- Biological Sciences Department, Wayne State University, Detroit, MI, United States
Among insects, Orthoptera (crickets and grasshoppers) have evolved a set of highly specialized structures for acoustic communication, with males engaged in sound production and amplification (stridulation) and females in sound reception. These structures in males are located on their forewings, suggesting that some of the wing genes may have been co-opted for new roles in stridulation. As previous studies have identified nubbin (nub) as one of the key genes in the wing development network, we examined its function in the house cricket, Acheta domesticus. Our results show that nub regulates the overall size and venation of both fore- and hindwings in both sexes, consistent with its general role in wing development. Moreover, in males this gene is also involved in the development of sound resonators (primarily the harp, anal area, mirror, and chord). The distinct morphology of these structures is generated by either the activation (in the harp and anal area) or suppression (in the mirror and chord) of vein and cross-connection development in localized regions of the forewings. Finally, nub RNAi males do not stridulate, confirming that the observed changes in the morphology of resonators are functionally significant and responsible for the loss of sound production.
Introduction
Among insects, Orthoptera (crickets, grasshoppers, and katydids) are characterized by two distinguishing features: their greatly enlarged hind legs (adapted for jumping) and modified forewings (adapted for sound production). The former trait is controlled by the homeotic gene Ultrabithorax (Ubx), which was shown to regulate the allometric leg growth in both orthopterans and hemipterans (Mahfooz et al., 2004, 2007; Khila et al., 2014). These studies also suggest that the transcriptional modification of Ubx expression may be a universal mechanism for the evolutionary diversification of insect hind legs. In contrast, very little is known regarding the genetic mechanisms that control the development of specific morphological structures on orthopteran forewings, which in turn, are critical for enabling sound production.
As a part of studies regarding the diversification of insect appendages, we have previously examined the embryonic functions of POU homeodomain gene nubbin (nub) in several insect species, including crickets (Hrycaj et al., 2008; Turchyn et al., 2011). The generated results show that nub can have a variety of roles during embryogenesis, including morphogenesis of mouthparts, joint formation in legs, and the establishment of a limbless abdomen. In adults, however, this gene is recognized for its function in growth and proximal–distal patterning of wings (Ng et al., 1995; Cifuentes and Garcia-Bellido, 1997; Neumann and Cohen, 1998). These observations led us to investigate the roles of nub in the development of wings in Acheta domesticus (house cricket). Our results show that its depletion (via RNAi) results in smaller wings, with each pair displaying an altered vein pattern. Moreover, there is a specific effect in male forewings that lost and/or modified their unique structures involved in sound production.
The above results suggest a dual role of nub in cricket wing development. First, there is a general function in regulating the size and overall shape of both fore- and hindwings. This is further corroborated by recent functional studies in a variety of insects, including Onthophagus taurus (scarab beetle), Blatella germanica (cockroach), and Oncopeltus fasciatus (milkweed bug), which all show that knockdowns of nub cause a reduction in adult wing size (Medved et al., 2015; Hu and Moczek, 2021; Fernandez-Nicolas et al., 2022). Second, nub has a lineage-specific role in developing the singing apparatus in male crickets. While preliminary in nature, this study provides a first step toward identifying a genetic network underlying the evolution of sound production in orthopterans.
Materials and methods
Both Acheta nymphs and adults were raised at room temperature of 25°C and relative humidity of about 60% on a diet of fresh lettuce supplemented with dry cat food and water. Under these conditions, they underwent eight nymphal stages to become adults in about three months.
RNA isolation, cDNA synthesis, and cloning were conducted using methods previously outlined in established protocols (Li and Popadic, 2004; Hrycaj et al., 2008). The 387-bp long PCR fragment of Acheta nub, which includes the highly conserved 5’ POU homeodomain, a hypervariable linker region, and the 3’ homeodomain was isolated with the help of degenerated primers: EQFAKT (5´-GGAATTCGARCARTT YGCIA-ARAC-3´) and KEKRINP (5´-GCTCTAGAGGRTTIATICKYTTYCYTT-3´). It was then subsequently inserted into a pCR4-TOPO vector and confirmed through sequencing. This fragment was utilized not only to induce specific nub RNAi phenotypes in embryos (Turchyn et al., 2011), but also in our current analysis.
To investigate the functional importance of nub during postembryonic development, four to six μl of a 2.5 μg/μl nub double-stranded RNA (dsRNA) were injected into Acheta dorsal midline between the first and second abdominal (A1 and A2) segments using a Hamilton syringe with a pulled glass capillary needle. More specifically, multiple rounds of nub RNAi treatments were administered at different juvenile stages, starting from the 4th nymphal stage (n=51) up to the 5th (n=7) and 6th (n=53). Each injection was followed by an additional dose of nub dsRNA every ten days until these nymphs emerged as adults or died. Among the total of 111 injected nymphs, 37 died, 12 displayed wild-type characteristics, and 62 exhibited specific nub RNAi phenotypes ranging in severity from moderate to strong. As described previously (Hrycaj et al., 2010), positive control was performed by using universal 18S (QuantumRNA™ 18S Internal Standards, Ambion, Austin, TX, USA) primers with competimer (3:7 ratio) in both wild type and nub-depleted Acheta. The PCR conditions were as follows: denaturation at 95°C for 2 minutes, followed by annealing at 58.3°C and extension at 72°C for 30 seconds, repeated for 33 cycles.
Results
nub RNAi affects the overall wing size and vein pattern
Orthopteran forewings (tegmina) are oval-shaped leathery coverings that protect hindwings and are divided into two regions, the anterior (remigium) and the posterior (vannus). In Acheta, these areas are separated by a long narrow boundary that displays dense brown pigmentation (Figure 1A). The female remigium has a thick net-like venation pattern (Figure 1A1), which is greatly modified for sound production in males (Figure 1B1). To improve their visibility, male-specific stridulating and sound-resonating structures are artificially colored in violet (anal area), purple (harp), brown (mirror), green (chord), and orange (plectrum). The vannus region is similar in both sexes, featuring thick parallel veins (with fewer cross-veins between) and a distinct lobe on its proximal end.
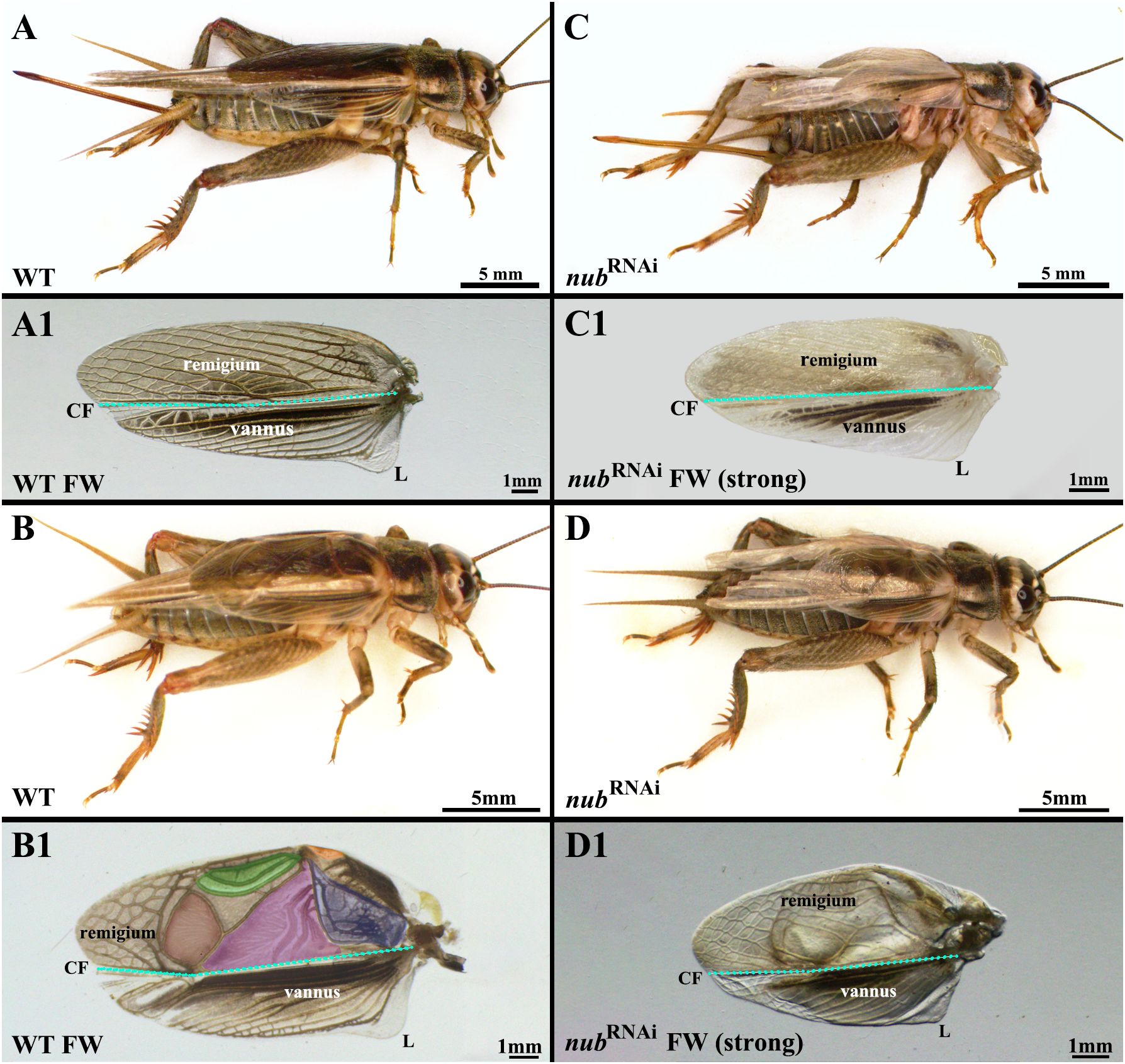
Figure 1 Analysis of nub RNAi forewing phenotypes in Acheta domesticus. (A, B) Whole body images of wild type female and male crickets. Both sexes have a pair of overlapping rigid forewings, which neatly cover their delicate hindwings that extend far beyond the abdomen. (A1) Forewings are divided by the claval fold (CF; blue dotted line) into two distinct regions, the anterior (remigium) and the posterior (vannus), respectively. The former exhibits a sclerotized, net-like venation with major veins giving off smaller branch veins (cross-veins). In the latter, however, veins run in a parallel-like fashion from the wing base towards its posterior margin and have fewer cross-veins (compared to remigium). The proximal posterior end of the forewing has a lobe (L) in both genders. (B1) In males, the morphology of the remigium is greatly altered for a new function in acoustic communication. It features specialized structures for sound production (stridulatory organs), which are artificially colored for easier visualization: anal area (violet), harp (purple), mirror (brown), chord (green), and plectrum (orange). (C, D) In nub RNAi female and male adults, forewings are reduced in size and do not fold as neatly as in wild types. (C1, D1) In both sexes, veins in forewings are less pronounced and only slightly melanized. The main change is observed in males (D1), which display large changes in the morphology of the sound-producing structures.
As illustrated in Figures 1C–D1, the forewings in nub RNAi adults of both sexes are smaller and shorter compared to the wild type. While the female remigium and vannus (Figure 1C1) largely retain the original vein pattern, the veins are much less pronounced, and they do not undergo melanization. Consequently, the entire wing appears lighter in color. The proximal lobe is also smaller and assumes an elongated shape. By far the largest effect is observed in the remigium of RNAi males, which display a significant alteration in its sound-resonating structures. First, the harp has been reduced, and three S-shaped thick veins have been replaced by several longitudinal thinner veins. Second, the previously large, veinless oval-shaped mirror region has been transformed into a much smaller rectangular shape. Furthermore, its anterior half now features several longitudinal veins that are connected with cross-veins. Third, the lattice-like vein pattern localized at the distal wing region in the wild type has expanded into the chord region. While the original chordal veins are still present, they are much thinner and connected with cross-veins.
Acheta hindwings are longer than forewings, and their distal ends extend over the abdomen in the wild type (Figures 1A, B). They feature a greatly expanded vannus region and a dense array of melanized veins that radiate from the wing base and form a fan-like pattern (Figures 2A, C). These veins are linked by cross-veins, which are most numerous in a narrow brown region just below the claval fold. Unlike the forewings, the hindwing morphology is very similar between the males and females. In nub RNAi adults, though, hindwings are smaller and barely reach the tip of the abdomen (Figures 1C, D). Furthermore, the number of veins in the vannus is significantly reduced, creating larger cells in the space between the two veins (Figures 2B, D). nub RNAi hindwings also appear lighter as these veins are not melanized, which is similar to observations in forewings.
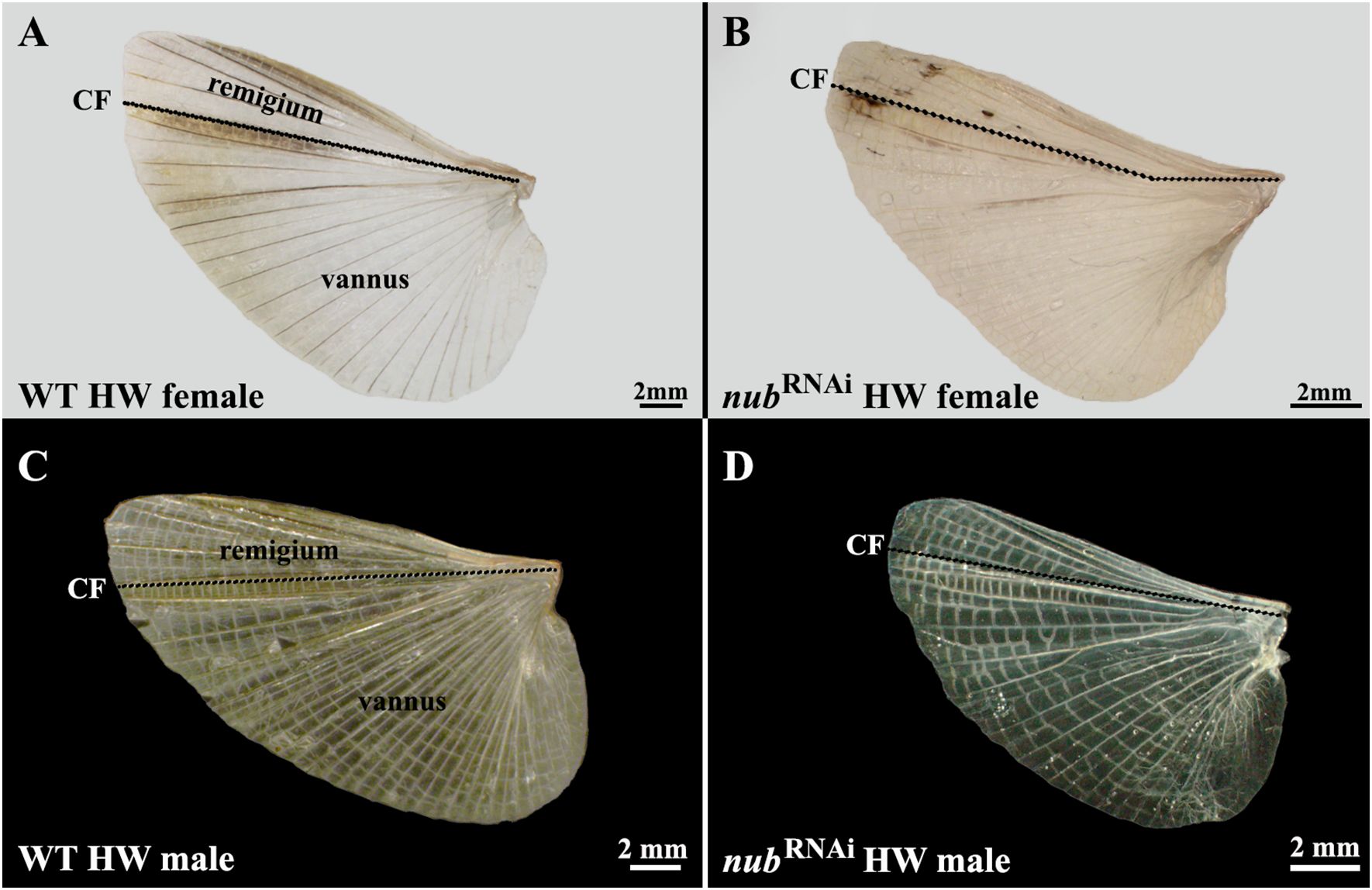
Figure 2 Comparison of hindwings in wild type and nub RNAi Acheta adults. (A, C) Dissected wild type hindwings in females and males, respectively. In contrast to oval, leathery forewings, the hindwings are fan-shaped, membranous, and have no lobe at their proximal posterior end. Their vannus is bigger than the remigium, and both regions feature longitudinal veins with numerous cross-veins. (B, D) Dissected nub RNAi hindwings in both sexes. These wings are visibly smaller and have a reduced number of longitudinal veins in their vannal region (especially in males). A black dotted line represents the CF that separates the remigium from the vannus.
nub regulates the development of sound-resonating structures in crickets
To better understand the observed morphological changes in male forewings (Figure 1D1), we examined all six regions that play important roles in sound production. As illustrated in Figure 3, our analysis includes both the moderate and strong nub RNAi phenotypes. In the proximal region, we focused on the anal area (Figure 3A1) that includes two L-shaped thick veins: A1 (black arrowhead) and Cu2 (white arrowhead). The Cu2 vein plays a critical role in sound production as it contains a row of sharp protrusions on its ventral side that create a “file-like” morphology. A stridulating sound is generated when the file strikes the plectrum (scraper) of the opposite wing. In nub knockdowns, the A1 vein disappears while Cu2 becomes much thinner and loses its original L-shape (Figures 3B1–C1). In contrast, no changes were observed in the morphology of the plectrum (Figures 3A5, B5, C5).
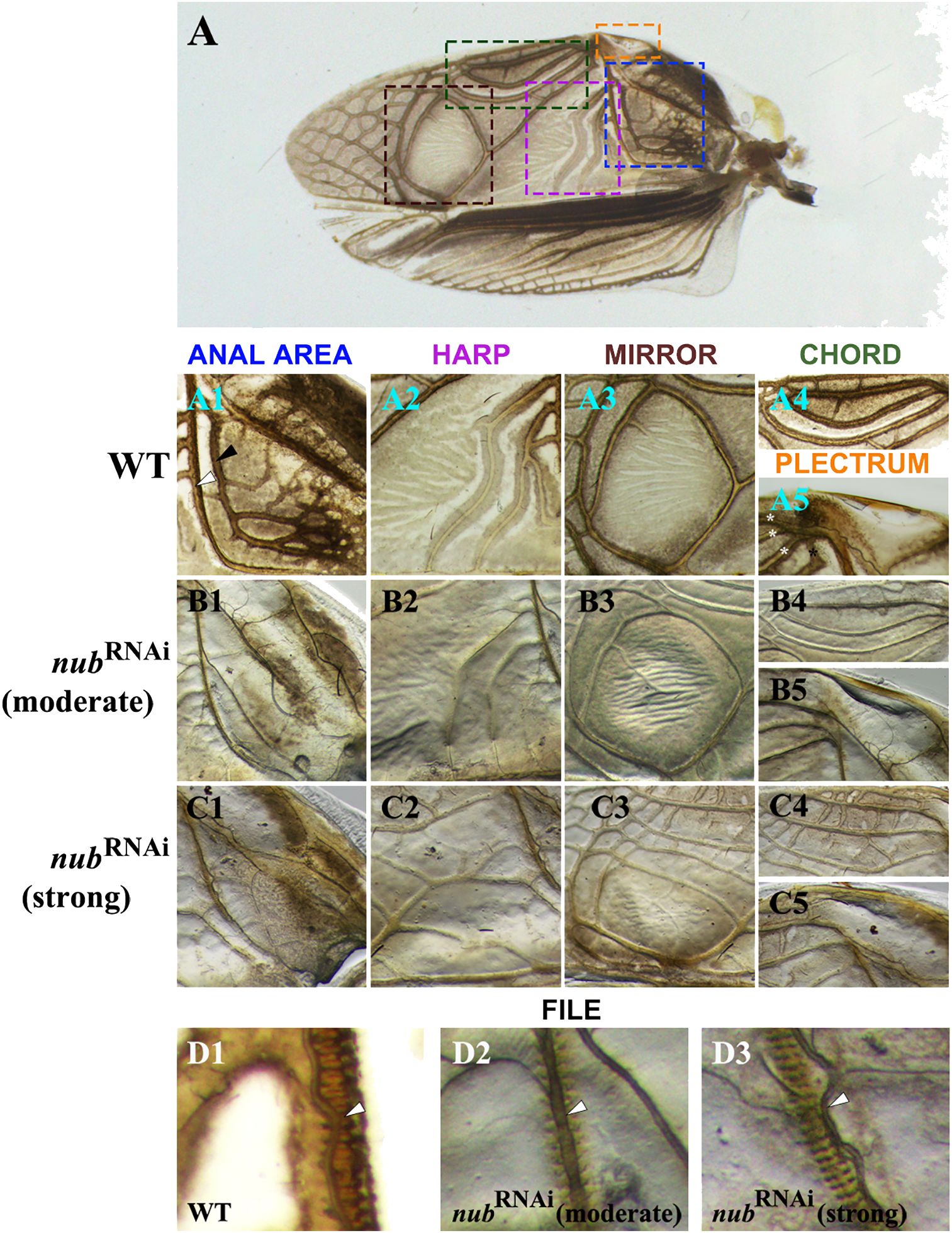
Figure 3 The details of the altered morphology in male forewings. (A) Wild-type adult forewing with dashed line squares indicating the structures associated with sound production, which are displayed in rows below. (A1-A5) Magnified images of the anal area, harp, mirror, chord, and plectrum of a wild type, respectively. Black and white arrowheads in the (A1) panel point to the A1 and Cu2 veins, respectively. The latter forms sharp protrusions on the ventral side, producing a file-like structure. Posterior to the Cu2 vein is a triangular area called harp (A2), which is followed by the mirror (A3). Along the outer edge of the wing lies an oval-shaped area known as the chord with three longitudinal veins (A4) and a hardened ridge (A5) known as the plectrum (scraper). (B1-B5, C1-C5) Close-up views of the corresponding structures in moderate and strong nub RNAi phenotypes, respectively. (D1-D3) The magnified images of the file in wild type and nub-depleted adults. White arrowhead points to the Cu2 vein.
Next, we examined changes in the three sound resonators (harp, mirror, and chord). As previously described (Desutter-Grandcolas, 1995), the harp region features several thick, contoured annulate veins with a ridge-like appearance. These veins are still present in a moderate phenotype, albeit thinner and barely contoured (Figure 3B2). In a strong phenotype, however, the original S-shaped veins disappear entirely and are replaced with several irregularly shaped longitudinal veins (Figure 3C2). Similar trend is observed in the mirror region. In the wild type, the mirror is formed by a thick, circular vein that delineates an oval-shaped flat region (Figure 3A3). In a moderate phenotype, this circular vein becomes thinner and less contoured (Figure 3B3). In addition, several smaller veins now appear in the mirror. In the strong phenotype, a network of thick longitudinal veins and short cross-veins appears in the previously veinless inside area of the mirror (Figure 3C3). Finally, we examined the last resonating structure, the chord. In wild type, the chord is an oval-shaped region that encompasses three longitudinal veins (Figure 3A4), which become much thinner in RNAi adults (Figure 3B4). Furthermore, several distinct cross-connections develop between the longitudinal veins in strong nub RNAi phenotypes (Figure 3C4). In addition, we noticed changes in the proximal region of the chord, which is labeled as anal node (Supplementary Figure 1A). This is the area where the three longitudinal veins merge with the Cu1 vein. In the moderate phenotype, this vein does not reach the anal node (asterisk in Supplementary Figure 1A1). Even more dramatic change is observed in the strong nub RNAi phenotype, in which the Cu1 acquires a completely different morphology bifurcating and merging with the Cu2 vein well below the anal node (Supplementary Figure 1B1).
The singing apparatus in male crickets is composed of a sound production part (file and plectrum) and a sound amplification part (encompassing the harp, mirror, and chord). While the above results highlight the role of nub in the development of sound resonators, they also show that the morphology of the plectrum is not affected in RNAi phenotypes. However, the closer inspection of the file (Figures 3D1–D3) revealed visible changes in its anatomical features. In wild type, there are sharp protrusions (“teeth”) on the ventral side of the Cu2 vein, which provide an overall ladder-like appearance. In nub RNAi phenotypes these protrusions are less pronounced, and the Cu2 vein is greatly reduced (Figures 3D2–D3). What is worth noting is that while the shape of the vein has been altered, the position of the file remains unchanged (Figure 3D3). In other words, the file is now positioned adjacent to but not exactly beneath the Cu2 vein.
While performing the above described experiments, we noticed that nub RNAi males are “silent” and do not stridulate. Aside from this feature, these insects were indistinguishable from the wild type. This observation shows that the changes in the morphology of stridulating and sound-resonating structures are functional and result in silencing of the males.
Discussion
The classical research in Drosophila established that nub plays a key role in the proximal–distal patterning of wings (Ng et al., 1995; Cifuentes and Garcia-Bellido, 1997; Neumann and Cohen, 1998; Zirin and Mann, 2007). The complete knockdown results in a vestigial tissue with a small bump-like appearance, highlighting its essential function during wing primordia development. However, studies in coleopterans (beetles) and hemipterans (milkweed bugs) show that nub depletion via RNAi at later nymphal stages (after the primordia are formed) cause a moderate reduction in wing size (Medved et al., 2015; Hu and Moczek, 2021). These observations reveal that nub is continuously required during wing development. Early on, it is essential for formation of wing primordia, while its later expression is necessary for wings to reach their full size. As illustrated in Figures 1 and 2, wings in Acheta nub RNAi adults are visibly smaller compared to wild type, consistent with the latter function. In addition, the patterning and melanization of veins are also affected (especially in hindwings). Aside from these general roles in both pairs of wings in both sexes, nub has a distinct function in Acheta males only (Figures 3, 4A), where it regulates the development of sound amplification structures on forewings (mainly the harp, anal area, mirror, and chord). More specifically, in the harp, nub controls the formation of the three large S-shaped annulated veins as well as the overall size of this structure (Figure 3C2). Similar function is observed in the adjacent region (anal area), where nub promotes the development of two L-shaped thick veins: A1 and Cu2 (Figure 3C1). In the mirror, though, nub suppresses vein formation, resulting in a vein-free region (Figure 3C3). Similarly, in the chord, it prevents the growth of cross-connections between the three longitudinal veins (Figure 3C4). Therefore, nub can either activate (harp and anal area) or suppress (mirror and chord) vein and cross-connection development in the distinct regions of the forewings. In addition, it is involved in regulating the size and shape of these regions.
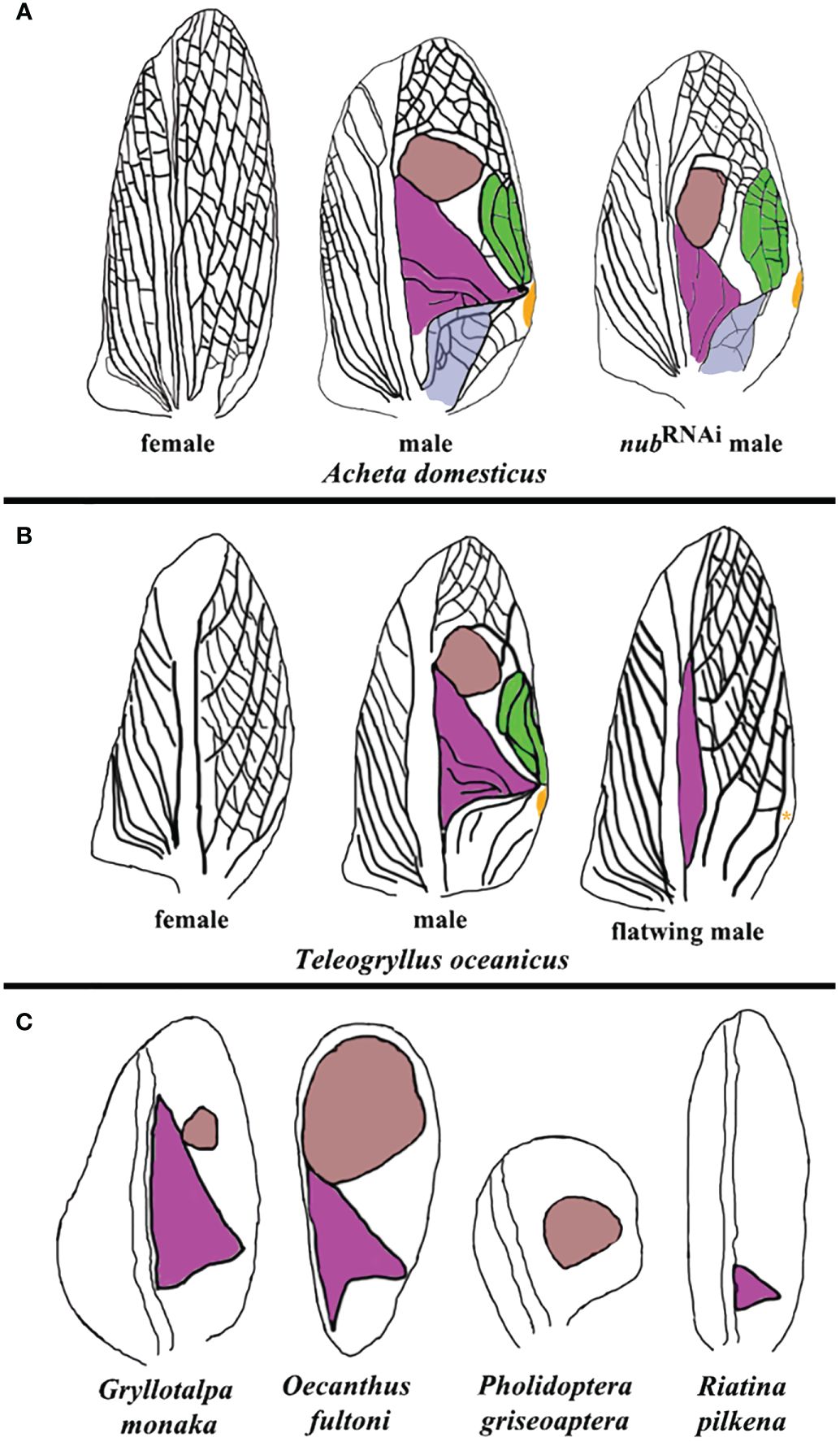
Figure 4 Summary of morphological variations in sound-producing structures across different cricket species. (A) Forewings from wild-type female, wild-type male, and nub RNAi male Acheta adults. The anal area, harp, mirror, chord, and plectrum are highlighted in violet, purple, brown, green, and orange, respectively. (B) “flatwing” males of Teleogryllus oceanicus have feminized wings, lacking the mirror, chord, and plectrum, and possessing a reduced harp. An asterisk indicates that plectrum may be present in some flatwing males. (C) A sample of naturally occurring variations in the morphology and presence of the harp and mirror in additional cricket species. Oecanthus fultoni (snowy tree cricket) features a large mirror and a smaller harp, while Gryllotalpa monanka (dark night mole cricket) has a small mirror and a large harp. In Pholidoptera griseoaptera (bush cricket), only a mirror is present, whereas another bush cricket species, Riatina pilkena, has only a harp. The forewing diagrams in (B, C) are based on studies by Pascoal et al. (2014); Pascoal et al. (2016), and Bailey et al. (2019).
The above described alterations in the morphology of resonators are functionally significant, as Acheta nub RNAi males do not stridulate. Intriguingly, twenty years ago a similar silencing event was observed in natural populations of the Hawaiian field cricket Teleogryllus oceanicus (Zuk et al., 2006). In that instance, a mutant wing morph (flatwing) evolved spontaneously to render males silent as a protection against predatory parasitoid (Figure 4B). Extensive follow up research in Teleogryllus provided a wealth of knowledge regarding the evolution of “flatwing” males, including the identification of the gene doublesex (dsx) as potentially responsible for male silencing (Desutter-Grandcolas, 1997; Montealegre et al., 2011; Gu et al., 2012; Pascoal et al., 2014; Bailey et al., 2019; Zhang et al., 2021). As illustrated in Figure 4B, though, the path towards male cricket silencing in Teleogryllus is quite different from the present observations in Acheta. First, the mirror and the chord do not develop in flatwing males. Second, the plectrum (scraper) is absent in some populations (Kauai), but present in others (Oahu). Third, the forewings in these individuals display a trend toward feminization by which they acquire the overall vein patterning very similar to the one present in female crickets. Overall, the insights from Teleogryllus and Acheta are complementary, and provide a better understanding of the level of integration between different components involved in sound production. In Teleogryllus, it is the large perturbations of the system (the absence of the mirror and chord, and the change of the wing identity) that cause the loss of the function. On the other hand, changes in Acheta are more nuanced and encompass changes in the size and morphology of the resonators.
In nature, crickets display a surprisingly large variation in the design of their sound production apparatus, some of which is depicted in Figure 4C. For example, the size of the mirror can range from small (as in Gryllotalpa monaka) to large (as in Oecanthus fultoni). There are species that do not have a mirror at all (such as Riatina pilkena), while in others a harp is absent (as in Pholidoptera griseoaptera). These observations suggest that the formation of sound resonators is regulated by distinct gene regulatory networks (GRNs) that can be activated either independently or in a coordinated fashion. Studies in Teleogryllus point to dsx as the obvious candidate as the common regulator of such GRNs. Similarly, results in Acheta suggest that nub may be co-opted for a role in the development of distinct resonator morphologies. For both genes, it is necessary to extend functional studies to other species in order to determine whether their roles are general or lineage-specific. Moreover, as resonators are spatially precisely defined, their respective GRNs should have matching spatially localized expression patterns. For example, in scarab beetles, dsx is preferentially expressed in the horn imaginal disks in large males causing the subsequent differential enlargement of these appendages in adults (Kijimoto et al., 2012). Combining the functional analyses with the expression pattern studies of genes such as dsx and nub and identifying their downstream targets, may provide critical new insights into the genetic architecture of sound-producing structures in insects.
Data availability statement
The datasets presented in this study can be found in online repositories. The names of the repository/repositories and accession number(s) can be found below: https://www.ncbi.nlm.nih.gov/genbank/, HQ543076.
Ethics statement
The manuscript presents research on animals that do not require ethical approval for their study.
Author contributions
NT: Writing – review & editing, Formal analysis, Investigation, Methodology, Validation, Visualization. AP: Writing – review & editing, Conceptualization, Funding acquisition, Resources, Supervision, Writing – original draft.
Funding
The author(s) declare financial support was received for the research and authorship of this article. This work was supported by NIH grant GM071927 to AP and a Wayne State University Graduate Enhancement Research Fellowship to NT.
Conflict of interest
The authors declare that the research was conducted in the absence of any commercial or financial relationships that could be construed as a potential conflict of interest.
Publisher’s note
All claims expressed in this article are solely those of the authors and do not necessarily represent those of their affiliated organizations, or those of the publisher, the editors and the reviewers. Any product that may be evaluated in this article, or claim that may be made by its manufacturer, is not guaranteed or endorsed by the publisher.
Supplementary material
The Supplementary Material for this article can be found online at: https://www.frontiersin.org/articles/10.3389/fevo.2024.1411228/full#supplementary-material
References
Bailey N. W., Pascoal S., Montealegre Z. F. (2019). Testing the role of trait reversal in evolutionary diversification using song loss in wild crickets. Proc. Natl. Acad. Sci. U.S.A. 116, 8941–8949. doi: 10.1073/pnas.1818998116
Cifuentes F. J., Garcia-Bellido A. (1997). Proximo-distal specification in the wing disc of Drosophila by the nubbin gene. Proc. Natl. Acad. Sci. U.S.A. 94, 11405–11410. doi: 10.1073/pnas.94.21.11405
Desutter-Grandcolas L. (1995). Functional forewing morphology and stridulation in crickets (Orthoptera, Grylloidea). J. Zool. Lond. 236, 243–252. doi: 10.1111/j.1469-7998.1995.tb04491.x
Desutter-Grandcolas L. (1997). A phylogenetic analysis of the evolution of the stridulatory apparatus in true crickets (Orthoptera, grylloidea). Cladistics 13, 101–108. doi: 10.1111/j.1096-0031.1997.tb00244.x
Fernandez-Nicolas A., Ventos-Alfonso A., Kamsoi O., Clark-Hachtel C., Tomoyasu Y., Belles X. (2022). Broad complex and wing development in cockroaches. Insect Biochem. Mol. Biol. 147, 103798. doi: 10.1016/j.ibmb.2022.103798
Gu J. J., Montealegre Z. F., Robert D., Engel M. S., Qiao G. X., Ren D. (2012). Wing stridulation in a Jurassic katydid (Insecta, Orthoptera) produced low-pitched musical calls to attract females. Proc. Natl. Acad. Sci. U.S.A. 109, 3868–3873. doi: 10.1073/pnas.1118372109
Hrycaj S., Chesebro J., Popadic A. (2010). Functional analysis of Scr during embryonic and post-embryonic development in the cockroach, Periplaneta americana. Dev. Biol. 341, 324–334. doi: 10.1016/j.ydbio.2010.02.018
Hrycaj S., Mihajlovic M., Mahfooz N., Couso J. P., Popadic A. (2008). RNAi analysis of nubbin embryonic functions in a hemimetabolous insect, Oncopeltus fasciatus. Evol. Dev. 10, 705–716. doi: 10.1111/j.1525-142X.2008.00284.x
Hu Y., Moczek A. P. (2021). Wing serial homologues and the diversification of insect outgrowths: insights from the pupae of scarab beetles. Proc. Biol. Sci. 288, 20202828. doi: 10.1098/rspb.2020.2828
Khila A., Abouheif E., Rowe L. (2014). Comparative functional analyses of ultrabithorax reveal multiple steps and paths to diversification of legs in the adaptive radiation of semi-aquatic insects. Evolution 68, 2159–2170. doi: 10.1111/evo.12444
Kijimoto T., Moczek A. P., Andrews J. (2012). Diversification of doublesex function underlies morph-, sex-, and species-specific development of beetle horns. Proc. Natl. Acad. Sci. U.S.A. 109, 20526–20531. doi: 10.1073/pnas.1118589109
Li H., Popadic A. (2004). Analysis of nubbin expression patterns in insects. Evol. Dev. 6, 310–324. doi: 10.1111/j.1525-142X.2004.04039.x
Mahfooz N., Turchyn N., Mihajlovic M., Hrycaj S., Popadic A. (2007). Ubx regulates differential enlargement and diversification of insect hind legs. PloS One 2, e866. doi: 10.1371/journal.pone.0000866
Mahfooz N. S., Li H., Popadic A. (2004). Differential expression patterns of the hox gene are associated with differential growth of insect hind legs. Proc. Natl. Acad. Sci. U.S.A. 101, 4877–4882. doi: 10.1073/pnas.0401216101
Medved V., Marden J. H., Fescemyer H. W., Der J. P., Liu J., Mahfooz N., et al. (2015). Origin and diversification of wings: Insights from a neopteran insect. Proc. Natl. Acad. Sci. U.S.A. 112, 15946–15951. doi: 10.1073/pnas.1509517112
Montealegre Z. F., Jonsson T., Robert D. (2011). Sound radiation and wing mechanics in stridulating field crickets (Orthoptera: Gryllidae). J. Exp. Biol. 214, 2105–2117. doi: 10.1242/jeb.056283
Neumann C. J., Cohen S. M. (1998). Boundary formation in Drosophila wing: Notch activity attenuated by the POU protein Nubbin. Science 281, 409–413. doi: 10.1126/science.281.5375.409
Ng M., Diaz-Benjumea F. J., Cohen S. M. (1995). Nubbin encodes a POU-domain protein required for proximal-distal patterning in the Drosophila wing. Development 121, 589–599. doi: 10.1242/dev.121.2.589
Pascoal S., Cezard T., Eik-Nes A., Gharbi K., Majewska J., Payne E., et al. (2014). Rapid convergent evolution in wild crickets. Curr. Biol. 24, 1369–1374. doi: 10.1016/j.cub.2014.04.053
Pascoal S., Liu X., Ly T., Fang Y., Rockliffe N., Paterson S., et al. (2016). Rapid evolution and gene expression: a rapidly evolving Mendelian trait that silences field crickets has widespread effects on mRNA and protein expression. J. Evol. Biol. 29, 1234–1246. doi: 10.1111/jeb.12865
Turchyn N., Chesebro J., Hrycaj S., Couso J. P., Popadic A. (2011). Evolution of nubbin function in hemimetabolous and holometabolous insect appendages. Dev. Biol. 357, 83–95. doi: 10.1016/j.ydbio.2011.06.014
Zhang X., Rayner J. G., Blaxter M., Bailey N. W. (2021). Rapid parallel adaptation despite gene flow in silent crickets. Nat. Commun. 12, 50. doi: 10.1038/s41467-020-20263-4
Zirin J. D., Mann R. S. (2007). Nubbin and Teashirt mark barriers to clonal growth along the proximal-distal axis of the Drosophila wing. Dev. Biol. 304, 745–758. doi: 10.1016/j.ydbio.2007.01.025
Keywords: Acheta domesticus, nubbin, sound resonators, harp, mirror
Citation: Turchyn N and Popadić A (2024) Role of nubbin in the development of forewing sound-producing structures in Acheta domesticus (house cricket). Front. Ecol. Evol. 12:1411228. doi: 10.3389/fevo.2024.1411228
Received: 02 April 2024; Accepted: 21 June 2024;
Published: 12 July 2024.
Edited by:
Anupama Prakash, The University of Sheffield, United KingdomCopyright © 2024 Turchyn and Popadić. This is an open-access article distributed under the terms of the Creative Commons Attribution License (CC BY). The use, distribution or reproduction in other forums is permitted, provided the original author(s) and the copyright owner(s) are credited and that the original publication in this journal is cited, in accordance with accepted academic practice. No use, distribution or reproduction is permitted which does not comply with these terms.
*Correspondence: Aleksandar Popadić, YXBvcGFkaWNAd2F5bmUuZWR1