. doi: 10.1186/1471-2105-14-60
Methé B. A., Nelson K. E., Deming J. W., Momen B., Melamud E., Zhang X., et al. (2005). The psychrophilic lifestyle as revealed by the genome sequence of Colwellia psychrerythraea 34H through genomic and proteomic analyses. Proc. Natl. Acad. Sci. U.S.A. 102, 10913–10918. doi: 10.1073/pnas.0504766102
Mikheenko A., Prjibelski A., Saveliev V., Antipov D., Gurevich A. (2018). Versatile genome assembly evaluation with QUAST-LG. Bioinformatics 34, i142–i150. doi: 10.1093/bioinformatics/bty266
Minh B. Q., Schmidt H. A., Chernomor O., Schrempf D., Woodhams M. D., von Haeseler A., et al. (2020). IQ-TREE 2: New models and efficient methods for phylogenetic inference in the genomic Era. Mol. Biol. Evol. 37, 1530–1534. doi: 10.1093/molbev/msaa015
Minnikin D., O’donnell A., Goodfellow M., Alderson G., Athalye M., Schaal A., et al. (1984). An integrated procedure for the extraction of bacterial isoprenoid quinones and polar lipids. J. Microbiol. Methods 2, 233–241. doi: 10.1016/0167-7012(84)90018-6
Nogi Y., Hosoya S., Kato C., Horikoshi K. (2004). Colwellia piezophila sp. nov., a novel piezophilic species from deep-sea sediments of the Japan Trench. Int. J. Syst. Evol. Microbiol. 54, 1627–1631. doi: 10.1099/ijs.0.03049-0
On S. L. W., Miller W. G., Biggs P. J., Cornelius A. J., Vandamme P. (2020). A critical rebuttal of the proposed division of the genus Arcobacter into six genera using comparative genomic, phylogenetic, and phenotypic criteria. Syst. Appl. Microbiol. 43, 126108. doi: 10.1016/j.syapm.2020.126108
Page A. J., Cummins C. A., Hunt M., Wong V. K., Reuter S., Holden M. T., et al. (2015). Roary: rapid large-scale prokaryote pan genome analysis. Bioinformatics 31, 3691–3693. doi: 10.1093/bioinformatics/btv421
Park S., Choi J., Won S. M., Yoon J. H. (2018). Thalassotalea insulae sp. nov., isolated from tidal flat sediment. Int. J. Syst. Evol. Microbiol. 68, 1321–1326. doi: 10.1099/ijsem.0.002671
Park S., Jung Y. T., Kang C. H., Park J. M., Yoon J. H. (2014). Thalassotalea ponticola sp. nov., isolated from seawater, reclassification of Thalassomonas fusca as Thalassotalea fusca comb. nov. and emended description of the genus Thalassotalea. Int. J. Syst. Evol. Microbiol. 64, 3676–3682. doi: 10.1099/ijs.0.067611-0
Park S., Jung Y. T., Yoon J. H. (2016). Colwellia sediminilitoris sp. nov., isolated from a tidal flat. Int. J. Syst. Evol. Microbiol. 66, 3258–3263. doi: 10.1099/ijsem.0.001183
Park M. J., Kim Y. J., Park M., Yu J., Namirimu T., Roh Y. R., et al. (2022). Establishment of genome based criteria for classification of the family Desulfovibrionaceae and proposal of two novel genera, Alkalidesulfovibrio gen. nov. and Salidesulfovibrio gen. nov. Front. Microbiol. 13. doi: 10.3389/fmicb.2022.738205
Park S., Park J.-M., Yoon J.-H. (2019). Colwellia ponticola sp. nov., isolated from seawater. Int. J. systematic evolutionary Microbiol. 69, 3062–3067. doi: 10.1099/ijsem.0.003590
Parks D. H., Imelfort M., Skennerton C. T., Hugenholtz P., Tyson G. W. (2015). CheckM: assessing the quality of microbial genomes recovered from isolates, single cells, and metagenomes. Genome Res. 25, 1043–1055. doi: 10.1101/gr.186072.114
Plotkin J. B., Kudla G. (2011). Synonymous but not the same: the causes and consequences of codon bias. Nat. Rev. Genet. 12, 32–42. doi: 10.1038/nrg2899
Qin Q. L., Xie B. B., Zhang X. Y., Chen X. L., Zhou B. C., Zhou J., et al. (2014). A proposed genus boundary for the prokaryotes based on genomic insights. J. Bacteriol 196, 2210–2215. doi: 10.1128/jb.01688-14
Rey-Velasco X., Lucena T., Belda A., Gasol J. M., Sánchez O., Arahal D. R., et al. (2024). Genomic and phenotypic characterization of 26 novel marine bacterial strains with relevant biogeochemical roles and widespread presence across the global ocean. Front. Microbiol. 15. doi: 10.3389/fmicb.2024.1407904
Rodriguez-R L. M., Konstantinidis K. T. (2014). Bypassing cultivation to identify bacterial species. Microbe 9, 111–118. doi: 10.1128/microbe.9.111.1
Rzhetsky A., Nei M. (1992). A simple method for estimating and testing minimum-evolution trees. Mol. Biol. Evol. 9, 945–967. doi: 10.1093/oxfordjournals.molbev.a040771
Saitou N., Nei M. (1987). The neighbor-joining method: a new method for reconstructing phylogenetic trees. Mol. Biol. Evol. 4, 406–425. doi: 10.1093/oxfordjournals.molbev.a040454
Sasser M. (1990). Identification of bacteria by gas chromatography of cellular fatty acids. MIDI Tech. Note. Newark, DE: MIDI Inc.
Seemann T. (2014). Prokka: rapid prokaryotic genome annotation. Bioinformatics 30, 2068–2069. doi: 10.1093/bioinformatics/btu153
Segata N., Börnigen D., Morgan X. C., Huttenhower C. (2013). PhyloPhlAn is a new method for improved phylogenetic and taxonomic placement of microbes. Nat. Commun. 4, 2304. doi: 10.1038/ncomms3304
Sharp P. M., Bailes E., Grocock R. J., Peden J. F., Sockett R. E. (2005). Variation in the strength of selected codon usage bias among bacteria. Nucleic Acids Res. 33, 1141–1153. doi: 10.1093/nar/gki242
Sheu S. Y., Liu L. P., Tang S. L., Chen W. M. (2016). Thalassotalea euphylliae sp. nov., isolated from the torch coral Euphyllia glabrescens. Int. J. Syst. Evol. Microbiol. 66, 5039–5045. doi: 10.1099/ijsem.0.001466
Sievert C. (2020). Interactive web-based data visualization with R, plotly, and shiny. Chapman and Hall/CRC. Available online at: https://plotly-r.com.
Soutar C. D., Stavrinides J. (2022). Phylogenomic analysis of the Erwiniaceae supports reclassification of Kalamiella piersonii to Pantoea piersonii comb. nov. and Erwinia gerundensis to the new genus Duffyella gen. nov. as Duffyella gerundensis comb. nov. Mol. Genet. Genomics 297, 213–225. doi: 10.1007/s00438-021-01829-3
Stamatakis A. (2014). RAxML version 8: a tool for phylogenetic analysis and post-analysis of large phylogenies. Bioinformatics 30, 1312–1313. doi: 10.1093/bioinformatics/btu033
Sun C., Huo Y.-Y., Liu J.-J., Pan J., Qi Y.-Z., Zhang X.-Q., et al. (2014). Thalassomonas eurytherma sp. nov., a marine Proteobacterium. Int. J. systematic evolutionary Microbiol. 64, 2079–2083. doi: 10.1099/ijs.0.058255-0
Suresh G., Lodha T. D., Indu B., Sasikala C., Ramana C. V. (2019). Taxogenomics resolves conflict in the genus Rhodobacter: A two and half decades pending thought to reclassify the genus Rhodobacter. Front. Microbiol. 10. doi: 10.3389/fmicb.2019.02480
Tamura K., Stecher G., Kumar S. (2021). MEGA11: molecular evolutionary genetics analysis version 11. Mol. Biol. Evol. 38, 3022–3027. doi: 10.1093/molbev/msab120
Tatsumi Y., Kato A., Sango K., Himeno T., Kondo M., Kato Y., et al. (2019). Omega-3 polyunsaturated fatty acids exert anti-oxidant effects through the nuclear factor (erythroid-derived 2)-related factor 2 pathway in immortalized mouse Schwann cells. J. Diabetes Investig. 10, 602–612. doi: 10.1111/jdi.12931
Techtmann S. M., Fitzgerald K. S., Stelling S. C., Joyner D. C., Uttukar S. M., Harris A. P., et al. (2016). Colwellia psychrerythraea strains from distant deep sea basins show adaptation to local conditions. Front. Environ. Sci. 4, 33. doi: 10.3389/fenvs.2016.00033
Thompson F. L., Barash Y., Sawabe T., Sharon G., Swings J., Rosenberg E. (2006). Thalassomonas loyana sp. nov., a causative agent of the white plague-like disease of corals on the Eilat coral reef. Int. J. Syst. Evol. Microbiol. 2), 365–368. doi: 10.1099/ijs.0.63800-09
Wang Y., Liu T., Ming H., Sun P., Cao C., Guo M., et al. (2018). Thalassotalea atypica sp. nov., isolated from seawater, and emended description of Thalassotalea eurytherma. Int. J. Syst. Evol. Microbiol. 68, 271–276. doi: 10.1099/ijsem.0.002495
Wang W., Tang K., Wang X. (2024). High temperatures increase the virulence of Vibrio bacteria towards their coral host and competing bacteria via type VI secretion systems. PloS Biol. 22, e3002788. doi: 10.1371/journal.pbio.3002788
Wang Y., Zhao R., Ji S., Li Z., Yu T., Li B., et al. (2013). Litorilituus sediminis gen. nov. sp. nov., isolated from coastal sediment of an amphioxus breeding zone in Qingdao, China. Antonie Van Leeuwenhoek 104, 423–430. doi: 10.1007/s10482-013-9967-4
Xu Z. X., Lu D. C., Liang Q. Y., Chen G. J., Du Z. J. (2016). Thalassotalea sediminis sp. nov., isolated from coastal sediment. Antonie Van Leeuwenhoek 109, 371–378. doi: 10.1007/s10482-015-0639-4
Xu Z., Masuda Y., Wang X., Ushijima N., Shiratori Y., Senoo K., et al. (2021). Genome-based taxonomic rearrangement of the order Geobacterales including the description of Geomonas azotofigens sp. nov. and Geomonas diazotrophica sp. nov. Front. Microbiol. 12. doi: 10.3389/fmicb.2021.737531
Xu Z.-X., Zhang H.-X., Han J.-R., Dunlap C. A., Rooney A. P., Mu D.-S., et al. (2017). Colwellia agarivorans sp. nov., an agar-digesting marine bacterium isolated from coastal seawater. Int. J. systematic evolutionary Microbiol. 67, 1969–1974. doi: 10.1099/ijsem.0.001897
Yamano R., Yu J., Haditomo A. H. C., Jiang C., Mino S., Romalde J. L., et al. (2023). Genome taxonomy of the genus Thalassotalea and proposal of Thalassotalea hakodatensis sp. nov. isolated from sea cucumber larvae. PloS One 18, e0286693. doi: 10.1371/journal.pone.0286693
Yoon S. H., Ha S. M., Kwon S., Lim J., Kim Y., Seo H., et al. (2017a). Introducing EzBioCloud: a taxonomically united database of 16S rRNA gene sequences and whole-genome assemblies. Int. J. Syst. Evol. Microbiol. 67, 1613–1617. doi: 10.1099/ijsem.0.001755
Yoon S. H., Ha S. M., Lim J., Kwon S., Chun J. (2017b). A large-scale evaluation of algorithms to calculate average nucleotide identity. Antonie Van Leeuwenhoek 110, 1281–1286. doi: 10.1007/s10482-017-0844-4
Yu Y., Li H. R., Zeng Y. X. (2011). Colwellia chukchiensis sp. nov., a psychrotolerant bacterium isolated from the Arctic Ocean. Int. J. Syst. Evol. Microbiol. 61, 850–853. doi: 10.1099/ijs.0.022111-0
Zhang C., Guo W., Wang Y., Chen X. (2017). Colwellia beringensis sp. nov., a psychrophilic bacterium isolated from the Bering Sea. Int. J. systematic evolutionary Microbiol. 67, 5102–5107. doi: 10.1099/ijsem.0.002423
Zhang L., Liu X., Liu J., Zhang Z. (2013). Characteristics and function of sulfur dioxygenase in Echiuran worm Urechis unicinctus. PloS One 8, e81885. doi: 10.1371/journal.pone.0081885
Zhang Y., Tang K., Shi X., Zhang X. H. (2014). Description of Thalassotalea piscium gen. nov., sp. nov., isolated from flounder (Paralichthys olivaceus), reclassification of four species of the genus Thalassomonas as members of the genus Thalassotalea gen. nov. and emended description of the genus Thalassomonas. Int. J. Syst. Evol. Microbiol. 64, 1223–1228. doi: 10.1099/ijs.0.055913-0
Zhang D. C., Yu Y., Xin Y. H., Liu H. C., Zhou P. J., Zhou Y. G. (2008). Colwellia polaris sp. nov., a psychrotolerant bacterium isolated from Arctic sea ice. Int. J. Syst. Evol. Microbiol. 58, 1931–1934. doi: 10.1099/ijs.0.65596-0
Keywords: amino acid identity, Colwelliaceae, genome-based classification, in silico analysis, phylogenetic revision, marine microbes
Citation: Lee MS, Yang S-H, Kim YJ, Park Y-J, Park M-J and Kwon KK (2025) Genome-based classification and phylogenetic revision of the family Colwelliaceae with proposals for new genera and species. Front. Ecol. Evol. 13:1532186. doi: 10.3389/fevo.2025.1532186
Received: 21 November 2024; Accepted: 26 March 2025;
Published: 23 April 2025.
Edited by:
Dapeng Wang, China University of Petroleum (East China), ChinaReviewed by:
Tiago Belintani, São Paulo State University, BrazilZhijun Zhang, Zhejiang Agriculture and Forestry University, China
Yanping Wei, Xinjiang Academy of Agricultural Sciences, China
Hayrettin Saygin, Ondokuz Mayıs University, Türkiye
Copyright © 2025 Lee, Yang, Kim, Park, Park and Kwon. This is an open-access article distributed under the terms of the Creative Commons Attribution License (CC BY). The use, distribution or reproduction in other forums is permitted, provided the original author(s) and the copyright owner(s) are credited and that the original publication in this journal is cited, in accordance with accepted academic practice. No use, distribution or reproduction is permitted which does not comply with these terms.
*Correspondence: Mi-Jeong Park, bWlqZW9uZzE2QGtpb3N0LmFjLmty; Kae Kyoung Kwon, a2trd29uQGtpb3N0LmFjLmty