- 1Key Laboratory of National Forestry and Grassland Administration on Plant Conservation and Utilization in Southern China, South China Botanical Garden, Chinese Academy of Sciences, Guangzhou, China
- 2State Key Laboratory of Plant Diversity and Specialty Crops, South China Botanical Garden, Chinese Academy of Sciences, Guangzhou, China
- 3College of Life Sciences, University of Chinese Academy of Sciences, Beijing, China
- 4Nanning Qingxiushan Scenic and Historic Tourism Development Co., Ltd., Nanning Botanical Garden, Nanning, China
- 5Ting Jie Natural History Studio, Yangjiang, China
Cycas fairylakea is an endemic and Level-One National Key Protected Wild Plant in China. It is distributed in the Meilin Reservoir and Tanglangshan Country Park in Shenzhen. This study investigated two newly discovered wild populations in Taishan and Yangjiang, Guangdong Province. The forest community characteristics and population structure of these newly discovered populations remain unknown, and the sex ratio as well as the species’ responses to environmental factors have never been reported, despite their importance for the in-situ conservation of this Cycad. We conducted a comprehensive investigation of the ecological traits (soil properties, community characteristics, and phylogenetic diversity) and population characters (size, age structure, sex ratio, and spatial pattern) of C. fairylakea and determined the impact of environmental factors on the age structure and sex ratio. The results showed that the species number and composition of the two communities differed, yet no significant differences were observed in the plant diversity indices, with close phylogenetic affinities among species. Moreover, the sex ratio, determined by the MADS-Y marker, was relatively balanced. The age structure of the two populations exhibited a “reverse-J” shape, and the survival curves were of the Deevey’s type III, indicating low natural regeneration capacities with high mortality in the early stage. Redundancy analysis identified the soil properties as constraining factors of the C. fairylakea sex ratio, while climate factors and community species diversity and evenness indices positively impacted the sex ratio. The combined effects of soil, climate, topography, and shrub layer plant diversity were the main factors influencing the age structure and sex ratio of the C. fairylakea populations. A balanced sex ratio ensures reproductive capacity, which maintains population stability. However, the structural and numerical characteristics of C. fairylakea in the two regions are constrained by environmental pressures. The implementation of well-considered artificial conservation strategies and the safeguarding of the habitat are intended to bolster the survival rate of the seedlings, promote the healthy growth of middle-aged individuals, and maintain the sustained stability of the natural population of C. fairylakea.
1 Introduction
Cycads represent one of the most ancient groups of seed plants, with origins dating back to the mid-Permian period approximately 300 million years ago. They were once the dominant flora of terrestrial ecosystems during the Mesozoic era, often renowned as “living fossils” (Raven et al., 2005; Nagalingum et al., 2011). However, the modern landscape for Cycads is starkly different, with two-thirds of the species listed as threatened on the International Union for the Conservation of Nature (IUCN) Red List (IUCN, 2024). The survival of these ancient plants in the wild is gravely endangered by factors such as over-exploitation and habitat degradation (Yang et al., 2017). Among the Cycads, Cycas fairylakea D. Y. Wang is particularly at risk, classified as Critically Endangered (CR) on the IUCN Red List (Qin et al., 2017; Yang, 2021). This species is endemic to China and recognized for its limited population size. It is designated as a Level-One National Key Protected Wild Plant in China (State Forestry and Grassland Administration and the Ministry of Agriculture and Rural Affairs, P. R. China, 2021). Furthermore, C. fairylakea is under international protection from the Convention on International Trade in Endangered Species of Wild Fauna and Flora (CITES, 2021).
Cycads are long-lived, dioecious, and woody plants. The gender of the Cycads plant remains unknown until it reaches the flowering age. Dioecy, the condition of having separate male and female individuals, is a fundamental evolutionary strategy that promotes cross-fertilization and maintains genetic diversity and heterozygosity (Dellaporta and Calderon-Urrea, 1993; Hamrick and Godt, 1996; Edwards, 2000). Typically, the initial sex ratio in dioecious species is evenly split between females and males, assuming equal resource investment in offspring (Fisher, 1930). However, female plants often invest more resources in reproduction due to the need to bear fruits and seeds (Obeso, 2002). Dioecious plants may exhibit a variety of sex ratios, with a male bias (46%), balance (33%), or female bias (21%), influenced by factors such as environmental pressures, parental investment, and other ecological dynamics (Barrett et al., 2010). In Cycads, sex determination is linked to heteromorphic chromosomes (Abraham and Mathew, 1962). Scholars have identified a sex-associated region, which is highly differentiated between male and female Cycas genomes. The XY sex-determination system is located on chromosome 8 (Liu et al., 2022). Specifically, in C. panzhihuaensis, a region of the Y chromosome containing a MADS-box transcription factor is exclusively expressed in male cones. This gene, named CYCAS_034085 as MADS-Y, is suggested to be a potential sex determination factor. The sequence of this region was reported by Gong et al. (2022), and can be utilized to design appropriate primers for C. fairylakea. To date, no published reports have described the sex ratio of C. fairylakea. Therefore, we present for the first time the sex ratio of this species using a PCR-based gender identification method.
C. fairylakea was first described in the seminal work “Cycads of China” by Wang Dingyue in 1996 (Wang et al., 1996). It was published based on cultivated specimens from the Fairy Lake Botanical Garden, Shenzhen, China, which were originally misidentified as C. taiwaniana. The discovery of C. fairylakea’s wild population remained elusive until November 1999, when researchers identified wild populations of C. fairylakea thriving in Meilin Reservoir and Tanglangshan Country Park (TLS) in Shenzhen (Figure 1A). The Meilin Reservoir alone was home to over 2,000 plants, while Tanglangshan Country Park hosted fewer than 700 individuals (Wang et al., 2003; Fu and Liu, 2017). In response to this discovery, Shenzhen City established the Shenzhen Meilin Reservoir Cycas fairylakea Protection Area to safeguard this precious botanical resource. Further explorations revealed additional wild populations in Lion Mountain of Luokeng Town and Zhangshi Town, Qujiang District, Shaoguan City, Guangdong Province, as well as in Heshan City, Jiangmen City. These findings revealed that C. fairylakea prefers humid environments and is commonly found along the sides of streams and ditches, often growing in soil that is relatively rich and fertile, and is less commonly seen in arid and barren environments (Jian et al., 2005a, 2005b). Scholars subsequently conducted a series of studies on various aspects of C. fairylakea, including community characteristic research (Wang et al., 2004), revealing that the C. fairylakea community belongs to south subtropical secondary evergreen broad-leaved forests, comprising dominant populations of Sterculia lanceolata, Heptapleurum heptaphyllum, Aporosa dioica, Itea chinensis, and Acronychia pedunculata. Research on biological traits (Jian et al., 2005a) indicated that due to over-exploitation, urbanization, environmental pollution, and diseases and pests, the number of its wild populations and individuals is continuously decreasing, the age structure is pyramid-shaped, the number of flowering plants and seeds is small, and the germination rate is low. Moreover, only the Shenzhen population can produce normally developed embryos, while the germination of one- or two-year-old seedlings from the seeds of the other three populations has not been observed. Research on age structure (Wang et al., 2007) shows that the C. fairylakea population is declining. Spatial distribution patterns of the major species in the community (Wang et al., 2009) indicate that the cycad population is a clustered distribution with a high degree of aggregation. Moreover, there is an ecological niche overlap between the C. fairylakea population and the tree layer species in its community (Wang et al., 2006; Sun et al., 2019). In summary, the previous literature concludes that the C. farylakea populations are in decline and in urgent need of conservation efforts, highlighting the need to restore the forest environment in which this species depends for its survival.
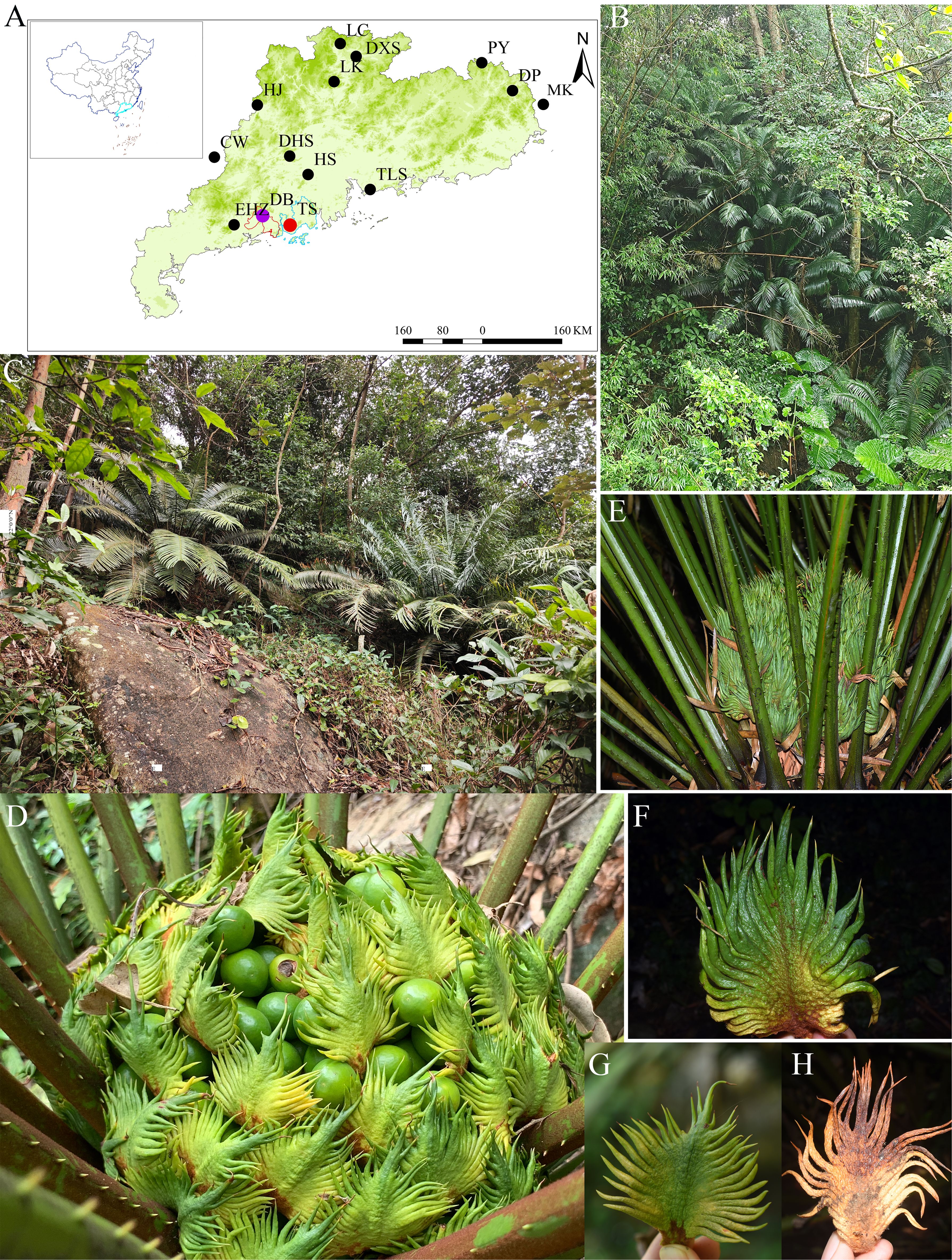
Figure 1. (A) Distribution of Cycas fairylakea in Taishan (TS) and Yangjiang (DB), as well as other distributions in Guangdong Province, China (Figure number: GS (2019)1882); (B, C) Plant morphology and habit of C. fairylakea in DB and TS, respectively; (D, G, H) Female cone bearing seeds and megasporophylls of C. fairylakea; (E, F) Female cone of C. szechuanensis. Photography and cartography: Dan Liang.
Previous populations in Luokeng Town, Qujiang District (LK), and Gulao Town, Heshan City (HS), have seen a decline due to inadequate protection, leaving only a few cultivated individuals and two clusters of female plants, respectively. C. fairylakea has also been identified in other regions, including Lechang City (LC) and Danxia County (DXS) in Shaoguan City, Pingyuan County (PY) and Dabu County (DP) in Meizhou City, Dinghushan National Nature Reserve (DHS) and Huaji County (HJ) in Zhaoqing City, and Ehuangzhang Provincial Nature Reserve (EHZ) in Yangchun City, Guangdong Province. Inhabited areas extend beyond Guangdong to Makeng Village (MK) in Zhao’an County, Fujian Province, and Wuzhou City (CW) in Guangxi Province (Figure 1A). However, according to our field observations and genetic sex determined by the MADS-Y marker, most of these populations (LK, HS, LC, DXS, PY, DP, MK, and CW) consist only of similar-aged and female individuals, and the natural regeneration of seedlings has not been observed in these areas. This leads to the assumption that these populations represent remnants rather than thriving wild populations (Bierzychudek, 2014). In a comprehensive resource survey of Cycads in Guangdong Province, two additional wild populations of C. fairylakea were uncovered in Taishan City (TS) and Yangjiang City (DB) (Figure 1A). This discovery highlights TS and DB as significant wild populations, following those identified at Meilin Reservoir and Tanglangshan Country Park (TLS) in Shenzhen. Our field survey estimates nearly 450 individuals in Taishan and over 2000 in Yangjiang. Moreover, our survey of local villagers in the two distributions revealed that during times of scarcity, C. fairylakea was utilized for sustenance, with its stems processed into powder or sliced for consumption. Historically, the species thrived in the understory and along forest edges at elevations below 100 m before the advent of Eucalyptus forestry in Taishan. The surviving Taishan C. fairylakea community is now primarily found on steep slopes between 150–300 m above sea level, an environment that is less than ideal for the species’ survival. Differences are observed among the Yangjiang community. The plants are distributed on gentle slopes and between 25–300 m above sea level and are threatened by local forestry (mainly by Cunninghamia laceolata and less by Pinus massoniana and Eucalyptus). Residents also cultivate C. fairylakea for ornamental purposes in their courtyards. Increased awareness and protection efforts have led to a reduction in poaching, as the public recognizes C. fairylakea as a nationally key protected species. Despite this, current populations face challenges such as habitat fragmentation, human disturbance, and threats from invasive plant species. Motivated by these findings, we conducted a study to delve into these two newly discovered populations, focusing on: (1) habitat characteristics; (2) the distribution range, population size, age structure, sex ratio, and spatial distribution patterns; and (3) the impacts of environmental factors on the population size and sex ratio of C. fairylakea, and how the population adapts to external disturbances. The results are of significant value for the in-situ conservation of C. fairylakea, offering well-informed conservation strategies. The study contributes to a comprehensive understanding of the species distribution and habitat, offering essential foundational data and valuable conservation insights for Cycads in China.
1.1 Choice of the scientific name
Cycas fairylakea was denoted as a synonym of C. szechuanensis by Feng et al. (2021) and accepted by IUCN (2024) and Yang (2021). However, in this study, we employed the scientific name C. fairylakea over C. szechuanensis for three principal reasons: (1) the name C. fairylakea is currently listed in the national key protected plants register (State Forestry and Grassland Administration and the Ministry of Agriculture and Rural Affairs, P. R. China, 2021), which underscores its relevance and recognition in conservation efforts; (2) previous research on C. szechuanensis and C. fairylakea encountered issues such as the lack of available voucher specimens and incomplete or inaccessible sample collections (Wang, 2019; Feng et al., 2021). which hindered the comprehensive understanding and accurate classification of these species; and (3) field observations have revealed distinct differences in the morphology of the macrosporophylls and female cones between C. szechuanensis and C. fairylakea. C. szechuanensis is characterized by its dispersed and spiny macrosporophylls, which do not form layered wraps and are ovate in their overall shape. The terminal segments are either inconspicuous or often skewed to one side, sometimes making them indistinguishable from the lateral segments. The lateral lobes are arranged in a crowded fashion with the upper and lower lobes similar in length and an uneven distal axial surface of the macrosporophylls (Figures 1E, F). In contrast, C. fairylakea exhibits macrosporophylls that are tightly wrapped into globular forms, with the overall shape of the macrosporophylls resembling rhombuses or triangles that are wide at the base and narrow at the top. The lateral lobes are easily distinguishable from the terminal lobes, and their length tapers gradually from the base upwards. The distal axial surface of the macrosporophylls in C. fairylakea is notably smooth (Figures 1D, G, H). These clear morphological distinctions justify our choice of C. fairylakea as the scientific name for the species in question, reflecting a commitment to taxonomic accuracy and facilitating targeted conservation strategies for this unique Cycad.
2 Materials and methods
2.1 Study area
Guangdong Province is located in southern China (20°09′–25°31′ N, 109°45′–117°20′ E) and is known for its diverse geography and subtropical climate. It boasts a long coastline along the South China Sea, with numerous rivers, plains, and mountain ranges. The climate is characterized by warm temperatures (average annual temperature of 18–24°C) and abundant rainfall (annual precipitation of 1300–2500 mm), encompassing a humid subtropical zone affected by typhoons. The soil type of Guangdong is mainly red, reddish, and yellow soils. Taishan and Yangjiang City are adjacent to each other in southwest China. They are bordered by the South China Sea to the south and have a subtropical oceanic monsoon climate characterized by prevailing southerly winds in the summer and northerly winds in the winter. Vegetation types mainly include northern tropical monsoon rainforests, southern subtropical evergreen broad-leaved forests, mid-subtropical typical evergreen broad-leaved forests, and coastal mangroves.
2.2 Plot setting and survey
Based on our field survey of the Cycas fairylakea distribution, we established ten sampling plots (50 m × 50 m), five on the mountains of Taishan City (plot code: TSS), five on Yangdong District (plot code: DBS), Yangjiang City. Within these plots, we conducted a thorough investigation and recorded the diameter at breast height (DBH) and height of all tree species with DBH ≥ 2.0 cm in the plots. In addition, we documented the presence of all plant species and noted habitat factors such as elevation (ELE), slope degree (SD), and slope aspect (SA) for each plot. Each encountered individual of C. fairylakea was carefully tagged, localized and sampled. The stem length was precisely measured to ascertain the population size and age class. The samples were then utilized for sex identification, with the recorded locations adopted for further observations and spatial point pattern analysis.
To obtain a detailed assessment of the soil conditions within our study plots, we collected soil samples from diverse topographical positions using a ring knife. Three samples were obtained at a depth of 30 cm below the ground on each plot on the upper, middle, and lower slopes. In addition, 30 samples were collected to test pH (NY/T 1121.2-2006), organic matter (OM: NY/T 1121.6-2006), total nitrogen (TN: HJ717-2014), total phosphorus (TP: LY/T 1232-1999), total potassium (TK: LY/T 1234-2015), alkaline dissolved nitrogen (AN: LY/T1229-1999), quick-acting phosphorus (AP: LY/T 1232-2015) and quick-acting potassium (AK: LY/T 1234-2015).
2.3 Community characteristics and phylogenetic diversity
The abundance, relative abundance, frequency, relative frequency, DBH, relative dominance, species richness (SR), importance value (IV), and α-diversity indices (P-Simpson’s dominance index, H-Shannon–Wiener’s variation index, and E-evenness index) were calculated based on previous literature (Fang et al., 2009).
The plant species list was organized according to the survey results. Based on the macro phylogenetic tree of seed plants constructed by Zanne et al. (2014), the species and their family information were input into the plant genealogy software Phylomatic (Webb and Donoghue, 2005) to obtain the evolutionary relationships among species, and the bladj module of the Phylocom 4.2 (Webb et al., 2008) package was used to add branch lengths to the resulting evolutionary tree. The phylogenetic diversity index (PD) was calculated using the pd module of Phylocom 4.2. Moreover, the net relative index (NRI), nearest taxonomic unit coefficients (NTI), and PD residuals were calculated using the construct module of Phylocom 4.2 for the two communities.
2.4 Environmental factors
A total of 40 environmental factors were investigated (Supplementary Tables 1). Factors including topography (SD, SA, and ELE), climate, soil, and community diversity indices from each plot were recorded as topography factors, and the slope aspect was converted from 0°–360° to 0–1 using the TRASP index (Roberts and Cooper, 1989). The 19 bioclimatic variables were obtained from WorldClim version 2 (Fick and Hijmans, 2017). The eight soil properties (Supplementary Tables 2) were also used to compute the soil fertility (S) (Supplementary Tables 3, 4) (Zhang et al., 2015; Jiang et al., 2023) of the sample sites based on the affiliation function and principal component analysis (PCA) (Zhang et al., 2015; Feng et al., 2018). Canoco 5.0 (Šmilauer and Lepš, 2014) was used to perform redundancy analysis (RDA) of species (number of individuals of each age class and sex ratio) and environmental factors. Significant differences in the topography, soil properties, and community diversity indices between the two communities were tested using independent samples t-tests in SPSS 26.0 (IBM, SPSS Inc.).
2.5 Population characteristics
2.5.1 Genetic sex ratio
Primers were designed based on the complete sequences of MADS-box transcription factors in Cycads (Liu et al., 2022; Gong et al., 2022). The MADS-Y gene sequence for sex obtained for C. fairylakea was amplified using PCR. The sex was determined based on images of agarose gel electrophoresis, with positive results indicating that the plant was male (Supplementary Figure 1). The primer sequences were designed as follows: MSY-3F, AAGAAGGCGCACGAACTCTC; MSY-3R, CGTGGACAGCGAATTGAGGT. DNA extraction and amplification were conducted by Beijing Tsingke Biotech Co., Ltd. Sex ratios were calculated separately for each age class. The chi-square (χ2) test was employed to examine the number of individuals of both sexes deviating from the 0.5 level (i.e., the ratio of individuals of a particular sex to all individuals) using SPSS 26.0.
2.5.2 Population age structure, static life table, and survival curves
The stem length measured for all C. fairylakea individuals in the study area was used to classify the ages into the following classes (Jian et al., 2005a): A (older individuals), stem length ≥ 1 m; B (sub-aged individuals), 50 cm ≤ stem length< 1 m; C (adult individuals, which can produce female and male cones), 25 cm ≤ stem length< 50 cm; D (young individuals, which generally cannot produce female and male cones), 5 cm ≤ stem length< 25 cm, with the stem obviously outside the soil; and E (newly emerged seedlings), stem length< 5 cm. Based on the data from individuals of the five age classes, we compiled a static life table using the space-for-time method and calculated the survival rate (Si), cumulative mortality rate (Fi), mortality density (fi), and hazard factor (λi) (Pielou, 1977; Silvertown, 1982; Jiang, 1992; Li et al., 2018). The data were then fit to Deevey’s type II and III survival curves (Hett and Loucks, 1976) in SPSS 26.0.
2.5.3 Spatial patterns
Based on the coordinate information of the distribution of the C. fairylakea individuals, the univariate g1,1(r) and bivariate g1,2(r) functions of the point pattern analysis were used to analyze the spatial distribution pattern among all sampled individuals and between male and female individuals in ten sampling plots. Analysis was performed using Progamita 2014 (Wiegand and Moloney, 2014) with a circle width of 1 m. The upper and lower envelope trajectories were calculated after 100 Monte-Carlo test fits to obtain 99% confidence intervals. The univariate g1,1(r) and bivariate g1,2(r) functions were calculated as follows:
where K(r) is Ripley’s K function (Besag and Diggle, 1977; Lotwick and Silverman, 1982; Diggle, 1983; Ma et al., 2018; Liu et al., 2019; Ning et al., 2020), and r is the spatial scale distance. The complete spatial randomness (CSR) model was used to test the degree of significance of distributional deviations from the complete randomness assumption (Zhang et al., 2017). When the value of g1,1(r) is above the upper limit of the confidence interval, the population is clustered; between the confidence intervals, the population is randomly distributed; below the lower limit of the confidence interval, the population is randomly distributed. When the value of g1,2(r) is above the upper limit of the confidence interval, males and females are positively correlated; below the lower limit of the confidence interval, they are negatively correlated; and between the confidence intervals, they are independent of each other (Yan et al., 2010).
3 Results
3.1 Ecological traits
3.1.1 Community characteristics and species composition
The species α-diversity values of the tree and shrub layers in the two C. fairylakea communities are shown in Table 1. Simpson’s dominance (P, P > 0.05), Shannon–Wiener’s variation (H, P > 0.05), and evenness (E, P > 0.05) indices indicate insignificant differences in the tree layers between the two communities. Shannon–Wiener’s index in Taishan was significantly lower than that in Yangjiang (P< 0.05), while the evenness index was higher than that in Yangjiang (P< 0.05), and Simpson’s index was equal in both regions (P > 0.05).
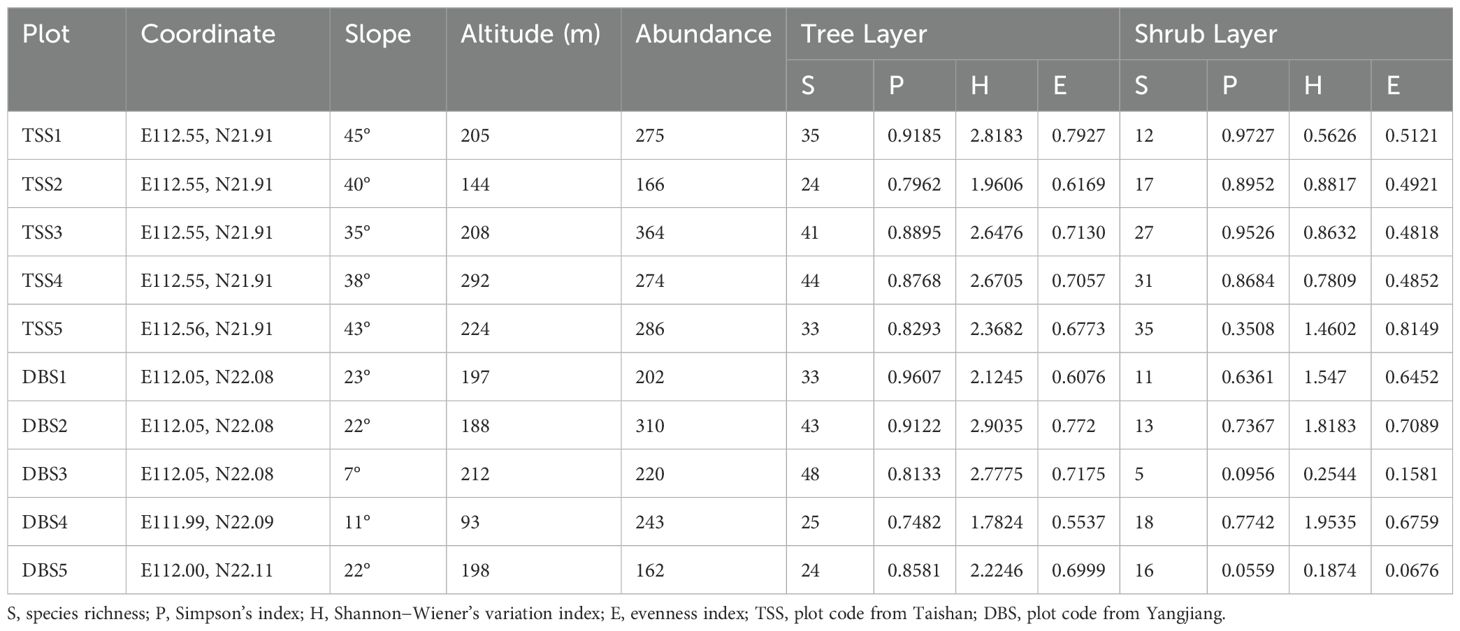
Table 1. Five plots’ species diversity indices of Cycas fairylakea communities in Taishan and Yangjiang.
A total of nine families and 15 species of Lycophytes and ferns, two families and three species of Gymnosperms, and 69 families and 168 species of angiosperms were recorded in the Taishan community of C. fairylakea. In terms of family composition, the Fabaceae family had the greatest number of species (16 species). This was followed by Lauraceae and Rubiaceae, which had 13 and 12 species, respectively. Community types included Garcinia oblongifolia + Mallotus paniculatus − Memecylon nigrescens + Psychotria asiatica − Hedyotis acutangula + Cibotium barometz + Blechnopsis orientalis, which belonged to a typical subtropical evergreen monsoon rainforest broad-leaved forest. The species composition of the five plots in the community was similar, with 76 trees, 28 shrubs, 41 lianas, and 40 herb species. The Yangjiang community of C. fairylakea was rich in plant species, including 10 families and 18 species of Lycophytes and ferns, three families and three species of Gymnosperms, and 64 families and 182 species of angiosperms. This community was characterized by a mix of Litchi chinensis + Canarium album + Dracontomelon duperreanum − Psychotria asiatica + Ardisia quinquegona + Fordia cauliflora −Tectaria simonsii − Leptochilus digitatus − Pandanus austrosinensis, forming a subtropical evergreen monsoon rainforest with planted forests. In terms of family composition, the Rubiaceae family was the most diverse, with 13 species. Moraceae and Fabaceae each had 11 species. The community included 70 tree species, 44 shrub species, 35 lianas species, and 53 herb species. Important values of the top ten species in the two communities are shown in Supplementary Tables 5 and 6.
3.1.2 Phylogenetic diversity
Phylogenetic diversity analysis of the two communities shows that the PD indices, NRI, and NTI were equal to each other (P > 0.05). The NRI and NTI calculated by the null model were both greater than 0 (Table 2), indicating that the phylogenetic structures of the two C. fairylakea communities were aggregated and that most of the species in the community had close affinities and similar habits. The communities were subjected to environmental filtering. The phylogenetic trees of the two communities are shown in Supplementary Figures 2 and 3.
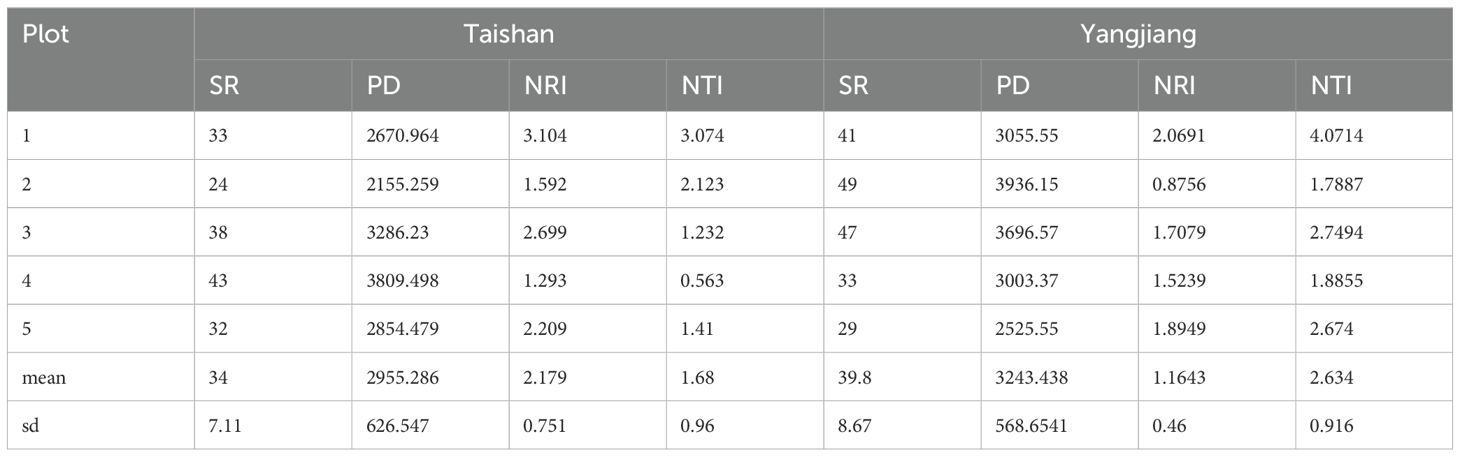
Table 2. Species richness (SR), phylogenetic diversity (PD), net relatedness index (NRI) and net nearest taxa index (NTI).
3.2 Population structure
3.2.1 Genetic sex ratio
The number of individuals with both age–class classification and successful sex detection was 322 (165 females and 157 males) in Taishan, and 1237 (667 females and 570 males) in Yangjiang. The χ2 test results showed that the female-to-male ratio was close to 1:1, and there was a tendency of females-bias in terms of numbers, yet this was not significant (P > 0.05). No preferences were observed at the overall level, across each plot, or within a single age class in Taishan. However, in Yangjiang, a significant female bias was observed in plot 4 (age classes B, C, and E and the total, P< 0.01), plot 1 (age class A, P< 0.05), and for the total individuals of the five plots (P< 0.01). The seedlings (E, P > 0.05) and young individuals (D, P > 0.05) in Yangjiang were sex balanced (Figure 2; Supplementary Tables 7, 8).
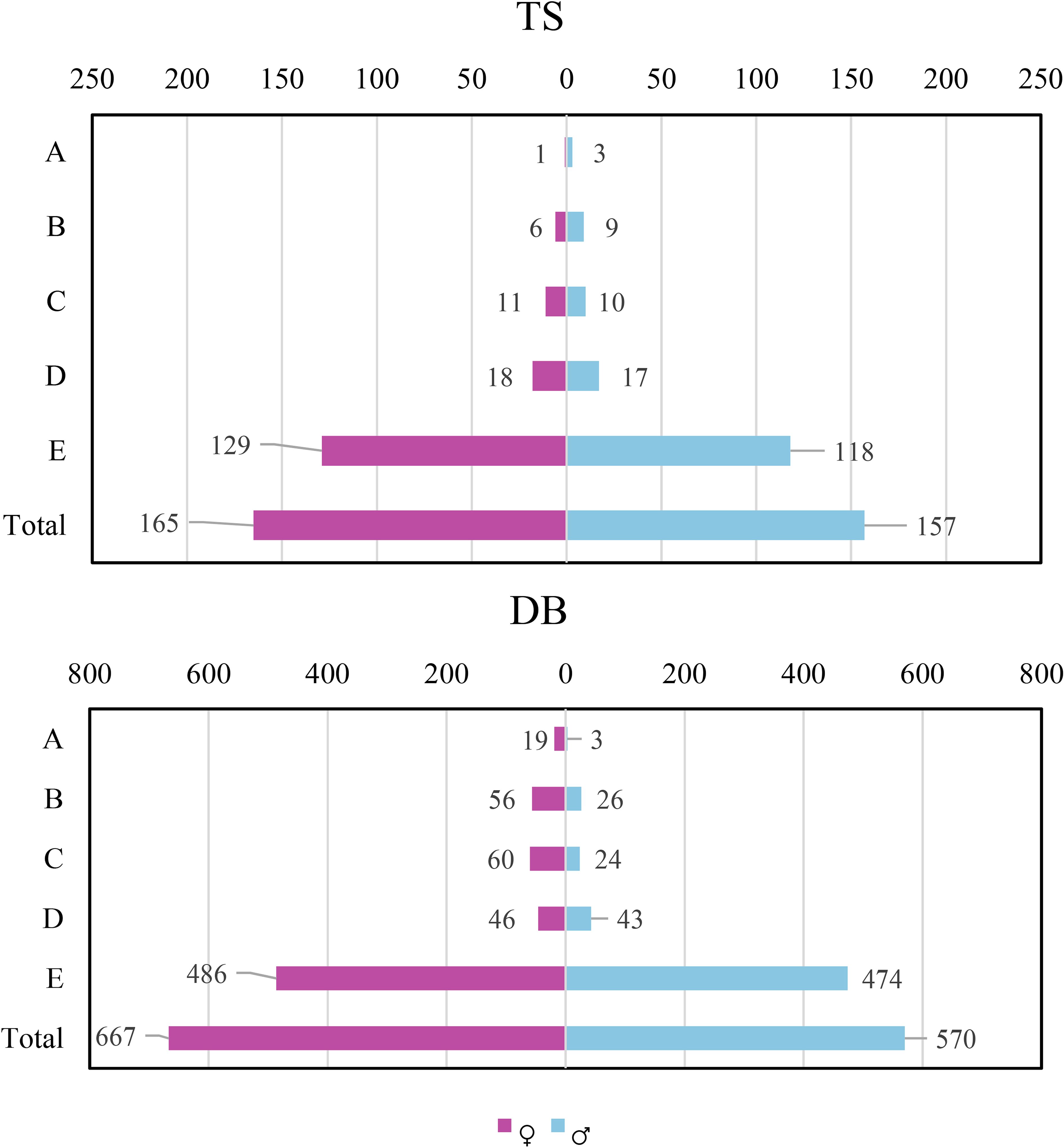
Figure 2. Sex ratio of male and female Cycas fairylakea in Taishan and Yangjiang communities (TS, Taishan, DB, Yangjiang).
3.2.2 Population size and age structure
A total of 450 individuals of C. fairylakea were surveyed within Taishan City, with 401 individuals surveyed within the sample plot. The height of plants growing on cliffs was difficult to measure, and thus only 336 individuals in the five plots (n = 79, 63, 47, 83, 64) could be divided into the five age classes. In Yangjiang, a total of 2091 individuals were surveyed and 1237 were observed in the five sample plots (n = 515, 279, 80, 255, 108), all distributed in Yangdong District. All ten plots from the two populations showed a decrease in the number of individuals as the age level increased, namely, there were more seedlings and fewer individuals in the middle and old ages (Figures 3A, B).
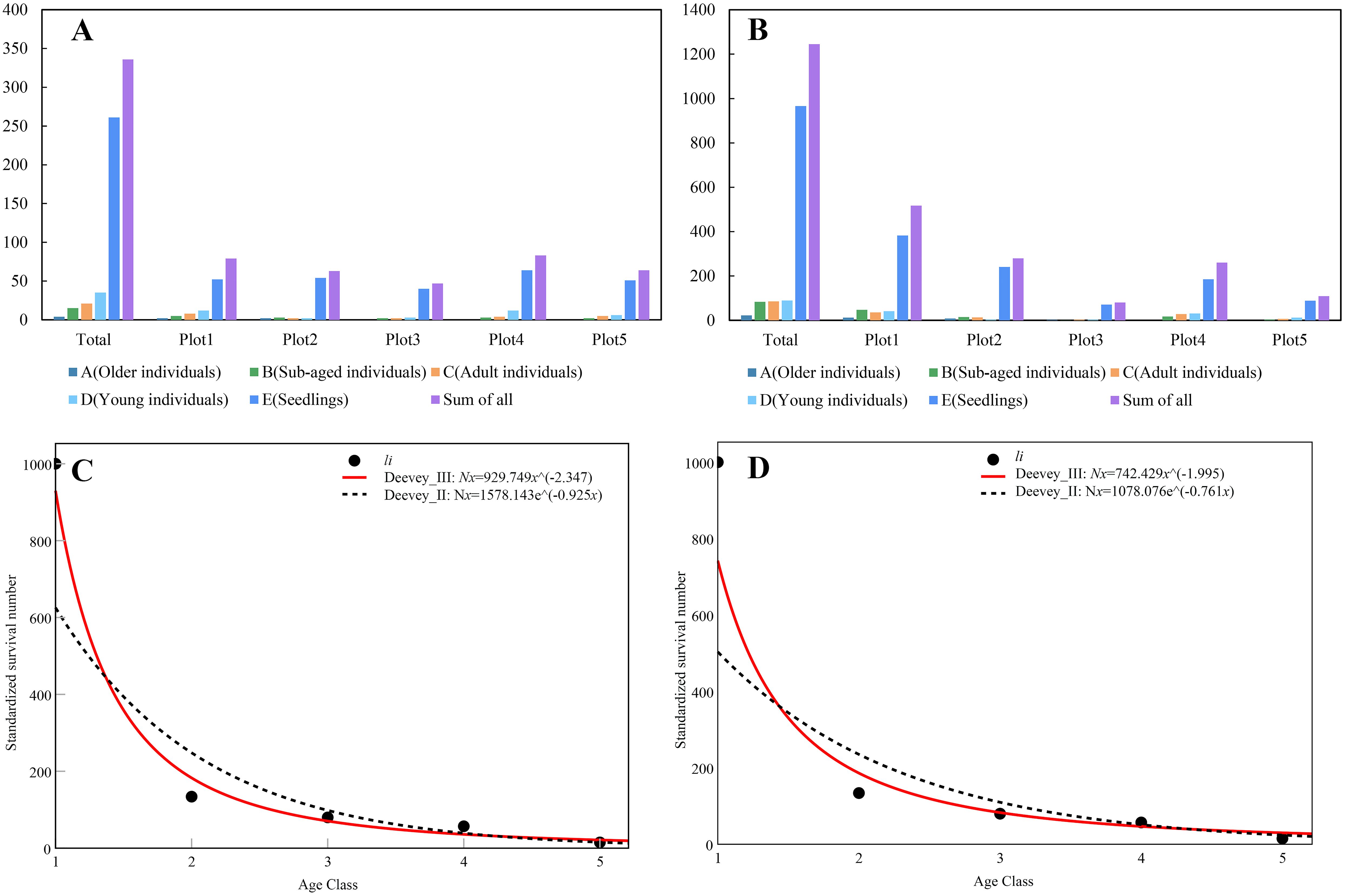
Figure 3. (A, B) Distribution of the number of individuals of different age classes in the five sampling plots from two sample sites (A, Older individuals, stem length ≥ 1 m; B, Sub-aged individuals, 50 cm ≤ stem length< 1 m; C, Adult individuals, 25 cm ≤ stem length< 50 cm; D, Young individuals, 5 cm ≤ stem length< 25 cm; E, Seedlings, stem length< 5 cm); (C, D) Survival curves of the Taishan and Yangjiang populations.
3.2.3 Static life table and survival curves
The number of individuals corresponding to each age class in the five plots was combined to determine the static life table for the two sample sites (Table 3). The table reveals that the sites shared consistent trends in the life patterns of the two populations. The standardized survival number (li) of different age classes varied greatly and decreased gradually with the increase in age classes. The highest mortality rate (qi) was in age class E, followed by age class B. The highest disappearance rate (Ki) was in age class A, followed by age classes E and B. With the increase in age class, the mortality rate and disappearance rate of age classes A–C gradually decreased and subsequently increased. The highest population life expectancy (ei) was in age class D (1.64 and 2.63, respectively), followed by age classes C and E, and reached 1.40 and 1.74 at age class C. As the age increased, the survival rate monotonically decreased while the cumulative mortality rate (Fi) remained high. Moreover, the hazard coefficient (λi) gradually decreased, with age class E exhibiting the highest value, which then decreased abruptly, while a slight upward trend was observed in age class B. The density of deaths (fi) was maximized in age class E, which then stabilized. The following survival models were obtained:
where x is the standardized survival li. The population age structure showed a “reverse-J” shape (Figures 3C, D). Comparing the R values of the correlations and F-test values of the above two models indicates that the survival curves were of Deevey’s type III (Equations 4, 6).
3.3 Spatial distribution patterns
3.3.1 Spatial group patterns
Univariate g-function spatial pattern analysis of the location of individuals from five plots revealed that individuals were clustered at the 0–10 m scale, randomly distributed at the 11–20 m scale (which subsequently transitioned to a uniform distribution in Taishan) (Supplementary Figures 4F–J), and exhibited an aggregated pattern at the 0–25 m scale (which shifted to a uniform pattern at the 26–30 m scale in Yangjiang) (Supplementary Figures 5F–J).
3.3.2 Spatial relationships between male and female populations
Analysis of the spatial relationships between the male and female populations showed that the spatial relationships among them were positively correlated at the scales of 0–10 m in Taishan and 0–25 m in Yangjaing, and independent of each other at scales of 11–25 m in Taishan and 26–30 m in Yangjiang (Supplementary Figures 4, 5K–O). The females and males were interdependent at small spatial scales, and competition for resources was low when the spatial scales were enlarged.
3.4 Relationship between population structure and environmental factors
The RDA ranking plot of the population structure and environmental factors in C. fairylakea communities is shown in Figure 4. TN, TK, AK, Shrub-E, bio2, and bio13 accounted for 99.99% of the total variance in the population size, the number of female and male individuals, and sex ratios in each age class, with the first- and second-ranked axes contributing to 98.26% and 1.44%, respectively, and a cumulative 99.7% of the overall variance explained. In particular, bio13 and Shrub-E contributed to 40.6% (P< 0.05) and 24.6% (P< 0.05) of the variance in the population size, the number of female and male individuals, and sex ratios of the Taishan and Yangjiang C. fairylakea communities. These two environmental factors mainly influenced the individual number and sex ratio of C. fairylakea. TP, Shrub-H, and AP explained 100.0% of the total variance in the Taishan C. fairylakea community, with the first and second sorting axes contributing to 93.41% and 4.95%, respectively. Notably, the explanatory contribution of Shrub-H was 48.3% (P< 0.05). Shrub-E, NTI, and SA explained 100.0% of the total variance in the Yangjiang C. fairylakea community, with the first and second sorting axes contributing to 97.42% and 1.61%, respectively. In particular, NTI contributed to 38.8% (P< 0.05) of the variance.
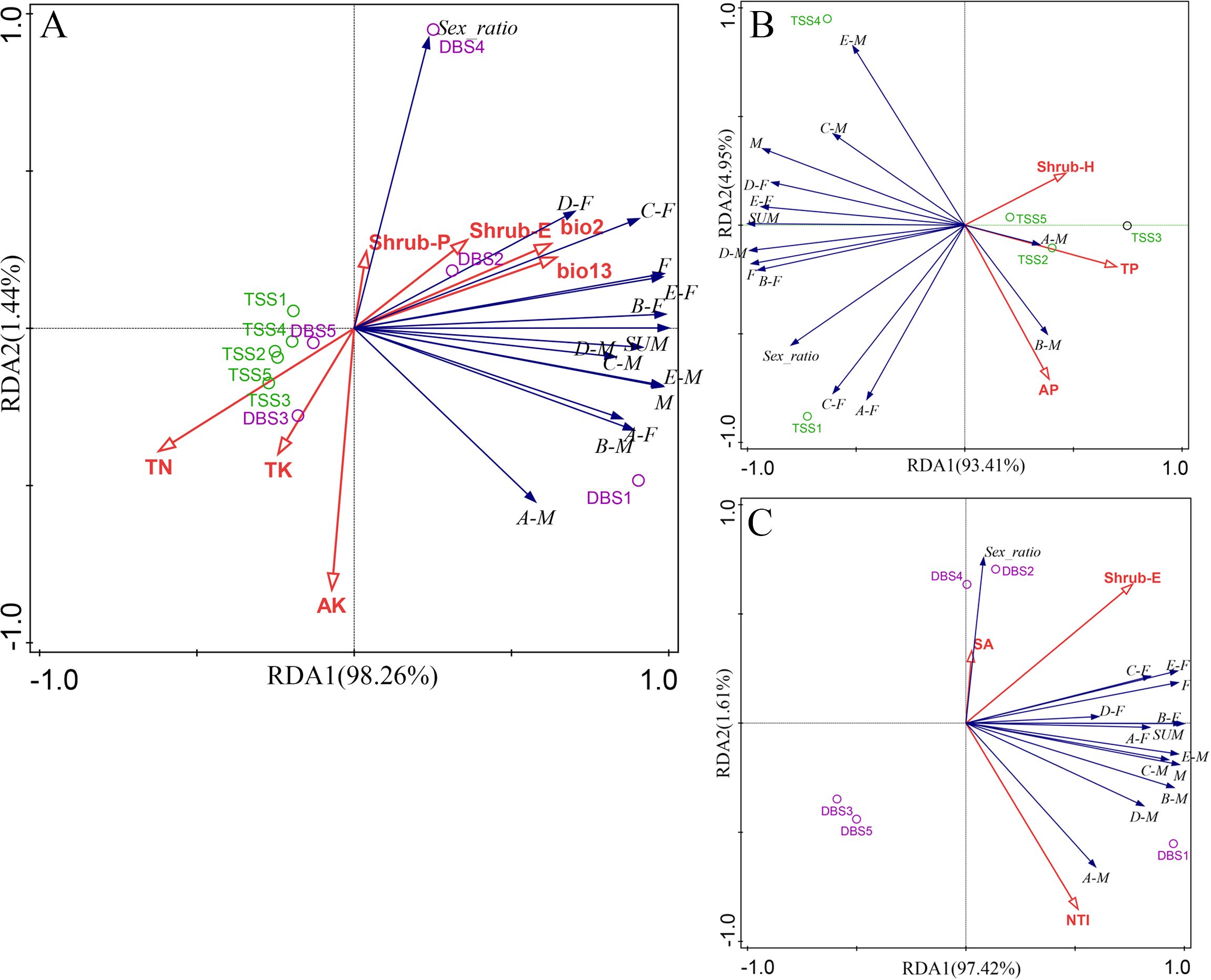
Figure 4. RDA ranking of population size, number of female and male individuals of each age class, and the sex ratio of Taishan and Yangjiang C. fairylakea communities with environmental factors. (A) Combined analysis of Taishan and Yangjiang; (B) Taishan community; (C) Yangjiang community; F, M, A-F, B-F, C-F, D-F, E-F. A-M, B-M, C-M, D-M, E-M, SUM, and Sex_ratio respectively represent female and male number; female number, and male number of each age class, the number of total individuals, and the ratio of females to males. TN, TP, TK, AP, AK, SA, Shrub-H, Shrub-E, NTI, bio2, and bio13 are soil total nitrogen, total phosphorus, total potassium, quick-acting phosphorus, quick-acting potassium, slope aspect, Shannon–Wiener index and evenness index of shrub layers, net nearest species index, mean diurnal range, and precipitation of wettest month, respectively.
4 Discussion
4.1 Ecological traits
Both C. farylakea communities in Taishan and Yangjiang experienced varying degrees of damage due to encroachment from the surrounding plantation forests of Eucalyptus, Cunninghamia, and Pinus. This resulted in two distinct situations for the two populations. In Taishan, C. fairylakea is currently distributed on steep slopes with numerous cliffs and rocks. Its relative isolation from human interference, possibly due to natural barriers, has helped preserve a greater number of individuals in these rugged habitats. However, as previously mentioned, C. fairylakea prefers to grow in moist and fertile soil, and is often found near streams and ditches (Jian et al., 2005a). The habitat of the C. fairylakea in Taishan does not belong to this type, implying that C. fairylakea can adapt to drier and more barren environments. In contrast, in Yangjiang, C. fairylakea mainly inhabits non-commercial forests and valleys with gentle slopes, which is more consistent with the aforementioned habitat. No significant differences were observed in the tree layers of the two new C. fairylakea communities based on the three biodiversity indices (P > 0.05). The average values of the Shannon–Wiener indices (H) were 2.493 ± 0.339 and 2.363 ± 0.468 for Taishan and Yangjiang, respectively, both of which are lower than those of Shenzhen (H = 2.956) (Wang et al., 2004). In contrast, the Shannon–Wiener and evenness indices of shrub layers exhibited significant differences between Taishan (H = 0.91 ± 0.333) and Yangjiang (H = 1.152 ± 0.863) (P< 0.05), with values also lower than those in Shenzhen (H = 3.704). The species numbers and composition differ greatly between the two communities. A total of 186 plant species belonging to 80 families and 143 genera were recorded in Taishan, compared to 203 plant species belonging to 77 families and 157 genera in Yangjiang. There are 76 tree species in Taishan and 70 in Yangjiang, while the number of shrubs (28) and herb species (40) in Taishan is less than that in Yangjiang (44 shrubs and 53 herbs). The number of species in these two communities is not as numerous as in Shenzhen (75 families, 169 genera, and 226 species), yet the number of families and genera is slightly higher (Wang et al., 2004). The greater diversity of plant species at the Taishan sample site is attributed to the presence of native subtropical evergreen broad-leaved forests. In contrast, Yangjiang, which is dominated by secondary growth following the conversion of economic timber and fruit tree forests to non-commercial use, shows lower diversity. However, due to reforestation measures, the vegetation in Yangjiang has been able to survive and develop, resulting in a greater variety of shrubs and herbs. Therefore, the composition of dominant families and species in the two communities is also different. In Taishan, the dominant families are Fabaceae, Lauraceae, and Rubiaceae, with dominant species of Garcinia oblongifolia, Mallotus paniculatus, Heptapleurum heptaphyllum, Sterculia lanceolata, and Acronychia pedunculata. The dominant species in the tree and shrub layers are similar to those in the Shenzhen Cycas fairylakea community (Wang et al., 2004). In Yangjiang, the dominant families are Rubiaceae, Moraceae, and Fabaceae, with dominant species of Cunninghamia lanceolata, Litchi chinensis, Canarium album, Dracontomelon duperreanum, and Canarium pimela, most of which are economic timber species and fruit trees. The development of understory plants makes the understory environment unfavorable for the growth of Cycas fairylakea, due to a lack of light, poor ventilation and overly humid conditions that can lead to diseases. Phylogenetic diversity, a critical measure of biodiversity (Li, 2019), is reported to correlate with community stability and productivity (Cadotte et al., 2012; Cadotte, 2013; Chen et al., 2017). When NRI and NTI are greater than 0, the phylogenetic structure of the species in the sample is aggregated, indicating that the species in the community are mostly closely related and have similar habits, and the community is subject to environmental screening. When NRI and NTI are equal to 0, the phylogenetic structure of the species in the sample is random. When NRI and NTI are less than 0, the phylogenetic structure of the species in the sample is dispersed, the species in the community are more distantly related, and the structure is mainly affected by competition and exclusion (Webb, 2000; Webb et al., 2002; Chen et al., 2017). The PD indices do not show significant variation between the two communities (P > 0.05). The NRI index, which is positive in all plots, indicates a phylogenetic structure that tends toward aggregation, suggesting the community may be less resilient to environmental changes.
We also analyzed eight soil properties from the two sample sites (Supplementary Tables 2). No significant differences were observed in the soil properties between the two communities, except for soil organic matter (OM) (P< 0.05), and all properties exhibited a consistent variation pattern. C. fairylakea was found to thrive in acidic forest red soil, with pH values of 4.48 ± 0.04 (Taishan) and 4.73 ± 0.03 (Yangjiang). Soil organic matter, an important indicator of soil fertility (Zhou et al., 2017), was found to be at a medium level in Taishan (24.81 ± 8.49 g/kg) and Yangjiang (20.26 ± 1.04 g/kg), according to the second national soil classification standards, both of which are lower than that recorded in broad-leaved mixed forests (28.18 ± 2.41 g/kg) by Feng et al. (2018). Soil nutrient content, particularly effective nitrogen, phosphorus, and potassium, is indicative of soil fertility and its capacity to support plant growth (Li et al., 2022). The two sample sites exhibit high levels of alkaline dissolved nitrogen (AN), extremely low levels of quick acting-phosphorus (AP), and medium levels of quick-acting potassium (AK). The levels of total nitrogen (TN) and total phosphorus (TP) are low and extremely low, respectively, while total potassium (TK) is extremely high. The comprehensive soil fertility scores, calculated through PCA and the affiliation value function, ranged from 0.32 to 0.66 in Taishan and from 0.37 to 0.55 in Yangjiang (Supplementary Tables 3, 4), showing no significant differences between the two communities (P > 0.05). Previous work by Wang et al. (2005) on the breadth of soil resource utilization within the ecological niche for the C. fairylakea population in Tanglangshan Country Park, Shenzhen, indicated that the population reached its highest density under specific soil conditions, including soil OM (3.348%–3.382%), TN (0.164%–0.186%), and AP (2.092–2.868 mg/kg) and AK (108.34–137.28 mg/kg). The levels of soil OM, AP, and AK were lower in Taishan and Yangjiang than those in Tanglangshan Country Park, Shenzhen, suggesting a potential need for targeted soil enrichment strategies to support the C. fairylakea community in Taishan and Yangjiang.
4.2 Characterization of populations
The sex ratio, defined as the proportion of females to males within a population or age class, is pivotal for the stable progression of dioecious plant populations. A balanced sex ratio is essential for maintaining a robust population structure and dynamics (Fisher, 1930; Ma et al., 2018). The sex ratio in dioecious plants can fluctuate based on environmental factors such as moisture, nutrients, light, temperature, and disturbance (Yin and Li, 2007). Female plants are typically more prevalent in conditions of high moisture or nutrient availability, while male plants dominate in arid or nutrient-poor environments (Freeman et al., 1976; Dawson and Bliss, 1989; Dawson and Ehleringer, 1993). This pattern suggests a female bias in favorable conditions and a male bias under stress. Advancements in genomic data for the Cycas genus have enabled the precise determination of sex in unflowering C. fairylakea, with accuracy reaching 100% (Liu et al., 2022). Our analysis of five plots within the C. fairylakea community in Taishan revealed a sex ratio that is not skewed toward either gender. This contrasts with previous studies on Cycadaceae and Zamiaceae, which have reported varying sex ratios, including male-biased (Kono and Tobe, 2007; Yang et al., 2014; Segalla et al., 2021), female-biased (Newell, 1983; Mora et al., 2013), and balanced (He et al., 1995; Watkinson and Powell, 1997; Yu et al., 2007) scenarios. The inconsistency in these conclusions may stem from differences in observation time scales or the impact of population disturbances. In the case of C. fairylakea in Taishan, there is a noted scarcity of adult female plants. Interestingly, most cultivated adult plants found in Town were female, suggesting a historical preference for harvesting female plants for ornamental use. This selective harvesting may have contributed to the current sex ratio imbalance in the wild population. Moreover, one plot showed female bias (P< 0.05) in Yangjiang, resulting in female bias in age classes A, B, and C and as a whole.
The age structure of a population is a critical indicator of its dynamics and overall health. In this study, we determined the age classes of C. fairylakea using stem length measurements, a method consistent with that of Wang et al. (2007). Age structure analysis revealed a predominance of juvenile individuals and a scarcity of adults in the two wild populations of C. fairylakea in Taishan and Yangjiang. This disparity suggests a high mortality rate among seedlings, with a significant number failing to transition successfully from the juvenile to the youth phase. This challenge may be attributed to various habitat-related factors, such as rocky terrain, a thin soil layer, and seeds germinating in crevices in the Taishan population. Other contributing factors include light availability, predation by animals, diseases, and pests. Our findings indicate that as age classes progress from class E to C, the mortality and disappearance rates gradually decrease. However, an increasing trend in these rates among older age classes suggests a potential decline in the adult plant population due to ongoing environmental pressures. This trend paints a concerning picture of a population with an overabundance of seedlings and a dwindling number of mature individuals. The highest life expectancy (ei) was observed in the age class D, followed by the age classes C and A. This suggests that the population’s physiological activities are most robust during these stages, particularly as the plants enter the middle age class. At this point, life expectancy reaches 1.40 in Taishan and 1.64 in Yangjiang, indicating that C. fairylakea has reached individual maturity and possesses the resilience to withstand environmental pressures and competition. The age structure and static life table of C. fairylakea reflect its basic survival pattern. The data indicate that these populations are not in a state of healthy and stable development. In contrast to the findings of Taishan and Yangjiang, the age structure of the populations in Shenzhen, Heshan, Luokeng, and Zhangshi are inversely pyramid-shaped (Wang et al., 2007), with very few seedlings in their natural state. After conservation measures including artificial nurturing, the number of C. fairylakea in Shenzhen exceeds 5,000 individuals, and the number of seedlings exceeds 4,000 (Fu and Liu, 2017). The juvenile stage emerges as the most precarious period for survival. Therefore, once individuals successfully navigate this vulnerable phase, their life activities stabilize, suggesting a capacity for resilience and adaptation in the face of adversity.
Seedling competition is a common phenomenon in nature (Lewis and Tanner, 2000; Barberis and Tanner, 2005; Ding et al., 2016). Competition among seedlings can occur for various resources, such as light, water, nutrients, and growing space. This competition may lead to some seedlings not obtaining sufficient resources, resulting in poor growth or even death. In this fiercely competitive environment, weaker seedlings may be more susceptible to pests and diseases, thereby increasing the risk of death. In addition, under the pressure of natural selection, seedlings with poor adaptability may also be eliminated, a mechanism used by the population to maintain health and diversity. In this study, we also conducted point spatial pattern analysis using the geographic distribution of C. fairylakea individuals. The data, which were recorded in latitude and longitude coordinates, were first converted into Cartesian coordinates using the SoDA package in R (Chambers, 2008) before analysis, and then analyzed in Progamita (Wiegand and Moloney, 2014). Our results revealed that C. fairylakea exhibited an aggregated distribution pattern at small scales (0–10 m), a phenomenon largely attributed to the plant’s large and heavy seeds, which fall and germinate close to the mother plant due to gravity (Bell, 2019) (Supplementary Figures 4, 5). This is consistent with the distribution pattern of the Shenzhen population (Wang et al., 2009) and may lead to high mortality in seedlings. Environmental heterogeneity, particularly the presence of numerous large rocks in the mountains of Taishan, also played a role in this aggregation. Seeds often lodge in rock crevices, occupying specific microhabitats that are less accessible to many angiosperms due to the thin and narrow soil layer (Vovides, 1990). This natural barrier helps C. fairylakea avoid competition with flowering plants through mechanisms such as vine entanglement, shading by tall canopies, and impeded ventilation. In Yangjiang, seeds were observed to fall onto the gentle slopes to form a larger scale aggregation (0–25 m). As the scale increases, the distribution of C. fairylakea transitions from aggregated to random or uniform, a pattern indirectly confirmed by our analysis. The spatial relationship between male and female plants demonstrated a positive correlation at small scales (0–10 m in Taishan and 0–25 m in Yangjiang), suggesting the potential facilitation of pollination. As the scale increases, this correlation first becomes independent and then shifts to a negative correlation at larger scales (11–25 m in Taishan and 26–30 m in Yangjiang), which may correlate with the pollination distances typical of Cycads. Research indicates that the reproductive success of dioecious plants such as Cycads is positively correlated with population size (Cabral and Schurr, 2010). In smaller populations, the effective pollination distance is shorter, ensuring pollination success within confined areas. This dynamic is crucial for female plants to successfully pollinate, bear fruit, and thus contribute to the population’s successful reproduction. The aggregated distribution of C. fairylakea, coupled with the positive spatial correlation between male and female individuals, suggests that the species can effectively manage competitive pressures. This dynamic ensures the population’s continuous growth and the potential for a gradual expansion of its distribution range.
According to the RDA ranking results, the C. fairylakea population in Taishan is distributed in areas with less environmental heterogeneity and under adverse conditions, strongly influenced by the soil TN, followed by the influence of TK and AK, while the Yangjiang population has a more variable habitat. Soil TN, TK, and AK are key factors that constrain the individual development of C. fairylakea, and they are negatively correlated with both the number of individuals and the sex ratio of each age class. Among these, TN and TK are the dominant factors that limit the development of female individuals in each age class. AK exhibits a positive correlation with the number of male individuals in the older (A) and sub-aged (B) age classes, and a moderate increase in AK will have a positive impact on the individual numbers in age classes A and B. For the Taishan population, the Shannon–Wiener biodiversity index of the shrub layer (Shrub-H), TP, and AP have a negative impact on the number of individuals and sex ratio of all age classes, except for A and B male individuals. Among these, the negative impact of Shrub-H on the sex ratio is the strongest, TP is negatively correlated with the number of male individuals, female seedlings, and seedlings (E) individuals, and AP has a negative impact on the number of male seedlings. For the Yangjiang population, NTI, SA, and the evenness indices (Shrub-E) of the shrub layer have a positive impact on the number of individuals in each age class of the population, with SA having a slight impact on the sex ratio. The combined effect of soil conditions and community characteristics somewhat influences the population structure of C. fairylakea, and the Taishan population is affected by soil conditions significantly.
4.3 Protection recommendations
The population of C. fairylakea exhibits a balanced sex ratio, which is instrumental in preserving its genetic diversity. Nonetheless, the community in Taishan faces challenges, including moderate soil fertility and ecosystem degradation, alongside a concerning decline in its age structure. In light of these issues, we propose the following conservation recommendations for C. fairylakea: (1) Habitat protection: Prioritize the protection of habitats essential for C. fairylakea’s survival. We recommend that the forestry department expedite the creation of conservation areas and restrict the encroachment of plantation forests. (2) Artificial tending: Implement artificial tending practices to improve the habitats. This includes judicious fertilization to improve soil nutrient levels, the strategic opening of canopy gaps to allow more light penetration, and the removal of dense ground cover and bryophytes that may compete with the Cycads for resources. (3) Seedling regeneration: Foster seedling regeneration by closely monitoring the fruiting status of female individuals. Employ ex situ conservation methods to enhance seed germination and seedling survival rates, thereby bolstering the next generation of the population. (4) Conservation genetics research: Conduct research into the conservation genetics of C. fairylakea to elucidate its genetic structure and diversity. Such research will provide valuable insights and inform scientific strategies for the identification and protection of significant conservation units of this species. These recommendations are designed to address the immediate and long-term needs of the C. fairylakea populations in Taishan and Yangjiang.
Data availability statement
The original contributions presented in the study are included in the article/Supplementary Material. Further inquiries can be directed to the corresponding author.
Author contributions
DL: Formal analysis, Investigation, Methodology, Software, Writing – original draft, Writing – review & editing. YMW: Data curation, Investigation, Writing – review & editing. P’AW: Investigation, Methodology, Writing – review & editing. XTL: Investigation, Writing – review & editing. PHW: Investigation, Writing – review & editing. YJL: Investigation, Writing – review & editing. SYZ: Investigation, Writing – review & editing. RJW: Funding acquisition, Resources, Supervision, Writing – review & editing.
Funding
The author(s) declare financial support was received for the research, authorship, and/or publication of this article. This research was funded by Guangdong Provincial Key R&D Programme “The Conservation and Utilizaiton of the Important Strategic Wild Plant Resource in Guangdong Province (Grant No. 2022B1111040003)” and the Guangdong Flagship Project of Basic and Applied Basic Research (2023B0303050001). The project was supported by the Forestry Bureau of Guangdong Province and the Forestry Bureau of Taishan and Yangjiang City.
Acknowledgments
We are grateful to Mr. Zhang Shouzhou, researcher of Fairy Lake Botanical Garden, Shenzhen & Chinese Academy of Sciences, Shenzhen, China, for his comments and suggestions during the preparation of this paper, to Mr. Lin Jiangbin, Sichuan Cycas panzhihuaensis National Nature reserve, panzhihua, China, for providing the usage method of the Progamita software, and to Mr. Liu Yueyao, Mr. Chen Yuqiang, Miss Chen Sisi, Mr. Peng Yuanhang and Miss Gong Lingling, for their help in the fieldwork.
Conflict of interest
Author DL was employed by Nanning Qingxiushan Scenic and Historic Tourism Development Co., Ltd.
The remaining authors declare that the research was conducted in the absence of any commercial or financial relationships that could be construed as a potential conflict of interest.
Publisher’s note
All claims expressed in this article are solely those of the authors and do not necessarily represent those of their affiliated organizations, or those of the publisher, the editors and the reviewers. Any product that may be evaluated in this article, or claim that may be made by its manufacturer, is not guaranteed or endorsed by the publisher.
Supplementary material
The Supplementary Material for this article can be found online at: https://www.frontiersin.org/articles/10.3389/fevo.2024.1490107/full#supplementary-material
Supplementary Figure 1 | Molecular marker electrophoresis images of Cycas fairylakea, using DL2000 Plus DNA Marker as a control, with male individuals displaying bands and female individuals not displaying bands.
Supplementary Figure 2 | Phylogenetic tree of plant species in Taishan.
Supplementary Figure 3 | Phylogenetic tree of plant species in Yangjiang.
Supplementary Figure 4 | Spatial patterns of the C. fairylakea population based on complete spatial randomness (CSR) of five plots in Taishan. (A–E) indicate the individual distribution points, ○, female individuals, ▲, male individuals, and X, unknown gender individuals. (F–J) indicate the spatial patterns. (K–O) indicate the spatial association of the male and female individuals of five plots. Solid and dashed lines denote the g(r) value and 99% confidence envelopes, respectively.
Supplementary Figure 5 | Spatial patterns of the C. fairylakea population based on complete spatial randomness (CSR) of five plots in Yangjiang. (A–E) indicate the individual distribution points, ○, female individuals, ▲, male individuals, and X, unknown gender individuals. (F–J) indicate the spatial patterns. (K–O) indicate the spatial association of the male and female individuals of five plots. Solid and dashed lines denote the g(r) value and 99% confidence envelopes, respectively.
References
Abraham A., Mathew P. M. (1962). Cytological studies in the Cycads: sex chromosomes in Cycas. Ann. Bot. 26, 261–266. doi: 10.1093/oxfordjournals.aob.a083792
Barberis I. M., Tanner E. V. J. (2005). Gaps and root trenching increase tree seedling growth in Panamanian semi-evergreen forest. Ecology 86, 667–674. doi: 10.1890/04-0677
Barrett S. C. H., Yakimowski S. B., Field D. L., Pickup M. (2010). Ecological genetics of sex ratios in plant populations. Philos. Trans. R. Soc B Biol. Sci. 365, 2549–2557. doi: 10.1098/rstb.2010.0002
Bell S. (2019). Macrozamia flexuosa C. Moore (Zamiaceae): a review of distribution, habitat and conservation status of an endemic cycad from the Hunter Region of New South Wales. Cunninghamia 19, 7–27. doi: 10.7751/cunninghamia.2019.19.002
Besag J., Diggle P. J. (1977). Simple Monte Carlo tests for spatial pattern. Appl. Stat. 26, 327. doi: 10.2307/2346974
Bierzychudek P. (2014). Plant biodiversity and population dynamics. In: Monson R. (eds) Ecology and the Environment. The Plant Science, vol 8 (New York, NY: Springer). doi: 10.1007/978-1-4614-7501-9_15
Cabral J. S., Schurr F. M. (2010). Estimating demographic models for the range dynamics of plant species. Glob. Ecol. Biogeogr. 19, 85–97. doi: 10.1111/j.1466-8238.2009.00492.x
Cadotte M. W. (2013). Experimental evidence that evolutionarily diverse assemblages result in higher productivity. Proc. Natl. Acad. Sci. 110, 8996–9000. doi: 10.1073/pnas.1301685110
Cadotte M. W., Dinnage R., Tilman D. (2012). Phylogenetic diversity promotes ecosystem stability. Ecology 93, 223–233. doi: 10.1890/11-0426.1
Chambers J. (2008). Software for Data Analysis: Programming with R (New York, NY: Springer New York). doi: 10.1007/978-0-387-75936-4
Chen Y. Q., Zhu S. S., Wang G. T., Wen X. Y., Huang X. X., Zhou L. X., et al. (2017). Phylogenetic diversity analysis of the community of extremely small populations of Glyptostrobus pensilis. Plant Sci. J. 35, 667–678. doi: 10.11913/PSJ.2095-0837.2017.50667
CITES (2021).Appendices of convention on international trade of endangered species of wild fauna and flora. Available online at: https://cites.org/eng/app/appendices.php (Accessed July 8, 2024).
Dawson T. E., Bliss L. C. (1989). Patterns of water use and the tissue water relations in the dioecious shrub, Salix arctica: the physiological basis for habitat partitioning between the sexes. Oecologia 79, 332–343. doi: 10.1007/BF00384312
Dawson T. E., Ehleringer J. R. (1993). Gender-specific physiology, carbon isotope discrimination, and habitat distribution in Boxelder, Acer Negundo. Ecology 74, 798–815. doi: 10.2307/1940807
Dellaporta S. L., Calderon-Urrea A. (1993). Sex determination in flowering plants. Plant Cell 5, 1241–1251. doi: 10.1105/tpc.5.10.1241
Ding Y., Zang R., Letcher S. G., Liu W., Lu X. (2016). Aboveground and belowground competition affect seedling performance and allometry in a tropical monsoon forest. New For. 47, 529–540. doi: 10.1007/s11056-016-9529-5
Edwards A. W. F. (2000). The genetical theory of natural selection. Genetics 154, 1419–1426. doi: 10.1093/genetics/154.4.1419
Fang J. Y., Wang X. P., Shen Z. H., Tang Z. Y., He J. S., Yu D., et al. (2009). Methods and protocols for plant community inventory. Biodivers. Sci. 17, 533–548. doi: 10.3724/SP.J.1003.2009.09253
Feng J. Y., Chu S. S., Wang J., Wu D. M., Mo Q. F., Zeng S. C. (2018). Comprehensive evaluation of soil fertility of five typical forest stands in South China. J. South China Agric. Univ. 39, 73–81. doi: 10.7671/j.issn.1001-411X.2018.03.012
Feng X. Y., Wang X. H., Chiang Y. C., Jian S. G., Gong X. (2021). Species delimitation with distinct methods based on molecular data to elucidate species boundaries in the Cycas Taiwaniana complex (Cycadaceae). TAXON 70, 477–491. doi: 10.1002/tax.12457
Fick S. E., Hijmans R. J. (2017). WorldClim 2: new 1-km spatial resolution climate surfaces for global land areas. Int. J. Climatol. 37, 4302–4315. doi: 10.1002/joc.5086
Fisher R. A. (1930). The genetical theory of natural selection (Oxford: Clarendon Press). doi: 10.5962/bhl.title.27468
Freeman D. C., Klikoff L. G., Harper K. T. (1976). Differential resource utilization by the sexes of dioecious plants. Science 193, 597–599. doi: 10.1126/science.193.4253.597
Fu Q. F., Liu M. H. (2017). National first-class protected plants: Cycas fairylakea rescued (Beijing: China Agricultural Science and Technology Press).
Gong Y. Q., Zhang S. Z., Wei T., Liu H., Yang L. L. (2022).Molecular markers, primer pairs and their applications, and methods for determining the sex of Cycads. Available online at: https://kns.cnki.net/kcms2/article/abstract?v=LeTZRn7a1NIZz2Y4gnJwbuME_DVoQGMub8rMSebcjcSV5RbxSNbsywIPTTHKt3TjMgcRgYFHzWOPTjgy7FirEx2-qPa9S1SGVsg1ADupXDbw0iLGRF6TSc-Zj1KqUKPVOj10a-cIFOA=&uniplatform=NZKPT&language=CHS (Accessed July 8, 2024).
Hamrick J. L., Godt M. J. W. (1996). Effects of life history traits on genetic diversity in plant species. Philos. Trans. Biol. Sci. 351, 1291–1298. doi: 10.1098/rstb.1996.0112
He Y. H., Wang Q., Shi P. L. (1995). Biological properties, trunk anatomy and growth patterns of Cycas panzhihuaensis. Acta Bot. Sin. 37, 443–451.
Hett J. M., Loucks O. L. (1976). Age structure models of balsam fir and eastern hemlock. J. Ecol. 64, 1029. doi: 10.2307/2258822
IUCN (2024).The IUCN red list of threatened species. Available online at: https://www.iucnredlist.org/en (Accessed October 16, 2024).
Jian S. G., Liu N., Gao Z. Z., Wei Q., Xie Z. H., Wu M., et al. (2005a). Biological characteristics of wild Cycas fairylakea population in Guangdong Province. Acta Sci. Nat. Univ. Sunyatseni 44, 97–100.
Jian S. G., Wei Q., Gao Z. Z., Xie Z. H., Lin S. H., Liu N. (2005b). Characteristics and conservation of wild populations of Cycas fairylakea newly found in Qujiang of Guangdong Province. Guihaia 25, 97–101.
Jiang H. (1992). Studies on the Spruce (Picea asperata) Population Ecology (Beijing: China Forestry Publishing House).
Jiang B., Wang S. T., Sun Z. B., Zhang H. R., Wang J., Liu Y. (2023). Evaluation of cultivated land soil fertility based on membership function and principal component analysis. Chin. Agric. Sci. Bull. 39, 22–27. doi: 10.11924/j.issn.1000-6850.casb2022-0063
Kono M., Tobe H. (2007). Is Cycas revoluta (Cycadaceae) wind- or insect-pollinated? Am. J. Bot. 94, 847–855. doi: 10.3732/ajb.94.5.847
Lewis S., Tanner E. (2000). Effects of above- and belowground competition on growth and survival of rain forest tree seedlings. Ecology 81, 2525–2538. doi: 10.2307/177472
Li S. C. (2019). Plant species diversity and community phylogenetic structure in Xisha Islands and Wanshan Islands (Guangzhou: South China Botanical Garden, Chinese Academy of Sciences).
Li R. B., Deng H. T., Bai K. L. (2022). Physical and chemical properties of soil in subtropical montane evergreen broad-leaved forest———A case study of Nankunshan Mountain. For. Inventory Plan. 47, 149–152+171. doi: 10.3969/j.issn.1671-3168.2022-02.024
Li W. Y., Li X., Gan X. H. (2018). Population structure and dynamics of endangered plant Tetracentron sinense. Subtrop. Plant Sci. 47, 222–228. doi: 10.3969/j.issn.1009-7791.2018.03.006
Liu Y., Wang S., Li L., Yang T., Dong S., Wei T., et al. (2022). The Cycas genome and the early evolution of seed plants. Nat. Plants 8, 389–401. doi: 10.1038/s41477-022-01129-7
Liu M. X., Xia S. J., Nan X. N., Li Q. D., Jiang X. X. (2019). Distribution pattern of Caragana roborovskyi population based on Ripley’s K(r) function. Arid Zone Res. 36, 606–613. doi: 10.13866/j.azr.2019.03.10
Lotwick H. W., Silverman B. W. (1982). Methods for analysing spatial processes of several types of points. J. R. Stat. Soc Ser. B Methodol. 44, 406–413. doi: 10.1111/j.2517-6161.1982.tb01221.x
Ma S. W., Liu G. H., Liu L. H., Zhang C., Liu G. Z., Guo J., et al. (2018). Sex ratio and spatial distribution of Salix gordejevii in Hunshandake Sandy Land. Acta Ecol. Sin. 38, 7708–7717. doi: 10.5846/stxb201711021960
Mora R., Yáñez-Espinosa L., Flores J., Nava-Zárate N. (2013). Strobilus and seed production of Dioon edule (Zamiaceae) in a population with low seedling density in San Luis Potosí, Mexico. Trop. Conserv. Sci. 6, 268–282. doi: 10.1177/194008291300600208
Nagalingum N. S., Marshall C. R., Quental T. B., Rai H. S., Little D. P., Mathews S. (2011). Recent synchronous radiation of a living fossil. Science 334, 796–799. doi: 10.1126/science.1209926
Newell S. J. (1983). Reproduction in a natural population of cycads (Zamia pumila L.) in Puerto Rico. Bull. Torrey Bot. Club 110, 464–473. doi: 10.2307/2996280
Ning M., Li N., Shi Q. D., Guo Y. C. (2020). A Ripley’s K function-based study of the spatial pattern of Populus euphratica population in Daliyaboyi oasis in desert hinterland. J. Southwest Univ. Nat. Sci. Ed. 42, 73–82. doi: 10.13718/j.cnki.xdzk.2020.12.009
Obeso J. R. (2002). The costs of reproduction in plants. New Phytol. 155, 321–348. doi: 10.1046/j.1469-8137.2002.00477.x
Qin H. N., Yang Y., Dong S. Y., He Q., Jia Y., Zhao L. N., et al. (2017). Threatened species list of China’s higher plants. Biodivers. Sci. 25, 696–744. doi: 10.17520/biods.2017144
Roberts D. W., Cooper S. V. (1989). Concepts and Techniques of Vegetation Mapping (New York: USDA Forest Service).
Segalla R., Pinheiro F., Barônio G. J., Morellato L. P. C. (2021). Male-biased effective sex ratio across populations of the threatened Zamia Boliviana (Zamiaceae). Plant Ecol. 222, 587–602. doi: 10.1007/s11258-021-01127-3
Šmilauer P., Lepš J. (2014). Multivariate Analysis of Ecological Data using CANOCO 5. 2nd Edn (Cambridge: Cambridge University Press). doi: 10.1017/CBO9781139627061
State Forestry and Grassland Administration and the Ministry of Agriculture and Rural Affairs, P. R. China (2021). List of Wild Plants Under State Protection. (Beijing: the State Council of the People's Republic of China).
Sun Y. J., Chen X. X., Fu Q. F., Gong Y. Q., Kuang J. H., Li N., et al. (2019). Niche characteristics of dominant species of Cycas fairylakea community in Meilin reservoir, Shenzhen. J. Cent. South Univ. For. Technol. 39, 63–70. doi: 10.14067/j.cnki.1673-923x.2019.11.010
Vovides A. (1990). Spatial distribution, survival, and fecundity of Dioon edule (Zamiaceae) in a tropical deciduous forest in Veracruz, Mexico, with notes on its habitat. Am. J. Bot. AMER J. Bot. 77, 1532–1543. doi: 10.2307/2444486
Wang X. H. (2019). Population Genetics of the Cycas Taiwaniana Complex (Guangzhou: South China Botanical Garden, Chinese Academy of Sciences).
Wang D. P., Chen F. P., Li B. F., Ji S. Y. (2006). Niche characteristics of dominant populations in Cycas fairylakea community. Chin. J. Ecol. 25, 399–404. doi: 10.13292/j1000-4890.2006.0078
Wang D. P., Ji S. Y., Chen F. P. (2009). The spatial distribution pattern of main populations in Cycas fairylakea community. J. Southwest China Norm. Univ. (Natural Sci. Ed. 34, 93–97. doi: 10.13718/j.cnki.xsxb.2009.01.010
Wang D. P., Ji S. Y., Chen F. P., Peng S. L. (2005). Niche breadth of Cycas fairylakea genet and clone population on soil resource. Ecol. Environ. 14, 913–916. doi: 10.16258/j.cnki.1674-5906.2005.06.024
Wang D. P., Ji S. Y., Chen F. P., Peng S. L. (2007). Age estimation and age structure of Cycas fairylakea population in Shenzhen City. Chin. J. Appl. Ecol. 17, 476–480.
Wang F. X., Liang H. B., Chen T. Q., Wang D. Y. (1996). Cycads in China (Guangzhou: Guangdong Science and Technology Press).
Wang D. P., Wang C. Y., Ji S. Y., Xing F. W., Peng S. L. (2004). Community characteristics of Cycas fairylakea, an endemic and endangered species. J. Beijing For. Univ. 26, 12–18. doi: 10.13332/j.1000-1522.2004.06.003
Wang D. P., Xing F. W., Ji S. Y., Chen F. P. (2003). A report on the wild Cycas fairylakea population. Chin. Wild Plant Resour. 22, 19–20.
Watkinson A. R., Powell J. C. (1997). The life history and population structure of Cycas armstrongii in monsoonal northern Australia. Oecologia 111, 341–349. doi: 10.1007/s004420050244
Webb C. O. (2000). Exploring the phylogenetic structure of ecological communities: an example for rain forest trees. Am. Nat. 156, 145–155. doi: 10.1086/303378
Webb C. O., Ackerly D. D., Kembel S. W. (2008). Phylocom: software for the analysis of phylogenetic community structure and trait evolution. Bioinforma. Oxf. Engl. 24, 2098–2100. doi: 10.1093/bioinformatics/btn358
Webb C. O., Ackerly D. D., McPeek M. A., Donoghue M. J. (2002). Phylogenies and community ecology. Annu. Rev. Ecol. Evol. Syst. 33, 475–505. doi: 10.1146/annurev.ecolsys.33.010802.150448
Webb C. O., Donoghue M. J. (2005). Phylomatic: tree assembly for applied phylogenetics. Mol. Ecol. Notes 5, 181–183. doi: 10.1111/j.1471-8286.2004.00829.x
Wiegand T., Moloney K. A. (2014). Handbook of Spatial Point-Pattern Analysis in Ecology. 0 Edn (Boca Raton: CRC Press). doi: 10.1201/b16195
Yan H. B., Han Y. Z., Yang X. Q., Wang L. Y., Xiang X. Y. (2010). Spatial distribution patterns and associations of tree species in typical natural secondary mountain forest communities of Northern China. Acta Ecol. Sin. 30, 2311–2321.
Yang Y. (2021). An updated red list assessment of gymnosperms from China (Version 2021). Biodivers. Sci. 29, 1599. doi: 10.17520/biods.2021342
Yang Y., Liu B., Njenga M. D. (2017). Red list assessment and conservation status of gymnosperms from China. Biodivers. Sci. 25, 758–764. doi: 10.5846/stxb201604180723
Yang Z. S., Yang Y. Q., Yang Y. (2014). Comprehensive investigation report of Sichuan Cycas panzhihuaensis National Nature Reserve (Beijing: China Forestry Publishing House).
Yin C. Y., Li C. Y. (2007). Gender differences of dioecious plants related sex ratio: recent advances and future prospect. Chin. J. Appl. Environ. Biol. 13, 419–425.
Yu Z. X., Yang Y. Q., Liu J., Gong L. L., Zhan C. L. (2007). Preliminary experimenton propagation of Cycas panzhihuaensis. J. Southwest For. Coll. 27, 36–41.
Zanne A. E., Tank D. C., Cornwell W. K., Eastman J. M., Smith S. A., FitzJohn R. G., et al. (2014). Three keys to the radiation of angiosperms into freezing environments. Nature 506, 89–92. doi: 10.1038/nature12872
Zhang P. J., Qing H., Zhang L., Xu Y. D., Mu L., Ye R. H., et al. (2017). Population structure and spatial pattern of Caragana tibetica communities in Nei Mongol shrub-encroached grassland. Chin. J. Plant Ecol. 41, 165–174. doi: 10.17521/cjpe.2015.0448
Zhang K. X., Shang K. K., Da L. J. (2015). Soil quality comprehensive assessment of different plant communities in Shanghai green belt. J. Nanjing For. Univ. Nat. Sci. Ed. 39, 71–77. doi: 10.3969/j.issn.1000-2006.2015.03.014
Keywords: Cycas fairylakea, community characteristics, phylogenetic diversity, sex ratio, spatial pattern
Citation: Liang D, Wei Y, Wang P, Liu X, Wang P, Liu Y, Zeng S and Wang R (2024) Comparative ecological traits and environmental responses of two distinct populations of the critically endangered Cycas fairylakea in Guangdong, China. Front. Ecol. Evol. 12:1490107. doi: 10.3389/fevo.2024.1490107
Received: 02 September 2024; Accepted: 04 November 2024;
Published: 22 November 2024.
Edited by:
Manel Leira, University of Santiago de Compostela, SpainReviewed by:
Qiang Fan, Sun Yat-sen University, ChinaAnders Lindstrom, Nong nooch botanical garden, Thailand
Copyright © 2024 Liang, Wei, Wang, Liu, Wang, Liu, Zeng and Wang. This is an open-access article distributed under the terms of the Creative Commons Attribution License (CC BY). The use, distribution or reproduction in other forums is permitted, provided the original author(s) and the copyright owner(s) are credited and that the original publication in this journal is cited, in accordance with accepted academic practice. No use, distribution or reproduction is permitted which does not comply with these terms.
*Correspondence: Ruijiang Wang, d2FuZ3JqQHNjYmcuYWMuY24=
†These authors have contributed equally to this work and share first authorship