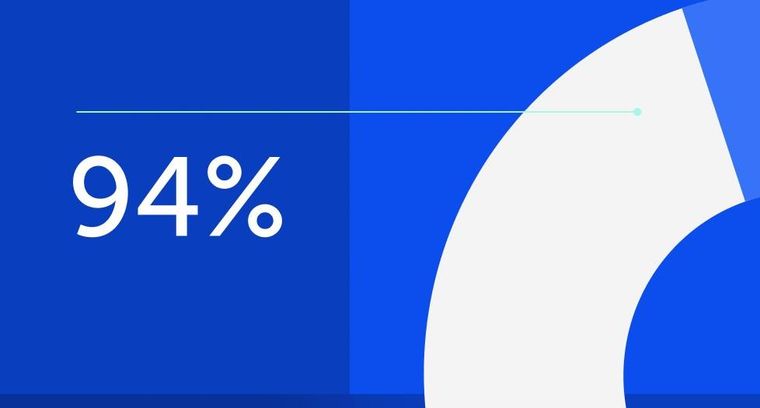
94% of researchers rate our articles as excellent or good
Learn more about the work of our research integrity team to safeguard the quality of each article we publish.
Find out more
ORIGINAL RESEARCH article
Front. Ecol. Evol., 15 April 2025
Sec. Behavioral and Evolutionary Ecology
Volume 13 - 2025 | https://doi.org/10.3389/fevo.2025.1492411
This article is part of the Research TopicEcology, Evolution, and Diversity of Papionini PrimatesView all articles
Introduction: Papionins are a well-studied and morphologically diverse clade of cercopithecid monkeys. Understanding how craniodental morphology varies in this clade has implications for interpreting taxonomic variation in the fossil record and for our understanding of primate evolution.
Methods: We quantified the phenotypic relationship between facial length and dental proportions in N=314 cercopithecid individuals across 10 species (six papionins, two cercopithecins, and two colobines) using dental ratios MMC (molar module component, ratio of the lengths of the third and first molars) and PMM (premolar-molar module, ratio of the lengths of the second molar and the fourth premolar) and two metrics of facial length: palatal length and prosthion – glabella.
Results: Facial length and molar dental proportions are significantly correlated interspecifically across cercopithecids (PGLS, p<0.01), where species with longer faces have relatively longer maxillary and mandibular third molars. These traits are generally not correlated intraspecifically in the cercopithecids sampled, with some exceptions.
Discussion: Our data demonstrate that prognathic faces evolved convergently at least twice in papionins, with parsimony supporting that Papio/Theropithecus shared a prognathic ancestor after the divergence of Lophocebus. Additionally, this study lends support to the hypothesis that facial reduction and third molar reduction in human evolution were coordinated and may have been the result of pleiotropy alongside changes in diet.
Papionini is a tribe of monkeys within Cercopithecidae—a speciose extant family of primates distributed across Africa and Asia today—and one of the most well-studied groups of primates due to their widespread use in biomedical studies (VandeBerg et al., 2009). Fossil papionins also commonly co-occur with hominid fossils in African deposits, making them of significant relevance to human evolutionary studies (e.g., Delson, 1984; 1988; Heaton, 2006; Frost et al., 2022; Jablonski and Frost, 2010; McKee, 1993; McKee et al., 1995; Williams et al., 2007). This diverse tribe is represented by both arboreal and terrestrial taxa with diets that range from grazing herbivorous and folivorous to more omnivorous diets that can include invertebrates and small mammals (Jablonski and Frost, 2010; Nowak, 1999). Most papionins are at least partially frugivorous, and the diets of almost all papionin species consist of more than 50% fruit (DeCasien et al., 2017).
The phylogenetic relationships between papionins have historically been obscured by morphological convergence (Figure 1). Papio, Theropithecus, and Mandrillus are all characterized by elongate faces and palates, and Macaca, Lophocebus, and Cercocebus have shorter faces and palates, more similar to other medium-bodied cercopithecid primates, with short faces presumed to be the ancestral phenotype (Benefit and McCrossin, 1991, 1993; Collard and O’Higgins, 2001; Fleagle et al., 2010; Pugh and Gilbert, 2018; Pugh et al., 2023; Ravosa and Profant, 2000; Simons et al., 2018; Singleton, 2002, 2005).
Figure 1. Phylogeny of cercopithecids sampled in this study, alongside dorsal views of species-representative crania. Divergence dates are from Perelman et al. (2011). Crania and branch lengths are not to scale. Recently named genus Rungwecebus, not included in this study, is inferred to belong to the monophyletic clade including Papio, Lophocebus, and Theropithecus (Davenport et al., 2006).
The mangabeys, Lophocebus and Cercocebus, both short-faced papionins, were not recognized as distinct genera belonging to different clades within Papionini until within the last few decades, based primarily on molecular data (Cronin and Sarich, 1975; Fleagle and McGraw, 1999; Groves, 1978; Harris and Disotell, 1998; Page and Goodman, 2001). Current molecular data support three major groups: 1) Macaca, a genus that diverged basally, approximately 8-10 million years ago (Ma), 2) Papio, Lophocebus, and Theropithecus, and 3) Mandrillus and Cercocebus, with these latter two clades having diverged from each other approximately 6-7 Ma. Even with disagreements about the phylogenetic relationships between Papio, Lophocebus, and Theropithecus (e.g., Chatterjee et al., 2009; Fabre et al., 2009; Guevara and Steiper, 2014; Perelman et al., 2011; Pozzi et al., 2014; Pugh and Gilbert, 2018; Springer et al., 2012), there is consensus that elongate snouts, sometimes referred to as prognathism, evolved at least twice in papionins. Hypotheses for why Papio and Mandrillus converged on prognathic faces range from allometric explanations, with selection on growth rates or growth spurts likely shaping variation in craniofacial shape in papionins (Leigh, 2007), to adaptive hypotheses focused on the jaw gape necessary to accommodate the long canines that characterize these large-bodied papionins (e.g., Plavcan and Ruff, 2008).
Tribe Papionini is well-represented in the Plio-Pleistocene African fossil record with extensive fossil samples from eastern and southern Africa (reviewed in Jablonski and Frost, 2010). Papionins begin appearing in the later Miocene of northern Africa with Macaca, the sister taxon to all other extant papionins (Figure 1), a genus that is especially well-represented in the later Plio-Pleistocene records of Europe and Asia.
Genus Parapapio appears in the later Miocene and early Pliocene record of eastern Africa, followed by larger samples in the Plio-Pleistocene of southern Africa. The exact phylogenetic position of this genus remains unclear, but current data support a basal phylogenetic position for Parapapio relative to other extant and fossil genera. Parapapio appears to have regionally diversified in the Pleistocene of southern Africa, with some earlier population potentially giving rise to the extant African papionin lineages (Jablonski, 2002; Jablonski and Frost, 2010; Pugh and Gilbert, 2018; Williams et al., 2007).
Following the presumed divergence of Theropithecus from Papio and Lophocebus around 4 million years ago (Figure 1; Cronin and Meikle, 1979; Perelman et al., 2011), Theropithecus dominates eastern African assemblages between ~3.5 to 1.5 million years ago, with increases in body size eventually leading to one of the largest monkey species ever known (Jablonski, 2002). During this time, southern African Pleistocene assemblages include representatives of Parapapio, Theropithecus, and notably, early Papio (e.g., Freedman, 1957, 1960, 1961, 1965; Heaton, 2006; Jones, 1937; Pugh and Gilbert, 2018; Szalay and Delson, 1979).
Starting in the Early Pleistocene, Papio hamadryas is well documented in southern Africa, and the species has been recognized in the Middle Pleistocene of the eastern African fossil record (Jablonski and Frost, 2010; Frost and Alemseged, 2007; Brasil et al., 2023). While the fossil records for Theropithecus and Papio are relatively rich among the extant papionin genera, the record of Lophocebus is limited to fragmentary remains from a few eastern African sites; Cercocebus is even more poorly represented, found only in the Early Pleistocene of southern Africa, and Mandrillus is entirely absent from the fossil record (Jablonski, 2002; Jablonski and Frost, 2010). Despite the uneven representation of papionin taxa in the fossil record, the relatively rich records of Papio, Theropithecus, and their candidate ancestor Parapapio, with many relatively complete cranial specimens, provide valuable data for assessing the evolution of craniodental anatomy in several papionin lineages.
Significant and pervasive craniofacial covariation characterizes many, but not all, cercopithecid species, generating species-specific patterns of covariation in this family (Monson, 2020). It has been hypothesized that the evolution of craniofacial morphology in more prognathic species like Papio is under greater constraint because of shared underlying genetic and developmental factors (Monson, 2020; Porto et al., 2009). The extent to which dental anatomy covaries with craniofacial anatomy in primates has been less well-studied (but see Polychronis and Halazonetis, 2014) despite the well-documented covariation between many areas of the cranium, including the face, braincase, and basicranium (e.g., Ackermann, 2002, 2005, 2009; Cheverud, 1982; Dayan et al., 2002; Esteve-Altava et al., 2015; Hallgrímsson et al., 2004, 2007; Lieberman, 2011; Neaux, 2017; Pérez-Claros et al., 2015; Polanski and Franciscus, 2006; von-Cramon-Taubadel and Smith, 2012).
Papio and Theropithecus have longer postcanine tooth rows relative to body mass than other cercopithecids, hypothesized to be related to their prognathic faces (Martin, 1993). Facial length is correlated with RUNX2 gene expression in anthropoid primates and linked to craniodental disorders in humans, commonly with the presence of extra teeth (Ritzman et al., 2017). Multivariate studies of tooth length also reveal a significant correlation with mandibular length, supporting that larger teeth are associated with larger jaws (Hikita et al., 2018).
Previous work has posed the hypothesis that the long third molars of papionins (relative to the other molars) are phenotypically associated with longer faces among the papionin clade (Monson et al., 2022b). This hypothesis builds from earlier studies that identified a strong correlation between average postcanine tooth area and facial size in a sample of cercopithecids (Scott, 2011). The aim of the present study is to investigate whether proportions of postcanine tooth length (relative to other postcanine teeth) are correlated with measures of facial length in a comparative phylogenetic framework. We used paired data from individuals to analyze both inter- and intraspecific variation in facial length and postcanine tooth proportions. Quantifying how these phenotypes covary has significant implications for our understanding of craniodental evolution and development; limited covariation suggests that traits may have been evolving independently, while significant covariation suggests shared genetic, developmental, and/or functional networks between traits, often resulting in linked evolutionary histories.
Additionally, this investigation stands to bear on our interpretations of taxonomic diversity in the fossil record. As the majority of primate fossil remains are craniodental, morphological traits of the cranium are commonly used to differentiate fossil species (e.g., Hartwig, 2002; Jablonski and Frost, 2010). In addition to cranial morphology, relative proportions of the third molar, as well as the first molar and fourth premolar, have been cited extensively in taxonomic classification schemes for extant and fossil papionins (e.g., Fleagle and McGraw, 1999; Gilbert, 2013), making an understanding of the allometric relationship between these traits fundamental to refining taxonomic and phylogenetic hypotheses.
Moreover, the last two million years of Homo evolution are characterized by a general reduction in jaw length, dental arcade length, tooth size, and facial length, with variation in these traits used to distinguish among hypothesized Plio-Pleistocene fossil species (e.g., Spoor et al., 2005). Investigating whether these changes are phenotypically linked is important for our understanding of craniodental variation and the selective pressures that shaped human evolution. Extreme facial length has evolved convergently in several cercopithecid genera, making them an ideal clade in which to investigate phenotypic covariation among craniodental anatomy (Collard and O’Higgins, 2001; Leigh, 2007; Monson, 2020; Singleton, 2005).
Our key goal for this work was to test whether facial length, as captured by palatal length and prosthion - glabella length, is phenotypically correlated with postcanine tooth proportions. A thorough assessment of craniofacial and dental anatomy in extant primates, where traits are paired and measured from the same individual, is essential for interpreting patterns of covariation in these traits. To meet this goal, we measured facial length and postcanine tooth lengths for N=314 extant cercopithecid individuals spanning 10 species across 10 genera, and addressed the following questions:
1. Are postcanine dental proportions (including relative proportions of the premolars compared to the molars) correlated with measures of facial length in papionin primates?
2. Specifically, do cercopithecid primates with longer faces also have relatively longer third molars (compared to other postcanine teeth)?
Our sample includes cranial and dental measurements for N=314 extant cercopithecid individuals, held in osteological collections at the American Museum of Natural History (AMNH) in New York, NY, USA, the Human Evolution Research Center (HERC) and the Museum of Vertebrate Zoology (MVZ) in Berkeley, CA, USA, the National Museum of Natural History (NMNH) in Washington, DC, USA, the Center for Evolutionary Origins of Human Behavior at Kyoto University (PRISK) in Inuyama, Japan, and the Zurich Natural History Museum (UZH) in Zurich, Switzerland.
All measurements were taken using analog DialMax calipers. Cranial and dental data were matched, measured from the same individual. Matching data requires that specimens are complete and in good condition, and grants the statistical power needed to assess both inter- and intraspecific correlations between traits. All individuals included in this study had a complete cranium and face, with complete maxillary and mandibular postcanine dentitions.
We only measured adult individuals as assessed by the eruption of the third molars. We attempted to measure sex-balanced samples whenever possible, but many papionins (e.g., Theropithecus, Mandrillus) are extremely rare in museum collections. Our sample includes n=190 papionins, n=57 cercopithecins, and n=67 colobines. See Table 1 for sample size details.
The extant and fossil data used in this study represent years of data collection in many different museum collections around the world. Given the complexity of gathering a dataset of this size, and in favor of maximizing taxonomic breadth and sample sizes, we focused on linear metrics for this study, collecting two cranial and eight dental measurements from all individuals sampled. The two cranial measurements provide different measures of facial length: (1) facial length (measured from prosthion to glabella) and (2) palatal length (measured from orale to staphylion). We did not focus on cranial length as a metric in this study because: 1) cranial length is a proxy for body size, and larger individuals often possess robust muscle attachments on the occipital bone, introducing measurement error (e.g., resulting from variation in external occipital crest morphology), and 2) very few fossil specimens preserve enough anatomy to confidently measure total cranial length; palatal and facial length, however, are preserved more frequently. Given these caveats, we focused on facial and palatal length, and the phenotypic association between these cranial traits and postcanine dental proportions, for this study. However, we did calculate relative facial length for a subsample (as noted by minimum sample sizes in Table 1) of extant primates (prosthion - glabella length divided by maximum cranial length) to investigate the effects of body size variation on the relationship between facial length and dental proportions.
We also measured the lengths of the fourth premolar and all three molars (P4-M3) for both the maxilla and the mandible to calculate two dental ratios: MMC (molar module component, measured as M3 length divided by M1 length), and PMM (premolar-molar module, measured as M2 length divided by P4 length; Hlusko et al., 2016). Quantitative genetic analyses demonstrate that these dental ratios are heritable (e.g., h2 mandibular MMC=0.69, h2 mandibular PMM=0.45) and do not covary with sex or body size in baboons (genus Papio; Hlusko et al., 2016; Monson et al., 2022a). Because molar lengths are guided by iterative developmental processes (e.g., Kavanagh et al., 2007), ratios of relative third molar length are more informative than absolute tooth lengths or widths, which are frequently linked to body size (e.g., Hlusko et al., 2016). The MMC and PMM dental ratios also have strong phylogenetic signal in mammals (e.g., Monson et al., 2019a; Zuercher et al., 2021). Additionally, because these dental ratios carry taxonomic signal, and teeth are relatively abundant in the hominid fossil record, MMC and PMM have already been employed in paleoanthropological investigations (e.g., Brasil et al., 2020; Monson et al., 2018, 2022a).
As part of our analysis, we also collected data on molar, fourth premolar, and facial/palatal lengths for n=50 fossil papionins from genera Parapapio, Procercocebus, Papio, and Theropithecus. Data for Victoriapithecus, a putative early ancestor of the cercopithecid primate family, were taken from the literature (Benefit, 1993; Benefit and McCrossin, 1997) and represent compiled data for multiple specimens. All other cranial and dental fossil data were measured by the authors and are matched, measured from individual fossils that retain all relevant morphology. The fossils are held in repositories at the Ditsong Museum of Natural History and the Evolutionary Studies Institute at the University of the Witwatersrand (South Africa), the National Museum of Ethiopia, and the University of California Museum of Paleontology (USA). See SI for raw data used in this study.
We log-corrected all cranial measurements and tested that all traits were distributed normally prior to analysis. We used the describeBy function in the psych package (Revelle and Revelle, 2015) to generate descriptive statistics and characterize the data. Average values for all traits are reported in Table 1 (extant cercopithecids). Average values for each trait were used to run a series of phylogenetic generalized least squares (PGLS) analyses, which test for covariation between traits while accounting for phylogenetic relationships. These analyses examine whether traits are correlated more than would be expected from neutral evolution and phylogenetic relatedness. All phylogenetic analyses were conducted using a published molecular supertree trimmed to species of interest (Faurby and Svenning, 2015). We chose this molecular phylogeny because it retains Papio, Lophocebus, and Theropithecus as sister taxa in an unresolved polytomy (in contrast to 10ktrees [Arnold et al., 2010] which places Lophocebus as sister to Theropithecus in a monophyletic clade with Papio placed basally, and more recent mammalian supertrees [e.g., Upham et al., 2019] that continue to place Lophocebus and Cercocebus in a monophyletic mangabey clade in contrast to current consensus that these species are within different clades of the papionin phylogeny [P/L/T vs. C/M]).
We ran PGLS analyses comparing trait variation between palatal length, facial length, relative facial length, and the two dental ratios (PMM and MMC, for both the maxillary and mandibular dentitions), using the pgls function in caper (Orme et al., 2013). All PGLS analyses are interspecific comparisons of quantitative trait values. We also compared trait values across clades with phylogenetic ANOVA using aov.phylo in geiger (Harmon et al., 2008). We performed a preliminary assessment of the impact of sexual dimorphism on trait covariation in our extant sample using ANOVA.
To assess intraspecific variation in craniodental traits, we calculated correlations between trait values within a single species using the rcorr function in the Hmisc package (Harrell and Harrell, 2019). We aimed for sample sizes of n=30 for each species included in the analyses. Seven species had adequate sample sizes for correlation analyses: Cercopithecus mitis, Cercocebus torquatus, Lophocebus albigena, Macaca mulatta, Papio hamadryas, Colobus guereza, and Presbytis rubicunda. We also included Mandrillus sphinx, with a minimum sample size of n=28, in the correlation analyses.
Craniodental trait variation was visualized at species and subfamily/tribe levels for both extant and fossil taxa using bivariate regressions, plotted with ggplot2 (Wickham, 2016). We plotted dental ratios, as well as one dental length (which increased fossil sample size), against palatal and facial length. All analyses were conducted in the statistical programming software R version 4.3.2 (R Core Team, 2021).
Facial length and palatal length, as well as relative facial length, are all highly correlated with relative third molar length in the maxilla and mandible (as reflected by MMC) in extant cercopithecid primates (Figure 2; Supplementary Figure S1; Table 2). Specifically, species with longer faces and palates tend to have relatively longer third molars (as compared to M1, measured via MMC). Three extant taxa—Mandrillus sphinx, Papio hamadryas, and Theropithecus gelada—sit at the upper end of the regression between molar proportions and facial/palatal length and are differentiated from other cercopithecids by having longer faces and palates, as well as relatively longer third molars (Figure 2, Supplementary Figure S1).
Figure 2. Species-level variation in palatal length compared to (A) maxillary and (B) mandibular MMC, and (C) maxillary and (D) mandibular PMM. The mathematical formulas for calculating MMC and PMM are presented to the right of panel (D) alongside a diagram of primate (human) postcanine dentition (mandibular). See Supplementary Figure S1 for bivariate comparisons of tooth proportions with facial length. Palatal and facial lengths are significantly correlated with maxillary and mandibular MMC but not PMM.
In contrast to relative third molar length, other postcanine tooth proportions are not correlated with facial and palatal length, or relative facial length, in extant cercopithecids. For example, raw metrics for maxillary second molar and fourth premolar lengths are correlated with palatal length (PGLS p<0.001), but the ratio of second molar to fourth premolar length is not (p>0.05), likely at least partially driven by the range of palatal lengths observed in extant Mandrillus. Likewise, we note a wide range of PMM values exhibited by cercopithecids, where taxa with similar facial and palatal lengths can have either high PMM values (relatively larger second molars, e.g., Theropithecus) or low ones (second molars and fourth premolars are more similar in size, e.g., Mandrillus).
Our data demonstrate that individuals cluster by species in postcanine proportions vs. prognathism morphospace (Figure 2). We note high taxonomic discrimination across cercopithecids when comparing facial and palatal length with PMM, which captures relative second molar to fourth premolar lengths. Mandibular PMM is the only trait that is significantly different between clades when assessed using phylogenetic ANOVA. The mandibular PMM dental ratio is significantly larger in Papio/Lophocebus/Theropithecus relative to Cercocebus/Mandrillus, Colobinae, Cercopithecini, and Macaca, likely driven by the higher PMM values (i.e., relatively longer second molars) of Theropithecus. Although statistical power is limited by small sample sizes, covariation between most traits varies significantly between males and females, likely related to the extreme sexual dimorphism that characterizes some cercopithecid species.
We also assessed PMM in fossil cercopithecids, including Parapapio (a putative ancestor of Papio and possibly other modern African papionins, Lophocebus and Theropithecus), and Procercocebus (hypothesized to be more closely related to Cercocebus and Mandrillus). The larger-bodied papionins have longer palates and second molar lengths and are distinguished in trait morphospace. While extant Papio and Mandrillus are distinguished by second molar and palatal lengths, fossil Papio overlaps with both extant taxa. Fossil Theropithecus clusters well outside the range of variation for extant Theropithecus and is quite distinct from other taxa in this study. Parapapio and Procercocebus have palatal lengths that are intermediate to the short- and long-faced extant papionins. Both Parapapio and Procercocebus overlap in morphospace with fossil and extant Papio but are distinct from Cercocebus and almost all specimens of Mandrillus (Figure 3).
Figure 3. Comparison of (A) maxillary PMM and (B) maxillary second molar length against palatal length in extant and fossil papionins.
Victoriapithecus, a short-faced basal cercopithecoid, looks very similar to Lophocebus, Macaca, and Colobus in relative and absolute second molar length, and palatal length. Maxillary second molar length compared to palatal length discriminates among several papionin taxa, short and long-faced taxa in particular. All prognathic taxa, including all fossil papionins included in this sample, sit at the upper end of the regression, with longer second molars and palates.
While facial and palatal length are significantly correlated with molar proportions across cercopithecids interspecifically, we do not find strong support for intraspecific correlations for most traits. There are some exceptions: Mandrillus is distinct in having dental proportions that are significantly correlated with facial and palatal lengths, excepting relative maxillary fourth premolar length (Table 3); Macaca is also characterized by significant correlations between maxillary molar proportions and facial and palatal lengths; Mandibular MMC is correlated with palatal length in Colobus, and mandibular PMM is correlated with both palatal and facial length in Papio and Cercopithecus; Relative facial length is also correlated with maxillary molar proportions in Presbytis and Cercopithecus, mandibular molar proportions in Presbytis, maxillary molar/premolar proportions in Colobus, and mandibular molar/premolar proportions in Mandrillus.
Cercopithecid monkeys with longer faces and palates are characterized by relatively longer third molar lengths (as compared to first molars). This evolutionary allometry has significant implications for our understanding of craniodental variation and evolution in primates and may also bear on hypotheses about human morphological evolution.
The tight interspecific correlation between cranial and dental traits speaks to potential genetic constraints underlying the evolution of facial/palatal length and molar proportions. As papionin faces evolved to be longer over the last 5 million years, third molars in these species also increased in relative length, potentially due to pleiotropy and/or overlapping developmental effects. Although the developmental and genetic mechanisms regulating facial length are still relatively unknown (Boughner and Hallgrímsson, 2008; Boughner et al., 2008; Usui and Tokita, 2018), there is a growing body of evidence that the RUNX2 gene is implicated in primate facial length (Ritzman et al., 2017). RUNX2 has also been implicated in early and late tooth development, as well as enamel and root formation (e.g., Chu et al., 2018; Komori, 2010; Wen et al., 2020), providing a potential genetic mechanism for covariation between facial length and molar proportions in primates.
There have been many attempts to characterize patterns of phenotypic covariation in this anatomical region, and there is strong evidence for conserved craniofacial covariation structure across mammals (e.g., Cardini, 2019; Cardini and Polly, 2013; Monson, 2020; Monson et al., 2017), although patterns of covariation can differ significantly across even closely related taxa (e.g., baboons and macaques; Monson, 2020). The lack of intraspecific correlation, or static allometry, between cranial and dental morphology in the majority of cercopithecid species sampled is consistent with other allometric trends, where environmental variation within species drowns out the anatomical covariation that shapes clade-level morphological diversification (e.g., Hlusko et al., 2006; Laughlin et al., 2017).
Cardini et al. (2015) proposed an evolutionary pattern called craniofacial allometry (CREA), arguing that small species tend to have short faces, and large species tend to have long faces (Cardini et al., 2015) and relatively smaller braincases (Cardini, 2019). Postnatal changes in body size can also result in changes in facial length, and allometry may enable craniofacial diversification in some clades (Cardini and Polly, 2013; Monson, 2020). We extend this previous work by demonstrating that long faces are significantly correlated with relatively longer third molars. As the third molars are the last teeth to develop (Kavanagh et al., 2007), the ontogeny of facial morphology may also play a role in craniodental covariation (Collard and O’Higgins, 2001; Singleton, 2002; Simons and Frost, 2021).
The strong taxonomic discrimination provided by measures of facial length and relative premolar size supports that these traits are heritable (as has already been demonstrated for relative premolar size; Hlusko et al., 2016; Monson et al., 2022a) and may be important for facilitating occupation of distinctive morphospaces associated with ecological and dietary niches.
Extant cercopithecids occupy distinctive morphospaces of postcanine dental proportions and facial/palatal lengths. While PMM does not appear to be associated with diet broadly across mammals (Monson et al., 2019a), this study clearly demonstrates the diversity of second molar and fourth premolar lengths relative to facial/palatal length in cercopithecid primates (Figure 3). These craniodental traits also partition the Cercocebus/Mandrillus and Papio/Lophocebus/Theropithecus clades (Figure 2D), aligning with previous work by Fleagle and McGraw (1999, 2002) demonstrating the relatively larger fourth premolar in Cercocebus and Mandrillus compared to Papio, Lophocebus, and Theropithecus, which they attribute to feeding behaviors and hard-object processing. Fleagle and McGraw (2002) also demonstrate that Macaca, which they use as an analogue for the papionin ancestor, has relatively larger fourth premolars, and they hypothesize that the enlarged first molars of Papio/Lophocebus/Theropithecus evolved independently in that clade.
A growing body of work has identified a strong allometric component to primate craniofacial variation with important implications for fossil taxonomy and paleontological studies (e.g., Collard and O’Higgins, 2001; Gilbert, 2013; Monson et al., 2017; Plavcan, 2002, 2003; Shirai and Marroig, 2010). Our work supports these previous studies and emphasizes that dental variation, and particularly molar proportions, should also be considered in assessments of primate craniofacial allometry and evolutionary relationships.
Most researchers now agree that prognathism is derived and evolved convergently in papionins (Benefit and McCrossin, 1991, 1993; Collard and O’Higgins, 2001; Cronin and Sarich, 1975; Delson, 1975; Disotell, 1994, 1996; Harris, 2000, 2002; Jolly, 1970; Strasser and Delson, 1987). The position of Victoriapithecus, a basal cercopithecoid, in trait morphospace suggests that the ancestral papionin morphology of dental proportions and facial lengths is likely similar to that seen in extant Macaca, Cercocebus, and Lophocebus. Fossil Papio, Parapapio and Procercocebus all look similar to extant baboons, although they tend to have relatively shorter third molars and shorter faces than extant taxa. These findings support that Parapapio and Procercocebus may represent an intermediate fossil morphology between the ancestral phenotypes typified by Victoriapithecus and other short-faced papionins, and the derived longer face in extant Papio and Mandrillus (see Brasil et al. [2025, this same issue] for a detailed history of the taxonomy and phylogenetic hypotheses for Parapapio). Further assessment of the relationships between postcanine tooth length proportions and facial/palatal length may help provide some indication of ancestor-descendent relationships, although additional fossils will be required to more thoroughly test these hypotheses.
If some early population of Parapapio is ancestral to other papionins, this would support that long faces evolved convergently in Papio/Theropithecus, and Mandrillus, with Parapapio as a likely medium-faced ancestor (this scenario supports that Lophocebus is an outgroup to Papio/Theropithecus). Alternatively, long faces may have evolved separately in all three lineages (Papio, Theropithecus, and Mandrillus) with short faces retained, or secondarily evolved, in Lophocebus and Cercocebus. A third scenario, less parsimonious based on available evidence, is that papionins shared a prognathic ancestor, currently undiscovered in the fossil record, and that Lophocebus and Cercocebus secondarily evolved shorter faces convergently.
In all scenarios, prognathic faces evolved convergently at least twice in papionins, and potentially three times, supporting that this trait is highly labile in this clade. The most parsimonious explanation is that Papio and Theropithecus shared a prognathic ancestor after the divergence of Lophocebus, and that long faces evolved twice in papionins (the first scenario described in the paragraph above). There continues to be debate about the phylogenetic relationships among papionins (e.g., Guevara and Steiper, 2014), with many researchers settling on a polytomy for Lophocebus/Papio/Theropithecus. Our work weighs in on this debate by providing morphological evidence that Lophocebus more likely diverged earliest in this clade, and prognathic faces evolved in a more recent common ancestor shared by Papio/Theropithecus.
It is possible that long-faced primates converged on relatively longer third molars through selection for longer teeth and/or selection for longer faces, as both traits have been hypothesized to assist in chewing and digesting a diet dominated by tough C4 plants and other abrasive materials (e.g., Cerling et al., 2011; Jablonski, 1993; Singleton, 2005). Future comparative studies looking at facial elongation in other mammals (e.g., Carnivora; Machado et al., 2018; Sears et al., 2007; Tamagnini et al., 2017), particularly herbivorous species (e.g., horses; Janis et al., 2024), as well as the biomechanics of chewing and muscular anatomy, may provide additional insights into the likely convergent evolution of prognathism in papionins.
Facial retraction in humans remains one of the most enigmatic changes in hominid cranial evolution. Decades of hypotheses attempting to explain the reduction of supraorbital robusticity and facial length in humans have focused on diet and the biomechanics of chewing, cognition and brain size, and other functional adaptations (e.g., Demes, 1987; Gómez-Robles et al., 2017; Preuschoft and Witzel, 2004). More recently, research has demonstrated the strong roles of craniodental integration and evolutionary allometry in shaping primate facial morphology. Although there is a large and growing body of research on cranial variation and modularity in anthropoids (e.g., Cummings et al., 2012; Grunstra et al., 2021; Kay and Kirk, 2000; Ravosa and Profant, 2000), key unanswered questions about the evolution of cranial anatomy remain, particularly for traits related to facial retraction.
Relative third molar lengths also reduced dramatically in Homo throughout the Plio-Pleistocene, leading to small maxillary and mandibular third molars in modern humans (e.g., Gómez-Robles et al., 2017; Ungar, 2012). High rates of agenesis of these teeth are also reported in humans, with occurrence of agenesis significantly associated with shorter facial and jaw lengths (e.g., Fekonja and Čretnik, 2022; Gkantidis et al., 2021). The identification of a common set of genes influencing both craniofacial development and tooth size has led researchers to hypothesize that reductions in prognathism and changes in tooth size proportions in human evolution are pleiotropically linked (e.g., Polychronis and Halazonetis, 2014). The impetus for reduced third molar length remains unexplained in human evolution, although a growing body of evidence suggests that these evolutionary changes may also be linked to changes in fetal growth and gestation (Monson et al., 2019b; 2022b).
Previous investigation of evolutionary changes in craniofacial and dental anatomy in fossil hominids has suggested that these traits may not be tightly linked (Gómez-Robles et al., 2017). However, this previous work focused on fossil samples and brain size rather than facial projection, and data for craniofacial and dental anatomy are often collected from unassociated specimens. Given the anatomical relationship between cranial and dental morphology seen in cercopithecids in this study, it is reasonable to hypothesize that the relatively reduced third molar length in humans may be associated with our shorter faces rather than resulting exclusively from any adaptive or functional role. Further analysis of morphological covariation between facial/palatal length and postcanine dental proportions across a broader sample of primates, particularly apes, will help test this hypothesis.
Facial and palatal lengths are significantly correlated with relative third molar length in cercopithecid primates. Species with longer faces have relatively longer third molars (as compared to first molars). The allometric relationship between cranial and dental variation in primates illuminates the tangled genetic and developmental networks that guide morphological diversification in this clade. Long prognathic faces evolved at least twice, and potentially three times in papionins (in Papio, Theropithecus, and Mandrillus), but the evolutionary history of this convergence remains obscured. Given the current morphological data, parsimony supports an evolutionary scenario where Papio and Theropithecus share a prognathic ancestor, and Lophocebus is a more basal sister species. Bringing a more comprehensive understanding of craniofacial covariation to paleontological studies promises to inform our approach to taxonomy and phylogenetic inference in the fossil record. We also highlight the possibility that selection for shorter faces, and/or relatively shorter third molars, may help explain coordinated patterns of facial reduction and reduced third molar length throughout the last several million years of human evolution.
The original contributions presented in the study are included in the article/Supplementary Material. Further inquiries can be directed to the corresponding author.
TM: Conceptualization, Data curation, Formal analysis, Funding acquisition, Investigation, Project administration, Visualization, Writing – original draft, Writing – review & editing. MB: Conceptualization, Data curation, Funding acquisition, Investigation, Writing – review & editing.
The author(s) declare that financial support was received for the research and/or publication of this article. This study was funded by the Human Evolution Research Center, the Leakey Foundation (grant to TAM), the National Science Foundation in the United States (NSF #2235771 to TAM and MFB), the National Science Foundation in Switzerland, the Palaeontological Scientific Trust (PAST), and Western Washington University.
The authors thank curators at the following repositories for assisting access to collections: American Museum of Natural History, NYC; Center for the Evolutionary Origins of Human Behavior, Kyoto University; Ditsong National Museum; Evolutionary Anthropology Collection, Zurich Natural History Museum; Evolutionary Studies Institute, University of the Witwatersrand; Human Evolutionary Research Center, Berkeley; Museum of Vertebrate Zoology, Berkeley; National Museum of Natural History, Washington, DC; and University of California Museum of Paleontology, Berkeley. We thank the two reviewers for their constructive comments that improved this manuscript. We are grateful to the editors of this Research Topic, Laura Martínez, Vivek Venkataraman, and Antoine Souron, for the invitation to contribute.
The authors declare that the research was conducted in the absence of any commercial or financial relationships that could be construed as a potential conflict of interest.
All claims expressed in this article are solely those of the authors and do not necessarily represent those of their affiliated organizations, or those of the publisher, the editors and the reviewers. Any product that may be evaluated in this article, or claim that may be made by its manufacturer, is not guaranteed or endorsed by the publisher.
The Supplementary Material for this article can be found online at: https://www.frontiersin.org/articles/10.3389/fevo.2025.1492411/full#supplementary-material
Ackermann R. R. (2002). Patterns of covariation in the hominoid craniofacial skeleton: implications for paleoanthropological models. J. Hum. Evol. 43, 167–187. doi: 10.1006/jhev.2002.0569
Ackermann R. R. (2005). Ontogenetic integration of the hominoid face. J. Hum. Evol. 48, 175–197. doi: 10.1016/j.jhevol.2004.11.001
Ackermann R. R. (2009). Morphological integration and the interpretation of fossil hominin diversity. Evol. Biol. 36, 149–156. doi: 10.1007/s11692-009-9050-2
Arnold C., Matthews L. J., Nunn C. L. (2010). The 10kTrees website: a new online resource for primate phylogeny. Evol. Anthropol.: Issues News Rev. 19, 114–118. doi: 10.1002/evan.20251
Benefit B. K. (1993). The permanent dentition and phylogenetic position of Victoriapithecus from Maboko Island, Kenya. J. Hum. Evol. 25, 83–172. doi: 10.1006/jhev.1993.1041
Benefit B. R., McCrossin M. L. (1991). Ancestral facial morphology of Old World higher primates. Proc. Nat. Acad. Sci. 88, 5267–5271. doi: 10.1073/pnas.88.12.5267
Benefit B. R., McCrossin M. L. (1993). Facial anatomy of Victoriapithecus and its relevance to the ancestral cranial morphology of Old World monkeys and apes. Am. J. Phys. Anthropol. 92, 329–370. doi: 10.1002/ajpa.1330920307
Benefit B. R., McCrossin M. L. (1997). Earliest known Old World monkey skull. Nature 388, 368–371. doi: 10.1038/41078
Boughner J. C., Hallgrímsson B. (2008). Biological spacetime and the temporal integration of functional modules: a case study of dento–gnathic developmental timing. Dev. Dyn.: Off. Publ. Am. Assoc. Anatomists 237, 1–17. doi: 10.1002/dvdy.v237:1
Boughner J. C., Wat S., Diewert V. M., Young N. M., Browder L. W., Hallgrímsson B. (2008). Short-faced mice and developmental interactions between the brain and the face. J. Anat. 213, 646–662. doi: 10.1111/j.1469-7580.2008.00999.x
Brasil M. F., Monson T. A., Schmitt C. A., Hlusko L. J. (2020). A genotype: phenotype approach to testing taxonomic hypotheses in hominids. Sci. Nat. 107, 1–16. doi: 10.1007/s00114-020-01696-9
Brasil M. F., Monson T. A., Stratford D. J., Hlusko L. J. (2025). A hypothesis-based approach to species identification in the fossil record: a papionin case study. Front. Ecol. Evol. 12, 1481903. doi: 10.3389/fevo.2024.1481903
Brasil M. F., Monson T. A., Taylor C. E., Yohler R. M., Hlusko L. J. (2023). A Pleistocene assemblage of near-modern Papio hamadryas from the Middle Awash study area, Afar Rift, Ethiopia. Am. J. Biol. Anthropol. 180, 48–76. doi: 10.1002/ajpa.v180.1
Cardini A. (2019). Craniofacial allometry is a rule in evolutionary radiations of placentals. Evol. Biol. 46, 239–248. doi: 10.1007/s11692-019-09477-7
Cardini A., Polly P. D. (2013). Larger mammals have longer faces because of size-related constraints on skull form. Nat. Commun. 4, 2458. doi: 10.1038/ncomms3458
Cardini A., Polly D., Dawson R., Milne N. (2015). Why the long face? Kangaroos and wallabies follow the same ‘rule’ of cranial evolutionary allometry (CREA) as placentals. Evol. Biol. 42, 169–176. doi: 10.1007/s11692-015-9308-9
Cerling T. E., Wynn J. G., Andanje S. A., Bird M. I., Korir D. K., Levin N. E., et al. (2011). Woody cover and hominin environments in the past 6 million years. Nature 476 (7358), 51–56. doi: 10.1038/nature10306
Chatterjee H. J., Ho S. Y., Barnes I., Groves C. (2009). Estimating the phylogeny and divergence times of primates using a supermatrix approach. BMC Evol. Biol. 9, 1–19. doi: 10.1186/1471-2148-9-259
Cheverud J. M. (1982). Phenotypic, genetic, and environmental morphological integration in the cranium. Evolution 36, 499–516. doi: 10.2307/2408096
Chu Q., Gao Y., Gao X., Dong Z., Song W., Xu Z., et al. (2018). Ablation of Runx2 in ameloblasts suppresses enamel maturation in tooth development. Sci. Rep. 8, 9594. doi: 10.1038/s41598-018-27873-5
Collard M., O’Higgins P. (2001). Ontogeny and homoplasy in the papionin monkey face. Evol. Dev. 3, 322–331. doi: 10.1046/j.1525-142X.2001.01042.x
Cronin J. E., Meikle W. E. (1979). The phyletic position of Theropithecus: congruence among molecular, morphological, and paleontological evidence. Syst. Biol. 28, 259–269. doi: 10.1093/sysbio/28.3.259
Cronin J. E., Sarich V. M. (1975). Molecular systematics of the New World monkeys. J. Hum. Evol. 4, 357–375. doi: 10.1016/0047-2484(75)90018-4
Cummings J. R., Muchlinski M. N., Kirk E. C., Rehorek S. J., DeLeon V. B., Smith T. D. (2012). Eye size at birth in prosimian primates: life history correlates and growth patterns. PLoS One 7, e36097. doi: 10.1371/journal.pone.0036097
Davenport T. R., Stanley W. T., Sargis E. J., De Luca D. W., Mpunga N. E., Machaga S. J., et al. (2006). A new genus of African monkey, Rungwecebus: morphology, ecology, and molecular phylogenetics. Science 312, 1378–1381. doi: 10.1126/science.1125631
Dayan T., Wool D., Simberloff D. (2002). Variation and covariation of skulls and teeth: modern carnivores and the interpretation of fossil mammals. Paleobiol. 28, 508–526. doi: 10.1666/0094-8373(2002)028<0508:VACOSA>2.0.CO;2
DeCasien A. R., Williams S. A., Higham J. P. (2017). Primate brain size is predicted by diet but not sociality. Nat. Ecol. Evol. 1, 0112. doi: 10.1038/s41559-017-0112
Delson E. (1984). Cercopithecoid biochronology of the African Plio-Pleistocene: correlation among eastern and southern hominid-bearing localities. Cour. Forsch. Inst. Senckenberg 69, 199–218.
Delson E. (1988). “Chronology of South African australopith site units,” in Evolutionary history of the ‘‘robust’’ australopithecines. Ed. Grine F. E. (Aldine, New York), p 317–p 324.
Demes B. (1987). Another look at an old face: biomechanics of the Neandertal facial skeleton reconsidered. J. Hum. Evol. 16, 297–303. doi: 10.1016/0047-2484(87)90005-4
Disotell T. R. (1994). Generic level relationships of the Papionini (Cercopithecoidea). Am. J. Phys. Anthropol. 94 (1), 47–57. doi: 10.1002/ajpa.1330940105
Disotell T. R. (1996). The phylogeny of Old World monkeys. Evol. Anthropol. 5 (1), 18–24. doi: 10.1002/(SICI)1520-6505(1996)5:1<18::AID-EVAN6>3.0.CO;2-S
Esteve-Altava B., Boughner J. C., Diogo R., Villmoare B. A., Rasskin-Gutman D. (2015). Anatomical network analysis shows decoupling of modular lability and complexity in the evolution of the primate skull. PLoS One 10, e0127653. doi: 10.1371/journal.pone.0127653
Fabre P. H., Rodrigues A., Douzery E. J. P. (2009). Patterns of macroevolution among Primates inferred from a supermatrix of mitochondrial and nuclear DNA. Mol. Phylogenet. Evol. 53, 808–825. doi: 10.1016/j.ympev.2009.08.004
Faurby S., Svenning J. C. (2015). A species-level phylogeny of all extant and late Quaternary extinct mammals using a novel heuristic-hierarchical Bayesian approach. Mol. Phylogenet. Evol. 84, 14–26. doi: 10.1016/j.ympev.2014.11.001
Fekonja A., Čretnik A. (2022). Comparison of craniofacial morphology in individuals with and without hypodontia with a special focus on the number of congenitally missing teeth. Front. Public Health 10, 1013862. doi: 10.3389/fpubh.2022.1013862
Fleagle J. G., Gilbert C. C., Baden A. L. (2010). Primate cranial diversity. Am. J. Phys. Anthropol. 142, 565–578. doi: 10.1002/ajpa.v142:4
Fleagle J. G., McGraw W. S. (1999). Skeletal and dental morphology supports diphyletic origin of baboons and mandrills. Proc. Natl. Acad. Sci. 96, 1157–1161. doi: 10.1073/pnas.96.3.1157
Fleagle J. G., McGraw W. S. (2002). Skeletal and dental morphology of African papionins: unmasking a cryptic clade. J. Hum. Evol. 42, 267–292. doi: 10.1006/jhev.2001.0526
Freedman L. (1960). Some new fossil cercopithecoid specimens from Makapansgat, South Africa. Palaeont. Afr. 7, 7–45.
Freedman L. (1961). New cercopithecoid fossils, including a new species, from Taung, Cape Province, South Africa. Ann. South Afr. Museum 46, 1–14.
Freedman L. (1965). Fossil and subfossil primates from the limestone deposits at Taung, Bolt’s Farm, and Witkrans, South Africa. Palaeont. Afr. 9, 19–48.
Frost S. R., Alemseged Z. (2007). Middle Pleistocene fossil Cercopithecidae from Asbole, Afar Region, Ethiopia. J. Hum. Evol. 53, 227–259. doi: 10.1016/j.jhevol.2007.02.003
Frost S. R., White F. J., Reda H. G., Gilbert C. C. (2022). Biochronology of South African hominin-bearing sites: a reassessment using cercopithecid primates. Proc. Natl. Acad. Sci. 119, e2210627119. doi: 10.1073/pnas.2210627119
Gilbert C. C. (2013). Cladistic analysis of extant and fossil African papionins using craniodental data. J. Hum. Evol. 64, 399–433. doi: 10.1016/j.jhevol.2013.01.013
Gkantidis N., Tacchi M., Oeschger E. S., Halazonetis D., Kanavakis G. (2021). Third molar agenesis is associated with facial size. Biol. 10, 650. doi: 10.3390/biology10070650
Gómez-Robles A., Smaers J. B., Holloway R. L., Polly P. D., Wood B. A. (2017). Brain enlargement and dental reduction were not linked in hominin evolution. Proc. Nat. Acad. Sci. 114, 468–473. doi: 10.1073/pnas.1608798114
Groves C. P. (1978). Phylogenetic and population systematics of the mangabeys (Primates: Cercopithecoidea). Primates 19, 1–34. doi: 10.1007/BF02373224
Grunstra N. D., Bartsch S. J., Le Maître A., Mitteroecker P. (2021). Detecting phylogenetic signal and adaptation in papionin cranial shape by decomposing variation at different spatial scales. Syst. Biol. 70, 694–706. doi: 10.1093/sysbio/syaa093
Guevara E. E., Steiper M. E. (2014). Molecular phylogenetic analysis of the Papionina using concatenation and species tree methods. J. Hum. Evol. 66, 18–28. doi: 10.1016/j.jhevol.2013.09.003
Hallgrímsson B., Lieberman D. E., Young N. M., Parsons T., Wat S., Bock G., et al. (2007). Tinkering: the microevolution of development. Eds. Bock G. R., Goode J. A. (London, England: Wiley), 164–190.
Hallgrímsson B., Willmore K., Dorval C., Cooper D. M. (2004). Craniofacial variability and modularity in macaques and mice. J. Exp. Zoology Part B: Mol. Dev. Evol. 302, 207–225. doi: 10.1002/jez.b.v302b:3
Harmon L., Weir J., Brock C., Glor R., Challenger W. (2008). GEIGER: investigating evolutionary radiations. Bioinform. 24, 129–131. doi: 10.1093/bioinformatics/btm538
Harris E. E. (2000). Molecular systematics of the Old World monkey tribe Papionini: analysis of the total available genetic sequences. J. Hum. Evol. 38 (2), 235–256. doi: 10.1006/jhev.1999.0318
Harris E. E. (2002). Why such long faces? Response to Collard O'Higgins. Evol. Dev. 4 (3), 167–168. doi: 10.1046/j.1525-142X.2002.02006.x
Harris E. E., Disotell T. R. (1998). Nuclear gene trees and the phylogenetic relationships of the mangabeys (Primates: Papionini). Mol. Biol. Evol. 15, 892–900. doi: 10.1093/oxfordjournals.molbev.a025993
Hartwig W. C. (Ed.) (2002). The primate fossil record (Vol. 33). (Cambridge: Cambridge University Press).
Heaton J. L. (2006). Taxonomy of the Sterkfontein fossil Cercopithecinae: the Papionini of Member 2 and 4 (Gauteng, South Africa). Indiana University, Bloomington, IN.
Hikita Y., Yamaguchi T., Tomita D., Adel M., Nakawaki T., Katayama K., et al. (2018). Growth hormone receptor gene is related to root length and tooth length in human teeth. Angle Orthodont. 88, 575–581. doi: 10.2319/092917-659.1
Hlusko L. J., Lease L. R., Mahaney M. C. (2006). Evolution of genetically correlated traits: tooth size and body size in baboons. Am. J. Phys. Anthropol. 131, 420–427. doi: 10.1002/ajpa.v131:3
Hlusko L. J., Schmitt C. A., Monson T. A., Brasil M. F., Mahaney M. C. (2016). The integration of quantitative genetics, paleontology, and neontology reveals genetic underpinnings of primate dental evolution. Proc. Natl. Acad. Sci. 113, 9262–9267. doi: 10.1073/pnas.1605901113
Jablonski N. G. (1993). Theropithecus: the rise and fall of a primate genus (Cambridge: Cambridge University Press).
Jablonski N. G. (2002). Fossil Old World monkeys: the late Neogene radiation. In: The primate fossil record. Ed. Hartwig W. C. (Cambridge: Cambridge University Press), 225–300.
Jablonski N. G., Frost S. R. (2010). “Cercopithecoidea,” in Cenozoic mammals of Africa. (Berkeley, CA: University of California Press).
Janis C. M., Richardson W., Morales-García N. M., Baird C. N. (2024). A horse walks into a bar: an explanation for the long face. Ann. Zool. Fennici 61, 503–529. doi: 10.5735/086.061.0130
Jolly C. J. (1970). The seed-eaters: a new model of hominid differentiation based on a baboon analogy. Man 5 (1), 5. doi: 10.2307/2798801
Jones T. R. (1937). A new fossil primate from Sterkfontein, Krugersdorp, Transvaal. S. Afr. J. Sci. 33, 709–728.
Kavanagh K. D., Evans A. R., Jernvall J. (2007). Predicting evolutionary patterns of mammalian teeth from development. Nature 449, 427–432. doi: 10.1038/nature06153
Kay R. F., Kirk E. C. (2000). Osteological evidence for the evolution of activity pattern and visual acuity in primates. Am. J. Phys. Anthropol. 113, 235–262. doi: 10.1002/1096-8644(200010)113:2<235::AID-AJPA7>3.0.CO;2-9
Komori T. (2010). Regulation of osteoblast and odontoblast differentiation by RUNX2. J. Oral. Biosci. 52 (1), 22–25. doi: 10.1016/S1349-0079(10)80004-0
Laughlin D. C., Lusk C. H., Bellingham P. J., Burslem D. F., Simpson A. H., Kramer-Walter K. R. (2017). Intraspecific trait variation can weaken interspecific trait correlations when assessing the whole-plant economic spectrum. Ecol. Evol. 7, 8936–8949. doi: 10.1002/ece3.2017.7.issue-21
Leigh S. R. (2007). Homoplasy and the evolution of ontogeny in papionin primates. J. Hum. Evol. 52, 536–558. doi: 10.1016/j.jhevol.2006.11.016
Machado F. A., Zahn T. M. G., Marroig G. (2018). Evolution of morphological integration in the skull of Carnivora (Mammalia): changes in Canidae lead to increased evolutionary potential of facial traits. Evol. 72 (7), 1399–1419.
Martin R. D. (1993). “Allometric aspects of skull morphology in Theropithecus,” in Theropithecus: the rise and fall of a primate genus. Ed. Jablonski N. G. (Cambridge University Press, Cambridge), 273–p 298.
McKee J. K. (1993). Faunal dating of the Taung hominid fossil deposit. J. Hum. Evol. 25, 363–376. doi: 10.1006/jhev.1993.1055
McKee J. K., Thackeray J. F., Berger L. R. (1995). Faunal assemblage seriation of southern African Pliocene and Pleistocene fossil deposits. Am. J. Phys. Anthropol. 96, 235–250. doi: 10.1002/ajpa.1330960303
Monson T. A. (2020). Patterns and magnitudes of craniofacial covariation in extant cercopithecids. Anat. Rec. 303, 3068–3084. doi: 10.1002/ar.v303.12
Monson T. A., Armitage D. W., Hlusko L. J. (2018). Using machine learning to classify extant apes and interpret the dental morphology of the chimpanzee-human last common ancestor. PaleoBios 35, ucmp_paleobios_40776. doi: 10.5070/P9351040776
Monson T. A., Boisserie J. R., Brasil M. F., Clay S. M., Dvoretzky R., Ravindramurthy S., et al. (2019a). Evidence of strong stabilizing effects on the evolution of boreoeutherian (Mammalia) dental proportions. Ecol. Evol. 9, 7597–7612. doi: 10.1002/ece3.2019.9.issue-13
Monson T. A., Brasil M. F., Mahaney M. C., Schmitt C. A., Taylor C. E., Hlusko L. J. (2022a). Keeping 21st century paleontology grounded: quantitative genetic analyses and ancestral state reconstruction re-emphasize the essentiality of fossils. Biol. 11, 1218. doi: 10.3390/biology11081218
Monson T. A., Coleman J. L., Hlusko L. J. (2019b). Craniodental allometry, prenatal growth rates, and the evolutionary loss of the third molars in New World monkeys. Anat. Rec. 302, 1419–1433. doi: 10.1002/ar.23979
Monson T. A., Brasil M. F., Stratford D. J., Hlusko L. J. (2017). Patterns of craniofacial variation and taxonomic diversity in the South African Cercopithecidae fossil record. Palaeo. Elec. 20.1.7A, 1–20. doi: 10.26879/690
Monson T. A., Weitz A. P., Brasil M. F., Hlusko L. J. (2022b). Teeth, prenatal growth rates, and the evolution of human-like pregnancy in later Homo. Proc. Natl. Acad. Sci. 119, e2200689119. doi: 10.1073/pnas.2200689119
Neaux D. (2017). Morphological integration of the cranium in Homo, Pan, and Hylobates and the evolution of hominoid facial structures. Am. J. Phys. Anthropol. 162, 732–746. doi: 10.1002/ajpa.v162.4
Nowak R. M. (1999). Walker’s Mammals of the World. (Baltimore, Maryland: The Johns Hopkins University Press).
Orme D., Freckleton R., Thomas G., Petzoldt T., Fritz S., Isaac N., et al. (2013). The caper package: comparative analysis of phylogenetics and evolution in R. R Package version 5, 1–36.
Page S. L., Goodman M. (2001). Catarrhine phylogeny: noncoding DNA evidence for a diphyletic origin of the mangabeys and for a human–chimpanzee clade. Mol. Phylogenet. Evol. 18, 14–25. doi: 10.1006/mpev.2000.0895
Perelman P., Johnson W. E., Roos C., Seuánez H. N., Horvath J. E., Moreira M. A., et al. (2011). A molecular phylogeny of living primates. PLoS Genet. 7, e1001342. doi: 10.1371/journal.pgen.1001342
Pérez-Claros J. A., Jiménez-Arenas J. M., Palmqvist P. (2015). Neurocranium versus face: a morphometric approach with classical anthropometric variables for characterizing patterns of cranial integration in extant hominoids and extinct hominins. PLoS One 10, e0131055. doi: 10.1371/journal.pone.0131055
Plavcan J. M. (2002). Taxonomic variation in the patterns of craniofacial dimorphism in primates. J. Hum. Evol. 42, 579–608. doi: 10.1006/jhev.2001.0542
Plavcan J. M. (2003). Scaling relationships between craniofacial sexual dimorphism and body mass dimorphism in primates: implications for the fossil record. Am. J. Phys. Anthropol. 120, 38–60. doi: 10.1002/ajpa.v120:1
Plavcan J. M., Ruff C. B. (2008). Canine size, shape, and bending strength in primates and carnivores. Am. J. Phys. Anthropol. 136, 65–84. doi: 10.1002/ajpa.v136:1
Polanski J. M., Franciscus R. G. (2006). Patterns of craniofacial integration in extant Homo, Pan, and Gorilla. Am. J. Phys. Anthropol. 131, 38–49. doi: 10.1002/(ISSN)1096-8644
Polychronis G., Halazonetis D. J. (2014). Shape covariation between the craniofacial complex and first molars in humans. J. Anat. 225, 220–231. doi: 10.1111/joa.2014.225.issue-2
Porto A., de Oliveira F. B., Shirai L. T., De Conto V., Marroig G. (2009). The evolution of modularity in the mammalian skull I: morphological integration patterns and magnitudes. Evol. Biol. 36, 118–135. doi: 10.1007/s11692-008-9038-3
Pozzi L., Hodgson J. A., Burrell A. S., Sterner K. N., Raaum R. L., Disotell T. R. (2014). Primate phylogenetic relationships and divergence dates inferred from complete mitochondrial genomes. Mol. Phylogenet. Evol. 75, 165–183. doi: 10.1016/j.ympev.2014.02.023
Preuschoft H., Witzel U. (2004). Functional structure of the skull in Hominoidea. Folia Primatol. 75, 219–252. doi: 10.1159/000078936
Pugh K. D., Catalano S. A., Pérez de los Ríos M., Fortuny J., Shearer B. M., Vecino Gazabón A., et al. (2023). The reconstructed cranium of Pierolapithecus and the evolution of the great ape face. Proc. Natl. Acad. Sci. 120, e2218778120. doi: 10.1073/pnas.2218778120
Pugh K. D., Gilbert C. C. (2018). Phylogenetic relationships of living and fossil African papionins: combined evidence from morphology and molecules. J. Hum. Evol. 123, 35–51. doi: 10.1016/j.jhevol.2018.06.002
Ravosa M. J., Profant L. P. (2000). “Evolutionary morphology of the skull in Old World monkeys,” in Old World Monkeys. Eds. Whitehead P. F., Jolly C. J. (Cambridge University Press, Cambridge), 237–268.
R Core Team (2021). R: a language and environment for statistical computing (Vienna, Austria: R Foundation for Statistical Computing). Available at: https://www.R-project.org/ (Accessed March 1, 2025).
Ritzman T. B., Banovich N., Buss K. P., Guida J., Rubel M. A., Pinney J., et al. (2017). Facing the facts: the Runx2 gene is associated with variation in facial morphology in primates. J. Hum. Evol. 111, 139–151. doi: 10.1016/j.jhevol.2017.06.014
Scott J. E. (2011). Folivory, frugivory, and postcanine size in the Cercopithecoidea revisited. Am. J. Phys. Anthropol. 146, 20–27. doi: 10.1002/ajpa.v146.1
Sears K. E., Goswami A., Flynn J. J., Niswander L. A. (2007). The correlated evolution of Runx2 tandem repeats, transcriptional activity, and facial length in Carnivora. Evol. Dev. 9, 555–565. doi: 10.1111/j.1525-142X.2007.00196.x
Shirai L. T., Marroig G. (2010). Skull modularity in neotropical marsupials and monkeys: size variation and evolutionary constraint and flexibility. J. Exp. Zool. Part B: Mol. Dev. Evol. 314, 663–683. doi: 10.1002/jez.b.v314b:8
Simons E. A., Frost S. R., Singleton M. (2018). Ontogeny and phylogeny of the cercopithecine cranium: a geometric morphometric approach to comparing shape change trajectories. J. Hum. Evol. 124, 40–51. doi: 10.1016/j.jhevol.2018.08.001
Simons E. A., Frost S. R. (2021). Ontogenetic allometry and scaling in catarrhine crania. J. Anat. 238, 693–710. doi: 10.1111/joa.v238.3
Singleton M. (2002). Patterns of cranial shape variation in the Papionini (Primates: Cercopithecinae). J. Hum. Evol. 42, 547–578. doi: 10.1006/jhev.2001.0539
Singleton M. (2005). “Functional shape variation in the cercopithecine masticatory complex,” in Modern morphometrics in physical anthropology. Ed. Slice D. E. (Springer, Boston), 319–348.
Spoor F., Leakey M. G., Leakey L. N. (2005). Correlation of cranial and mandibular prognathism in extant and fossil hominids. Trans. R. Soc. South Afr. 60, 85–89. doi: 10.1080/00359190509520482
Springer M. S., Meredith R. W., Gatesy J., Emerling C. A., Park J., Rabosky D. L., et al. (2012). Macroevolutionary dynamics and historical biogeography of primate diversification inferred from a species supermatrix. PLoS One 7, e49521. doi: 10.1371/journal.pone.0049521
Strasser E., Delson E. (1987). Cladistic analysis of cercopithecid relationships. J. Hum. Evol. 16 (1), 81–99. doi: 10.1016/0047-2484(87)90061-3
Tamagnini D., Meloro C., Cardini A. (2017). Anyone with a long-face? Craniofacial evolutionary allometry (CREA) in a family of short-faced mammals, the Felidae. Evol. Biol. 44, 476–495. doi: 10.1007/s11692-017-9421-z
Ungar P. S. (2012). Dental evidence for the reconstruction of diet in African early Homo. Curr. Anthropol. 53, S318–S329. doi: 10.1086/666700
Upham N. S., Esselstyn J. A., Jetz W. (2019). Inferring the mammal tree: species-level sets of phylogenies for questions in ecology, evolution, and conservation. PLoS Biol. 17, e3000494. doi: 10.1371/journal.pbio.3000494
Usui K., Tokita M. (2018). Creating diversity in mammalian facial morphology: a review of potential developmental mechanisms. EvoDevo 9, 1–17. doi: 10.1186/s13227-018-0103-4
VandeBerg J. L., Williams-Blangero S., Tardif S. D. (Eds.) (2009). The Baboon in Biomedical Research (New York, NY: Springer Science & Business Media).
von-Cramon-Taubadel N., Smith H. F. (2012). The relative congruence of cranial and genetic estimates of hominoid taxon relationships: implications for the reconstruction of hominin phylogeny. J. Hum. Evol. 62, 640–653. doi: 10.1016/j.jhevol.2012.02.007
Wen Q., Jing J., Han X., Feng J., Yuan Y., Ma Y., et al. (2020). Runx2 regulates mouse tooth root development via activation of WNT inhibitor NOTUM. J. Bone Miner. Res. 35, 2252–2264. doi: 10.1002/jbmr.4120
Wickham H. (2016). ggplot2: Elegant Graphics for Data Analysis (Springer-Verlag New York). Available at: https://ggplot2.tidyverse.org (Accessed March 1, 2025).
Williams F. L., Ackermann R. R., Leigh S. R. (2007). Inferring Plio-Pleistocene southern African biochronology from facial affinities in Parapapio and other fossil papionins. Am. J. Phys. Anthropol. 132, 163–174. doi: 10.1002/ajpa.v132:2
Keywords: dental proportions, prognathism, Papionini, African fossil record, allometry
Citation: Monson TA and Brasil MF (2025) The evolution of facial length and molar proportions in cercopithecid monkeys. Front. Ecol. Evol. 13:1492411. doi: 10.3389/fevo.2025.1492411
Received: 06 September 2024; Accepted: 14 March 2025;
Published: 15 April 2025.
Edited by:
Vivek Venkataraman, University of Calgary, CanadaReviewed by:
Stephen Frost, University of Oregon, United StatesCopyright © 2025 Monson and Brasil. This is an open-access article distributed under the terms of the Creative Commons Attribution License (CC BY). The use, distribution or reproduction in other forums is permitted, provided the original author(s) and the copyright owner(s) are credited and that the original publication in this journal is cited, in accordance with accepted academic practice. No use, distribution or reproduction is permitted which does not comply with these terms.
*Correspondence: Tesla A. Monson, bW9uc29udDJAd3d1LmVkdQ==
Disclaimer: All claims expressed in this article are solely those of the authors and do not necessarily represent those of their affiliated organizations, or those of the publisher, the editors and the reviewers. Any product that may be evaluated in this article or claim that may be made by its manufacturer is not guaranteed or endorsed by the publisher.
Research integrity at Frontiers
Learn more about the work of our research integrity team to safeguard the quality of each article we publish.