- 1Haub School of Environment and Natural Resources, University of Wyoming, Laramie, WY, United States
- 2Department of Zoology and Physiology, University of Wyoming, Laramie, WY, United States
- 3Wyoming Game and Fish Department, Lander, WY, United States
- 4National Wildlife Research Center, USDA APHIS Wildlife Services, Fort Collins, CO, United States
That predators ‘cull the sick and the weak’ is an adage in ecological texts, but the mechanisms by which disease is curtailed within ecosystems has puzzled ecologists for many years. Advances in our understanding of host-pathogen interactions have revealed defense mechanisms implemented by hosts that minimize infectious diseases in wild populations. Defense mechanisms for hosts include adaptations that ameliorate fitness loss or preemptively limit pathogen exposure, and these mechanisms underlie fundamental questions about how scavenging or predation influence pathogen transmission. A key lens for our understanding of predator-prey and scavenging dynamics include behaviorally-mediated trade-offs weighed by consumers between nutritional gains and pathogen exposure risks. Consequently, the degree to which pathogens and associated diseases perpetuate through food webs can be partly attributed to behavioral responses of predators and scavengers, particularly their selection or avoidance of diseased prey and infected carcasses. Even so, examinations of avoidance or preference by predators and scavengers to diseased carrion are underrepresented. Here we identify areas for future research focused on behavioral immunity that could illuminate where, when, and how pathogen transmission reverberates through ecological communities. While directly attributing behavioral responses to pathogen exposure may be challenging, particularly for organisms with low susceptibility to spillover, identifying these responses though experimentation or observation help describe complex systems regarding infectious disease.
1 Introduction
Because predation and scavenging often complete parasite life-cycles and promote pathogen transmission, scavenger-prey interactions lie at the heart of many topics in disease ecology. Consumption is often required for pathogen persistence, as a pathway from intermediate to definitive hosts where replication or reproduction occurs (Lafferty, 1999). Depending on the pathogen involved, its pathogenicity, virulence, and the predator-host response, these life-cycle stage impacts may range from minimal to detrimental within the definitive host. Consequently, many hosts have evolved behavioral, physiological, or genetic defense mechanisms to avoid infectious disease. Primary defense mechanisms for hosts include adaptations such as avoidance, resistance, and tolerance – all of which can ameliorate fitness loss or preemptively limit exposure to pathogens (Medzhitov et al., 2012; Curtis, 2014).
In response, pathogens can evolve traits that alter host behavior or impact host physiology or genetics to increase the likelihood of transmission. These responses are well described in many systems and provide foundational support for theories of parasite-host co-evolution (Figure 1). However, two of these mechanisms occur strictly within the host – tolerance and resistance. While resistance largely relies on host immune responses that reduce pathogen load, tolerance commonly reduces immune responses that cause tissue damage in an effort to promote host health, having little effect on the pathogen (McCarville and Ayres, 2018). While all mechanisms result in fitness consequences for the host, avoidance seems the only strategy that is behaviorally driven (i.e., behavioral immunity [de Roode and Lefèvre, 2012]), requiring no internal physiological responses or adaptations within the host. Therefore, pathogen avoidance is a primary factor explicitly linked to the prevention, not just mitigation, of infectious disease.
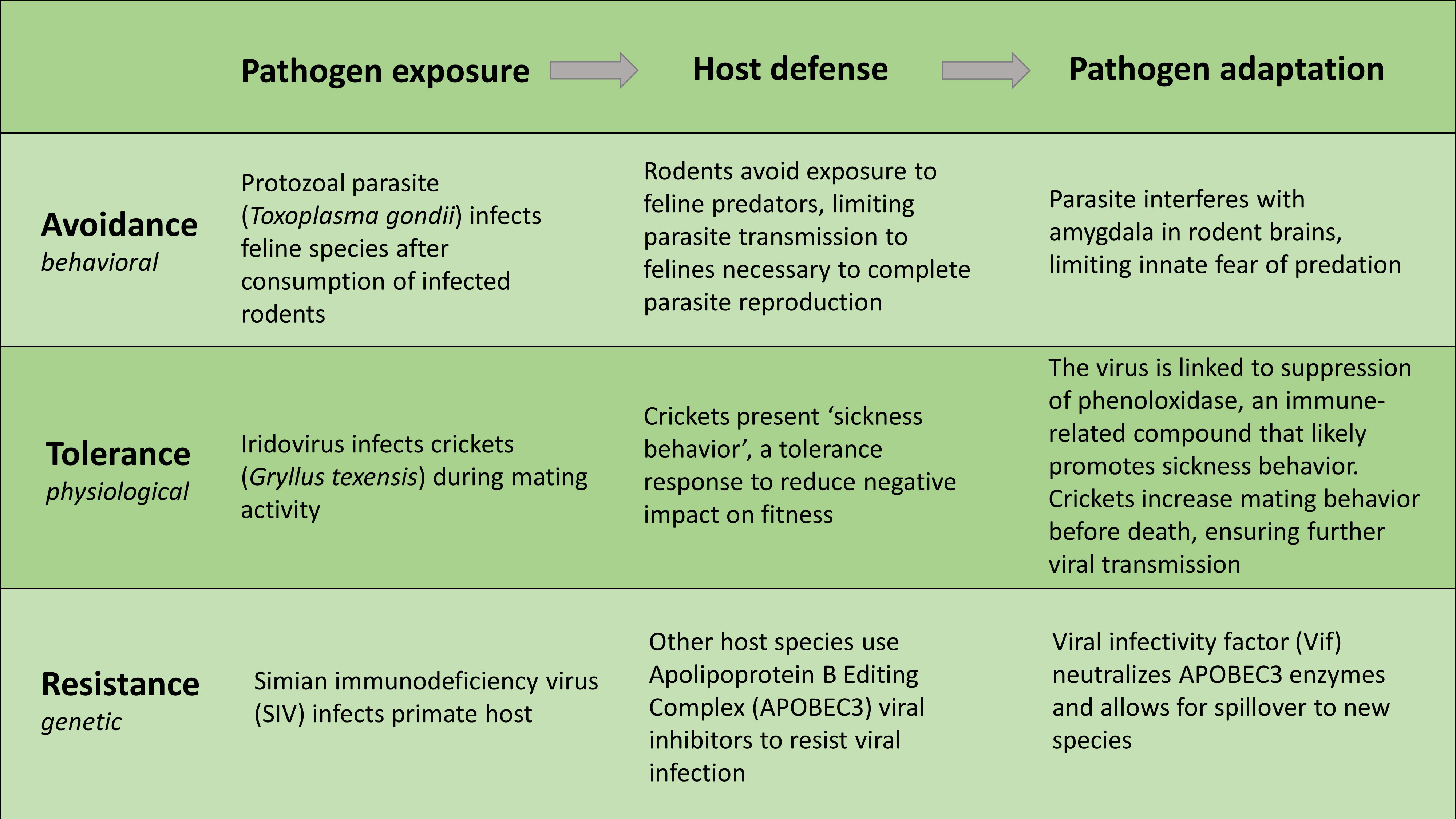
Figure 1. The host-pathogen arms race describes ongoing adaptations of both parasites and hosts that drive co-evolution (Wang et al., 2021). Figure examples of pathogen adaptations to compensate for host responses to exposure have been identified across various host defense mechanisms including avoidance (Tong et al., 2021), tolerance (Adamo et al., 2014), and resistance (Nakano et al., 2020). Defenses can also manifest behaviorally, physiologically, or genetically; supporting co-evolution theory suggesting pathogens often find pathways to avoid, influence, or circumvent host defenses to promote their fitness and increase transmission.
While widely acknowledged that predators select infected prey and scavengers consume diseased carrion (Lafferty, 1992; Behringer et al., 2018; Vicente and VerCauteren, 2019), evidence of preference against diseased resources also exist to support theory on pathogen avoidance (e.g., Sarabian et al., 2023). Here, we discuss underlying mechanisms of behavioral pathogen avoidance and potential ramifications for disease transmission. Hypotheses concerning behavioral-driven foraging strategies are predicated upon trade-offs in nutritional gains, which incorporate evolutionary adaptations to pathogen susceptibility as well as awareness of pathogen exposure risk. Although these strategies drive fundamental questions about how active predation or scavenging influence pathogen transmission, examinations of avoidance by predators and scavengers to diseased carrion are underrepresented, and more data is needed through experimental or observational studies to disentangle behaviorally-driven trade-offs that influence disease dynamics in natural systems.
2 Pathogen avoidance and trade-off hypotheses – from microbes to mammals
Pathogen avoidance behaviors have been identified and studied in many systems, at multiple trophic levels, and across various taxa. At the microbial level, C. elegans nematodes apply learned behavior, using neuronal sensory mechanisms to identify and subsequently avoid harmful bacteria (Meisel and Kim, 2014). Fruit flies (Drosophila nigrospiracula) have been shown to use phototaxis, selectively moving along light−dark gradients to limit exposure to parasitic mites (Macrocheles subbadius [Horn et al., 2022]). Avian species are of particular interest, as they implement multiple strategies to minimize pathogen exposure, including hygiene and body maintenance (e.g., preening, sunning, dusting, bathing) as well as avoidance behaviors including pathogen consideration during nest site selection or even nest abandonment to actively avoid parasites (Bush and Clayton, 2018). Examples of pathogen avoidance range from insects (Eakin et al., 2014) to aquatic organisms (Behringer et al., 2006) to wild ungulates (Ezenwa, 2004) to primates (Sarabian and MacIntosh, 2015); indeed, behaviorally-mediated strategies to avoid infectious disease occur in nearly every relevant biotic system.
Specific to predator-prey and scavenging systems, avoidance is often described in the context of trade-offs (Fry, 1996), where risks of tropically-transmitted pathogen exposure are weighed against nutritional gains acquired through consumption (e.g., Oliva-Vidal et al., 2021). While it is generally accepted that the majority of infected prey are not avoided but rather selected by predators (Lafferty, 1992; Behringer et al., 2018), examples of reduced consumption of infected prey also exist (Figure 2). Two primary pathways mediate this process. The first is driven through pathogen influence on infected prey to avoid predation. An interesting example by Soghigian et al. (2017) described an obligate predator (Toxohrynchites rutilus) exposed to Ascogregarina barretti-infected and uninfected mosquito larvae (Aedes triseriatus) resulted in lower predation on infected larvae. The authors concluded that the parasite influenced larval behavior to seek refuge from predation, likely because there was no benefit of trophic transmission from the definitive larval host to the dead-end predator species. In another example, tiger salamander (Ambystoma tigrinum stebbinsi) larvae exposed to Ambystoma tigrinum virus (ATV) incurred lower predation from dragonflies (Anax junius) than uninfected salamanders, but showed significantly higher activity in the absence of predators. Again, because this single-host pathogen relies on prey host survival for transmission, it was concluded that the virus increased anti-predator responses within its host (Parris et al., 2004).
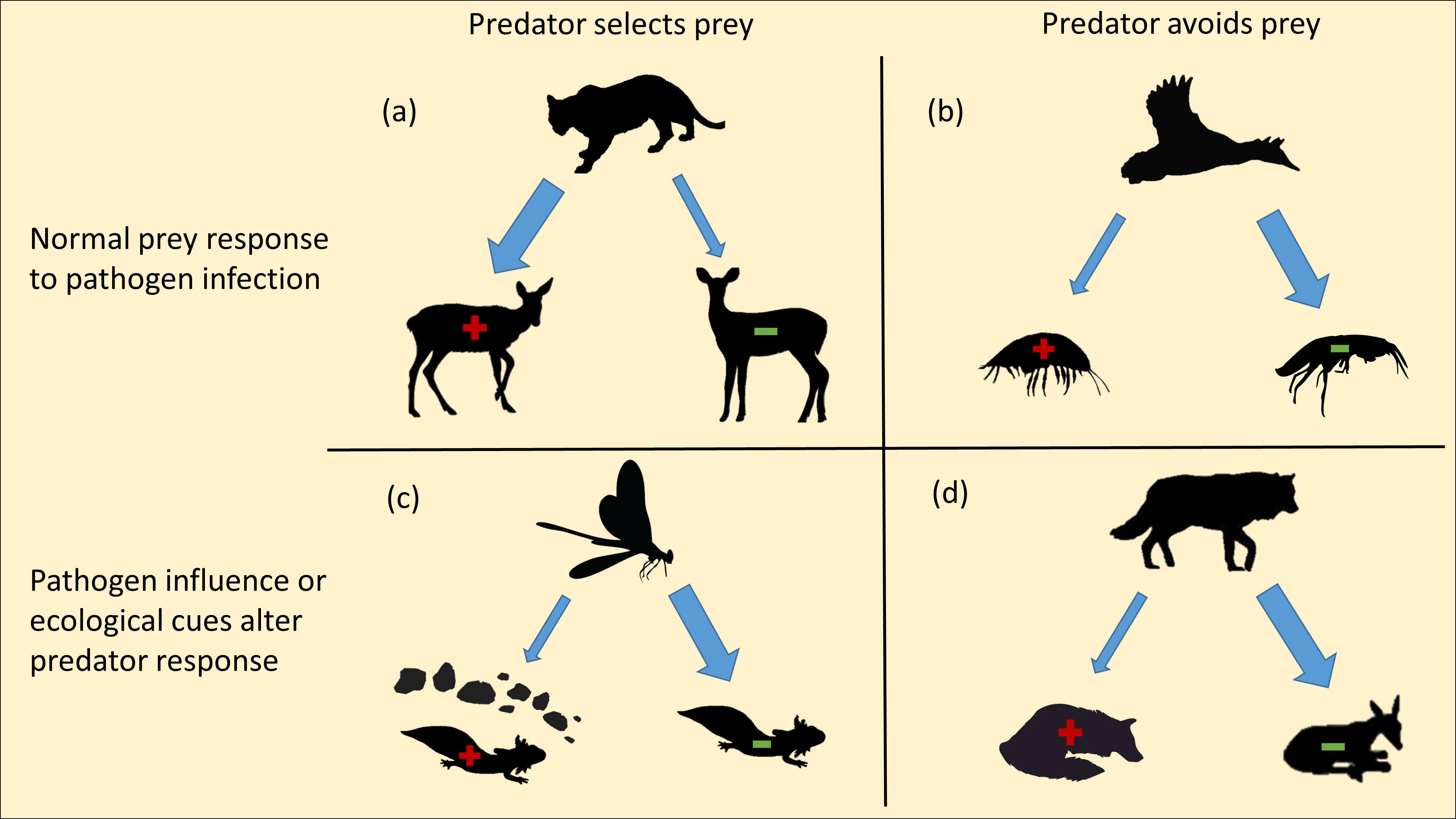
Figure 2. Differing scenarios with evidence of selection or avoidance of pathogens by predators or scavengers under various mechanisms that could influence outcomes. (A) Mountain lions select for CWD-infected deer (Krumm et al., 2010). (B) Stellar’s eiders avoid infected amphipods (Bustnes and Galaktionov, 2004). (C) Viral pathogen influences salamander larvae to avoid predation by dragonflies (Parris et al., 2004). (D) Carnivore-carrion hypothesis shows scavengers select against carrion of similar species (Moleón et al., 2017). Green symbols indicate reduced disease, red symbols indicate high disease.
The next more obvious mechanism of reduced consumption is through direct avoidance of infected prey by the predator itself. For instance, near-starvation was the primary state in which Stellar’s eiders (Polysticta stelleri) would consume parasitized amphipods (Bustnes and Galaktionov, 2004); otherwise, they exhibited avoidance. Additionally, a meta-analysis conducted by Flick et al. (2016) concluded that predators preferred healthy over infected prey, and because fitness decreased in predators that consumed pathogen-infected prey, these items were considered a low-quality resource. While these examples are limited in comparison to predator selection for infected prey, avoidance or preference against infected prey is certainly more difficult to identify in wild populations, and therefore most occurrences are documented within controlled laboratory experiments (Sarabian et al., 2023). It has been suggested, however, that these processes are more widespread than current evidence suggests (Buck et al., 2018).
Trade-offs may be readily apparent when the predator or scavenger is aware of the risk, but even without direct knowledge, tendencies in differential prey selection or carrion partitioning by scavengers exists. For example, phylogenetic similarity has been linked to scavenging behavior, particularly in the carnivore-carrion avoidance hypothesis, where predators and scavengers avoid carnivore carrion and cannibalism (Moleón et al., 2017; Peers et al., 2021). Carrion partitioning has been further extended to community-level responses, where carnivore-carrion avoidance by vertebrate scavengers increased carrion insect diversity (Muñoz-Lozano et al., 2019). These behavioral shifts can cause a competitive release, increasing scavenger richness and potentially contributing to a ‘dilution effect’ where high consumer diversity correlates to reduced infection prevalence (Civitello et al., 2015; Khalil et al., 2016). Pfennig (2000) describe theory on the trade-off between pathogen exposure risk when consuming phylogenetically similar species and the nutritional gains acquired from predation, including an experimental approach that resulted in differing host fitness (quantified by growth rate) of Scaphiopus couchii tadpoles when fed infected conspecific versus allospecific prey. However, stochastic environmental conditions lending to poor resource availability (Oliva-Vidal et al., 2021), or relatively high carrion abundance (Cagnacci et al., 2003; Remonti et al., 2005), may override avoidance behaviors, making state-dependent factors such as hunger a confounding component in the general avoidance of conspecific prey or carrion. This is analogous to the starvation-predation hypothesis (Sinclair and Arcese, 1995), which suggests animals are more inclined to engage with risky circumstances as they experience sustained hunger.
Although cross-species transmission positively correlates with host phylogenetic relatedness, further examination is warranted to determine the degree at which parasites may be shared among hosts, particularly within predation interactions (Stephens et al., 2019). Extent, duration, or magnitude of exposure to various pathogens through predation or scavenging may correlate with spillover probability to non-target species. For example, the bioaccumulation of various pathogens transmitted through consumptive predation could have cumulative or additive effects that increase the likelihood of spillover infections (Malmberg et al., 2021). In addition, generalist predators are exposed to a broader spectrum of pathogens as they consume a wider variety of intermediate hosts (Scholz et al., 2020), allowing increased potential for parasite adaptations or co-infections that could increase novel spillover risk.
Some hosts directly identify the pathogen (typically a parasite or vector) they are intentionally trying to avoid (e.g., caribou avoidance of mosquito swarms [Johnson et al., 2021]), while others identify pathogens through various sensory mechanisms (e.g., discrimination against detectable fungi in ants [Pereria and Detrain, 2020]; bacteria detection and avoidance by C. elegans [Meisel and Kim, 2014]). Therefore, it is generally accepted that avoidance requires the host to recognize pathogen risk, typically through olfactory, gustatory, or visual cues (Medzhitov et al., 2012; Sarabian et al., 2018). Even tactile cues have been investigated as a sensory mechanism to identify pathogen presence (Oum et al., 2011). On the contrary, evolutionary responses to environmental or behavioral cues may not be directly associated with known pathogens. The ‘ecology of disgust’ (Buck et al., 2018; Sarabian et al., 2023), described in relation to the ‘ecology of fear’ (Clinchy et al., 2013), can both be generalized as the non-consumptive effects of risk avoidance in natural systems, as well as the trophic consequences thereof. Although the ‘ecology of fear’ is contextualized as avoidance of predation, while ‘ecology of disgust’ aligns with avoidance of disease, both are predicated upon environmental cues that trigger avoidance behavior, often times without direct evidence of risk. Furthermore, evidence suggests that disgust-mediated avoidance behavior is not only learned, but an innate process (Curtis, 2014). For instance, the carnivore-carrion avoidance hypothesis portrays no specific disease may be evident, although carnivores do generally carry more helminths than their ungulate prey (Stephens et al., 2019). Also, recent experimental evidence has shown large group sizes of carrion on the landscape impart environmental cues that suggest a disease-related die-off. These larger groups incurred lower scavenging rates than single carcasses, indicating behavioral avoidance by scavengers due to disease risk (Olson et al., 2022). These cues to ambiguous pathogen exposure extend well into human psychology (Curtis et al., 2004), even so far as to demonstrate that disease cues led women to avoid asymmetrical male faces thought to be linked to immunocompromised individuals (Ainsworth and Maner, 2019). Also, the tendency to generally avoid conspecifics is common across many species, and reductions in group sizes of social animals reduces exposure to pathogens (Curtis, 2014). Ultimately, although specific pathogens may be identified and behaviorally avoided after a learned experience (Meisel and Kim, 2014), general avoidance driven by ecological cues may result in these behaviors even when no detrimental pathogens exist; a sometimes costly consequence of avoidance.
3 Pathogen persistence and environmental reservoirs
Within predator-prey disease dynamics, specific pathogens are likely disproportionally impacted by disease avoidance. The ability for pathogens to persist outside a live host influence this process, such as the variable persistence of viruses − largely impacted by dynamic abiotic conditions (Labadie et al., 2020). Infectious bacterial pathogens also vary in persistence, from moderate persistence of Brucella abortus bacteria that result in bovine brucellosis (21 − 81 days [Aune et al., 2011]), to highly persistent anthrax spores (Bacillus anthracis) which can remain infectious for decades (Carlson et al., 2018). However, assemblages of prion pathogens may be considered some of the most resilient infectious agents identified to date. For example, chronic wasting disease (CWD) represents one unique form of many transmissible spongiform encephalopathy diseases that result from the transmission of misfolded infectious proteins, or prions (Escobar et al., 2020). These prions have fatal neurodegenerative effects on infected individuals, with CWD specifically adapted to multiple wild cervid species (Haley and Hoover, 2015). Because of the complexities involved in CWD transmission in wild populations, including predation and scavenging of diseased prey and multiple modes of transmission for prions, this disease provides a unique system for consideration of pathogen avoidance, which we discuss in further detail.
Selective predation influences disease dynamics and system complexity, with outcomes dependent upon factors such as the degree of selection, predation intensity, and overall ecosystem productivity (Hall et al., 2005; Brandell et al., 2022). In fact, CWD models incorporating highly selective predation versus random removal of host species predicted rapid declines in disease prevalence and even elimination of the pathogens in closed population models (Wild et al., 2011). Specifically, some evidence suggests that apex predators such as mountain lions (Puma concolor) show selection for CWD-infected mule deer (Odocoileus hemionus [Krumm et al., 2010]) that may limit prevalence of the disease in wild populations (Fisher et al., 2022). At this time, CWD in cervids has no identifiable negative fitness consequences for consumers of ungulates that harbor the prion pathogens (e.g., Jennelle et al., 2009), and optimal foraging suggests shifts away from diseased resources should only be observed if there is a high fitness cost of becoming infected (Lozano, 1991). However, the disease itself manifests obvious physiological symptoms and conditions that indicate disease (Mysterud and Edmunds, 2019), potentially activating avoidance in some consumer species via ‘ecology of disgust’ theory. Interestingly, anecdotal evidence from our observations within endemic CWD regions suggest scavengers often avoid carcasses of ungulates which succumb to clinical infection, resulting in carcasses persisting on the landscape longer than mortalities not associated with the disease. In addition, observations during ungulate necropsies suggest olfactory and visual cues indicative of CWD infection emanate from CWD-infected carcasses during inspection. Because it is generally accepted that animal sense and perception is more acute and attuned than of humans (Scanes, 2018), it is unlikely these cues would go unnoticed by scavengers.
Why is this important? Although CWD infection occurs by direct transmission through live animal interactions, environmental transmission also provides a pathway of infection to new hosts (Escobar et al., 2020). Because CWD prions are environmentally maintained within soil, various fomites, and even taken up by plants (Escobar et al., 2020), the impact of local infectious zones (LIZ) in the environment created by the death of infected organisms can have major consequences to efforts aimed at reducing pathogen spread (Lozano, 1991). While many disease models (e.g., SIR1 [Bjørnstad et al., 2020]) depend on estimates of host ‘infectious periods’, they cannot easily account for LIZ impacts, and the interminable persistence of environmental prions compound the risk of exposure through LIZs both spatially and temporally (Blackburn et al., 2019). Accordingly, evidence suggests CWD disease dynamics may heavily shift toward these environmental reservoirs, exacerbating disease spread and rendering current management efforts less effective in mitigating CWD (Almberg et al., 2011).
Because vertebrate scavengers are positively associated with the speed at which carcasses decompose (Wenting et al., 2022), preferences associated with scavenging behavior can impact the persistence of prions within the environment associated with diseased carrion. It should be noted, however, that scenario-dependent feeding behaviors, movement characteristics, or digestive processes of consumers can influence pathogen transmission trajectories (Duffy et al., 2019); although the net impacts of these processes are largely unexplored in natural systems (Box 1). Even if unrelated to pathogen avoidance, various scavenging strategies including responses to carcass size (Moleón et al., 2015), carcass type (Olson et al., 2016), or alternative prey availability (Peers et al., 2020) likely influence environmental pathogen persistence in carrion. Resultantly, the hardy nature of prions render viable pathogen transmission to new host species that can extend years (Haley and Hoover, 2015). Therefore, consumptive processes that may dilute, sequester, or eliminate pathogens can impede the spread of disease, but disentangling these dynamics requires increasing our understanding of scavenging patterns (Carrasco-Garcia et al., 2018).
Box 1. Consequences of Scavenging: Increased or decreased pathogen transmission?
The contention over carrion-mediated transmission of CWD lies in whether the consumption of infected tissues reduces prion load and new host accessibility, or if the spatial distribution and movement ecology of consumers exacerbates the spread of prions in the environment (I). Predators and scavengers alike may resist infection of high concentrations of CWD prions in similar fashion as identifiable genetic adaptations in other species that provide resistance to a variety of harmful pathogens (e.g., vultures [Chung et al., 2015]), or through natural spillover barriers that exist among different carnivore species (Otero et al., 2015; Wolfe et al., 2022). Concerning the spread and transmission of CWD, eliminating or sequestering prions through digestion has been found, with studies showing both ephemeral persistence and significant reductions in prion inoculum after being passed through carnivore digestive systems (Nichols et al., 2015; Buane et al., 2021). However, even if these reductions occur, passage of viable prions through the digestive track of predators and scavengers persist nonetheless, and have been identified in multiple species (Fischer et al., 2013; Nichols et al., 2015; Buane et al., 2021). Ultimately, while evidence suggests that scavenging likely limits disease spread in most scenarios, even if scavengers become infected (Le Sage et al., 2019; Vicente and VerCauteren, 2019), others contend scavengers can play an active role in perpetuating disease (Carucci et al., 2022).
I. Various consumers encounter CWD-infected carcasses in natural systems. Species range across taxa and likely vary in their ability to promote or impede prion transmission.
4 Conclusions
Advances in infectious disease research continuously unveil systems and processes which bolster our understanding of the host-pathogen interface. While much research has focused on how pathogens interact within hosts post-inoculation, less focus has been placed on investigating behavioral mechanisms of pathogen engagement or avoidance. For infectious diseases like CWD, obvious best-case scenarios include a combination of high selectivity of infected prey by predators, and near-absolute consumption of diseased carrion. These mechanisms, in tandem, minimize horizontal transmission from live infectious animals and limit the development of environmental LIZs created through infected prey mortalities. While research has been applied to show these two mechanisms exist, we have asked comparatively few questions concerning the situations where they do not. Within predator-prey or scavenging systems, is avoidance of infected organisms more common than we suspect in natural systems, or is it simply more difficult to detect and therefore less apparent outside of controlled laboratory experiments? The combination of ecological theory supporting behavioral pathogen avoidance, and limited yet compelling evidence of its existence in natural systems warrants consideration of these processes. As increasing evidence mounts to support behaviorally-mediated avoidance (Sarabian et al., 2023), questions also remain as to what degree predators and scavengers require cognizance of the risk posed by specific pathogens in order to influence their feeding behavior, or alternatively if innate responses to ambiguous disease cues largely impact disease-nutrition trade-offs? Particularly for scavenging, if carrion are not directly avoided by scavengers because of disease cues, but instead due to cues associated with poor carrion condition confounded by disease status, preferences against these low-quality resources would still play a key role in infectious disease dynamics. Finally, if both theory of selection for diseased prey by predators and theory of avoidance of diseased carrion by scavengers hold, thinking critically about these seemingly opposing forces on the status, transmission, and sustainability of infectious diseases in wild populations will be essential to mitigate or manage their spread. Therefore, identifying and understanding behavioral immunity in natural systems among predator and scavenger species, even with low likelihood of spillover risk, will enhance our understanding of how species’ may behaviorally promote or prevent infectious disease.
Data availability statement
The original contributions presented in the study are included in the article/supplementary material. Further inquiries can be directed to the corresponding author.
Author contributions
JC: Conceptualization, Writing – original draft, Writing – review & editing. JM: Conceptualization, Writing – review & editing. JH: Conceptualization, Supervision, Writing – review & editing.
Funding
The author(s) declare that no financial support was received for the research, authorship, and/or publication of this article.
Conflict of interest
The authors declare that the research was conducted in the absence of any commercial or financial relationships that could be construed as a potential conflict of interest.
Publisher’s note
All claims expressed in this article are solely those of the authors and do not necessarily represent those of their affiliated organizations, or those of the publisher, the editors and the reviewers. Any product that may be evaluated in this article, or claim that may be made by its manufacturer, is not guaranteed or endorsed by the publisher.
Footnotes
- ^ Compartmental model of Susceptible, Infectious, and/or Recovered cohorts to describe spread of disease.
References
Adamo S. A., Kovalko I., Easy R. H., Stoltz D. (2014). A viral aphrodisiac in the cricket (Gryllus texensis). J. Exp. Biol. 217, 19701976. doi: 10.1242/jeb.103408
Ainsworth S. E., Maner J. K. (2019). Pathogen avoidance mechanisms affect women’s preference for symmetrical male faces. Evol. Behav. Sci. 13, 265271. doi: 10.1037/ebs0000139
Almberg E. S., Cross P. C., Johnson C. J., Heisey D. M., Richards B. J. (2011). Modeling routes of chronic wasting disease transmission: environmental prion persistence promotes deer population decline and extinction. PLoS One 6, e19896. doi: 10.1371/journal.pone.0019896
Aune K., Rhyan J. C., Russell R., Roffe T. J., Corso B. (2011). Environmental persistence of Brucella abortus in the Greater Yellowstone Ecosystem. J. Wild. Mngmt. 76, 253261. doi: 10.1002/jwmg.274
Behringer D. C., Butler M. J., Shields J. D. (2006). Avoidance of disease by social lobsters. Nature 441, 4211. doi: 10.1038/441421a
Behringer D. C., Karvonen A., Bojko J. (2018). Parasite avoidance behaviours in aquatic environments. Phil. Trans. R. Soc B. 373, 20170202. doi: 10.1098/rstb.2017.0202
Bjørnstad O. N., Shea K., Krzywinski M., Altman N. (2020). Modeling infectious epidemics. Nat. Methods 17, 455–456. doi: 10.1038/s41592-020-0822-z
Blackburn J. K., Ganz H. H., Ponciano J. M., Turner W. C., Ryan S. J., Kamath P., et al. (2019). Modeling R0 for pathogens with environmental transmission: animal movements, pathogen populations, and local infectious zones. Int. J. Environ. Res. Public Health 16, 954. doi: 10.3390/ijerph16060954
Brandell E. E., Cross P. C., Smith D. W., Rogers W., Galloway N. L., MacNulty D. R., et al. (2022). Examination of the interaction between age-specific predation and chronic disease in the Greater Yellowstone Ecosystem. J. Anim. Ecol. 91, 1373–1384. doi: 10.1111/1365-2656.13661
Buane C., Wolfe L. L., Schott K. C., Griffin K. A. (2021). Reduction of chronic wasting disease prion seeding activity following digestion by mountain lions. msphere 6, e00812–e00821. doi: 10.1128/msphere.00812-21
Buck J. C., Weinstein S. B., Young H. S. (2018). Ecological and evolutionary consequences of parasite avoidance. Trends Ecol. Evol. 33, 619–632. doi: 10.1016/j.tree.2018.05.001
Bush S. E., Clayton D. H. (2018). Anti-parasite behaviour of birds. Phil. Trans. R. Soc B. 373, 20170196. doi: 10.1098/rstb.2017.0196
Bustnes J. O., Galaktionov K. V. (2004). Evidence of a state-dependent trade-off between energy intake and parasite avoidance in Steller’s eiders. Can. J. Zool. 82, 1566–1571. doi: 10.1139/z04-139
Cagnacci F., Lovari S., Meriggi A. (2003). Carrion dependence and food habits of the red fox in an alpine area. Ital. J. Zool. 70, 3138. doi: 10.1080/11250000309356493
Carlson C. J., Getz W. M., Kausrud K. L., Cizauskas C. A., Blackburn J. K., Bustos Carrillo F. A., et al. (2018). Spores and soil from six sides: interdisciplinarity and the environmental biology of anthrax (Bacillus anthracis). Biol. Rev. 93, 1813–1831. doi: 10.1111/brv.2018.93.issue-4
Carrasco-Garcia R., Barroso P., Perez-Olivares J., Montoro V., Vicente J. (2018). Consumption of big game remains by scavengers: a potential risk as regards disease transmission in central Spain. Front. Vet. Sci. 5, 4. doi: 10.3389/fvets.2018.00004
Carucci T., Whitehouse-Tedd K., Yarnell R. W., Collins A., Fitzpatrick F., Botha A., et al. (2022). Ecosystem services and disservices associated with vultures: A systematic review and evidence assessment. Ecosyst. Serv. 56, e101447. doi: 10.1016/j.ecoser.2022.101447
Chung O., Jin S., Cho S., Lim J., Kim H., Jho S., et al. (2015). The first whole genome and transcriptome of the cinereous vulture reveals adaptation in the gastric and immune defense systems and possible convergent evolution between the Old and New World vultures. Geno. Biol. 16, 215. doi: 10.1186/s13059-015-0780-4
Civitello D. J., Cohen J., Fatima H., Halstead N. T., Liriano J., McMahon T. A., et al. (2015). Biodiversity inhibits parasites: broad evidence for the dilution effect. Proc. Natl. Acad. Sci. 112, 8667–8671. doi: 10.1073/pnas.1506279112
Clinchy M., Sheriff M. J., Zanette L. Y. (2013). Predator-induced stress and the ecology of fear. Funct. Ecol. 27, 5665. doi: 10.1111/fec.2013.27.issue-1
Curtis V. A. (2014). Infection-avoidance behaviour in humans and other animals. Trends Immun. 35, 457464. doi: 10.1016/j.it.2014.08.006
Curtis V., Aunger R., Rabie T. (2004). Evidence that disgust evolved to protect from risk of disease. Proc. Biol. Sci. 271, S131–S133. doi: 10.1098/rsbl.2003.0144
de Roode J. C., Lefèvre T. (2012). Behavioral immunity in insects. Insects 3, 789820. doi: 10.3390/insects3030789
Duffy M., Cáceres C. E., Hall S. R. (2019). “Healthy herds or predator spreaders? Insights from plankton into how predators suppress and spread disease.” in Wildlife Disease Ecology: Linking Theory to Data and Application eds (Wilson K., Fenton A., Tompkins D.) (Cambridge, UK: Cambridge University Press), 458–474.
Eakin L., Wang M., Dwyer G. (2014). The effects of the avoidance of infectious hosts on infection risk in an insect-pathogen interaction. Am. Nat. 185, 100–112. doi: 10.1086/678989
Escobar L. E., Pritzkow S., Winter S. N., Grear D. A., Kirchgessner M. S., Dominguez-Villegas E., et al. (2020). The ecology of chronic wasting disease in wildlife. Biol. Rev. 95, 393–408. doi: 10.1111/brv.12568
Ezenwa V. O. (2004). Selective defecation and selective foraging: antiparasite behavior in wild ungulates? Ethology 110, 851862. doi: 10.1111/j.1439-0310.2004.01013.x
Fischer J. W., Phillips G. E., Nichols T. A., VerCauteren K. C. (2013). Could avian scavengers translocate infectious prions to disease-free areas initiating new foci of chronic wasting disease? Prion 7, 263–266. doi: 10.4161/pri.25621
Fisher M. C., Prioreschi R. A., Wolfe L. L., Runge J. P., Griffin K. A., Swanson H. M., et al. (2022). Apparent stability masks underlying change in a mule deer herd with unmanaged chronic wasting disease. Commun. Biol. 5, 15. doi: 10.1038/s42003-021-02951-z
Flick A. J., Acevedo M. A., Elderd B. D. (2016). The negative effects of pathogen-infected prey on predators: a meta-analysis. Oikos 125, 1554–1560. doi: 10.1111/oik.03458
Fry J. D. (1996). The evolution of host specialization: are trade-offs overrated? Am. Nat. 148, S84S107. doi: 10.1086/285904
Haley N. J., Hoover E. A. (2015). Chronic wasting disease of cervids: current knowledge and future perspectives. Annu. Rev. Anim. Biosci. 3, 305325. doi: 10.1146/annurev-animal-022114-111001
Hall S. R., Duffy M. A., Cáceres C. E. (2005). Selective predation and productivity jointly drive complex behavior in host-parasite systems. Am. Nat. 165, 70–81. doi: 10.1086/426601
Horn C. J., Wasylenko J. A., Luong L. T. (2022). Scared of the dark? Phototaxis as behavioural immunity in a host–parasite system. Biol. Lett. 18, 20210531. doi: 10.1098/rsbl.2021.0531
Jennelle C. S., Samuel M. D., Nolden C. A., Keane D. P., Barr D. J., Johnson C., et al. (2009). Surveillance for transmissible spongiform encephalopathy in scavengers of white-tailed deer carcasses in the chronic wasting disease area of Wisconsin. J. Tox. Env. Health 72, 1018–1024. doi: 10.1080/15287390903084249
Johnson H. E., Golden T. S., Adams L. G., Gustine D. D., Lenart E. A., Barboza P. S.. (2021). Dynamic selection for forage quality and quantity in response to phenology and insects in an Arctic ungulate. Ecol. Evol. 11, 11664–11688. doi: 10.1002/ece3.v11.17
Khalil H., Ecke F., Evander M., Magnusson M., Hörnfeldt B. (2016). Declining ecosystem health and the dilution effect. Nat. Sci. Rep. 6, 31314. doi: 10.1038/srep31314
Krumm C. E., Connor M. M., Thompson Hobbs N., Hunter D. O., Miller M. W. (2010). Mountain lions prey selectively on prion-infected mule deer. Biol. Lett. 6, 209–211. doi: 10.1098/rsbl.2009.0742
Labadie T., Batejat C., Leclercq I., Manuguerra J. (2020). Historical discoveries on viruses in the environment and their impact on public health. Intervirol. 63, 1732. doi: 10.1159/000511575
Lafferty K. D. (1992). Foraging on prey that are modified by parasites. Am. Nat. 140, 854867. doi: 10.1086/285444
Lafferty K. D. (1999). The evolution of trophic transmission. Parasitol. Today 15, 111115. doi: 10.1016/S0169-4758(99)01397-6
Le Sage M. J., Towey B. D., Brunner J. L. (2019). Do scavengers prevent or promote disease transmission? The effect of invertebrate scavenging on Ranavirus transmission. Func. Ecol. 33, 1342–1350. doi: 10.1111/fec.2019.33.issue-7
Lozano G. A. (1991). Optimal foraging theory: a possible role for parasites. Oikos 60, 391–395. doi: 10.2307/3545084
Malmberg J. L., White L. A., VandeWoude S. (2021). Bioaccumulation of pathogen exposure in top predators. Trends Ecol. Evol. 36, 411–420. doi: 10.1016/j.tree.2021.01.008
McCarville J. L., Ayres J. S. (2018). Disease tolerance: concept and mechanism. Curr. Opin. Immunol. 50, 88–93. doi: 10.1016/j.coi.2017.12.003
Medzhitov R., Schneider D. S., Soares M. P. (2012). Disease tolerance as a defense strategy. Science 335, 936–941. doi: 10.1126/science.1214935
Meisel J. D., Kim D. H. (2014). Behavioral avoidance of pathogenic bacteria by Caenorhabditis elegans. Trends Immunol. 35, 465470. doi: 10.1016/j.it.2014.08.008
Moleón M., Martínez-Carrasco C., Muellerklein O. C., Getz W. M., Muñoz-Lozano C., Sánchez-Zapata J. A., et al. (2017). Carnivore carcasses are avoided by carnivores. J. Anim. Ecol. 86, 1179–1191. doi: 10.1111/jane.2017.86.issue-5
Moleón M., Sánchez-Zapata J. A., Sebastián-González E., Owen-Smith N. (2015). Carcass size shapes the structure and functioning of an African scavenging assemblage. Oikos 124, 1391–1403. doi: 10.1111/oik.2015.v124.i10
Muñoz-Lozano C., Martín-Vega D., Martínez-Carrasco C., Sánchez-Zapata J. A., Morales-Reyes Z., Gonzálvez M., et al. (2019). Avoidance of carnivore carcasses by vertebrate scavengers enables colonization by a diverse community of carrion insects. PLoS One 14, e0221890. doi: 10.1371/journal.pone.0221890
Mysterud A., Edmunds D. R. (2019). A review of chronic wasting disease in North America with implications for Europe. Eur. J. Wild. Res. 65, 26. doi: 10.1007/s10344-019-1260-z
Nakano Y., Yamamoto K., Ueda M. T., Soper A., Konno Y., Kimura I., et al. (2020). A role for gorilla APOBEC3G in shaping lentivirus evolution including transmission to humans. PloS Pathog. 16, e1008812. doi: 10.1371/journal.ppat.1008812
Nichols T. A., Fischer J. W., Spraker T. R., Kong Q., VerCauteren K. C. (2015). CWD prions remain infectious after passage through the digestive system of coyotes (Canis latrans). Prion 9, 367–375. doi: 10.1080/19336896.2015.1086061
Oliva-Vidal P., Tobajas J., Margalida A. (2021). Cannibalistic necrophagy in red foxes: Do the nutritional benefits offset the potential costs of disease transmission? Mamm. Biol. 101, 11151120. doi: 10.1007/s42991-021-00184-5
Olson Z. H., Beasley J. C., Rhodes O. E. Jr. (2016). Carcass type affects local scavenger guilds more than habitat connectivity. PLoS One 11, e0147798. doi: 10.1371/journal.pone.0147798
Olson Z. H., Torlone C., Russell C. M., Wood C. A., Welch J. F., Burkholder K. M., et al. (2022). Foraging risk in scavenging ecology: a study of scavenger behavior and patterns of bacterial growth. Basic App. Ecol. 61, 10–19. doi: 10.1016/j.baae.2022.03.006
Otero A., Duque Valásquez C., Aiken J., McKenzie D. (2015). Chronic wasting disease: a cervid prion infection looming to spillover. Vet. Res. 52, 115. doi: 10.1186/s13567-021-00986-y
Oum R. E., Lieberman D., Aylward A. (2011). A feel for disgust: tactile cues to pathogen presence. Cog. Emot. 25, 717725. doi: 10.1080/02699931.2010.496997
Parris M. J., Davis A., Collins J. P. (2004). Single-host pathogen effects on mortality and behavioral responses to predators in salamanders (Urodela: Ambystomatidae). Can. J. Zool. 82, 1477–1483. doi: 10.1139/z04-127
Peers M. J. L., Konkolics S. M., Lamb C. T., Majchrzak Y. N., Menzies A. K., Studd E. K., et al. (2020). Prey availability and ambient temperature influence carrion persistence in the boreal forest. J. Anim. Ecol. 89, 2156–2167. doi: 10.1111/1365-2656.13275
Peers M. J. L., Konkolics S. M., Majchrzak Y. N., Menzies A. K., Studd E. K., Boonstra R., et al. (2021). Vertebrate scavenging dynamics differ between carnivore and herbivore carcasses in the northern boreal forest. Ecosphere 12, e03691. doi: 10.1002/ecs2.v12.8
Pereria H., Detrain C. (2020). Pathogen avoidance and prey discrimination in ants. R. Soc Open Sci. 7, 191705. doi: 10.1098/rsos.191705
Pfennig D. W. (2000). Effect of predator-prey phylogenetic similarity on the fitness consequences of predation: a trade-off between nutrition and disease? Am. Nat. 155, 335–345. doi: 10.1086/303329
Remonti L., Balestrieri A., Domenis L., Banchi C., Lo Valvo T., Robetto S., et al. (2005). Red fox (Vuples vulpes) cannibalistic behaviour and the prevalence of Trichinella britovi in NW Italian Alps. Parisitol. Res. 97, 431–l435. doi: 10.1007/s00436-005-1481-9
Sarabian C., Curtis V., McMullan R. (2018). Evolution of pathogen and parasite avoidance behaviours. Phil. Trans. R. Soc B. 373, 20170256. doi: 10.1098/rstb.2017.0256
Sarabian C., Wilkinson A., Sigaud M., Kano F., Tobajas J., Darmaillacq A., et al. (2023). Disgust in animals and the application of disease avoidance to wildlife management and conservation. J. Anim. Ecol 92, 1489–1508. doi: 10.1111/1365-2656.13903
Sarabian C., MacIntosh A. J. J. (2015). Hygienic tendencies correlate with low geohelminth infection in free-ranging macaques. Biol. Lett. 11, 20150757. doi: 10.1098/rsbl.2015.0757
Scanes C. G. (2018). “Animal perception including differences with humans,” in Animals and Human Society. eds. Scanes C. G., Toukhsati S. A. (Cambridge, MA, USA: Elsevier Publishing Inc), 1–11.
Scholz C., Firozpoor J., Kramer-Schadt S., Gras P., Schulze C., Kimmig S. E., et al. (2020). Individual dietary specialization in a generalist predator: a stable isotope analysis of urban and rural red foxes. Ecol. Evol. 10, 8855–8870. doi: 10.1002/ece3.v10.16
Sinclair A. R. E., Arcese P. (1995). Population consequences of predation-sensitive foraging: the Serengeti wildebeest. Ecology 76, 882891. doi: 10.2307/1939353
Soghigian J., Valsdottir L. R., Livdahl T. P. (2017). A parasite’s modification of host behavior reduces predation on its host. Eco. Evol. 7, 1453–1461. doi: 10.1002/ece3.2017.7.issue-5
Stephens P. R., Altizer S., Ezenwa V. O., Gittleman J. L., Moan E., Han B., et al. (2019). Parasite sharing in wild ungulates and their predators: Effects of phylogeny, range overlap, and trophic links. J. Anim. Ecol. 88, 1017–1028. doi: 10.1111/jane.2019.88.issue-7
Tong W. H., Pavey C., O’Handley R., Vyas A. (2021). Behavioral biology of Toxoplasma gondii infection. Parasites Vectors 14, 77. doi: 10.1186/s13071-020-04528-x
Vicente J., VerCauteren K. (2019). “The role of scavenging in disease dynamics,” in Carrion Ecology and Management. Eds. Olea P., Mateo-Tomás P., Sánchez-Zapata J. (Springer, Cham), 161–182.
Wang Q., Shakoor N., Boyher A., Veley K. M., Berry J. C., Mockler T. C., et al. (2021). Escalation in the host-pathogen arms race: a host resistance response corresponds to a heightened bacterial virulence response. PLoS Pathog. 17, e1009175. doi: 10.1371/journal.ppat.1009175
Wenting E., Rinzema S. C. Y., van Langevelde F. (2022). Functional differences in scavenger communities and the speed of carcass decomposition. Ecol. Evol. 12, e8576. doi: 10.1002/ece3.v12.2
Wild M. A., Thompson Hobbs N., Graham M. S., Miller M. W. (2011). The role of predation in disease control: a comparison of selective and nonselective removal on prion disease dynamics. J. Wildl. Dis. 47, 7893. doi: 10.7589/0090-3558-47.1.78
Keywords: behavioral avoidance, disease, pathogen, predation, scavenging
Citation: Clapp JG, Malmberg JL and Holbrook JD (2024) Examining pathogen avoidance in predator-prey and scavenging systems. Front. Ecol. Evol. 12:1481290. doi: 10.3389/fevo.2024.1481290
Received: 15 August 2024; Accepted: 17 October 2024;
Published: 06 November 2024.
Edited by:
Antoni Margalida, Spanish National Research Council (CSIC), SpainReviewed by:
Jorge Tobajas, University of Cordoba, SpainCopyright © 2024 Clapp, Malmberg and Holbrook. This is an open-access article distributed under the terms of the Creative Commons Attribution License (CC BY). The use, distribution or reproduction in other forums is permitted, provided the original author(s) and the copyright owner(s) are credited and that the original publication in this journal is cited, in accordance with accepted academic practice. No use, distribution or reproduction is permitted which does not comply with these terms.
*Correspondence: Justin G. Clapp, justin.clapp@wyo.gov