- 1School of Business, Chongqing College of Humanities, Science and Technology, Chongqing, China
- 2Institute for Ecology and Environmental Resources, Chongqing Academy of Social Sciences, Chongqing, China
- 3School of Architecture and Urban Planning, Shenzhen University, Shenzhen, China
The Daba Mountain area is recognized as one of the global biodiversity hotspots. In recent years, human–wildlife conflicts (HWCs) in this region have intensified significantly. Understanding and mitigating the damage caused by wildlife is crucial for maintaining ecological balance and enhancing public welfare. We analyzed the current status of HWCs in the southern foothills of the Daba Mountains through semi-structured interviews and field surveys. Additionally, using the collected data on HWC occurrences, we predicted HWC hotspots using the maximum entropy (MaxEnt) model. The results: (1) A total of 449 cases of actual HWC incidents were investigated, primarily involving wild boars (Sus scrofa; 81.96%) and Asiatic black bears (Ursus thibetanus; 18.04%). The main types of HWC included crop destruction and attacks on beehives. (2) Incidents of damage caused by wild boars and Asiatic black bears were concentrated in the summer months, particularly from June to August. (3) In the study area, 21 out of 25 townships reported HWC cases. Notably, the townships of Fuxing, Gaoyan and Gaoguan were identified as the most affected, with Gaoyan and Fuxing experiencing the highest amounts of loss. (4) The variable of DTP (Distance to Protected Area) was found to have the greatest influence on the hotspot distributions of HWCs. The hotspot zone exhibited a strip-like distribution along the northwest–southeast axis in the central part of the study area. The total area of the HWC hotspots was 1352.56 km2. The largest hotspot areas were located in Xiuqi Town, Gaoyan Town, and Houping Township, accounting for 13.26%, 12.01%, and 7.83% of the total hotspot area, respectively. HWC hotspots require robust management measures to mitigate wildlife damage to local communities and enhance indigenous people’s engagement in wildlife conservation. Implementation of physical measures and compensation schemes may be necessary in these hotspots to alleviate the financial burden on indigenous populations. Our findings may provide valuable insights for the future formulation of biodiversity conservation policies and HWC mitigation strategies in the Daba Mountains.
1 Introduction
Amidst intensifying human activities and the continuous evolution of societies, the global panorama of biodiversity is marked by persistent erosion (AdDíaz et al., 2019). Within this contextual framework, the preservation of biodiversity, the pursuit of sustainable development, and the establishment of an ecological civilization have assumed paramount significance as strategic imperatives for the present-day international community (Wei et al., 2021). Therefore, recently, there has been a noticeable increase in the efficacy of conservation efforts; however, concurrently, instances of human–wildlife conflicts (HWCs) near protected areas have surged (Gupta, 2013; Dai et al., 2020). These incidents present specific challenges to the management of these areas and the execution of biodiversity conservation initiatives. In view of these unfolding dynamics, a comprehensive understanding of the dynamic interplay between human entities and wildlife, coupled with a proactive quest for modalities to ameliorate the prevalence of HWCs, stands as a pivotal linchpin in realizing a harmonious coexistence between humanity and the natural realm (Göttert and Starik, 2022; Wanghe et al., 2024). A report from the United Nations Environment Programme explicitly emphasizes the integration of HWC management into the strategies of sustainable development goals, further underscoring the importance of mitigating HWCs for biodiversity conservation (Gross et al., 2021). The convocation of the 15th Conference of the Parties to the Convention on Biological Diversity (COP15) convened in Kunming during 2021 bore a singular thematic focus on fortifying ecological civilization, augmenting biodiversity conservation endeavors, and propelling aspirations encompassing carbon emissions peaking and attaining carbon neutrality. This assemblage not only served as a crucible for infusing the international fraternity with conviction and momentum towards coherently constructing a shared destiny for the entire spectrum of life on Earth, and by extension, forging a pristine and resplendent world. Moreover, it catalyzed an escalated discourse surrounding the intricate interface between humankind and the natural environment.
The evolution of societies has established a historical continuum wherein humans and wildlife have shared existence for millennia, entailing both periods of strife and harmony (Reid et al., 2005; Jordan et al., 2020). During the nascent phases of human civilization, engagements with wildlife often manifested as reflexive reactions involving combat or evasion, prompted by the imperative to sustain a perpetual state of vigilance in daily life to elude being subsumed as prey by cohabiting wildlife (Hillman et al., 2001; Bruford et al., 2003). This paradigm persisted as human societies transitioned from a predominant hunter-gatherer mode to an agrarian and pastoral way of life, amplifying the persistence of HWCs. Owing to the detrimental impacts of wildlife on agricultural crops and livestock, as well as the potential risks posed to human safety, specific wildlife species were susceptible to lethal interventions (Treves et al., 2009). At a global level, the interplay between humans and wildlife presents a substantial conservation quandary (Wanghe et al., 2020a). The depredation of livestock, the obliteration of crops, and the menace to human property and well-being frequently instigate retaliatory culls of wildlife by local communities (Dickman, 2010; Nyhus, 2016). This intricate dynamic has fostered continual deliberations encompassing the concomitant risks and intrinsic value associated with safeguarding these species (Redpath et al., 2015). Over the past two decades, the realm of HWC research has demonstrated an exponential surge (Marchini et al., 2019). Contemporary investigations signify a pronounced augmentation in the volume of scholarly endeavors pertaining to the nuances of HWCs over the preceding decade, with documented occurrences of such conflicts spanning across 99 nations. Evidently, the continents of Asia (encompassing records from 33 counties) and Africa (embracing records from 19 counties) notably emerge as the geographical realms characterized by the highest incidence of reported conflict episodes (Torres et al., 2018).
Globally, the phenomenon of HWCs has emerged as a substantial menace imperiling the persistence of numerous species at risk of extinction. The intricacies inherent in these conflicts are underpinned by a myriad of determinants, encompassing the categorization of conflict, the specific species implicated, the extent of resultant impairment, and the localized levels of acceptance towards wildlife. Investigations underscore that within the milieu of developing counties, particularly within the rural expanses of Asia and Africa, the intricacies of coexisting with wildlife bear a heightened socioeconomic burden, one engendered by the exigencies of contending with heightened ecological competition for finite natural resources (Seoraj-Pillai and Pillay, 2016). Illustratively, the case of Africa serves to exemplify this multifaceted interaction. The foremost catalysts for conflicts manifest as indigenous carnivores, exemplified by lions (Panthera leo), African wild dog (Lycaon pictus) and cheetah (Acinonyx jubatus). These apex predators recurrently engage in livestock predation within traditional agricultural and pastoral landscapes contiguous to protected enclaves (Butler, 2000; Patterson et al., 2004). Concurrently, the herbivorous wildlife contingent proffers substantial impact through crop depredation. In the Yabello Protected Area of Ethiopia, instances are numerous where zebras cause substantial damage to agricultural produce (Fentaw and Duba, 2017). Analogously, the precincts neighboring Uganda’s forest reserves witness prominent contributions to agricultural and husbandry losses via the agency of primates (Hill, 1997). Even in India, where indigenous communities may express more lenient perspectives on wildlife courtesy of deeply ingrained cultural and religious ethos, occurrences of wildlife-induced decimation of crops and livestock endure tenaciously (Karanth et al., 2012). Previous studies have employed 3S technologies to analyze the spatial distribution patterns of HWCs. Techniques such as Hotspot Analysis and Kernel Density Estimation are commonly used to identify high-conflict areas (Bagheriyan et al., 2023). Additionally, Species Distribution Models (SDMs) are effective tools for predicting potential conflict hotspots. These models estimate the likelihood of conflict occurrence by analyzing the relationship between environmental variables and conflict events.
In China, instances of HWCs have been extensively documented (Liu et al., 2011; Alexander et al., 2015). However, due to the ecological diversity found within the various regional animal biogeographic zones, the types of conflicts experienced vary significantly across locations (Zhang and Wang, 2003; Li et al., 2013). Across different geographical regions in China, the attributes of HWCs manifest distinct regional disparities. Within the context of the Qinghai-Tibet Plateau, primary participants in conflicts encompass carnivorous animals, such as snow leopards (P. uncia), brown bears, and wolves (Dai et al., 2020; Li et al., 2013). In the southwestern and southern regions of China, HWCs primarily revolve around species like Asian elephants (Elephas maximus), Asiatic black bears (Ursus thibetanus), and wild boars (Sus scrofa) (Zhang and Wang, 2003; Xu et al., 2019). In proximity to Xishuangbanna National Nature Reserve in Yunnan Province, conflicts have arisen between local communities and Asian elephants. These disputes stem from habitat degradation, which in turn has led to incursions by Asian elephants into food crops, including wheat, rice, and bananas (Zhang and Wang, 2003). Conversely, within central and northern China, the spectrum of HWC encompasses a wide range, spanning from carnivores to primates and extending to ungulates. To exemplify, research outcomes from Shanxi Province bring to light intense conflicts between North China leopards (P. pardus japonensis) and residents, with profound negative economic implications (Consolee et al., 2020). Negative perceptions of this species, based on its perceived threat to livestock, have given rise to significant calls for its reduction or even eradication (Consolee et al., 2020). In the northeastern region, Amur tigers (P. tigris ssp. altaica) are identified as the predominant conflict species, followed by wild boars, alongside the presence of Asiatic black bears (Soh et al., 2014; Du et al., 2022; Li et al., 2022). Prolonged surveys conducted over years in Jilin Province underscore the significant crop losses attributed to the activities of wild boars (Li et al., 2022).
Nestled at the southern foothills of the Daba Mountains in the upper Yangtze River region, Chengkou County enjoys a strategically advantageous geographical placement. Its diverse terrain encompasses a variety of ecosystems, ranging from mountains and canyons to rivers and forests, thereby providing extensive habitats conducive to biodiversity. This exceptional ecological richness has earned it the distinction of being recognized as a repository of biological treasures within the Chongqing Municipality (The People’s Government of Chengkou County; http://www.cqck.gov.cn). Nevertheless, in recent times, occurrences of HWCs within Chengkou County have been on the rise (Wang et al., 2017). The failure to promptly institute effective management strategies could potentially trigger widespread and irreparable negative sentiments, thereby significantly hindering the conservation and management endeavors related to wildlife and protected areas. Of notable concern is the swift proliferation of Asiatic black bear and wild boar populations in recent years (Figure 1), leading to considerable economic losses for communities situated around protected areas. Given this context, Chengkou County faces an urgent need for the implementation of contingency plans dedicated to mitigating HWCs, with specific emphasis placed on the stringent regulation of wildlife, particularly focusing on the wild boar population (Wang et al., 2017). Consequently, a comprehensive understanding of the prevailing landscape of HWCs within Chengkou County becomes indispensable. This should be coupled with the strategic identification of priority regions for the implementation of control measures, ultimately geared towards augmenting the effectiveness and efficiency of conflict management initiatives. Such interventions extend beyond safeguarding local biodiversity; they also address wildlife-related challenges, facilitating sustainable coexistence models between human populations and wildlife species. Our study aims to understand the current status of HWCs in the Daba Mountains, identify HWC hotspots, and provide a basis for developing effective measures to mitigate HWC in the future.
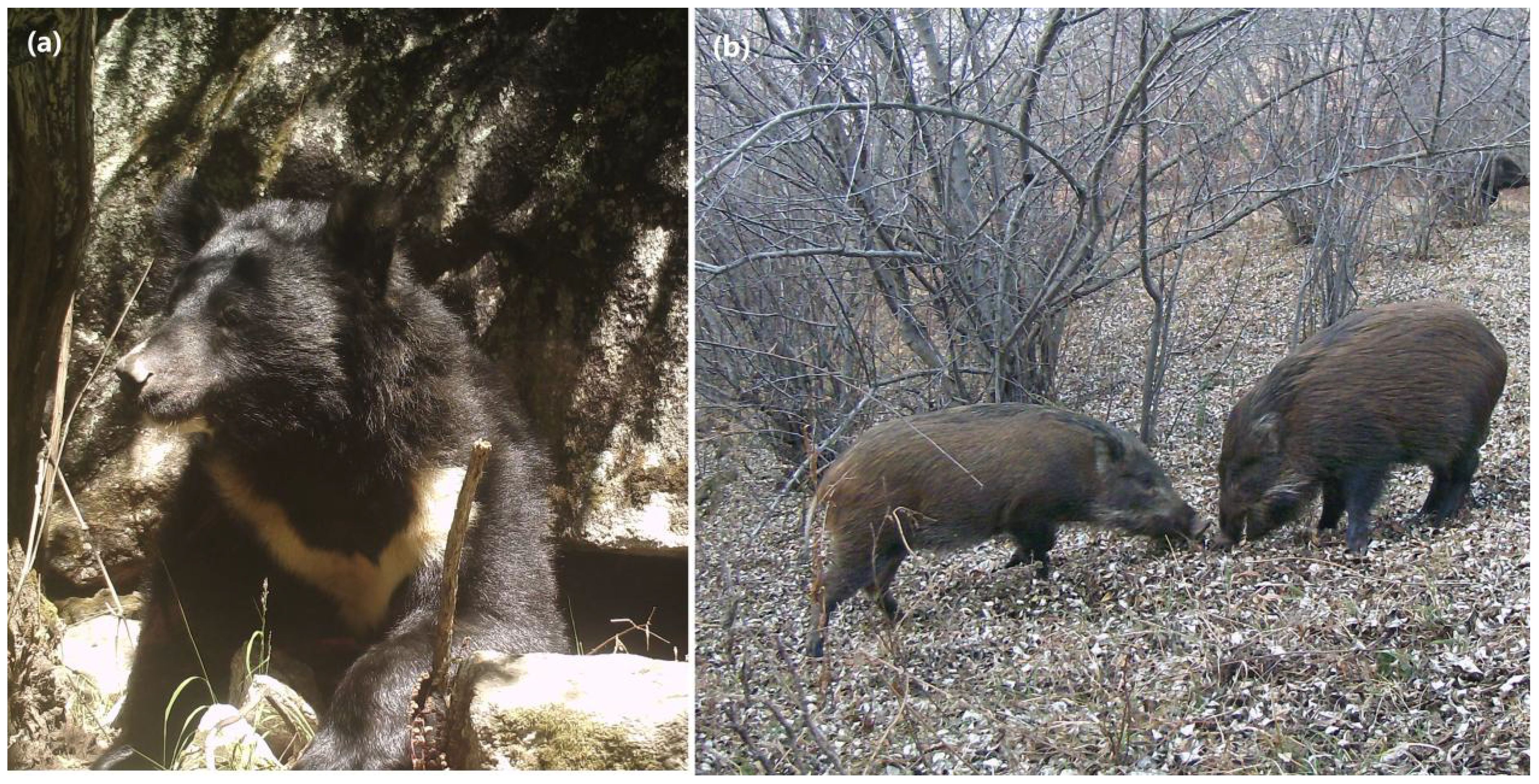
Figure 1 The Asiatic black bear (Ursus thibetanus) (A) and wild boars (Sus scrofa) (B) captured by our camera traps.
2 Materials and methods
2.1 Study area
Chengkou County is situated at the southern foothills of the Daba Mountains, within the upper reaches of the Yangtze River region and in the northeastern sector of Chongqing (Figure 2). It shares borders with the provinces of Sichuan, Chongqing, and Shaanxi, positioning itself geographically between 31°37′ to 32°12′ N, and 108°15′ to 109°16′ E. Chengkou falls within the subtropical monsoon climate zone of the northern Sichuan Basin. The biological diversity within Chengkou County is noteworthy, exemplified by the Daba Mountains National Nature Reserve located within its confines, hailed by biologists as a repository of invaluable biological resources and genetic reservoir. The area is home to a rich tapestry of wildlife, encompassing 706 species, inclusive of six first-tier protected species like clouded leopard (Neofelis nebulosa), golden eagle (Aquila chrysaetos), and forest musk deer (Moschus berezovskii), as well as 35 second-tier protected species, including Asiatic golden cat (Pardofelis temminckii), large Indian civet (Viverra zibetha), and Asiatic black bear (The People’s Government of Chengkou County; http://www.cqck.gov.cn).
2.2 Data collection and processing
2.2.1 Semi-structured interviews and wildlife damage compensation record
Our study conducted on-site investigations in areas of Chengkou County significantly impacted by HWCs (Appendix 1). The selection of areas for household interviews was primarily based on the wildlife damage compensation records provided by the Chengkou County Forestry Bureau (from October 1, 2021 to September 30, 2022). During the interviews, we focused on investigating the species causing damage, representative damage type, occurrence times, compensation amounts, and occurrence of wildlife damage. A total of 186 households were successfully surveyed (Figure 2). Since the household interviews did not cover all townships, we analyzed the economic losses caused by wildlife based on the data from the wildlife damage compensation records. We summarized and analyzed conflict incidents from each investigated region using Excel 2019, categorizing them by region, the wildlife species involved, and the respective months. Additionally, utilizing ArcGIS 10.6 (ESRI Inc., Redlands, CA, USA), we performed spatial vectorization and reclassification of the damage cases to enhance the clarity of the spatial distribution of identified wildlife cases.
2.2.2 Collection of HWC occurrences
Field surveys were conducted in Chengkou County to collect data on wildlife damage occurrences from 2020 to 2023. The coordinates of these HWC occurrences were recorded using GPS. The types of damage primarily included crop destruction, livestock predation, and damage to beehives. A total of 185 GPS coordinates corresponding to wildlife damage were collected. Among these, 118 GPS coordinates corresponded to crop damage, 65 GPS coordinates to beehive damage, and the remaining 2 GPS coordinates to livestock predation incidents. To mitigate spatial autocorrelation, we followed model optimization recommendations and previous research methods by randomly selecting one point from each 0.5 km2 grid. Consequently, 106 valid points were obtained for modeling analysis.
2.2.3 Environmental variables
We selected environmental variables based on geographic environmental characteristics, human disturbance, wildlife ecology, and those used in previously published literature (Miller, 2015; Li et al., 2018). Environmental variables were categorized into three groups (Table 1). All spatial variables were resampled to 30 m resolution, and unified projection coordinate system (WGS_1984) in ArcGIS 10.6 (ESRI Inc., Redlands, CA, USA).
2.3 HWC hotspots modeling
To identify HWC hotspots, we employed the MaxEnt model, configuring its parameters with a random test percentage of 25% and a regularization multiplier of 1. Fifteen replicates were executed alongside cross-validation. The significance of variables was assessed using percent contribution. Utilizing the average logistic threshold value of Maximum Training Sensitivity Plus Specificity (MTSPS) output by MaxEnt, we generated a hotspot map (Dai et al., 2019). Cells surpassing the MTSPS threshold were identified as HWC hotspots. Model performance was evaluated through the area under the receiver operating characteristic curve (AUC). AUC serves as an independent threshold to gauge model accuracy, with values ranging from 0 to 1. Higher AUC values indicate greater model accuracy, with 1 representing perfect accuracy (Phillips et al., 2006).
3 Results
3.1 Property losses caused HWC
From October 1, 2021 to September 30, 2022, a total of 449 successfully appraised HWC cases were collected, with a total loss amounting to 160851 CNY (Table 2). Notably, incidents involving wild boars were predominant, constituting 368 cases (81.96%), resulting in an overall economic loss of 97717 CNY. Incidents attributed to Asiatic black bears numbered 81 (18.04%), contributing to a total loss of 63134 CNY. Within this context, wild boars emerged as the primary culprits for substantial agricultural damage, notably affecting crops such as corn and potatoes. Concurrently, Asiatic black bears exhibited a preference for targeting beehives for destruction.
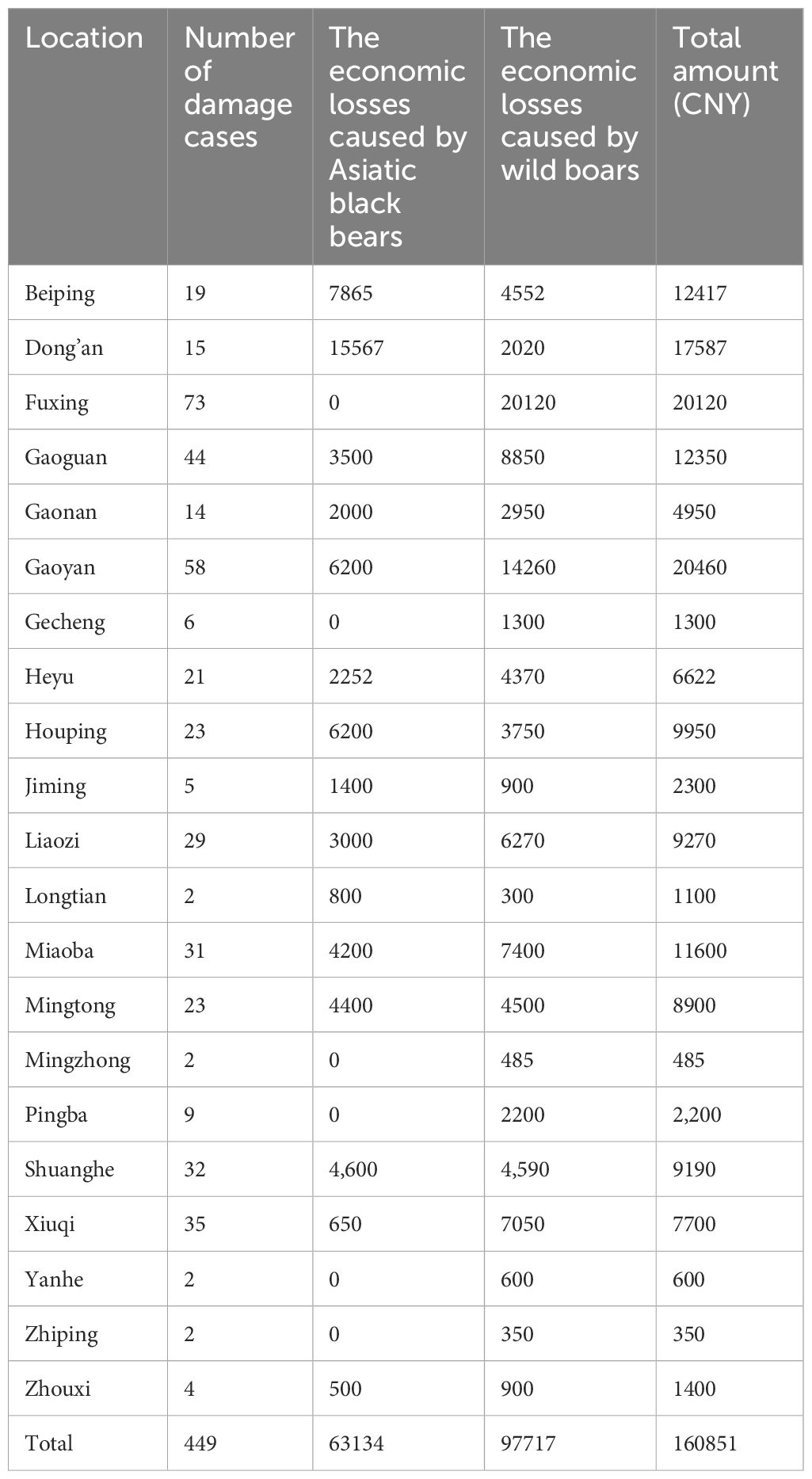
Table 2 The varying economic losses incurred due to different wild animal interactions within distinct locations.
3.2 Seasonal variations in HWC
For incidents attributed to Asiatic black bears, the highest frequency was observed in August, totaling 37 cases. July closely followed with 32 incidents, while June recorded only 2 incidents, indicating relatively lower numbers. In the case of incidents caused by wild boars, August and July presented the highest incidence rates, accounting for 159 and 138 cases respectively. Additionally, September witnessed a substantial number of incidents, totaling 66. June followed with a lower count of 4 incidents. Across the entire dataset, the occurrences of wild boar-induced incidents notably surpassed those attributed to Asiatic black bears. It is noteworthy that both wild boars and Asiatic black bears exhibited heightened activity levels during the summer months, specifically in June, July, and August (Figure 3).
3.3 Spatial distribution of HWC
In the study area, 21 out of 25 townships reported HWC cases. Among these, incidents involving both wild boars and Asiatic black bears occurred in 15 townships. The top five townships in terms of reported and successfully assessed cases were Fuxing, Gaoyan, Gaoguan, Xiuqi, and Shuanghe, with 73, 58, 44, 35, and 32 cases respectively (Figure 4). In terms of monetary losses, the leading townships were Gaoyan, Fuxing, Dong’an, Beiping, and Gaoguan, with respective compensations of 20460, 20120, 17587, 12417, and 12350 CNY (Table 2).
3.4 Prediction of HWC hotspots
3.4.1 Model performance
The percent contribution of model variables ranked from highest to lowest were distance to protected area (54.8%), DEM (14.9), human influence index (14.6%), distance to rivers (9.3%), aspect (2.5%), Normalized Difference Vegetation Index (1.7%), slope (1.2%), land use type (0.6%), and human population density (0.3%). Distance to protected area was found to have the greatest influence on the hotspot distributions of HWCs. The cross validation value illustrated sufficient performance for model outputs (average testing AUC was 0.735 ± 0.073; Figure 5).
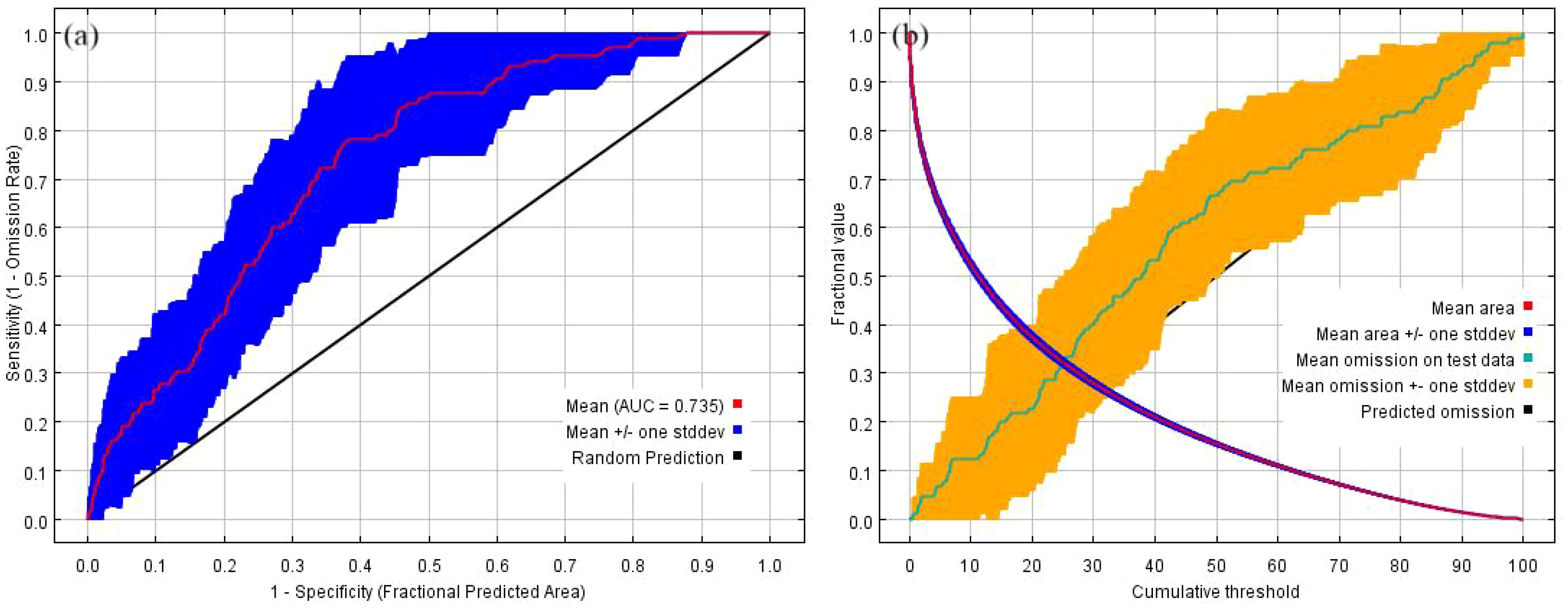
Figure 5 Statistical graphs of MaxEnt model output results. (A) Receiver operating characteristic (ROC) curve and average test AUC for accuracy analysis of hotspot prediction. (B) Analysis of test omission rate and predicted area, where values indicate the training gain only with variables.
3.4.2 Distributions of the HWC hotspots
The possible distribution probabilities of HWCs were shown in Figure 6 (MTSPS=0.3954). The total area of the HWC hotspots was 1352.56 km2. Spatially, the hotspot zone exhibited a strip-like distribution along the northwest–southeast axis in the central part of the study area. The largest hotspot areas were located in Xiuqi Town, Gaoyan Town, and Houping Township, with distribution areas of 179.30 km², 162.38 km², and 105.85 km², respectively, accounting for 13.26%, 12.01%, and 7.83% of the total hotspot area (Figure 6; Table 3).
4 Discussion
In recent years, the global consciousness regarding wildlife conservation has witnessed a significant surge, prompting nations worldwide to redouble their efforts in safeguarding wild fauna (Dai et al., 2019; Corlett, 2020). This impetus has led to the implementation of a series of substantial conservation measures across numerous countries (Manfredo et al., 2020; Wanghe et al., 2020b, Wanghe et al., 2022). Initiatives encompassing natural forest preservation, reforestation of former agricultural land, and afforestation in restricted zones have been pursued with the overarching objective of enhancing wildlife habitats. These endeavors have yielded noteworthy outcomes, progressively contributing to the recuperation and proliferation of wildlife populations, while also broadening their territorial expanse (Tuanmu et al., 2016; Akçakaya et al., 2018). Notably, the populations of species such as wild boars, tigers, and black bears have demonstrated annual increments, reflective of the amelioration in their natural habitats within the wild. Wildlife instinctively extends the boundaries of their territories in pursuit of adequate sustenance and habitation. However, this positive trend is accompanied by a suite of adverse repercussions, among which is a pronounced escalation in conflicts between wildlife and human activities and production (Nyhus, 2016). As wildlife populations rebound, the demarcations between their habitats and human settlements become increasingly indistinct (Gurung et al., 2008). Consequently, wildlife increasingly encroach upon human residential areas in quest of sustenance or shelter, culminating in a surge of direct interactions between wildlife and humans. This proximity poses a threat not solely to human assets and agricultural yields, but also harbors the potential to instigate a succession of HWCs, thereby presenting a latent hazard to human well-being. Hence, the escalating prevalence of wildlife-induced harm has emerged as a matter of profound concern.
The variations in HWC across different spatial regions are influenced by a multifaceted interplay of factors, encompassing the geographical environment, natural conservation policies, geographic features, and human activity patterns (Nyhus, 2016; Dai et al., 2020). These combined elements collectively shape the dynamic interaction between wildlife and human populations in each area, consequently exerting a direct impact on the frequency of damage incidents (Nyhus, 2016). In the central zone of the study area, particularly in locales like Fuxing and Gaoyan, there is a conspicuously higher incidence of reported wildlife-induced damage cases. However, it is imperative to approach this phenomenon with circumspection, as it may not unequivocally signify a heightened prevalence of wildlife-induced damage in this area compared to others. This observation may be attributed to the fact that the administrative hub of Chengkou County is situated in the central part of the study area, enjoying accessible transportation and a relatively concentrated population. This ease of accessibility and population density could facilitate local farmers in reporting cases of wildlife-induced damage and conducting assessments of losses, consequently leading to a greater number of reported cases (Dai et al., 2020). Conversely, remote areas, exemplified by regions like Yanhe and Zhouxi in the western part of the study area, tend to host more abundant wildlife populations. However, due to their considerable distance from the county seat and less convenient transportation, conducting assessments for insurance claims assessors may pose relative challenges. This factor may contribute to a lower inclination among local farmers to report cases, resulting in a reduced incidence of wildlife-induced damage cases in these remote areas. Therefore, when considering the broader context, one of the primary factors contributing to the relatively limited number of reported cases in remote areas may be attributed to the geographical remoteness of these regions. This factor hinders the ease with which insurance claims assessors can conduct assessments and, concurrently, diminishes the proactive reporting tendencies of local farmers.
Seasonal variations exert a profound influence on the life strategies and behavioral patterns of numerous wildlife species. Spring, functioning as the reproductive season for many animals, signifies the period during which they procreate (Metz et al., 2011). During this phase, animals display heightened activity levels, actively foraging for food and constructing nests to furnish abundant sustenance and secure habitats for the next generation (Crupi, 2004). However, this heightened activity may also lead to a rise in interactions with human activities, consequently elevating the incidence of harm incidents (Dai et al., 2019). In contrast, autumn prompts certain animals to intensify their activities, driven by the need to amass winter food resources (Li et al., 2013). In this interval, they may adopt more proactive behaviors to ensure sufficient reserves for enduring the cold season. This heightened activity may culminate in increased contact with human activities, thereby amplifying the frequency of harm incidents (Dai et al., 2020). The seasonal fluctuation in resource availability constitutes a pivotal factor influencing animal behavior. Particularly in winter, food resources in the wild become comparatively scarce, compelling some wildlife to increasingly rely on resources within human-inhabited areas (Dai et al., 2020). This renders human residential areas pivotal locales for their food acquisition, consequently heightening interactions with humans and resulting in a surge of harm incidents during the cold season. In our study area, we noted that incidents involving wild boars and Asiatic black bears are predominantly concentrated in the summer. This phenomenon can be ascribed to the bountiful harvest of summer crops in the local vicinity, encompassing corn and honey, which furnish a plentiful source of sustenance for wildlife. Additionally, it is noteworthy that the hibernation behavior of Asiatic black bears also influences their activity patterns, rendering summer their period of heightened activity.
In predicting hotspots of HWCs, we found that the variable “distance to protected area” contributed the most significantly in the model. The proximity of protected areas to target regions may have a substantial impact on the population density and diversity of wildlife in those areas. When areas are closer to protected areas, wildlife is generally more susceptible to influences, as they may traverse these regions in search of food, habitat, or migration routes. Additionally, the management policies and measures implemented in protected areas may also influence the behavior and migration patterns of wildlife in surrounding areas. For instance, wildlife within protected areas may receive stricter protection, leading them to migrate to surrounding areas in search of food or habitat, thereby increasing the likelihood of HWCs. Consequently, it is recommended to enhance awareness of wildlife conservation and strategies for mitigating HWCs in communities closer to protected areas, and to implement additional physical measures in these areas to prevent occurrences of HWCs.
Preventive measures and protective actions for wild boars and Asiatic black bears should be meticulously designed and executed in a scientifically rigorous and all-encompassing manner to mitigate conflicts with human activities and uphold ecological equilibrium. First, the establishment of a robust monitoring system is imperative (Yin et al., 2021). Leveraging cutting-edge technologies like infrared cameras and satellite tracking, real-time monitoring and documentation of the movements and migration routes of targeted species, such as wild boars and Asiatic black bears, should be undertaken to acquire a foundation of scientifically precise data. Subsequently, a heightened focus on habitat protection and restoration is warranted (Distefano, 2005). Specifically for wild boars, paramount importance should be accorded to the preservation of their critical natural habitats, encompassing wetlands and forests, to ensure an abundant supply of sustenance and appropriate living environments. As for Asiatic black bears, special consideration should be given to conserving their wintering and breeding grounds, thus safeguarding their distinctive life history requisites. Furthermore, the development of scientifically-grounded habitat management strategies is essential (Dai et al., 2019). Through prudent land-use planning and the demarcation of zones for wildlife conservation and human activities, the convergence and clashes between the two realms can be curtailed, thereby furnishing secure habitats for both wild boars and black bears. In terms of farmland protection, physical measures are the most effective, such as electric fences. It is particularly necessary to use physical measures to enhance the protection of high-yield crops inside HWC hotspots. In addition, an ecological compensation mechanism ought to be instituted. This mechanism can establish a rational system for compensating groups, such as farmers, impacted by wildlife intrusions. It serves to incentivize the adoption of corresponding protective measures while concurrently promoting community engagement in endeavors for environmental preservation.
In response to the current scenario characterized by low insurance payouts and a passive approach to reporting incidents among farmers, the following strategies are proposed: Firstly, a thorough assessment and fine-tuning of the existing insurance framework are imperative. Collaborative efforts with insurance companies should be undertaken to evaluate the scope of coverage and payout thresholds of current insurance schemes. This evaluation aims to ensure their alignment with the actual needs of stakeholders. Subsequent adjustments can then be implemented to augment the level of coverage, thereby guaranteeing farmers receive adequate financial protection in the event of wildlife-induced incidents. Secondly, the exploration of spatial differentiated premium policies is advised. Tailoring premium standards based on the HWC hotspots and historical data across different regions is a viable approach. For areas characterized by higher risk factors, moderate increases in premiums can be implemented. This strategic move seeks to incentivize insurance companies to offer higher payouts, consequently fostering a greater inclination among farmers to invest in insurance coverage. Additionally, the introduction of government subsidy policies to mitigate the financial burden on farmers in procuring insurance warrants consideration. The provision of a specified percentage of insurance premium subsidies or exemptions by the government can serve as a powerful catalyst. This measure effectively encourages farmers to engage in insurance, subsequently elevating their awareness of the scope and benefits of insurance coverage. Simultaneously, there is a pressing need to bolster communication and education between insurance entities and farmers. Regularly organizing seminars or training sessions focused on disseminating essential insurance knowledge is pivotal. This initiative serves to underscore the significance of insurance and elucidate the pertinent claims procedures to farmers. Ultimately, it fortifies farmers’ comprehension and trust in insurance, consequently amplifying their propensity to report incidents. Lastly, it is strongly recommended to establish a robust insurance claims mechanism, streamlining the claims process to heighten efficiency. Ensuring that farmers promptly receive insurance payouts following wildlife-induced incidents substantially enhances the tangible efficacy of insurance coverage. This, in turn, bolsters farmers’ confidence and proactive engagement in acquiring insurance.
The issue of HWC in the Daba Mountains presents a complex environmental challenge influenced by multiple interacting factors. Key challenges include the limited availability of long-term monitoring data, which constrains our understanding of the historical trends in HWC dynamics across the region. Additionally, precise identification of specific locations where HWC incidents occur remains incomplete, thereby limiting the accuracy of predicting hotspots of conflict risk. Our study acknowledges several limitations inherent in our modeling approach. Notably, our models did not comprehensively incorporate variables such as climate dynamics, animal behavior, and human activities, all of which can influence HWC dynamics. For instance, climate change impacts on phenomena like black bear hibernation and plant phenology indirectly affect bear food sources, potentially altering their spatial distribution and interactions with humans. While we integrated climate factors into our study design, obtaining high-precision current and future climate data at the local scale within the study area remained challenging. Furthermore, the scarcity of primary research data on HWC in the Daba Mountains necessitated reliance on survey data and simulated modeling approaches based on available data accessibility and utility. Despite these limitations, our scientific simulations provide valuable insights into the current status of HWC and identify critical hotspot areas of HWC. This foundational analysis is crucial for informing evidence-based strategies aimed at mitigating HWC in the Daba Mountains, offering a pathway for future research and conservation efforts in similar contexts.
5 Conclusions
Our study provided an in-depth analysis of HWCs in the southern foothills of the Daba Mountains, highlighting the significant impact of wild boars and Asiatic black bears on local agriculture, particularly during the summer months. The findings underscored the urgent need for targeted mitigation strategies, as evidenced by 449 documented cases primarily involving crop destruction and beehive attacks. The identification of Fuxing, Gaoyan, and Gaoguan townships as hotspots, with substantial economic losses, indicates a need for prioritized intervention. Using the MaxEnt model, we found that proximity to protected areas is a critical factor influencing HWC hotspots, which exhibit a strip-like distribution along the northwest–southeast axis in the central part of the study area. To address these conflicts, we recommend enhancing community awareness, improving land-use planning, and developing compensation schemes. This study not only enhances our understanding of HWC dynamics in the Daba Mountain area but also provides a foundation for developing effective mitigation strategies, with future research focusing on long-term monitoring and the effectiveness of these measures to ensure sustainable coexistence between humans and wildlife.
Data availability statement
The original contributions presented in the study are included in the article/Supplementary Material, further inquiries can be directed to the corresponding author/s.
Author contributions
QW: Conceptualization, Funding acquisition, Writing – original draft, Writing – review & editing. YD: Data curation, Methodology, Writing – original draft, Writing – review & editing. QS: Conceptualization, Funding acquisition, Writing – original draft, Writing – review & editing.
Funding
The author(s) declare financial support was received for the research, authorship, and/or publication of this article. This research was funded by the Humanities and Social Sciences Research Project of Chongqing Municipal Education Commission (Grant/Award Number: 24SKGH349), the Humanities and Social Sciences Research Project of Chongqing Municipal Education Commission (Grant/Award Number: 22SKGH492), the Guangdong Provincial Philosophy and Social Science Planning Project (Grant/Award Number: GD22YYS09), and the Guangdong Basic and Applied Basic Research Foundation (Grant/Award Number: 2023A1515110856).
Acknowledgments
We would like to express our gratitude to the Chengkou County Forestry Bureau for their invaluable assistance with the ground surveys.
Conflict of interest
The authors declare that the research was conducted in the absence of any commercial or financial relationships that could be construed as a potential conflict of interest.
Publisher’s note
All claims expressed in this article are solely those of the authors and do not necessarily represent those of their affiliated organizations, or those of the publisher, the editors and the reviewers. Any product that may be evaluated in this article, or claim that may be made by its manufacturer, is not guaranteed or endorsed by the publisher.
Supplementary material
The Supplementary Material for this article can be found online at: https://www.frontiersin.org/articles/10.3389/fevo.2024.1435811/full#supplementary-material
References
AdDíaz S., Settele J., Brondízio E. S., Ngo H. T., Agard J., Arneth A., et al. (2019). Pervasive human-driven decline of life on Earth points to the need for transformative change. Science 366, eaax3100. doi: 10.1126/science.aax31
Akçakaya H. R., Bennett E. L., Brooks T. M., Grace M. K., Heath A., Hedges S., et al. (2018). Quantifying species recovery and conservation success to develop an IUCN Green List of Species. Conserv. Biol. 32, 1128–1138. doi: 10.1111/cobi.13112
Alexander J., Chen P., Damerell P., Youkui W., Hughes J., Shi K., et al. (2015). Human wildlife conflict involving large carnivores in Qilianshan, China and the minimal paw-print of snow leopards. Biol. Conserv. 187, 1–9. doi: 10.1016/j.biocon.2015.04.002
Bagheriyan E., Karimi A., Yazdandad H. (2023). Assessing spatio-temporal patterns of human-wildlife conflicts in a human-dominated landscape: a case study from Iran. Biodiversity Conserv. 32, 4239–4257. doi: 10.1007/s10531-023-02685-w
Bruford M. W., Bradley D. G., Luikart G. (2003). DNA markers reveal the complexity of livestock domestication. Nat. Rev. Genet. 4, 900–910. doi: 10.1038/nrg1203
Butler J. R. (2000). The economic costs of wildlife predation on livestock in Gokwe communal land, Zimbabwe. Afr. J. Ecol. 38, 23–30. doi: 10.1046/j.1365-2028.2000.00209.x
Consolee K. T., Gao C., Vitekere K., Li C., Yan H., Jiang G. (2020). Human-leopard conflict: An emerging issue of North China leopard conservation in Tieqiaoshan provincial nature reserve in Shanxi Province, China. Animals 10, 996. doi: 10.3390/ani10060996
Corlett R. T. (2020). Safeguarding our future by protecting biodiversity. Plant Diversity 42, 221–228. doi: 10.1016/j.pld.2020.04.002
Crupi A. P. Jr (2004). Foraging behavior and habitat use patterns of brown bears (Ursus arctos) in relation to human activity and salmon abundance on a coastal Alaskan salmon stream (Logan, Utah, U.S.: Utah State University).
Dai Y., Hacker C. E., Zhang Y., Li W., Li J., Zhang Y., et al. (2019). Identifying the risk regions of house break-ins caused by Tibetan brown bears (Ursus arctos pruinosus) in the Sanjiangyuan region, China. Ecol. Evol. 9, 13979–13990. doi: 10.1002/ece3.5835
Dai Y., Xue Y., Hacker C. E., Zhang Y., Zhang Y., Liu F., et al. (2020). Human-carnivore conflicts and mitigation options in Qinghai province, China. J. Nat. Conserv. 53, 125776. doi: 10.1016/j.jnc.2019.125776
Dickman A. J. (2010). Complexities of conflict: the importance of considering social factors for effectively resolving human–wildlife conflict. Anim. Conserv. 13, 458–466. doi: 10.1111/j.1469-1795.2010.00368.x
Distefano E. (2005). Human-Wildlife Conflict worldwide: collection of case studies, analysis of management strategies and good practices (Rome, Italy: Food and Agricultural Organization of the United Nations (FAO), Sustainable Agriculture and Rural Development Initiative (SARDI). Available at: http://www.fao.org/documents.
Du H., Xiaoliang Z., Minghai Z., Xiangdong R., Lee T. M. (2022). Spatial distribution and conservation strategies of large carnivores in human-dominated landscape: A case study of asiatic black bear in Jilin, China. Front. Ecol. Evol. 10, 882282. doi: 10.3389/fevo.2022.882282
Fentaw T., Duba J. (2017). Human–wildlife conflict among the pastoral communities of southern rangelands of Ethiopia: the case of Yabello protected area. J. Int. Wildlife Law Policy 20, 198–206. doi: 10.1080/13880292.2017.1346352
Göttert T., Starik N. (2022). Human–Wildlife Conflicts across Landscapes—General Applicability vs. Case Specificity. Diversity 14, 380. doi: 10.3390/d14050380
Gross E., Jayasinghe N., Brooks A., Polet G., Wadhwa R., Hilderink-Koopmans F. (2021). A future for all: the need for human-wildlife coexistence (Gland, Switzerland: WWF). Design and infographics by Levent Köseoglu, WWF-Netherlands Text editing by ProofreadNOW.com Cover photograph: DNPWC-WWF Nepal, 3.
Gupta A. C. (2013). Elephants, safety nets and agrarian culture: understanding human-wildlife conflict and rural livelihoods around Chobe National Park, Botswana. J. Political Ecol. 20, 238–254. doi: 10.2458/v20i1.21766
Gurung B., Smith J. L. D., McDougal C., Karki J. B., Barlow A. (2008). Factors associated with human-killing tigers in Chitwan National Park, Nepal. Biol. Conserv. 141, 3069–3078. doi: 10.1016/j.biocon.2008.09.013
Hill C. M. (1997). Crop-raiding by wild vertebrates: the farmer’s perspective in an agricultural community in western Uganda. Int. J. Pest Manage. 43, 77–84. doi: 10.1080/096708797229022
Hillman G., Hedges R., Moore A., Colledge S., Pettitt P. (2001). New evidence of Lateglacial cereal cultivation at Abu Hureyra on the Euphrates. Holocene 11, 383–393. doi: 10.1191/095968301678302823
Jordan N. R., Smith B. P., Appleby R. G., van Eeden L. M., Webster H. S. (2020). Addressing inequality and intolerance in human–wildlife coexistence. Conserv. Biol. 34, 803–810. doi: 10.1111/cobi.13471
Karanth K. K., Gopalaswamy A. M., DeFries R., Ballal N. (2012). Assessing patterns of human-wildlife conflicts and compensation around a central Indian protected area. PloS One 7, e50433. doi: 10.1371/journal.pone.0050433
Li W., Liu P., Guo X., Wang L., Wang Q., Yu Y., et al. (2018). Human-elephant conflict in Xishuangbanna Prefecture, China: Distribution, diffusion, and mitigation. Global Ecol. Conserv. 16, e00462. doi: 10.1016/j.gecco.2018.e00462
Li Y., Powell J., Jin A., Ryoo H. K., Li H., Pandey P., et al. (2022). Community attitudes towards Amur tigers (Panthera tigris altaica) and their prey species in Yanbian, Jilin province, a region of northeast China where tigers are returning. PloS One 17, e0276554. doi: 10.1371/journal.pone.0276554
Li J., Yin H., Wang D., Jiagong Z., Lu Z. (2013). Human-snow leopard conflicts in the Sanjiangyuan Region of the Tibetan Plateau. Biol. Conserv. 166, 118–123. doi: 10.1016/j.biocon.2013.06.024
Liu F., McShea W. J., Garshelis D. L., Zhu X., Wang D., Shao L. (2011). Human-wildlife conflicts influence attitudes but not necessarily behaviors: Factors driving the poaching of bears in China. Biol. Conserv. 144, 538–547. doi: 10.1016/j.biocon.2010.10.009
Manfredo M. J., Teel T. L., Don Carlos A. W., Sullivan L., Bright A. D., Dietsch A. M., et al. (2020). The changing sociocultural context of wildlife conservation. Conserv. Biol. 34, 1549–1559. doi: 10.1111/cobi.13493
Marchini S., Ferraz K. M. P. M. B., Zimmermann A., Guimarães-Luiz T., Morato R., Correa P. L., et al. (2019). “Planning for coexistence in a complex human-dominated world,” in Human–wildlife interactions: Turning conflict into coexistence, 414–438.
Metz M. C., Vucetich J. A., Smith D. W., Stahler D. R., Peterson R. O. (2011). Effect of sociality and season on gray wolf (Canis lupus) foraging behavior: implications for estimating summer kill rate. PloS One 6, e17332. doi: 10.1371/journal.pone.0017332
Miller J. R. B. (2015). Mapping attack hotspots to mitigate human–carnivore conflict: approaches and applications of spatial predation risk modeling. Biodiversity Conserv. 24, 2887–2911. doi: 10.1007/s10531-015-0993-6
Nyhus P. J. (2016). Human–wildlife conflict and coexistence. Annu. Rev. Environ. Resour. 41, 143–171. doi: 10.1146/annurev-environ-110615-085634
Patterson B. D., Kasiki S. M., Selempo E., Kays R. W. (2004). Livestock predation by lions (Panthera leo) and other carnivores on ranches neighboring Tsavo National Parks, Kenya. Biol. Conserv. 119, 507–516. doi: 10.1016/j.biocon.2004.01.013
Phillips S. J., Anderson R. P., Schapire R. E. (2006). Maximum entropy modeling of species geographic distributions. Ecol. Model. 190, 231–259. doi: 10.1016/j.ecolmodel.2005.03.026
Redpath S. M., Gutiérrez R. J., Wood K. A., Young J. C. (Eds.) (2015). Conflicts in conservation: navigating towards solutions (Cambridge, U.K.: Cambridge University Press). doi: 10.1017/CBO9781139084574
Reid W. V., Mooney H. A., Cropper A., Capistrano D., Carpenter S. R., Chopra K., et al. (2005). Ecosystems and human well-being-Synthesis: A report of the Millennium Ecosystem Assessment (Washington D.C., U.S.: Island Press).
Seoraj-Pillai N., Pillay N. (2016). A meta-analysis of human–wildlife conflict: South African and global perspectives. Sustainability 9, 34. doi: 10.3390/su9010034
Soh Y. H., Carrasco L. R., Miquelle D. G., Jiang J., Yang J., Stokes E. J., et al. (2014). Spatial correlates of livestock depredation by Amur tigers in Hunchun, China: relevance of prey density and implications for protected area management. Biol. Conserv. 169, 117–127. doi: 10.1016/j.biocon.2013.10.011
Torres D. F., Oliveira E. S., Alves R. R. (2018). Conflicts between humans and terrestrial vertebrates: a global review. Trop. Conserv. Sci. 11, 1940082918794084. doi: 10.1177/1940082918794084
Treves A., Wallace R. B., White S. (2009). Participatory planning of interventions to mitigate human–wildlife conflicts. Conserv. Biol. 23, 1577–1587. doi: 10.1111/j.1523-1739.2009.01242.x
Tuanmu M. N., Vina A., Yang W., Chen X., Shortridge A. M., Liu J. (2016). Effects of payments for ecosystem services on wildlife habitat recovery. Conserv. Biol. 30, 827–835. doi: 10.1111/cobi.12669
Wang Z., Zhang H., Wu X. (2017). Investigation on the harm of wild boar in Mingtong Town, Chengkou, Chongqing. Agric. Sci. Technol. 18, 2446–2449.
Wanghe K., Ahmad S., Zhou X., Tian F., Liu S., Zhou B., et al. (2024). Spatially explicit estimation of freshwater fish stock biomass with limited data: A case study of an endangered endemic fish on the Tibetan Plateau, China. Sci. Total Environ. 912, 168717. doi: 10.1016/j.scitotenv.2023.168717
Wanghe K., Guo X., Ahmad S., Tian F., Nabi G., Strelnikov I. I., et al. (2022). FRESF model: An ArcGIS toolbox for rapid assessment of the supply, demand, and flow of flood regulation ecosystem services. Ecol. Indic. 143, 109264. doi: 10.1016/j.ecolind.2022.109264
Wanghe K., Guo X., Hu F., Ahmad S., Jin X., Khan T. U., et al. (2020a). Spatial coincidence between mining activities and protected areas of giant panda habitat: The geographic overlaps and implications for conservation. Biol. Conserv. 247, 108600. doi: 10.1016/j.biocon.2020.108600
Wanghe K., Guo X., Wang M., Zhuang H., Ahmad S., Khan T. U., et al. (2020b). Gravity model toolbox: An automated and open-source ArcGIS tool to build and prioritize ecological corridors in urban landscapes. Global Ecol. Conserv. 22, e01012. doi: 10.1016/j.gecco.2020.e01012
Wei F., Cui S., Liu N., Chang J., Ping X., Ma T., et al. (2021). Ecological civilization: China’s effort to build a shared future for all life on earth. Natl. Sci. Rev. 8, nwaa279. doi: 10.1093/nsr/nwaa279
Xu J., Wei J., Liu W. (2019). Escalating human–wildlife conflict in the Wolong Nature Reserve, China: A dynamic and paradoxical process. Ecol. Evol. 9, 7273–7283. doi: 10.1002/ece3.5299
Yin D., Yuan Z., Li J., Zhu H. (2021). Mitigate human-wildlife conflict in China. Science 373, 500–501. doi: 10.1126/science.abj8766
Keywords: human–wildlife conflicts, HWC hotspots, crops protection, mitigation strategies, maxent
Citation: Wu Q, Dai Y and Sun Q (2024) Human–wildlife conflict patterns and hotspot prediction in the southern foothills of the Daba Mountains, China. Front. Ecol. Evol. 12:1435811. doi: 10.3389/fevo.2024.1435811
Received: 21 May 2024; Accepted: 01 July 2024;
Published: 18 July 2024.
Edited by:
Kunyuan Wanghe, Chinese Academy of Sciences (CAS), ChinaReviewed by:
Shahid Ahmad, Hainan University, ChinaFeng Jiang, Chinese Academy of Sciences (CAS), China
Diqiang Li, Chinese Academy of Forestry, China
Copyright © 2024 Wu, Dai and Sun. This is an open-access article distributed under the terms of the Creative Commons Attribution License (CC BY). The use, distribution or reproduction in other forums is permitted, provided the original author(s) and the copyright owner(s) are credited and that the original publication in this journal is cited, in accordance with accepted academic practice. No use, distribution or reproduction is permitted which does not comply with these terms.
*Correspondence: Qiaoyun Sun, cWlhb3l1bkBzenUuZWR1LmNu