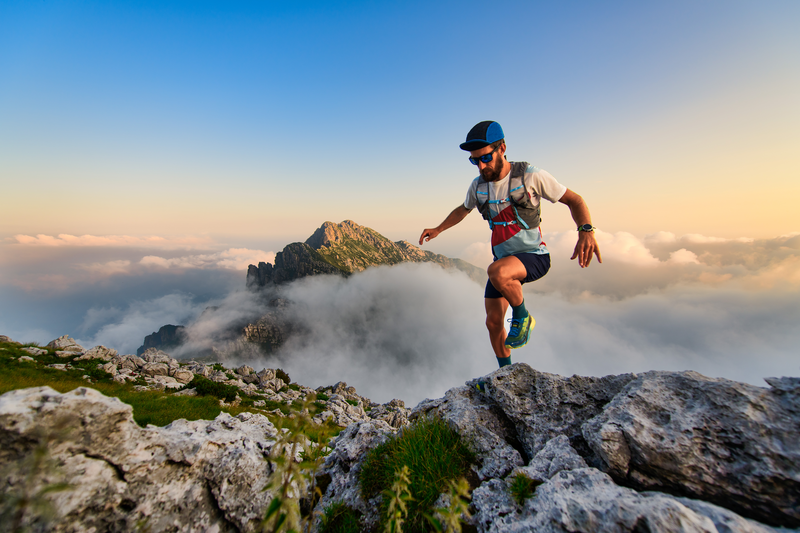
94% of researchers rate our articles as excellent or good
Learn more about the work of our research integrity team to safeguard the quality of each article we publish.
Find out more
HYPOTHESIS AND THEORY article
Front. Ecol. Evol. , 03 January 2025
Sec. Behavioral and Evolutionary Ecology
Volume 12 - 2024 | https://doi.org/10.3389/fevo.2024.1481903
This article is part of the Research Topic Ecology, Evolution, and Diversity of Papionini Primates View all articles
Modern papionin monkeys are a diverse group that encompasses a broad range of morphologies, behaviors, and ecologies. A fossil genus known from African Plio-Pleistocene deposits, Parapapio, is widely regarded as a candidate ancestor to later African papionins. However, despite general agreement that this genus sits at or near the base of the African papionin clade, the taxonomy within Parapapio remains highly contentious. This project evaluates the species-level taxonomy of Parapapio with an explicit hypothesis-based approach to interpreting morphological variation in this sample of fossils. We tested two hypotheses: (H1) the craniodental variation within Parapapio does not cluster into three groups that reflect the three known species, and (H2) all the Parapapio fossils can be accommodated within the craniodental shape and size variation observed for a single extant species of papionin. To test the first hypothesis, we assessed a subset of relatively complete and well-preserved Parapapio crania (n=16), intentionally without reference to previous taxonomic identifications. Specimens were sorted by similarity in cranial features and results were then compared with published taxonomic classifications. Our results demonstrate that morphological traits do not cluster consistently according to the current species categories within Parapapio, failing to reject our first hypothesis. To test our second hypothesis, we examined variation in cranial and dental metrics within Parapapio (n=64) relative to three extant papionin samples (n=310). Our results fail to reject the hypothesis that all Parapapio specimens could belong to a single species and suggest that the three-species paradigm does not reflect the anatomical variation of this genus. We recommend subsuming all Parapapio specimens within Parapapio broomi, the species name with taxonomic priority. The results of this hypothesis-testing approach to taxonomy carry substantial implications for the taxonomy of Parapapio, as well as for biochronological and paleoecological studies more generally, including the taxonomy and paleobiology of hominids recovered from these same deposits.
From the highland-dwelling chattering gelada baboons (Theropithecus gelada) to the snow-dwelling cooing Japanese macaques (Macaca fuscata), modern papionins span a diversity of morphologies, behaviors, and ecologies across their geographic range, which extends from southern Africa to Indonesia (Rowe and Myers, 2016). Morphological and molecular studies have largely resolved the phylogenetic relationships among this diverse crowd of extant papionins, which includes Macaca, Papio, Lophocebus, Theropithecus, Rungwecebus, Cercocebus, and Mandrillus (Gilbert et al., 2009; Perelman et al., 2011; Pozzi et al., 2014). However, relationships between fossil papionins and their modern counterparts have proven more difficult to ascertain, at least in part because of challenges posed by rampant homoplasy and critical gaps in the fossil record (Gilbert, 2013; Pugh and Gilbert, 2018; Brasil et al., 2023).
Molecular studies estimate that all modern papionins shared a common ancestor ca. 7–13 million years ago (Ma; Perelman et al., 2011; Liedigk et al., 2014; Pozzi et al., 2014). Following their split from Macaca, the African papionin lineages began to diverge ca. 5–8 Ma (Perelman et al., 2011). Morphological evidence combined with biogeographic data point to a candidate ancestor for African papionins: Genus Parapapio (Szalay and Delson, 1979; Jablonski, 2002). Parapapio is widely regarded as a stem or basal African papionin genus, with early populations preceding and perhaps ancestral to all African papionins emerging in the Pliocene and Pleistocene (Szalay and Delson, 1979; Frost, 2001; Jablonski and Frost, 2010; Gilbert, 2013; Pugh and Gilbert, 2018). However, despite the general agreement that this genus sits at or near the base of the African papionin clade, the taxonomy within Parapapio remains contentious, confounding the evolutionary origins of the papionin clade. In an attempt to bring more clarity to papionin evolution, we revisit the species-level taxonomy of South African Parapapio with a new method: an explicit hypothesis-testing approach. Before we detail our approach to reassessing the taxonomy within this genus, we provide a brief review of the history of Parapapio, as its rich history of study helps to contextualize the current taxonomic debate and the need for a fresh approach.
The genus Parapapio was named by Jones (1937) to describe a set of fossils recovered from the site of Sterkfontein, South Africa, all of which he placed in a new type species, P. broomi, in honor of South African paleontologist Robert Broom. Today, following decades of subsequent field and museum research, five Parapapio species are recognized. These species collectively span eastern and southern Africa from the Late Miocene to Early Pleistocene (reviewed in Jablonski, 2002 and Jablonski and Frost, 2010). The earliest taxa are known from the Late Miocene and Early Pliocene of eastern Africa (Leakey et al., 2003; Gilbert et al., 2010; and see Benefit et al., 2008 for possible appearance in northern Africa), around what appears to have been the first period of diversification within the genus, near the Miocene–Pliocene boundary (Pickford et al., 1992; Kullmer et al., 2011). Following a southward range expansion during the Pliocene, Parapapio underwent a second period of even greater diversification (Freedman, 1957, 1960, 1961, 1965; Maier, 1970, 1971; Freedman and Stenhouse, 1972; Keyser, 1991; Gommery et al., 2008; Monson et al., 2015). These fossil data demonstrate that prior to ca. 3 Ma, Parapapio was more abundant in eastern than southern Africa, whereas from the latest Pliocene onward, the genus dominates the southern Africa cercopithecoid fossil record (reviewed in Jablonski, 2002; Jablonski and Frost, 2010).
Two Parapapio species are known exclusively from eastern Africa: P. lothagamensis and P. ado (Hopwood, 1936; Leakey et al., 2003). P. lothagamensis is the oldest, smallest, and most basal papionin currently recognized, whose morphology has prompted the suggestion that this species deserves a unique generic rank (Gilbert, 2013). This early papionin species is known from the Late Miocene to earliest Pliocene sediments at Lothagam and possibly from additional sites in Kenya (Leakey et al., 2003; Harrison, 2011). Parapapio ado is a Pliocene species known from Laetoli, Lothagam, and possibly from Kanapoi and Allia Bay [Frost et al. (2020); note that Harrison (2011) suggests the specimens from the latter two sites are better aligned with P. lothagamensis]. The morphological definition of this species remains vague, unfortunately because of a lack of well-preserved facial morphology in the hypodigm (Jablonski and Frost, 2010; Harrison, 2011; Gilbert, 2013).
Three species of Parapapio are known from the Plio-Pleistocene of South Africa: P. broomi, P. jonesi, and P. whitei (Table 1 and Figure 1). Of these, P. broomi and P. whitei are exclusive to South African deposits. Both are known from Makapansgat and Sterkfontein (with the largest samples from the latter; Freedman, 1976; Jablonski, 2002; Heaton, 2006; Jablonski and Frost, 2010; Frost et al., 2022). Parapapio jonesi is known from both eastern and southern Africa, with eastern African Pliocene occurrences at Hadar and Middle Awash, and Plio-Pleistocene occurrences at the South African sites of Sterkfontein, Swartkrans, Kromdraai, and Makapansgat (White et al., 1984; Frost and Delson, 2002; Jablonski, 2002; Jablonski and Frost, 2010; Frost et al., 2022).
Table 1. Morphological features and site occurrences for Genus Parapapio and the three hypothesized South African species.
Figure 1. Holotypes of the three South African Parapapio species. From left to right: female P. whitei mandible (STS 563), male P. broomi cranium (STS 564), and female P. jonesi cranium (STS 565). Shown in anterior (top row), right lateral (center row), and superior (bottom row) views. Anterior is to the right in lateral and superior views. Scale is the same for all.
Parapapio broomi was the first species to be named (by Jones, 1937), followed a few years after by the reciprocal honorific naming of P. jonesi by Broom (1940) alongside the naming of P. whitei after “Mr. A. G. White,” Broom’s assistant in his exploration of the caves (Broom, 1940:93). The naming of P. jonesi by Broom (1940) was, by Broom’s account, “[returning] the compliment” that Jones had made three years prior—a decision made by Broom based on a cursory examination and without any detailed analysis of the type specimens (Thackeray et al., 2019:2). Given that the naming of these species may well have been rooted more in niceties than in biology, it is not surprising that the taxonomy of these three South African species has been the subject of an ongoing and lively decades-long debate (centering on the number of species recognized and where to draw the boundaries between them, e.g., Broom and Hughes, 1949; Freedman, 1957; Maier, 1970; Freedman, 1976; Thackeray and Myer, 2004; Williams et al., 2007; Fourie et al., 2008; Thackeray et al., 2019). We now turn to an overview of the anatomical distinctions used to differentiate these three species of Parapapio.
Parapapio is a moderately sized papionin distinguished largely by aspects of its facial morphology, in light of a general acknowledgement that its dental morphology is indistinguishable from Papio (Table 1; Eisenhart, 1974; Szalay and Delson, 1979; Jablonski, 2002). The most diagnostic trait of the genus is the lack of an anteorbital drop, with a lateral profile that is straight or only slightly concave. The temporal lines are weak, and the supraorbital torus is also weakly developed, lacking a prominent glabella and adjacent ophryonic groove. The maxillary ridges are also weak, and the maxillary and mandibular fossae are absent or weakly excavated. The muzzle is wide and relatively long, and the zygomatic arches are slender. The molars and premolars are typical of papionins, with no uniquely diagnostic characteristics for this genus. According to published descriptions, there is only slight dental sexual dimorphism outside of the canine–premolar honing complex (Freedman, 1957; Eisenhart, 1974; Heaton, 2006).
We note that the descriptions provided above, and the descriptive terminology used throughout this paper, follow from published descriptions of Parapapio morphology. Many of these terms are vague and imprecise (e.g., “weakly developed”, “robust”, etc.), particularly when used without specifying to which specimen or taxon the morphology is being compared. We employ these terms here to maintain consistency with the literature but recognize that some of them are unsatisfactory for meaningful and unambiguous morphological description.
While the diagnostic morphological criteria at the genus level are relatively clear and useful for distinguishing Parapapio from other fossil papionins, the within-genus taxonomy is less well-defined. Original species definitions for South African Parapapio were based almost exclusively on absolute dental dimensions, which at the time of discovery, appeared to cluster in three distinct size groups (Broom, 1940). The subsequent recovery of fossils that bridged the gaps between the three size morphs, combined with a lack of clear species-level apomorphies, has resulted in repeated reevaluation of Parapapio species validity and subsequent production of alternative taxonomic schemes (e.g., Freedman, 1957; Maier, 1971; Eisenhart, 1974; Heaton, 2006; Gilbert, 2007). We now provide a brief review of this taxonomic history.
Following Jones’ (1937) naming of Parapapio, in which he placed a set of fossils from Sterkfontein into the single species P. broomi, Broom (1940) later divided these fossils across three species of Parapapio based primarily on absolute dental size. Broom (1940) assigned the name P. whitei for the larger individuals, the name P. jonesi for the smaller ones, and kept the medium-sized individuals in the type species, P. broomi. In a following publication (Broom, 1948), he also reassigned some Papio fossils from Taung to a fourth Parapapio species, P. antiquus [making use of Haughton’s (1925) species name, originally named as Papio antiquus, but placing the species within Parapapio; see Freedman (1957) for a review of this early history]. P. antiquus, known only from Taung, was regarded as the most morphologically distinctive among the Parapapio species (Jablonski, 2002; Gilbert, 2007). In his assessment of Sterkfontein Parapapio, Heaton (2006) proposed that P. antiquus represented a subspecies of P. broomi. In the following year, Gilbert (2007) reassigned P. antiquus to the new genus Procercocebus on the basis of synapomorphies shared with extant Cercocebus and Mandrillus. Here, we interpret South African Parapapio to include the three species that are currently recognized by most researchers as belonging to this genus: P. whitei, P. broomi, and P. jonesi (see holotypes in Figure 1).
In the decades following the initial publications establishing Genus Parapapio and its constituent species, additional fossils were recovered and assigned to the three size-based species from a number of other South African deposits (Freedman, 1957, 1960, 1961, 1965; Maier, 1970; Freedman and Stenhouse, 1972). The three species taxonomic scheme, originally based primarily on dental size differences, has been mostly followed despite considerable discussion regarding species validity (Freedman, 1957; Szalay and Delson, 1979; Jablonski, 2002; Jablonski and Frost, 2010).
Following the initial descriptions (i.e., Jones, 1937; Broom, 1940), subsequent studies based on newly-recovered fossils reported additional cranial and dental differences between the species (Freedman and Stenhouse, 1972; Freedman, 1976). Eisenhart (1974) demonstrated continuous variation in molar measurements among Parapapio specimens spanning the three species from Sterkfontein and Makapansgat and suggested a series of diagnostic cranial criteria for taxonomic identification. More recently, Heaton (2006) reevaluated Parapapio from Sterkfontein and suggested an expanded species concept for P. broomi, including P. whitei as a junior synonym, although other researchers maintain P. whitei as a distinct and valid taxon (Jablonski and Frost, 2010; Gilbert, 2013; Pugh and Gilbert, 2018).
The fossils discussed herein derive from the South African sites of Sterkfontein, Makapansgat, Swartkrans, Bolt’s Farm, and Taung, often referred to collectively as the “Cradle of Humankind.” Controlling the stratigraphic, taphonomic, and chronological context of fossils from these deposits has been and continues to be challenging, to say the least. The accumulation and depositional histories of the fossils is often complex (e.g., Clarke, 2006; Kibii, 2007; Adams et al., 2007) and occurs over time spans that are long enough to inevitably conflate assemblages into palimpsests in which time-averaging affects many aspects of the assemblage (e.g., Hunt, 2004; O’Regan and Reynolds, 2009; Hopley and Maslin, 2010). The degree to which assemblages are time averaged, or stratigraphic associations between assemblages (or individual fossils) are preserved, is the subject of research and debate at many sites. This challenge fundamentally affects the capacity to interpret evolutionary and environmental changes within and between assemblages or deposits. Even in circumstances where detailed stratigraphic studies have been conducted alongside fine excavation practices, there is significant potential for biochronological, stratigraphic, and chronometric data to conflict [e.g., Member 2 at Sterkfontein; summarized in Stratford and Crompton (2021)].
Most of the fossils yielded from Cradle of Humankind sites before the 1990s were recovered from poorly controlled contexts, including breccia dumps created by the lime mining process (Tobias and Hughes, 1969; Latham et al., 2007), fossils excavated through destructive blasting methods (Broom, 1949), and stratigraphically insensitive excavations. In addition to natural processes that facilitate palimpsest formation and stratigraphic complexity in these caves, anthropogenic processes relating to mining and excavation, and by association fossil collection, have caused potentially significant artificial packaging and lumping of assemblages that are very difficult to disentangle retrospectively. The papionins considered here, and in other relevant studies, have complex depositional, chronological, taphonomic, and collection histories. These aspects and their potential influence on taxonomic assessment should be acknowledged.
Variation in specimen preservation, critical gaps in the fossil record, and a complicated taxonomic history have obscured the evolutionary history and taxonomic reality of Parapapio. It is increasingly clear that a reevaluation and systematic revision of Parapapio is needed (Szalay and Delson, 1979; Jablonski, 2002; Jablonski and Frost, 2010). Maier’s (1970:70) commentary on species definitions for South African Pleistocene cercopithecids remains as relevant today as it was over fifty years ago: “The species, however, are still a kind of working model, reflecting the morphological diversity of the fossil material, but possibly being far from representing natural units.”
During the earlier part of the twentieth century in human evolutionary studies, the discovery of new fossils led to the naming of new taxa (e.g., Australopithecus africanus, Plesianthropus transvaalensis, and Zinjanthropus boisei; Broom, 1938; Dart, 1925; Leakey, 1959). Considering the scientific context at the time, this increase in species names was to be expected, as very little was known about the human past until these fossils were recovered. Of course, limited fossil samples represent isolated specimens, leaving significant gaps in the fossil record. Scattered across time and space, these gaps can make fossils appear more distinct from one another than they may have actually been while creating convenient boundaries that can be drawn between taxa (Wood and Boyle, 2016). With increased fossil evidence and appreciation of extant variation, many of these taxonomic labels were later recognized as unnecessary, or rather, scientifically invalid (see critiques by Mayr, 1950; 1963).
Interestingly, in recent decades, primate taxonomy has seen a proliferation of new species (more than doubling between 1996 and 2016; Goodall, 2016). This is true for extant and fossil cercopithecids (e.g., Harrison, 1988; Frost, 2001; Frost and Delson, 2002; Hlusko, 2006, 2007; Gilbert, 2007; McKee et al., 2011; Hart et al., 2012; Rossie and Hill, 2018; Arenson et al., 2022). And, of course, the human fossil record continues to produce new species (e.g., Brown et al., 2004; Berger et al., 2010, 2015; Haile-Selassie et al., 2015; Détroit et al., 2019; Roksandic et al., 2022; and see commentary by Quintyn, 2009).
With dramatically increased fossil samples and new insights into modern variation, we argue that taxonomy can be approached with more scientific rigor. We propose a hypothesis-testing approach to taxonomy. We begin with the null hypothesis that a new fossil (or fossils) represent taxa that are already known. Consequently, the paleontologist needs to test (and reject) this null hypothesis before assuming that a new taxon should be named. Testing the alternative hypothesis first—that a new fossil represents a new taxon—sets up a study design aimed at finding differences. Given that no two individuals are identical, differences are therefore relatively easy to find, and the hypothesis is consequently difficult to reject. When applied to the same set of fossils, these two approaches need not, and likely often will not, return the same results (e.g., Gill, 2014).
We are not the first to propose a hypothesis-testing approach to taxonomy—however, the adherence to such an approach in paleontology is not common, and we hold that it should be. Other authors have applied this kind of approach within primates [testing the single-species hypothesis for Homo erectus, Villmoare (2005)] and in other mammalian taxa [Brontotheriidae, an extinct family of perissodactyls, Mihlbachler (2008)]. The latter example is particularly relevant to the present study, as it addressed the taxonomic challenges posed by early foundational work in the field that had produced a “severely oversplit alpha taxonomy” (Mihlbachler, 2008:9). These speciose taxonomies resulted from the interpretation of minor variation as taxonomically significant, generally ignoring the possibility that differences between specimens could reflect intraspecific variation.
For example, Mihlbachler (2008) revaluated the species-level taxonomy of the Brontotheriidae based on craniodental materials, making explicit the operational criteria that were applied for species delimitation. In detailing their approach to species-level taxonomy, they addressed some of the challenges of drawing population boundaries around clusters of fossils, including temporal scope—even if the fossils occur in the same geographic locality, they are often separated by geological time. The addition of a time element increases the difficulty of determining which differences result from intraspecific variation, versus which are taxonomically and evolutionarily significant. One way to address this uncertainty is to look to close extant relatives of the fossil taxon in question, to assess whether characters that vary among the fossils are polymorphic or monomorphic in the extant sample(s). If two fossils exhibit two different character states, but that character is seen to be polymorphic in the extant sample, conspecificity cannot be falsified (the null hypothesis is that a single species is represented by the two fossils). In this way, extant comparisons can be used as guides to find characters that are polymorphic within a species and are thereby unlikely to be informative of species boundaries. This allows the researcher to then examine only characters that do not show polymorphic tendencies in the extant reference sample. Unsurprisingly, the application of this approach to the Brontotheriidae resulted in the synonymization of many species names (Mihlbachler, 2008).
Although this approach employs explicit operational criteria and sounds relatively straightforward, in practice this can be difficult to apply. For example, Mihlbachler’s (2008:16) concept of a monomorphic trait is one that “shows monomorphic tendencies.” Monomorphism is therefore a classification that is based on the frequency of occurrences of different states, and which subsumes some amount of trait variation (albeit usually a small amount). Such a frequency-based approach can work well among extant taxa, where samples are more often large and complete, but poses great challenges when applied to fossils where data are limited (e.g., Halenar-Price et al., 2024).
Returning to cercopithecids, at the time that the three South African Parapapio species were named, little was known of the papionin fossil record, and access to extant comparative samples was limited [e.g., a limited and sex-imbalanced Papio hamadryas ursinus sample formed the comparative basis in Freedman’s (1957) landmark study]. Now, equipped with phenotypic data for hundreds of fossils and comparative extant organisms, we revisit the taxonomy of Parapapio with our hypothesis-testing approach. We test two hypotheses in this study:
H1: The craniodental variation within Parapapio does not cluster into three groups (i.e., does not reflect the three named species).
H2: All the Parapapio fossils can be accommodated within the craniodental shape and size variation observed for a single extant species of papionin.
To test the first hypothesis, we assessed craniodental variation among a subset of relatively complete and well-preserved Parapapio crania (n=16). To test the second hypothesis, we compared Parapapio dental metrics relative to extant samples to assess whether the Parapapio fossils are similar enough to be considered one species. We employ three extant comparative samples to set expectations for the range of variation in a papionin species of similar body size to Parapapio, and with varying degrees of sexual dimorphism. Through quantitative comparisons, morphological seriations, and sexual dimorphism estimation, we set out to test the hypothesis that the fossils can be accommodated within a single species. If this hypothesis cannot be rejected, it may be time to reconsider the enduring three-species paradigm.
The original Parapapio fossils on which this study is based (n=64, Table 2) were assessed in-person by LJH, MFB, and TAM at the Ditsong Museum of Natural History, the School of Anatomical Sciences at the University of the Witwatersrand, and the University of California Museum of Paleontology. The Parapapio sample includes only individuals recovered from South African deposits, representing the three size-based morphs (P. whitei, P. broomi, and P. jonesi).
To contextualize the variation observed among the Parapapio dental remains, we include linear dental measurements for three comparative extant samples: Macaca fascicularis, Papio hamadryas cynocephalus, and Papio hamadryas sspp. (Table 2). These samples were selected to provide comparisons with different degrees of variation, particularly in the degree of sexual dimorphism and geographic range size. Macaca fascicularis is included as a comparison with a similar body size to Parapapio, and with a moderate degree of size sexual dimorphism (Plavcan, 2001). Papio hamadryas cynocephalus represents a single population that was culled from a sisal plantation in Kenya (Hlusko and Mahaney, 2007, 2024), and therefore provides a sense for a particularly narrow range of intraspecific variation. Lastly, Papio hamadryas sspp. includes multiple subspecies of Papio hamadryas to provide a comparison with a broader range of intraspecific variation that encompasses a larger geographic area. We note that not all researchers agree with our Papio taxonomy [e.g., see review of this issue in Gilbert et al. (2018)], and we refer the reader to previous work justifying our taxonomic classifications [Brasil et al. (2023) and references therein].
Species-level classifications and sex estimations follow the published literature for each Parapapio specimen. In cases where classification conflicted between publications, we retained the most recent taxonomic identification. We used an earlier, more specific taxonomic identification than the most recent label for two individuals (i.e., specimens classified as Parapapio sp. by Fourie et al., 2008, but as P. broomi (MP 47) and P. whitei (MP 208) in earlier reports; Freedman, 1957; Williams et al., 2007).
Among the n=64 total Parapapio specimens included in this study, n=18 had conflicting published classifications [detailed in the dataset associated with this study, Brasil et al. (2024)]. Five of these specimens had been published as Parapapio sp., or as one of two possible species (e.g., P. broomi or P. whitei); for these five specimens, we used the most specific published classification available. Another three specimens had conflicting classifications between Maier (1970) and Freedman (1976); in these cases, we followed the classification in Freedman’s (1976) seminal paper reassessing the South African cercopithecoid fossil record as it was known at the time. For the remaining ten specimens with conflicting classifications, we followed Gilbert (2007, 2013) and Gilbert et al. (2018); in nearly all these cases, the majority of published classifications aligned with those we employed.
In cases with conflicting sex estimations, we marked the sex as uncertain unless there was agreement among a majority of publications. References for our taxonomic identifications (and alternate identifications) and sex estimations are included in the dataset associated with this study (Brasil et al., 2024).
Only adult Parapapio specimens (defined as having completely erupted third molars) were included in assessments of cranial anatomy to minimize variation introduced by ontogeny. Specimens were only assessed if third molar eruption could be confidently confirmed. Dental metrics were collected for subadult specimens only in cases where the permanent crown was fully erupted.
For the Parapapio fossils, dental metrics were collected by MFB and cranial metrics were collected by TAM from original fossils using Mitutoyo digital calipers and following standard protocols (Grieco et al., 2013; Monson et al., 2017; Monson, 2020). Dental metrics for the three comparative extant samples followed the same measurement definitions but were collected for previous research. The Pa. h. cynocephalus data were collected using Mitutoyo digital calipers by LJH as described by Hlusko and Mahaney (2007); these data are published and available (Hlusko and Mahaney, 2024). The M. fascicularis and Pa. hamadryas sspp. data were collected from digital photographs as described in Grieco et al. (2013). These data are published and available (Grieco et al., 2012). Dental metrics were not corrected for interstitial wear.
Craniodental trait assessments were made by MFB, LJH, and TAM. A subset of relatively complete Parapapio cranial specimens (n=16) was first seriated by overall cranial similarity. We then seriated by six specific morphological features that have been previously suggested to differentiate the three species (see Table 1). These six features include: maxillary first molar length, cranial size, muzzle length, lateral profile, maxillary fossae, and maxillary ridges.
Seriation based on overall cranial similarity organized specimens from most gracile to most robust, with greater robusticity meaning more pronounced cranial features (e.g., larger supraorbital torus, larger maxillary ridges, deeper maxillary fossae). For maxillary first molar length, cranial size, and muzzle length, specimens were seriated by absolute size. Seriation based on the lateral profile ranged from a weak or absent to a steep anteorbital drop (i.e., a more concave profile from glabella to rhinion). For the remaining maxillary features, specimens were seriated from shallow and weakly-developed fossae and ridges to deep and well-developed, respectively. Our descriptive terminology follows published descriptions of Parapapio morphology, and we note that some of these terms are vague and imprecise (see Section 1.1.2). In the case of our seriation, these terms are used to comparatively describe specimens within the Parapapio sample, relative to one another.
We undertook this morphological sorting exercise intentionally without reference to previous taxonomic assignments so that we could compare our unbiased results with previous taxonomic classifications.
Quantitative cranial comparisons among the three hypothesized Parapapio species were guided by previous reports of features that vary between them, and for which preservation allowed measurement of multiple individuals. Cranial metrics were visualized and compared using bivariate plots. We also compared variation in dental metrics for Parapapio with three extant samples: Macaca fascicularis, Papio hamadryas cynocephalus, and Papio hamadryas sspp. (see Table 2 for sample details). Molar dimensions, the original basis for naming the three species, were compared using bivariate plots. All data visualizations were produced using ggplot2 (v3.3.0, Wickham, 2016) in the R statistical environment v3.6.1 (R Core Team, 2019).
Descriptive statistics were calculated from dental metrics for Parapapio and the three extant samples. The sample size for Parapapio is relatively small and therefore unlikely to encompass the full range of variation in the genus. Coefficients of variation for Parapapio metrics were therefore calculated both without and with a sample-size correction to account for the small sample size relative to the extant samples (Plavcan and Cope, 2001). To determine whether the Parapapio coefficients of variation are statistically different from our comparative samples, we tested for equality of coefficients of variation with the modified signed-likelihood ratio test (Krishnamoorthy and Lee, 2014), using the cvequality package (v0.2.0, Marwick and Krishnamoorthy, 2019) in R v4.4.1 (R Core Team, 2019).
Considering the small and uneven sample sizes, we employed non-parametric statistical tests to test for significant differences in cranial and dental metrics between the hypothesized Parapapio species. We employed the Kruskal-Wallis test with a Dunn’s post hoc test using base R functions and the rstatix package (for the Dunn’s post hoc test, v0.7.2, Kassambara, 2023) in R v4.4.1 (R Core Team, 2019). We report the Dunn’s post hoc test results without a Bonferroni correction for multiple comparisons since the correction harshly overwhelmed nearly all statistical signal. Significance for all tests was set at p<0.05. We also calculated effect sizes for the Kruskal-Wallis test (as Eta squared, η2, Cohen, 1988) using the rstatix package (v0.7.2, Kassambara, 2023), and for the Dunn’s post hoc test (as Hedges’ g, Hedges and Olkin, 1985) using the effsize package (v0.8.1, Torchiano, 2020) in R v4.4.1 (R Core Team, 2019).
We estimated the degree of size sexual dimorphism for the pooled Parapapio sample to compare it with our three extant comparative samples. Macaca provides a reference for a moderate to strong degree of size sexual dimorphism, whereas Papio generally ranges from strong to extreme (Plavcan, 2001). We calculated the degree of sexual dimorphism in our comparative samples as the ratio of the mean male value divided by the mean female value (i.e., the sexual dimorphism index). We employed two approaches to sexual dimorphism estimation: the mean method and finite mixture analysis (Plavcan, 1994). The mean method divides the sample into two subsamples at the mean. The upper and lower means are taken to represent males and females, respectively. The sexual dimorphism index is then calculated using the means of these subsamples. In contrast, finite mixture analysis (FMA) estimates the maximum amount of dimorphism (i.e., the maximum separation between male and female means) that can be contained in a unimodal distribution (see Plavcan, 1994, for a summary of this method and Godfrey et al., 1993, for the calculation procedure).
Although the mean method assumes that the male and female ranges do not overlap (which is rare, except in cases of extreme dimorphism), most previous studies have reported that this method provides the most reliable estimate of sexual dimorphism in unsexed samples (Plavcan, 1994; Rehg and Leigh, 1999; Grabowski et al., 2015; Ruff et al., 2018; Villmoare et al., 2019). This method is most accurate when sexual dimorphism is moderate to high, and it can yield overestimates when actual dimorphism is low. However, neither of these methods can accurately estimate low levels of dimorphism, and no known estimation approach performs well in all conditions (Plavcan, 1994; Rehg and Leigh, 1999).
In his comparison of sexual dimorphism estimation methods, Plavcan (1994) found that the mean method was the most accurate even in cases with biased sex ratios and high intersexual variation. Given that the mean method usually overestimates dimorphism even slightly more than the FMA method at low levels of actual dimorphism (dimorphism indices <1.3), the mean method is useful for setting a conservative upper limit on the degree of dimorphism in a sample. In cases of low actual dimorphism, the lower estimates produced by the FMA method are usually more accurate. However, in cases of low intrasexual variation, such as for features that exhibit relatively low levels of variation (e.g., postcanine teeth), the FMA method may underestimate rather than overestimate dimorphism. Given this limitation, it is not recommended that this method is used alone (Plavcan, 1994), and so we apply it in conjunction with the mean method and report the estimates produced by both methods.
For our extant samples, for which individual sexes are known, we calculated actual sexual dimorphism indices. We also estimated the sexual dimorphism indices using the two methods detailed above for each extant sample to compare observed versus estimated values. We report actual and estimated dimorphism indices made using the maxillary second molar length, which yielded estimates similar to those based on the first and third maxillary molars.
Our initial seriation based on overall cranial robusticity aligns poorly with current taxonomic classifications, as is made clear by the commingled distribution of the three species (Figure 2). Among the seriations based on individual morphological features, this result is even more pronounced, where individuals from the three hypothesized species are shuffled around the seriations across the different traits (Figure 3). For example, MP 239 (P. whitei) is among the most robust specimens, among the least robust, and exactly in the middle of the spectrum when looking across the morphological features (Figure 3).
Figure 2. Parapapio crania seriated from generally most robust (right cranium at center top) clockwise to least robust (left cranium at center top). Scale is the same for all crania. See Methods for details on seriation criteria. Specimen IDs clockwise from top right to top left: BF 43, MP 239, MP 223, MP 221, MP 76, MP 2, MP 75, M 3070, SWP 389, STS 565, STS 397, and STS 254A. Consensus taxonomic identifications are adjacent to each cranium. Each specimen is assigned a color to facilitate visualization of seriation results for multiple morphological features (see Figure 3).
Figure 3. Seriation results for seven morphological features purported to differentiate between the three South African Parapapio species. Scale is the same for all crania. See Methods for details on seriation criteria. Each individual is assigned a color in the top row to facilitate visualization of seriation results (see Figure 2 for closer views of the specimens). In general, seriation results vary considerably across features and do not pattern consistently with the three species division. M1, first molar; P. br, Parapapio broomi; P. jo, Parapapio jonesi; P. wh, Parapapio whitei.
Whereas the sorting results for maxillary first molar length, muzzle length, and maxillary ridges are somewhat aligned with the expected pattern based on the species labels, there are notable deviations across the traits (e.g., P. whitei specimen MP 223, P. broomi specimen MP 76, and P. jonesi holotype STS 565; Figure 3). The results for lateral profile and maxillary fossae are particularly different from the expected pattern, as individuals assigned to the three species are scattered irregularly across the seriation (Figure 3).
Among those features that directly track size differences, the results generally align with expectations (e.g., maxillary first molar length and muzzle length; Monson and Brasil in review)1. This pattern is to be expected considering that the three species were initially named primarily based on dental size. But for other features, especially maxillary ridges and fossae, which have also been used to distinguish between the species and should be most developed (i.e., larger and deeper, respectively) in P. whitei and least in P. jonesi (Table 1), the seriation results are much noisier. Taken together, our results indicate that these morphological features do not pattern consistently across the hypothesized species, failing to reject our first hypothesis: The craniodental variation within Parapapio does not cluster into three groups (i.e., does not reflect the three named species).
Cranial metrics for the three hypothesized Parapapio species overlap considerably (Figure 4). None of the cranial variables were found to be significantly different among the three species (Kruskal-Wallis, p>0.05). Pairwise statistical comparisons among the three species for the seven cranial variables shown in Figure 4 yielded almost no significant differences (Table 3). The only significant difference was found for facial length. Although the Kruskal-Wallis result was insignificant for facial length (p=0.107), a significant difference was recovered by the Dunn’s post hoc test between P. jonesi and P. whitei (Table 3). Effect sizes for the Kruskal-Wallis test were all small or negligible (following Cohen, 1988), while eight of the 17 pairwise comparisons (Dunn’s post hoc test) yielded large effect sizes (Table 3).
Figure 4. Parapapio cranial metrics plotted by hypothesized species with 95% confidence ellipses. Plots include measurements of the palate (A–C), muzzle (D, E), and face (E, F). All measurements are in millimeters. Measurements are shown on the cranium at the right and include: (1) palatal width at the upper canines, (2) palatal length, (3) palatal width at the upper third molars, (4) muzzle width at the maxillary fossae, (5) maximum width of the muzzle at the upper canines, (6) facial length, and (7) maximum length of the cranium. For all metrics, P. jonesi falls near the center or at the low end of the overall distribution and is within the 95% confidence limits of the two larger size morphs. P. whitei and P. broomi ranges overlap considerably with a slight offset in palatal measurements (A–C). M3, third molar.
Dental metrics plot as expected for the three species, with P. jonesi at the low end of the size range and P. whitei at the upper end (Figure 5A). All dental variables were found to be significantly different among the three species (Kruskal-Wallis, p<0.05). Most of these differences are driven by significant results between P. jonesi and P. whitei, with fewer differences between P. broomi and P. jonesi, and only two significantly different variables between P. broomi and P. whitei (maxillary second and third molar lengths; Table 4). Large effect sizes for the Kruskal-Wallis test were only observed for four of the ten comparisons, whereas nearly all of the pairwise comparisons (Dunn’s post hoc test) yielded large effect sizes (with only two exceptions, Table 4). These results are as expected, considering that differences in dental size formed the original basis for the three species taxonomy.
Figure 5. Maxillary first versus second molar mesiodistal lengths for (A) the three hypothesized Parapapio species and an extant comparative sample, Papio hamadryas cynocephalus (see Materials and Methods for sample details), and (B) the pooled Parapapio sample and Papio h. cynocephalus, plotted by sex. Measurements are in millimeters. In (A) the Parapapio morphs follow the expected pattern with P. whitei at the larger end, P. jonesi at the smaller end, and P. broomi in the intermediate range. However, the range of variation across all three morphs is similar to a single extant subspecies of Papio hamadryas. In (B) the distribution of female and male Parapapio individuals is similar to Papio h. cynocephalus, with the only exception of a single male individual (STS 250) at the lower bound of the range. M2, second molar; M1, first molar.
However, considering that the three groups grade into each other to form a continuous range of variation (Figure 5A), we assessed whether the range of variation exceeds expected levels based on modern analogs. Comparison with a similarly-sized modern papionin (Papio hamadryas cynocephalus) demonstrates that the total range of variation within the entire Parapapio sample is similar to a modern population (related individuals all from the same troop of baboons), and not visibly beyond what should be expected for species-level variation (Figure 5A). Plotting the dental metrics by sex reveals a general trend of males at the upper end and females at the lower end, with one extreme exception, a male specimen classified as P. jonesi (STS 250; Freedman, 1957; Jablonski, 2002; Gilbert et al., 2018). Heaton (2006) identified this specimen as belonging to P. broomi (sex indeterminate) but notes that his taxonomic identification is tentative because of heavy wear and adhering breccia. The moderately large canine alveolus suggests that this individual is likely a male (Supplementary Figure S1). Its extreme position among the Parapapio sample, and especially among the males (Figure 5B), is in part due to reduced tooth lengths associated with deep dental wear (Supplementary Figure S1). However, this almost certainly does not account for the full extent of this individual’s small size; even correcting for interstitial wear, this individual was likely at the small end of the size range. Other than this single outlying individual, the distribution of males and females is similar to our modern population of Pa. h. cynocephalus (Figure 5B).
To quantitatively assess dental variation, we compared coefficients of variation (CVs) for the entire Parapapio sample to our three modern comparative samples: M. fascicularis, Pa. h. cynocephalus, and Pa. hamadryas sspp. Our results indicate that the degree of variation observed within Parapapio does slightly exceed that observed in the population of Pa. h. cynocephalus but does not necessarily exceed expectations for a single species (Table 5). The CVs indicate that the variation encompassed in Parapapio is greater than Pa. h. cynocephalus. This is reflected in the significant differences in CVs that were observed between Parapapio and Pa. h. cynocephalus, for approximately half of the variables assessed (Table 5). This result is to be expected given that this comparative sample represents a single population (see Materials for details). The CVs for Parapapio are also slightly greater on average than Pa. hamadryas sspp. and lower than M. fascicularis (Table 5). However, statistical comparison of CVs indicates that Parapapio is not significantly different from M. fascicularis or Pa. hamadryas sspp. (Table 5). Descriptive statistics for select dental metrics are reported in Table 6.
Table 5. Coefficients of variation and sample sizes for maxillary postcanine dental metrics in Parapapio and comparative extant samples.
Table 6. Descriptive statistics for Parapapio canine and postcanine dental measurements. Measurements are in millimeters.
The mean and finite mixture analysis (FMA) methods both produce slight overestimates relative to observed sexual dimorphism indices for the three comparative samples, and especially for M. fascicularis (Table 7). Among the estimated values, however, Parapapio is similar to M. fascicularis and Pa. hamadryas sspp. This similarity suggests that the dental metric variation observed within Parapapio does not exceed that of a single moderately dimorphic papionin species. These results combined with those detailed in the section above fail to reject our second hypothesis.
Table 7. Observed and estimated dimorphism indices for Parapapio (pooled species) and comparative extant samples, based on the mesiodistal crown length of the maxillary second molar.
We tested two hypotheses, that (H1) the craniodental variation within Parapapio does not cluster into three groups that reflect the three known species, and (H2) all the craniodental variation exhibited by the fossils within the South African Parapapio assemblage can be encompassed within a range of variation expected for a single species. We tested these hypotheses using the same traits that have been historically used for taxonomic diagnosis. Our results indicate that morphological features of the cranium do not cluster consistently according to the three hypothesized species (i.e., where individuals classified as the same species would be expected to align more closely with conspecifics than individuals of other species; Figures 2–4; Table 3), failing to reject H1. Although the dental variation plots as expected according to the three size morphs (Figure 5A), and there are significant differences and large effect sizes for some comparisons (Table 4), comparison with modern analogs demonstrates that the entire Parapapio sample does not exceed a range of variation expected for a moderately sexually dimorphic papionin species, failing to reject H2 (Figure 5; Tables 5, 7). These results carry substantial implications not only for the taxonomy of this genus, but also for biochronological and paleoecological studies, and for the use of Parapapio as a comparative reference for interpreting the taxonomy and paleobiology of hominids recovered from the same deposits (Beaudet, 2023; Delson, 1984, 1988; Frost et al., 2022; Jolly, 2001).
Freedman’s (1957) foundational study of South African fossil cercopithecoids laid out a comprehensive review of the evidence available at the time, updated genus- and species-level definitions, and evaluated fossil variation relative to an extant taxon, Papio h. ursinus. When compared to modern Pa. h. ursinus as a standard, the variation observed for a pooled sample of Parapapio from Sterkfontein was found to be too large for a single species (Freedman, 1957). However, Freedman (1957) notes that sexual dimorphism in Pa. h. ursinus is too great for a pooled-sample CV to be a useful comparison, and so he calculates CV only for the “total mature adult male group” (n=35). This approach yields a strikingly low coefficient of variation for mandibular M3 length (4.8 reported in-text, p. 158; 4.4 reported in Table 2, p. 148; Freedman, 1957), well below values calculated in the present study for molar dimensions among our much larger comparative samples (Table 5). Freedman (1957) also noted that there was no clear size trend in the Sterkfontein deposits and that the size distribution (i.e., of small, medium, and large individuals) through the deposits appeared random. He therefore retained Broom’s (1940) three-species framework as “the most logical interpretation of the available evidence” (Freedman, 1957:158).
Following this foundational study, Freedman and Stenhouse (1972) reported on new fossils and reexamined the Parapapio specimens from Sterkfontein. Postcanine tooth dimensions were considered, and the results upheld the validity of the three Parapapio species (P. jonesi, P. broomi, and P. whitei). T-tests of the postcanine dental metrics identified statistically significant differences between the three species. In contrast to the Freedman (1957) study, this later analysis did not include a modern analog.
The results of our pairwise comparisons of dental metrics by species echo the findings reported by Freedman and Stenhouse (1972). However, here we do employ extant analogs to frame the total variation observed in the pooled Parapapio sample. In our study, comparisons with Pa. h. cynocephalus, for example, demonstrate that Parapapio dental variation is surprisingly comparable to that of an extant population (Figure 5). Although not explicitly tested here, these comparisons also suggest that if the modern analog’s size variation (i.e., Pa. h. cynocephalus) was divided into three bins, the comparisons between those size-based subsamples would likely return statistically significant differences. However, the statistical support would be biologically suspect, as the sample would have been intentionally subdivided on the basis of the test parameter. A useful cautionary tale that demonstrates the problems with this type of embedded bias is the debate over Morton’s 19th century comparison of cranial capacity between subsets of humans (Kaplan et al., 2015).
Following the recovery of new cercopithecid fossils from South African deposits, Freedman (1976) revisited the assemblages from Makapansgat, Sterkfontein, and Taung. As he begins his assessment of Parapapio, he notes his uneasiness with the taxonomic scheme: “The situation of 3 apparently morphologically very similar species, of progressively increasing sizes, occurring at both Sterkfontein and Makapansgat has always appeared to be disturbing” (Freedman, 1976:303). Freedman (1976:303) provides the history of interpretation of Parapapio up until that point in time, explaining that the taxonomy had initially been based on the dentition, but that with the recovery of fairly complete cranial specimens, “a number of morphological differences between the 3 species are becoming apparent.” However, this newly recovered material had also prompted some taxonomic reassignments by Maier (1970) of specimens that had been previously classified by Freedman (1960, 1965). Freedman (1976) therefore re-assessed dental variation in the Parapapio sample from Makapansgat to evaluate the scope of variation and the taxonomic reclassifications posed by Maier (1970). He reports that the coefficients of variation appear large when compared to extant Papio species and concludes that it appears probable that at both Makapansgat and Sterkfontein, more than one taxon is sampled (Freedman, 1976). He does, however, note that “in both cases, the material probably accumulated over a considerable period of time” (Freedman, 1976:304).
Following his assessment of molar dimension distributions, Freedman (1976) notes that several specimens do not plot within the expected range based on their taxonomic classification and suggests that those specimens may be better referred to other species. He also suggests that, as an alternative route, the boundary between species (in this particular case, P. broomi and P. jonesi) could be shifted to align with the proposed taxonomy of Maier (1970). This discussion of cut-off points in dental metrics, and what shifting thresholds would mean for the taxonomic identifications of particular intermediate specimens, highlights the degree of subjectivity affecting the species boundaries. Freedman (1976:305) recognizes this in conceding that: “Clearly, at this point in our knowledge of the Parapapio species, there is a strong element of subjectivity in the lines one chooses to draw to separate the 3 species, at least on the basis of the M3 dimensions.”
Following Broom’s (1940) initial recognition of three species, and Freedman’s subsequent studies, the validity of the three-species scheme has been repeatedly questioned (Eisenhart, 1974; Thackeray and Myer, 2004; Heaton, 2006; Williams et al., 2007; Fourie et al., 2008; Thackeray et al., 2019; Beaudet, 2023). Heaton (2006) suggested that P. whitei fossils should be reclassified as P. broomi or Papio izodi. Thackeray and Myer (2004) suggested that P. broomi and P. jonesi may represent males and females, respectively, of the same sexually dimorphic species. Study of the semicircular canals of the P. broomi and P. jonesi holotypes suggests they are likely to belong to the same species, leading the authors to propose that they are in fact conspecific (Thackeray et al., 2019). Additional analyses of internal structures further suggest that taxonomic diversity within Parapapio may be overestimated as it currently stands (Beaudet et al., 2016a, 2016b; Beaudet, 2023).
Just as Freedman (1976) voiced his discomfort with three very similar species coexisting at Makapansgat and Sterkftontein, Brain (1981) questioned the validity of three contemporaneous Parapapio species occupying similar ecologies. Although this degree of sympatry is observed in some primates (e.g., guenons), there is no modern analog for this degree of sympatry among papionins (Fourie et al., 2008; it should be considered, however, that anthropogenic impacts on environments may be skewing modern distributions to some extent; Hill et al. 2020). This unusual situation among Parapapio has motivated numerous investigations of whether there is any evidence of niche separation among the three species that would suggest differences in their ecologies. An analysis of dental microwear among P. broomi and P. jonesi, which co-occur at Makapansgat and Sterkfontein, was employed to assess whether perceivable dietary differences exist between the two species (El-Zaatari et al., 2005). Different dietary niches were interpreted for the two species at Makapansgat (Members 3–4), whereas no significant difference was observed at Sterkfontein (Member 4). No differences were apparent between P. broomi from the two sites, whereas differences were found for the diet of P. jonesi—these differences are suggested to possibly reflect temporal change sampled by the different time intervals at these two sites (although see discussion of the limitations of drawing dietary inferences from microwear analysis, El-Zaatari et al., 2005). Study of locomotor signatures in postcranial material has also produced some evidence of different locomotor strategies between P. broomi (more arboreal) and P. jonesi (more terrestrial), suggesting some species-level differences in ecologies (Elton, 2001).
In a comparison of stable carbon isotope ratios in the teeth of P. broomi and P. jonesi, the mean values for the two species were found to be nearly identical (Thackeray, 2005). Additional study of stable isotopes and trace elements from fossil tooth enamel from the three species at Makapansgat coupled chemical with craniometric analyses to assess the dietary evidence alongside the morphological evidence (Fourie, 2006; Fourie et al., 2008). Two overlapping ecologies were found within Parapapio, loosely aligned with taxonomic categories (P. broomi values reflecting a more C3-dominated diet, whereas P. whitei and P. jonesi values reflect a diet with more C4 grasses; Fourie, 2006; Fourie et al., 2008). However, the authors noted that this pattern differs from the results from Sterkfontein, where P. whitei appears to have a more C3-dominated diet and P. broomi and P. jonesi appear to have consumed more C4 grasses (Codron et al., 2005; Fourie et al., 2008). Importantly, Fourie et al. (2008) noted that their finding—of two overlapping dietary regimes that do not correspond to current taxonomic divisions—does not necessary indicate that there are two distinct taxa sampled at Makapansgat. Instead, these differences may reflect dietary shifts in response to environmental changes over the several thousands of years sampled, especially considering that many papionins are “adaptable generalists” that can make use of a wide variety of habitats and resources (Fourie et al., 2008).
Additionally, their craniometric results yielded no clear taxonomic signal, suggesting that issues exist among the current Parapapio assignments, and that the three-species scheme “may not reflect real biological units” (Fourie et al., 2008:131). Similar to results reported in the present study, they also concluded that their assessments of anatomical variation indicate the Parapapio sample does not exceed the variation observed in a geographically restricted extant chacma baboon (Papio h. ursinus) sample (Fourie, 2006; Fourie et al., 2008). Based on these results, and the disjunctions between dietary ecologies and taxonomic assignments, Fourie et al. (2008) called for a reinvestigation of papionin taxonomy. Our results lend support to this uncertainty, suggesting that the lines drawn between species are arbitrary and subjective, and should be reconsidered in light of the sources of variation that may be at play in the Parapapio sample.
Variation associated with ontogenetic stage, geography, sexual dimorphism, and temporal range can complicate our understanding of a fossil assemblage (Beaudet, 2023). The South African Parapapio assemblage studied here derives from a relatively small geographic area, limiting the degree of variation introduced by geography. The sample in the present study is also restricted to adult individuals, to further limit confounding sources of variation. Two important sources of variation remain—sexual dimorphism and temporal range—and must be considered as we re-assess the taxonomy within Parapapio.
The fact that we do not know the degree of sexual dimorphism in a fossil taxon poses a significant limitation for sex estimation and for circumscribing species-level variation. Initial discoveries and descriptions can have longstanding implications for assumed levels of sexual dimorphism (e.g., debates about the level of sexual dimorphism in Australopithecus afarensis; McHenry, 1991; Reno et al., 2003; Reno and Lovejoy, 2015). Looking back to early descriptions, Parapapio species are described as only weakly sexually dimorphic in dental dimensions other than the canine and lower third premolar (Freedman, 1957, 1976). These initial assessments were based only on a subset of specimens for which sex could be estimated using the canine and/or lower third premolar, which introduces a circularity that skews the amount of interpreted dimorphism. Nonetheless, this early interpretation has subsequently patterned our expectations of how much variation this genus should circumscribe. But what if instead of three species with very low dimorphism, Parapapio encompasses only a single species with a moderate degree of dental dimorphism?
The debate over the taxonomic identity of specimens of intermediate size highlights how uncertainty about sexual dimorphism and sex designations can confound taxonomic practice. For example, two specimens, M3060 and M3061 (belonging to the same individual according to Maier, 1970) are identified as a large male of P. jonesi (Maier, 1970) and later reclassified as P. broomi (Freedman, 1976). This clearly raises the possibility that some specimens of intermediate size could potentially be a female of a larger morph, or a male of a smaller one. In an effort to explicitly test whether at least some of the specimens assigned to P. jonesi are actually females of P. broomi, Thackeray and Myer (2004) evaluated dental measurements from specimens of the two species. Based on comparisons of molar dimensions relative to an extant chacma baboon sample, they concluded that it cannot be ruled out that some, if not all P. jonesi specimens are in fact females of P. broomi, leading them to call for further assessment of the intrageneric taxonomy (Thackeray and Myer, 2004).
We tested the hypothesis that the level of sexual dimorphism in our entire Parapapio sample is similar to that observed among our modern papionin samples. Choosing an appropriate modern reference sample with a similar degree of sexual dimorphism to the fossil sample under study is important to ensure that the comparison is not “biologically unfair” (Plavcan and Cope, 2001:205). However, in the present case, as in most fossil samples, the exact degree of sexual dimorphism is unknown. For this reason, we employed multiple comparative samples that span a range of degrees of sexual dimorphism, providing multiple models for the degree of dimorphism we could expect to see in a single-species fossil sample. Our estimates of sexual dimorphism for Parapapio fall between estimated indices for M. fascicularis and Pa. hamadryas sspp., suggesting that the dental metric variation within our full Parapapio sample does not exceed expectations for a single moderately dimorphic species, in the case of M. fascicularis, or a species with a broad geographic range, in the case of Pa. hamadryas sspp. (Table 7). That our Parapapio estimates fall slightly above those for Pa. h. cynocephalus is unsurprising, given that the latter sample was included to provide a sense for a narrow range of intraspecific variation as it derives from a single population from a sisal plantation in Kenya, at a single point in time (Hlusko and Mahaney, 2007, 2024). Although it is worth noting that, other than a single outlying individual (see Section 3.2 for details), the distribution of males and females of Parapapio is similar to our population of Pa. h. cynocephalus (Figure 5B), further suggesting that some of the variation within Parapapio can be explained by at least a moderate degree of sexual dimorphism.
Previous studies of cranial morphometrics have found that P. whitei and P. broomi, and to some extent P. jonesi, overlap broadly and could be described as scaled versions of each other (Williams et al., 2007). This raises the question: could some of the qualitative cranial differences described between the species result from allometric effects? For example, P. jonesi is described as having the least developed maxillary ridges and fossae (i.e., smallest and shallowest, respectively; Table 1). Could this be related to size, and/or sex-based variation? Variation in cranial features is known to be associated with variation in body size across papionins (Singleton, 2002; Frost et al., 2003; Monson et al., 2017; Monson, 2020). Size differences among papionin taxa are related to variation in muzzle shape (e.g., muzzle length and height, and nasal length; Gilbert and Grine, 2010), and facial shape (Joganic and Heuzé, 2019; Nishimura et al., 2019), reinforcing the possibility that some of the variation in cranial features we see in Parapapio could primarily reflect differences in body size rather than taxonomic distinctions.
The well-documented effects of allometry on papionin cranial anatomy add to some broader concerns about the quality and taxonomic valence of features used in assessments of Parapapio. For example, can the relative development of traits like the maxillary fossae and ridges be used as a reliable taxonomic indicator or are these traits associated with ontogeny? Age-related changes in craniofacial variation, particularly in the facial skeleton and in regions of masticatory and nuchal musculature attachment, have been documented among adult female baboons, suggesting that post-maturational changes could be playing a role in the variation observed in the Parapapio sample (Joganic and Heuzé, 2019). Detailed study of these features in large samples of modern organisms is necessary before these criteria can be applied confidently to fossils (e.g., Pallas et al., 2024). Another promising way forward will be to assess variation and revisit taxonomic hypotheses using novel approaches, such as the comparative analysis of internal structures (Beaudet, 2023; e.g., expanding upon Beaudet et al., 2016a, 2016b, and Beaudet and Gilissen, 2018). Ideally these kinds of future studies will leverage insights from studies of large samples of modern organisms as a means of addressing the inherent limitations of working with small and taxonomically ambiguous datasets. The exploration of new types of morphological data would provide a way to further assess how closely the a priori classifications (i.e., the currently recognized species-level classifications) match classifications based on a broader dataset.
Freedman (1957) noted that among the Sterkfontein material studied by Broom (1940), the few specimens known at the time fell clearly into three size groups, which formed the basis for the species-level taxonomy. However, additional material that was subsequently recovered began to fill the perceived gaps between the species, which Freedman (1957:158) characterized as the “paradox of the additional material apparently obscuring the true relationships of the Sterkfontein Parapapio species.” Later, Maier (1970) noted that aside from subtle differences in dental size, P. broomi and P. whitei are morphologically very similar and appear to be sympatric. He argued it was reasonable, based on the available evidence at the time, to assume they were closely related species with different ecological roles. He also noted, however, that “Additional material may well fill the gap between the two forms and reveal the existence of one highly variable species” (Maier, 1970:81). Taken together, the available evidence about ecological factors weighs against niche partitioning and taxonomic diversity (Beaudet, 2023, and reviewed above), suggesting that Parapapio may, in fact, constitute one moderately variable species.
Gaps in the fossil record are convenient for drawing lines between taxa, but what should be done when these gaps are filled, and the initially perceived disparities between taxa begin to disappear? In the case of Eocene primates from the Bighorn Basin, Wyoming, type specimens that were established years ago were distinctive when the species were named (Bown and Rose, 1987). However, as additional fossils were recovered from stratigraphic intervals between them, their distinctiveness fell away (Bown and Rose, 1987). This Eocene primate analogy is limited by one key difference: the assemblage encompasses large samples with precise chronostratigraphic control. As Bown and Rose (1987) note, without knowing where specimens fit in a temporal sequence, there is “no way, therefore, to deal with temporally stratified variability. Once a stratigraphic sequence can be established, the relations between vertical-temporal and lateral variability become more clear and can be separated.” For this reason, the cave deposits from which Parapapio fossils are mostly recovered in South Africa make the taxonomy of this genus more challenging, as geological time is mixed and compressed.
Plavcan and Cope (2001) advise that the researcher should always consider that elevated sample variation may be a function of temporal variation and that comparisons of variation between fossil and extant samples must take into account both biological (e.g., pattern of sexual dimorphism) and geological (e.g., temporal variation) contexts. They highlight the work of Bown and Rose (1987) and Rose and Bown (1993) on Eocene omomyid primates, and Gingerich (1985) on Eocene hypsodontid ungulates, as examples of studies that track changes in tooth size across stratigraphic levels, reflecting phyletic evolution within lineages. In these examples, when tooth size is assessed within a stratigraphic level, tooth size variation is comparable to a living species. However, when specimens from across stratigraphic levels are pooled, a high degree of variation in tooth size is observed. Within this controlled stratigraphic framework, inflated tooth size variation is demonstrated to be the result of change through time (Gingerich, 1985; Bown and Rose, 1987; Rose and Bown, 1993). These examples demonstrate how temporal depth can influence the degree of variation in a fossil sample and underscore the problem of time-averaging, where fossils from different time periods appear synchronous in the record (Kowalewski, 1996).
Assessing variation in a fossil sample is necessary to decide how many species are represented, and extant species lineages provide a reference for the amount of variation we should expect in a fossil species (Villmoare, 2005; White, 2014; Beaudet, 2023). However, one of the limitations of modern analogs is that they sample a single snapshot in time, and so they should generally be treated as setting a conservative lower bound for the variation to be expected in a fossil species. The range of dental variation in Parapapio appears to slightly exceed that of a modern analog (Pa. h. cynocephalus, Figure 5). However, we must consider, following Plavcan and Cope’s (2001) advice, that the Parapapio sample likely encompasses a substantial depth of time, with time-averaged components (e.g., Member 4 of Sterkfontein; Beaudet, 2023). The temporal depth of the Parapapio sample in the present study may range from just over one million years to nearly two million years, depending on the chronological estimate (Frost et al., 2022; Granger et al., 2022, 2023). Variation observed among the sample may therefore result from phyletic evolution over this depth of time. Previous research suggests that the degree of morphological variation within Parapapio reflects a considerable time depth among the karstic deposits from which the fossils derive (especially Makapansgat and Sterkfontein; Williams et al., 2007). Our results are at odds with this conclusion, as we found that Parapapio dental variation is comparable to all three of our extant samples, which each represent a single temporal sample.
Whether the variation within Parapapio reflects taxonomic diversity or phyletic evolution will remain challenging to test with the current limitations on chronological resolution of the individual specimens. Sustained field efforts to recover new fossils within chronostratigraphically controlled contexts will be critically important for testing these hypotheses. However, what we can conclude at this point is that, considering the similarity to our modern samples, the dental size variation of Parapapio appears not to have evolved much over the one-to-two million years potentially represented by the fossils included in our study.
Fossils are not discovered with taxonomic labels affixed. It is the task of the paleontologist to identify the Linnaean group (i.e., taxon) to which a fossil belongs. The proposed taxonomic classification is a testable hypothesis with specific testable predictions (Gaston and Mound, 1993; Peters and Marcus, 2017). When new data, methods, or theoretical frameworks become available, these hypotheses should be reevaluated.
In this study, we asked: does the three-species paradigm of Parapapio reflect a biologically realistic trichotomy? If we were to lay out all the fossils and start over, what would the resulting taxonomy look like? Would we come up with the same classificatory scheme if these fossils were found today, or is the historical momentum of this three-species organization skewing our perspective? It should be noted here that, as with other scientific hypotheses, falsification of a taxonomic hypothesis does not reduce the value of the taxonomist (Gaston and Mound, 1993), especially when we consider hypotheses that were established when comparative samples and studies of variation were limited.
Following the original Parapapio species designations (primarily based on differences in dental size; Broom, 1940), morphological differences were pursued, and cranial features that were thought to distinguish them were added to the species definitions (Freedman, 1957; Maier, 1970; Freedman, 1976). The initial three-species taxonomic framework clearly influenced these subsequent interpretations. Perhaps the morphological features that were assigned to these species post hoc were the result of researchers looking for differences between the purported taxa. This possibility should be seriously considered given the historical context surrounding the naming of the subsequent species, which lacked a detailed analysis of the holotypes (see Thackeray et al., 2019) and fell short of the standards for describing a new species as they stand today (e.g., Braby et al., 2024).
Discussion and debate about taxonomy can seem like a matter of semantics, but taxonomic practice can be foundational to virtually all downstream study in both the neontological and paleontological realms, often underpinning studies across evolutionary biology, ecology, conservation biology, among a broad range of other biological sciences (Braby et al., 2024). Therefore, we are obliged to revisit even long-standing taxonomic schema if we aim for improved resolution in evolutionary biology (e.g., Mihlbachler, 2008).
We do not currently have the resolution across the Parapapio-bearing South African cave deposits to evaluate whether the morphology of this taxon patterns through time across a single lineage, or whether there were multiple contemporaneous species of Parapapio. The guenons may provide a useful analog among modern cercopithecids, as this group of cercopithecins is taxonomically diverse, but many of the features that distinguish them do not fossilize (e.g., soft tissue, pelage, and behavior; Taylor et al., 2023). Species-level, and to some extent, genus-level, differences are not visible in the guenon skeleton. It may be the case that Parapapio represents a similar situation, and that we are unable to infer species-level diversity from the hard tissue data that are preserved. As with some fossil guenons (e.g., Taylor et al., 2023), we cannot readily distinguish among possible species, at least for now—perhaps new data from paleoproteomic and ancient DNA studies may provide novel insights in the future.
With the resolution we currently have, we propose a more conservative taxonomic scheme, subsuming all Parapapio specimens within Parapapio broomi, the species name with priority (Jones, 1937). We note that we cannot rule out the possibility that the Parapapio sample includes more than one species, although the currently available data do not support this interpretation (Fourie et al., 2008). A high degree of variation can suggest the presence of multiple species within a sample, but a low degree of variation cannot be taken to mean that only a single species is sampled (Plavcan and Cope, 2001). It is entirely possible that cladogenetic events occurred within Parapapio and that multiple species are encompassed in the sample, but our results support previous findings in emphasizing that, if there are multiple species, they cannot be consistently separated (Fourie et al., 2008; Williams et al., 2007). It is also possible that there are multiple species that are skeletally indistinguishable (i.e., cryptic species; Tattersall, 2007). We cannot, however, exclude the possibility that we are sampling a phyletically evolving lineage, and we argue that in the interest of applying a pragmatic approach to this inferential study of the past, this should hold as our null hypothesis—the burden of proof should fall on alternative hypotheses that would split the genus into multiple species.
Our hypothesis-based approach relies on the assessment of morphological traits that are preserved in the physical fossil evidence. In testing our two hypotheses, we have assessed whether phenetic gaps exist within the Parapapio sample, and whether all specimens within this genus can be classified as a single species, that is, as “a phenetic group of a given diversity somewhat below the subgenus category” (Sneath and Sokal, 1973:365). This study design is thereby underlain by a phenetic species concept (Sokal and Crovello, 1970), which “[emphasizes] the evidence and procedures that are used to recognize species in practice” (de Queiroz, 1998: 63). Our pragmatic approach contributes a new perspective to this taxonomic challenge, which, with the hopeful recovery of new fossils with secure proveniences, and improved geochronological control, can be further evaluated with other approaches to species delimitation (e.g., the phylogenetic species concept, Cracraft, 1983; and see de Queiroz, 1998 for discussion of concepts within this umbrella). As new datasets are brought to bear on this debate, species-level taxonomy can be revisited through the lens of other species concepts as well [e.g., the ecological species concept in the case of isotopes and ecomorphology, Van Valen (1976); the isolation species concept if molecular data become available, Paterson (1985)].
Different epistemological views and different species concepts, sometimes relying on different types of data (e.g., morphological or molecular), can yield different taxonomies. For example, despite the availability of rich anatomical, behavioral, and genetic datasets, the taxonomy of modern humans and Neanderthals continues to be debated (e.g., Meneganzin and Stringer, 2024). Applying an evolutionary species concept, in which a lineage “has its own evolutionary tendencies and historical fate” (Wiley, 1978:18), humans and Neanderthals can be understood as two species lineages, with clearly distinct historical fates. On the other hand, in light of increasing evidence of repeated episodes of interbreeding between these two groups (Li et al., 2024), the application of the isolation species concept (Paterson, 1985), which is based on little to no genetic exchange, would recognize these as subgroups within a single species, H. sapiens. There is arguably one general species concept in modern biology that views species as segments of evolving lineages, with most of the fundamental differences in concepts arising from different criteria applied in species delimitation (de Queiroz, 1998). These different criteria provide a variety of means by which to test taxonomic hypotheses and are not necessarily mutually exclusive. In the present study, we have demonstrated that the application of a phenetic species concept does not support the existence of three morphologically distinct species.
The impossibility of truly knowing how closely a taxonomic system mirrors biological reality is a burden that paleontologists must carry. As effective pattern-seekers, we are drawn to organizing and categorizing data. But in the case of taxonomy, what do we gain from this practice if the resulting categories and labels do not reflect the reality of past organismal diversity? Is it better practice to construct our null hypothesis so that we place the burden of proof on ‘lumping’? Or should we place the burden of proof on the alternative hypothesis and thereby ‘splitting’? Within taxonomic practice—a uniquely human endeavor—this decision falls to the judgment of the taxonomist. However, we contend that by placing the burden of proof on ‘splitting’ (the alternative hypothesis), rather than ‘lumping’ (the null hypothesis), we avoid creating artificial boundaries that do not reflect real biological entities and muddling the many analyses that hinge on taxonomic classifications. And so, we circle back to 1976, when Freedman concluded: “It would therefore seem that it may not be possible to make firm taxonomic rulings on all Parapapio specimens at present” (p.305). Sometimes, we, as paleontologists, must be willing to accept and admit that there are limitations on what we can say about the past.
The datasets presented in this study can be found in online repositories. The names of the repository/repositories and accession number(s) can be found below: Figshare, https://doi.org/10.6084/m9.figshare.28035656.v1.
MB: Conceptualization, Data curation, Formal analysis, Funding acquisition, Investigation, Methodology, Visualization, Writing – original draft, Writing – review & editing. TM: Conceptualization, Funding acquisition, Investigation, Writing – review & editing. DS: Conceptualization, Funding acquisition, Resources, Writing – review & editing. LH: Conceptualization, Funding acquisition, Investigation, Project administration, Resources, Supervision, Writing – review & editing.
The author(s) declare financial support was received for the research, authorship, and/or publication of this article. Collection of extant primate dental data was funded by the National Science Foundation under grants BCS 0500179, 0130277, and 0616308 to LH. Travel funding was generously provided by the Palaeontological Scientific Trust and the Human Evolution Research Center. MB was supported by a University of California Berkeley Chancellor’s Fellowship, the John Templeton Foundation, and a Faculty Summer Research Grant from Western Washington University throughout the course of this study. LH is currently funded by the European Research Council within the European Union’s Horizon Europe (ERC-2021-ADG, Tied2Teeth, project number 101054659) and in part by a grant from the Spanish Ministerio de Ciencia e Innovación (Project PID2021-122355NB-C33 financed by MCIN/AEI/10.13039/501100011033/FEDER, UE). The authors acknowledge support from National Science Foundation grant BCS 2235771 to TM and MB.
We thank the following individuals for access to collections in their care: L. Kgasi and S. Potze at the Ditsong Museum of Natural History, B. Zipfel at the University of the Witwatersrand, and P. Holroyd at the University of California Museum of Paleontology. Human Evolution Research Center (UC Berkeley) personnel provided valuable discussion and feedback. We thank Brian Beatty, Laurent Pallas, and one additional reviewer for their constructive comments that enhanced this manuscript. We are grateful to the editors of this Research Topic, Laura Martínez, Vivek Venkataraman, and Antoine Souron, for the invitation to contribute.
The authors declare that the research was conducted in the absence of any commercial or financial relationships that could be construed as a potential conflict of interest.
All claims expressed in this article are solely those of the authors and do not necessarily represent those of their affiliated organizations, or those of the publisher, the editors and the reviewers. Any product that may be evaluated in this article, or claim that may be made by its manufacturer, is not guaranteed or endorsed by the publisher.
The Supplementary Material for this article can be found online at: https://www.frontiersin.org/articles/10.3389/fevo.2024.1481903/full#supplementary-material
Adams J. W., Herries A. I., Kuykendall K. L., Conroy G. C. (2007). Taphonomy of a South African cave: geological and hydrological influences on the GD 1 fossil assemblage at Gondolin, a Plio-Pleistocene paleocave system in the Northwest Province, South Africa. Quaternary Sci. Rev. 26, 2526–2543. doi: 10.1016/j.quascirev.2007.05.006
Arenson J. L., Harrison T., Sargis E. J., Taboada H. G., Gilbert C. C. (2022). A new species of fossil guenon (Cercopithecini, Cercopithecidae) from the Early Pleistocene Lower Ngaloba Beds, Laetoli, Tanzania. J. Hum. Evol. 163, 103136. doi: 10.1016/j.jhevol.2021.103136
Beaudet A. (2023). The Australopithecus assemblage from Sterkfontein Member 4 (South Africa) and the concept of variation in palaeontology. Evol. Anthropol. 32, 154–168. doi: 10.1002/evan.21972
Beaudet A., Dumoncel J., de Beer F., Duployer B., Durrleman S., Gilissen E., et al. (2016b). Morphoarchitectural variation in South African fossil cercopithecoid endocasts. J. Hum. Evol. 101, 65–78. doi: 10.1016/j.jhevol.2016.09.003
Beaudet A., Dumoncel J., Thackeray J. F., Bruxelles L., Duployer B., Tenailleau C., et al. (2016a). Upper third molar internal structural organization and semicircular canal morphology in Plio-Pleistocene South African cercopithecoids. J. Hum. Evol. 95, 104–120. doi: 10.1016/j.jhevol.2016.04.004
Beaudet A., Gilissen E. (2018). “Fossil primate endocasts: perspectives from advanced imaging techniques,” in Digital Endocasts: From Skulls to Brains. Eds. Bruner E., Ogihara N., Tanabe H. (Springer, Nagoya), 47–58.
Benefit B. R., McCrossin M. L., Boaz N. T., Pavlakis P. (2008). New fossil cercopithecoids from the Late Miocene of As Sahabi, Libya. Garyounis Sci. Bull. Special Issue 5, 265–282.
Berger L. R., de Ruiter D. J., Churchill S. E., Schmid P., Carlson K. J., Dirks P. H. G. M., et al. (2010). Australopithecus sediba: a new species of Homo-like australopith from South Africa. Science 328, 195–204. doi: 10.1126/science.1184944
Berger L. R., Hawks J., de Ruiter D. J., Churchill S. E., Schmid P., Delezene L. K., et al. (2015). Homo naledi, a new species of the genus Homo from the Dinaledi Chamber, South Africa. eLife 4, e09560. doi: 10.7554/eLife.09560.031
Bown T. M., Rose K. D. (1987). Patterns of dental evolution in early Eocene anaptomorphine primates (Omomyidae) from the Bighorn Basin, Wyoming. Memoir (The Paleontological Society) 23, 1–162. doi: 10.1017/S0022336000060911
Braby M. F., Hsu Y.-F., Lamas G. (2024). How to describe a new species in zoology and avoid mistakes. Zool. J. Linn. Soc., zlae043. doi: 10.1093/zoolinnean/zlae043
Brain C. K. (1981). “The fossil animals,” in The Hunters or the Hunted? An Introduction to African Cave Taphonomy. Ed. Brain C. K. (University of Chicago Press, Chicago), 147–190.
Brasil M., Monson T. A., Stratford D., Hlusko L. (2024). Dataset for “A hypothesis-based approach to species identification in the fossil record: a papionin case study.” figshare. doi: 10.6084/m9.figshare.28035656.v1
Brasil M. F., Monson T. A., Taylor C. E., Yohler R. M., Hlusko L. J. (2023). A Pleistocene assemblage of near-modern Papio hamadryas from the Middle Awash study area, Afar Rift, Ethiopia. Am. J. Biol. Anthropol. 180, 48–76. doi: 10.1002/ajpa.v180.1
Broom R. (1938). The Pleistocene anthropoid apes of South Africa. Nature 142, 377–379. doi: 10.1038/142377a0
Broom R., Hughes A. R. (1949). Notes on the fossil baboons of the Makapan caves. S. Afr. Sci. 2, 194–196.
Brown P., Sutikna T., Morwood M. J., Soejono R. P., Jatmiko, Wayhu Saptomo E., et al. (2004). A new small-bodied hominin from the Late Pleistocene of Flores, Indonesia. Nature 431, 1055–1061. doi: 10.1038/nature02999
Clarke R. J. (2006). A deeper understanding of the stratigraphy of Sterkfontein fossil hominid site. T. R. Soc. S. Afr. 61, 111–120. doi: 10.1080/00359190609519960
Codron D., Luyt J., Lee-Thorp J. A., Sponheimer M., De Ruiter D., Codron J. (2005). Utilization of savanna-based resources by Plio-Pleistocene baboons. S. Afr. J. Sci. 101, 245–248.
Cohen J. (1988). Statistical Power Analysis for the Behavioral Sciences (2nd ed.) (New York: Academic Press).
Cracraft J. (1983). Species concepts and speciation analysis. Curr. Ornithol. 1, 159–187. doi: 10.1007/978-1-4615-6781-3_6
Dart R. A. (1925). Australopithecus africanus: the man-ape of South Africa. Nature 115, 195–199. doi: 10.1038/115195a0
Delson E. (1984). Cercopithecid biochronology of the African Plio-Pleistocene: Correlation among eastern and southern hominid-bearing localities. Cour. Forsch. Senck. 69, 199–218.
Delson E. (1988). “Chronology of South African australopith site units,” in Evolutionary History of the “Robust” Australopithecines. Ed. Grine F. E. (Aldine de Gruyter, New York), 317–324.
Delson E., Terranova C. J., Jungers W. L., Sargis E. J., Jablonski N. G., Dechow P. C. (2000). Body mass in Cercopithecidae (Primates, Mammalia): estimation and scaling in extinct and extant taxa. Anthropol. Pap. Am. Mus. 83, 1–159.
de Queiroz K. (1998). “The general lineage concept of species, species criteria, and the process of speciation,” in Endless Forms: Species and Speciation. Eds. Howard D. J., Berlocher S. H. (Oxford University Press, Oxford), 57–75.
Détroit F., Mijares A. S., Corny J., Daver G., Zanolli C., Dizon E., et al. (2019). A new species of Homo from the Late Pleistocene of the Philippines. Nature 568, 181–186. doi: 10.1038/s41586-019-1067-9
Eisenhart W. L. (1974). The fossil cercopithecoids of Makapansgat and Sterkfontein. [Bachelor’s thesis]. Harvard University, Cambridge (MA).
Elton S. (2001). Locomotor and habitat classifications of cercopithecoid postcranial material from Sterkfontein Member 4, Bolt’s Farm and Swartkrans Members 1 and 2, South Africa. Palaeontol. Afr. 37, 115–126.
El-Zaatari S., Grine F. E., Teaford M. F., Smith H. F. (2005). Molar microwear and dietary reconstructions of fossil Cercopithecoidea from the Plio-Pleistocene deposits of South Africa. J. Hum. Evol. 49, 180–205. doi: 10.1016/j.jhevol.2005.03.005
Fourie N. H. (2006). Dietary ecology and niche separation among three closely related species (Parapapio jonesi, Pp. whitei and Pp. broomi) of South African Plio-Pleistocene Cercopithecoidea from Makapansgat Limeworks site. [Master’s thesis]. University of Cape Town, Cape Town (South Africa).
Fourie N. H., Lee-Thorp J. A., Ackermann R. R. (2008). Biogeochemical and craniometric investigation of dietary ecology, niche separation, and taxonomy of Plio-Pleistocene cercopithecoids from the Makapansgat Limeworks. Am. J. Phys. Anthropol. 135, 121–135. doi: 10.1002/ajpa.v135:2
Freedman L. (1960). Some new fossil cercopithecoid specimens from Makapansgat, South Africa. Palaeontol. Afr. 7, 7–45.
Freedman L. (1961). New cercopithecoid fossils, including a new species from Taung, Cape Province, South Africa. Ann. S. Afr. Mus. 46, 1–14.
Freedman L. (1965). Fossil and subfossil primates from the limestone deposits at Taung, Bolt’s Farm and Witkrans, South Africa. Palaeontol. Afr. 9, 19–48.
Freedman L. (1976). South African fossil Cercopithecoidea: a re-assessment including a description of new material from Makapansgat, Sterkfontein and Taung. J. Hum. Evol. 5, 297–310. doi: 10.1016/0047-2484(76)90033-6
Freedman L., Stenhouse N. (1972). The Parapapio species of Sterkfontein, Transvaal, South Africa. Palaeontol. Afr. 14, 93–111.
Frost S. R. (2001). New Early Pliocene Cercopithecidae (Mammalia: Primates) from Aramis, Middle Awash Valley, Ethiopia. Am. Mus. Novit. 3350, 1–36. doi: 10.1206/0003-0082(2001)350<0001:NEPCMP>2.0.CO;2
Frost S. R., Delson E. (2002). Fossil Cercopithecidae from the Hadar Formation and surrounding areas of the Afar Depression, Ethiopia. J. Hum. Evol. 43, 687–748. doi: 10.1006/jhev.2002.0603
Frost S. R., Marcus L. F., Bookstein F. L., Reddy D. P., Delson E. (2003). Cranial allometry, phylogeography, and systematics of large-bodied papionins (primates: Cercopithecinae) inferred from geometric morphometric analysis of landmark data. Anat. Rec. Part A 275A, 1048–1072. doi: 10.1002/ar.a.v275a:2
Frost S. R., Ward C. V., Manthi F. K., Plavcan J. M. (2020). Cercopithecid fossils from Kanapoi, West Turkana, Kenya, (2007–2015). J. Hum. Evol. 140, 102642. doi: 10.1016/j.jhevol.2019.102642
Frost S. R., White F. J., Reda H. G., Gilbert C. C. (2022). Biochronology of South African hominin-bearing sites: a reassessment using cercopithecid primates. Proc. Natl. Acad. Sci. U. S. A. 119, e2210627119. doi: 10.1073/pnas.2210627119
Gaston K. J., Mound L. A. (1993). Taxonomy, hypothesis testing and the biodiversity crisis. Proc. R. Soc. Lond. B. Biol. Sci. 251, 139–142. doi: 10.1098/rspb.1993.0020
Gilbert C. C. (2007). Craniomandibular morphology supporting the diphyletic origin of mangabeys and a new genus of the Cercocebus/Mandrillus clade, Procercocebus. J. Hum. Evol. 53, 69–102. doi: 10.1016/j.jhevol.2007.03.004
Gilbert C. C. (2013). Cladistic analysis of extant and fossil African papionins using craniodental data. J. Hum. Evol. 64, 399–433. doi: 10.1016/j.jhevol.2013.01.013
Gilbert C. C., Frost S. R., Pugh K. D., Anderson M., Delson E. (2018). Evolution of the modern baboon (Papio hamadryas): a reassessment of the African Plio-Pleistocene record. J. Hum. Evol. 122, 38–69. doi: 10.1016/j.jhevol.2018.04.012
Gilbert C. C., Frost S. R., Strait D. S. (2009). Allometry, sexual dimorphism, and phylogeny: a cladistic analysis of extant African papionins using craniodental data. J. Hum. Evol. 57, 298–320. doi: 10.1016/j.jhevol.2009.05.013
Gilbert C. C., Goble E. D., Hill A. (2010). Miocene Cercopithecoidea from the Tugen Hills, Kenya. J. Hum. Evol. 59, 465–483. doi: 10.1016/j.jhevol.2010.05.005
Gilbert C. C., Grine F. E. (2010). Morphometric variation in the papionin muzzle and the biochronology of the South African Plio-Pleistocene karst cave deposits. Am. J. Phys. Anthropol. 141, 418–429. doi: 10.1002/ajpa.v141:3
Gill F. B. (2014). Species taxonomy of birds: which null hypothesis? Auk 131, 150–161. doi: 10.1642/AUK-13-206.1
Gingerich P. D. (1985). Species in the fossil record: concepts, trends, and transitions. Paleobiology 11, 27–41. doi: 10.1017/S0094837300011374
Godfrey L. R., Lyon S. K., Sutherland M. R. (1993). Sexual dimorphism in large-bodied primates: the case of the subfossil lemurs. Am. J. Phys. Anthropol. 90, 315–334. doi: 10.1002/ajpa.1330900306
Gommery D., Thackeray J. F., Sénégas F., Potze S., Kgasi L. (2008). The earliest primate (Parapapio sp.) from the Cradle of Humankind World Heritage site (Waypoint 160, Bolt’s Farm, South Africa). S. Afr. J. Sci. 104, 405–408.
Goodall J. (2016). “Foreward,” in All the World’s Primates. Eds. Rowe N., Meyers M. (Pogonias Press), v-vii, Charlestown.
Grabowski M., Hatala K. G., Jungers W. L., Richmond B. G. (2015). Body mass estimates of hominin fossils and the evolution of human body size. J. Hum. Evol. 85, 75–93. doi: 10.1016/j.jhevol.2015.05.005
Granger D. E., Stratford D., Bruxelles L., Gibbon R. J., Clarke R. J., Kuman K. (2022). Cosmogenic nuclide dating of Australopithecus at Sterkfontein, South Africa. Proc. Natl. Acad. Sci. U. S. A. 119, e2123516119. doi: 10.1073/pnas.2123516119
Granger D. E., Stratford D., Bruxelles L., Heaton J. L., Pickering T. R., Kuman K., et al. (2023). Monkey fossils do not negate cosmogenic dating at Sterkfontein. Proc. Natl. Acad. Sci. U. S. A. 120, e2300314120. doi: 10.1073/pnas.2300314120
Grieco T. M., Rizk O. T., Hlusko L. J. (2012). Data from: A modular framework characterizes micro- and macroevolution of Old World monkey dentitions (Dryad Digital Repository). doi: 10.5061/dryad.693j8
Grieco T. M., Rizk O. T., Hlusko L. J. (2013). A modular framework characterizes micro- and macroevolution of Old World monkey dentitions. Evolution 67, 241–259. doi: 10.1111/j.1558-5646.2012.01757.x
Haile-Selassie Y., Gibert L., Melillo S. M., Ryan T. M., Alene M., Deino A., et al. (2015). New species from Ethiopia further expands Middle Pliocene hominin diversity. Nature 521, 483–488. doi: 10.1038/nature14448
Halenar-Price L. B., Klukkert Z. S., Almonte-Milán J. N., Lehman P., Sims Z. R., Cooke S. B. (2024). Craniomandibular variation in the endemic Hispaniolan primate, Antillothrix bernensis. J. Hum. Evol. 196, 103589. doi: 10.1016/j.jhevol.2024.103589
Harrison M. J. (1988). A new species of guenon (genus Cercopithecus) from Gabon. J. Zool. 215, 561–575. doi: 10.1111/j.1469-7998.1988.tb02860.x
Harrison T. (2011). “Cercopithecids (Cercopithecidae, primates),” in Paleontology and Geology of Laetoli: Human Evolution in Context: Fossil Hominins and the Associated Fauna, vol. Vol. 2 . Ed. Harrison T. (Springer, Dordrecht), 83–139.
Hart J. A., Detwiler K. M., Gilbert C. C., Burrell A. S., Fuller J. L., Emetshu M., et al. (2012). Lesula: a new species of Cercopithecus monkey endemic to the Democratic Republic of Congo and implications for conservation of Congo’s central basin. PloS One 7, e44271. doi: 10.1371/journal.pone.0044271
Haughton S. (1925). Note on the occurrence of a species of baboon in limestone deposits near Taungs. T. R. Soc. S. Afr. 12, 68.
Heaton J. L. (2006). Taxonomy of the Sterkfontein fossil Cercopithecinae: the Papionini of Members 2 and 4 (Gauteng, South Africa). [Dissertation]. Indiana University, Bloomington (IN).
Hill J. E., DeVault T. L., Wang G., Belant J. L. (2020). Anthropogenic mortality in mammals increases with the human footprint. Front. Ecol. Environ. 18, 13–18. doi: 10.1002/fee.v18.1
Hlusko L. J. (2006). A new large Pliocene colobine species (Mammalia: Primates) from Asa Issie, Ethiopia. Geobios 39, 57–69. doi: 10.1016/j.geobios.2004.09.001
Hlusko L. J. (2007). A new late Miocene species of Paracolobus and other Cercopithecoidea (Mammalia: Primates) fossils from Lemudong’o, Kenya. Kirtlandia 56, 72–85.
Hlusko L. J., Mahaney M. C. (2007). A multivariate comparison of dental variation in wild and captive populations of baboons (Papio hamadryas). Arch. Oral. Biol. 52, 195–200. doi: 10.1016/j.archoralbio.2006.09.008
Hlusko L. J., Mahaney M. C. (2024). Data from: Dental linear metrics from a wild population of baboons (Papio cynocephalus), Kenya (Dryad Digital Repository). doi: 10.5061/dryad.sj3tx96d6
Hopley P. J., Maslin M. A. (2010). Climate-averaging of terrestrial faunas: an example from the Plio-Pleistocene of South Africa. Paleobiology 36, 32–50. doi: 10.1666/0094-8373-36.1.32
Hopwood A. T. (1936). New and little-known fossil mammals from the Pleistocene of Kenya Colony and Tanganyika Territory. J. Nat. Hist. 17, 636–641. doi: 10.1080/00222933608655164
Hunt G. (2004). Phenotypic variation in fossil samples: modeling the consequences of time-averaging. Paleobiology 30, 426–443. doi: 10.1666/0094-8373(2004)030<0426:PVIFSM>2.0.CO;2
Jablonski N. G. (2002). “Fossil Old World monkeys: the late Neogene radiation,” in The Primate Fossil Record. Ed. Hartwig W. C. (Cambridge University Press, Cambridge), 255–299.
Jablonski N. G., Frost S. R. (2010). “Cercopithecoidea,” in Cenozoic Mammals of Africa. Eds. Werdelin L., Sanders W. J. (University of California Press, Berkeley), 393–428.
Joganic J. L., Heuzé Y. (2019). Allometry and advancing age significantly structure craniofacial variation in adult female baboons. J. Anat. 235, 217–232. doi: 10.1111/joa.2019.235.issue-2
Jolly C. J. (2001). A proper study for mankind: analogies from the papionin monkeys and their implications for human evolution. Yearb. Phys. Anthropol. 116, 177–204. doi: 10.1002/(ISSN)1096-8644
Jones T. R. (1937). A new fossil primate from Sterkfontein, Krugersdorp, Transvaal. S. Afr. J. Sci. 33, 709–728.
Kaplan J. M., Pigliucci M., Banta J. A. (2015). Gould on Morton, Redux: what can the debate reveal about the limits of data? Stud. Hist. Philos. Sci. 52, 22–31. doi: 10.1016/j.shpsc.2015.01.001
Kassambara A. (2023). rstatix: pipe-friendly framework for basic statistical tests. Available online at: https://CRAN.R-project.org/package=rstatix (Accessed September 28, 2024).
Keyser A. W. (1991). The palaeontology of Haasgat: a preliminary account. Palaeontol. Afr. 28, 29–33.
Kibii J. M. (2007). Taxonomy, taphonomy and palaeoenvironment of hominid and non-hominid primates from the Jacovec Cavern, Sterkfontein. S. Afr. Archaeol. Bull. 62, 90–97.
Kowalewski M. (1996). Time-averaging, overcompleteness, and the geological record. J. Geol. 104, 317–326. doi: 10.1086/629827
Krishnamoorthy K., Lee M. (2014). Improved tests for the equality of normal coefficients of variation. Comput. Stat. 29, 215–232. doi: 10.1007/s00180-013-0445-2
Kullmer O., Sandrock O., Kupczik K., Frost S. R., Volpato V., Bromage T. G., et al. (2011). New primate remains from Mwenirondo, Chiwondo Beds in northern Malawi. J. Hum. Evol. 61, 617–623. doi: 10.1016/j.jhevol.2011.07.003
Latham A. G., McKee J. K., Tobias P. V. (2007). Bone breccias, bone dumps, and sedimentary sequences of the western Limeworks, Makapansgat, South Africa. J. Hum. Evol. 52, 388–400. doi: 10.1016/j.jhevol.2006.10.005
Leakey M. G., Teaford M. F., Ward C. V. (2003). “Cercopithecidae from Lothagam,” in Lothagam: The Dawn of Humanity in Eastern Africa. Eds. Leakey M. G., Harris J. M. (Columbia University Press, New York), 201–248.
Li L., Comi T. J., Bierman R. F., Akey J. M. (2024). Recurrent gene flow between Neanderthals and modern humans over the past 200,000 years. Science 385, eadi1768. doi: 10.1126/science.adi1768
Liedigk R., Roos C., Brameier M., Zinner D. (2014). Mitogenomics of the Old World monkey tribe Papionini. BMC Evol. Biol. 14, 176. doi: 10.1186/s12862-014-0176-1
Maier W. (1970). New fossil Cercopithecoidea from the lower Pleistocene cave deposits of the Makapansgat Limeworks, South Africa. Palaeontol. Afr. 13, 69–107.
Maier W. (1971). Two new skulls of Parapapio antiquus from Taung and a suggested phylogenetic arrangement of the genus Parapapio. Ann. S. Afr. Mus. 59, 1–16.
Marwick B., Krishnamoorthy K. (2019). cvequality: tests for the equality of coefficients of variation from multiple groups. Available online at: https://CRAN.R-project.org/package=cvequality (Accessed September 28, 2024).
Mayr E. (1950). Taxonomic categories in fossil hominids. Cold Spring Harb. Sym. 15, 109–118. doi: 10.1101/SQB.1950.015.01.013
Mayr E. (1963). “The taxonomic evaluation of fossil hominids,” in Classification and Human Evolution. Ed. Washburn S. L. (Aldine, Chicago), 332–346.
McHenry H. M. (1991). Sexual dimorphism in Australopithecus afarensis. J. Hum. Evol. 20, 21–32. doi: 10.1016/0047-2484(91)90043-U
McKee J. K., von Mayer A., Kuykendall K. L. (2011). New species of Cercopithecoides from Haasgat, North West Province, South Africa. J. Hum. Evol. 60, 83–93. doi: 10.1016/j.jhevol.2010.08.002
Meneganzin A., Stringer C. (2024). Homo sapiens, Neanderthals and speciation complexity in palaeoanthropology. Evol. J. Linn. Soc., kzae033. doi: 10.1093/evolinnean/kzae033
Mihlbachler M. C. (2008). Species taxonomy, phylogeny, and biogeography of the Brontotheriidae (Mammalia: Perissodactyla). B. Am. Mus. Nat. Hist. 2008, 1–475. doi: 10.1206/0003-0090(2008)501[1:STPABO]2.0.CO;2
Monson T. A. (2020). Patterns and magnitudes of craniofacial covariation in extant cercopithecids. Anat. Rec. 303, 3068–3084. doi: 10.1002/ar.v303.12
Monson T. A., Brasil M. F., Hlusko L. J. (2015). Materials collected by the southern branch of the UC Africa Expedition with a report on previously unpublished Plio-Pleistocene fossil localities. PaleoBios 32, ucmp_paleobios_29407. doi: 10.5070/P9321029407
Monson T. A., Brasil M. F., Stratford D. J., Hlusko L. J. (2017). Patterns of craniofacial variation and taxonomic diversity in the South African Cercopithecidae fossil record. Palaeontol. Electron. 20.1.7A, 1–20. doi: 10.26879/690
Nishimura T., Morimoto N., Ito T. (2019). Shape variation in the facial part of the cranium in macaques and African papionins using geometric morphometrics. Primates 60, 401–419. doi: 10.1007/s10329-019-00740-1
O’Regan H. J., Reynolds S. C. (2009). An ecological reassessment of the southern African carnivore guild: a case study from Member 4, Sterkfontein, South Africa. J. Hum. Evol. 57, 212–222. doi: 10.1016/j.jhevol.2009.04.002
Pallas L., Nakatsukasa M., Kunimatsu Y. (2024). Anatomy of the mandibular symphysis of extant cercopithecids: taxonomy and variation. bioRxiv. doi: 10.1101/2024.03.31.587451
Paterson H. E. H. (1985). “The recognition concept of species,” in Species and Speciation. Ed. Vrba E. S. (Transvaal Museum, Pretoria), 21–29.
Perelman P., Johnson W. E., Roos C., Seuánez H. N., Horvath J. E., Moreira M. A. M., et al. (2011). A molecular phylogeny of living primates. PloS Genet. 7, e1001342. doi: 10.1371/journal.pgen.1001342
Peters M. J., Marcus J. M. (2017). Taxonomy as a hypothesis: testing the status of the Bermuda buckeye butterfly Junonia coenia bergi (Lepidoptera: Nymphalidae). Syst. Entomol. 42, 288–300. doi: 10.1111/syen.2017.42.issue-1
Pickford M., Mein P., Senut B. (1992). Primate bearing Plio-Pleistocene cave deposits of Humpata, Southern Angola. Hum. Evolut. 7, 17–33. doi: 10.1007/BF02437475
Plavcan J. M. (1994). Comparison of four simple methods for estimating sexual dimorphism in fossils. Am. J. Phys. Anthropol. 94, 465–476. doi: 10.1002/ajpa.1330940403
Plavcan J. M. (2001). Sexual dimorphism in primate evolution. Yearb. Phys. Anthropol. 116, 25–53. doi: 10.1002/(ISSN)1096-8644
Plavcan J. M., Cope D. A. (2001). Metric variation and species recognition in the fossil record. Evol. Anthropol. 10, 204–222. doi: 10.1002/evan.20001
Pozzi L., Hodgson J. A., Burrell A. S., Sterner K. N., Raaum R. L., Disotell T. R. (2014). Primate phylogenetic relationships and divergence dates inferred from complete mitochondrial genomes. Mol. Phylogenet. Evol. 75, 165–183. doi: 10.1016/j.ympev.2014.02.023
Pugh K. D., Gilbert C. C. (2018). Phylogenetic relationships of living and fossil African papionins: Combined evidence from morphology and molecules. J. Hum. Evol. 123, 35–51. doi: 10.1016/j.jhevol.2018.06.002
Quintyn C. (2009). The naming of new species in hominin evolution: a radical proposal—a temporary cessation in assigning new names. Homo 60, 307–341. doi: 10.1016/j.jchb.2009.05.001
R Core Team (2019). R: A language and environment for statistical computing. Available online at: https://www.R-project.org/ (Accessed September 28, 2024).
Rehg J., Leigh S. (1999). Estimating sexual dimorphism and size differences in the fossil record: A test of methods. Am. J. Phys. Anthropol. 110, 95–104. doi: 10.1002/(SICI)1096-8644(199909)110:1<95::AID-AJPA8>3.0.CO;2-J
Reno P. L., Lovejoy C. O. (2015). From Lucy to Kadanuumuu: balanced analyses of Australopithecus afarensis assemblages confirm only moderate skeletal dimorphism. PeerJ 3, e925. doi: 10.7717/peerj.925
Reno P. L., Meindl R. S., McCollum M. A., Lovejoy C. O. (2003). Sexual dimorphism in Australopithecus afarensis was similar to that of modern humans. Proc. Natl. Acad. Sci. U. S. A. 100, 9404–9409. doi: 10.1073/pnas.1133180100
Roksandic M., Radović P., Wu X.-J., Bae C. J. (2022). Resolving the “muddle in the middle”: the case for Homo bodoensis sp. nov. Evol. Anthropol. 31, 20–29. doi: 10.1002/evan.21929
Rose K. D., Bown T. M. (1993). “Species concepts and species recognition in Eocene primates,” in Species, Species Concepts, and Primate Evolution. Eds. Kimbel W. H., Martin L. B. (Plenum Press, New York), 299–330.
Rossie J. B., Hill A. (2018). A new species of Simiolus from the middle Miocene of the Tugen Hills, Kenya. J. Hum. Evol. 125, 50–58. doi: 10.1016/j.jhevol.2018.09.002
Ruff C. B., Burgess M. L., Squyres N., Junno J.-A., Trinkaus E. (2018). Lower limb articular scaling and body mass estimation in Pliocene and Pleistocene hominins. J. Hum. Evol. 115, 85–111. doi: 10.1016/j.jhevol.2017.10.014
Singleton M. (2002). Patterns of cranial shape variation in the Papionini (Primates: Cercopithecinae). J. Hum. Evol. 42, 547–578. doi: 10.1006/jhev.2001.0539
Sneath P. H. A., Sokal R. R. (1973). Numerical Taxonomy. The Principles and Practice of Numerical Classification (San Francisco: Freeman).
Sokal R. R., Crovello T. J. (1970). The biological species concept: a critical evaluation. Am. Nat. 104, 127–153. doi: 10.1086/282646
Stratford D., Crompton R. (2021). Introduction to special issue: A 3.67 Ma Australopithecus prometheus skeleton from Sterkfontein Caves, South Africa. J. Hum. Evol. 158, 103008. doi: 10.1016/j.jhevol.2021.103008
Tattersall I. (2007). Madagascar’s lemurs: cryptic diversity or taxonomic inflation? Evol. Anthropol. 16, 12–23. doi: 10.1002/evan.20126
Taylor C. E., Brasil M. F., Monson T. A., Yohler R. M., Hlusko L. J. (2023). Halibee fossil assemblages reveal later Pleistocene cercopithecins (Cercopithecidae: Primates) in the Middle Awash of Ethiopia. Am. J. Biol. Anthropol. 180, 6–47. doi: 10.1002/ajpa.v180.1
Thackeray J. F. (2005). Stable carbon isotope analyses of Parapapio tooth enamel from Sterkfontein and Swartkrans: correspondence. S. Afr. J. Sci. 101, 483–484.
Thackeray F. J., Dumoncel J., Gommery D., Kgasi L., Tawane G. M., de Beer F. C., et al. (2019). Morphometric comparison of semicircular canals of Parapapio broomi and P. jonesi from Sterkfontein, South Africa. S. Afr. J. Sci. 115, 1–3. doi: 10.17159/sajs.2019/a0303
Thackeray J., Myer S. (2004). Parapapio broomi and Parapapio jonesi from Sterkfontein: males and females of one species? Ann. Transvaal Mus. 41, 79–82.
Tobias P. V., Hughes A. R. (1969). The new Witwatersrand University excavation at Sterkfontein: progress report, some problems and first results. S. Afr. Archaeol. Bull. 24, 158–169. doi: 10.2307/3888295
Van Valen L. (1976). Ecological species, multispecies, and oaks. Taxon 25, 233–239. doi: 10.2307/1219444
Villmoare B. (2005). Metric and non-metric randomization methods, geographic variation, and the single-species hypothesis for Asian and African Homo erectus. J. Hum. Evol. 49, 680–701. doi: 10.1016/j.jhevol.2005.07.005
Villmoare B., Hatala K. G., Jungers W. (2019). Sexual dimorphism in Homo erectus inferred from 1.5 Ma footprints near Ileret, Kenya. Sci. Rep. 9, 7687. doi: 10.1038/s41598-019-44060-2
White T. D. (2014). Delimitating species in paleoanthropology. Evol. Anthropol. 23, 30–32. doi: 10.1002/evan.21391
White T. D., Moore R. V., Suwa G. (1984). Hadar biostratigraphy and hominid evolution. J. Vertebr. Paleontol. 4, 575–583. doi: 10.1080/02724634.1984.10012033
Wiley E. O. (1978). The evolutionary species concept reconsidered. Syst. Zool. 27, 17–26. doi: 10.2307/2412809
Williams F., Ackermann R., Leigh S. (2007). Inferring Plio-Pleistocene southern African biochronology from facial affinities in Parapapio and other fossil papionins. Am. J. Phys. Anthropol. 132, 163–174. doi: 10.1002/ajpa.v132:2
Keywords: Cercopithecidae, taxonomy, primate evolution, sexual dimorphism, variation, Parapapio
Citation: Brasil MF, Monson TA, Stratford DJ and Hlusko LJ (2025) A hypothesis-based approach to species identification in the fossil record: a papionin case study. Front. Ecol. Evol. 12:1481903. doi: 10.3389/fevo.2024.1481903
Received: 16 August 2024; Accepted: 06 December 2024;
Published: 03 January 2025.
Edited by:
Laura M. Martínez, University of Barcelona, SpainReviewed by:
Francisco Juan Prevosti, Museo de Antropología y Ciencias Naturales de la UNLAR. CONICET, ArgentinaCopyright © 2025 Brasil, Monson, Stratford and Hlusko. This is an open-access article distributed under the terms of the Creative Commons Attribution License (CC BY). The use, distribution or reproduction in other forums is permitted, provided the original author(s) and the copyright owner(s) are credited and that the original publication in this journal is cited, in accordance with accepted academic practice. No use, distribution or reproduction is permitted which does not comply with these terms.
*Correspondence: Marianne F. Brasil, YnJhc2lsbUB3d3UuZWR1
Disclaimer: All claims expressed in this article are solely those of the authors and do not necessarily represent those of their affiliated organizations, or those of the publisher, the editors and the reviewers. Any product that may be evaluated in this article or claim that may be made by its manufacturer is not guaranteed or endorsed by the publisher.
Research integrity at Frontiers
Learn more about the work of our research integrity team to safeguard the quality of each article we publish.