- 1Marine Research Center at Lomonosov Moscow State University, Moscow, Russia
- 2Lomonosov Moscow State University, Biological Faculty, Moscow, Russia
- 3Laboratory of the Arctic Land Shelf Interaction, National Tomsk State Research University, Tomsk, Russia
- 4Shirshov Institute of Oceanology, Russian Academy of Sciences, Moscow, Russia
- 5Laboratory of Arctic Oceanography, Moscow Institute of Physics and Technology, Dolgoprudny, Russia
- 6V.I. Il’ichev Pacific Oceanological Institute, Far Eastern Branch of the Russian Academy of Sciences (FEB RAS), Vladivostok, Russia
- 7V.I. Il’ichev Pacific Oceanological Institute, Far Eastern Branch, Russian Academy of Sciences, Vladivostok, Russia
Introduction: Methane seeps influence surrounding benthic communities in different ways from slight changes in benthic abundance and biomass to major altering the species composition.
Materials and Methods: We studied benthic communities of 14 methane seep flares in Laptev and East Siberian seas with comparative analysis of species composition and abiotic parameters at the nearby areas not affected by methane seeps. The species diversity was comparable at seep and non-seep sites varying from 3.9 to 39.6 taxa per 100 individuals and from 4.5 to 34.8 taxa per 100 individuals, correspondingly.
Results: The Laptev Slope community corresponds with the Polychaeta-community, encircling the upper continental slope area of the entire Siberian Arctic. The Lower Shelf community described in this study apparently corresponds with the Ophiocten sericeum community identified in the shelf areas of the Kara, Laptev and East Siberian seas. The Upper Shelf community is mostly inhabited by the bivalves species such as Portlandia arctica, Ennucula tenuis and Astarte montagui communities. The Estuarine community, which is the poorest by diversity but has high values of abundance and biomass is directly influenced by the Lena River runoff in terms of lower salinities and higher sedimentation rates.
Discussion: Throughout the study area, the differences between the Estuarine, Upper Shelf, Lower Shelf and Laptev Slope communities exceeded the differences between the seep and background non-seep areas. Several taxa demonstrated correlations with different environmental factors, including the latitude, depth, temperature, salinity, pH and methane content, not depending on the revealed benthic community. Eight taxa demonstrated correlations with the methane content measured at different sediment depths. Two siboglinids taxa demonstrated high abundances at stations with highest methane content deep in the sediment. At the Siberian shelf, our geochemical data for siboglinid habitats are the first to be published so far.
Introduction
Methane seeps are common in the Arctic Ocean but isolated from each other. Large methane seeps in the East Siberian arctic seas are zones of massive methane bubble emission with dissolved seawater methane concentrations elevated by factors of up to >100 above what would be expected from background (Shakhova et al., 2010). Recent discoveries with sonar images and geochemical quantification allow to suggest that the subsea permafrost is now thawing and releasing methane (Shakhova et al., 2010a; Shakhova et al., 2014; Shakhova et al., 2015; 2019; Leifer et al., 2017). It means that the permafrost “lid” is clearly perforated, and sedimentary CH4 is escaping to the water column and the atmosphere. The observed range in CH4 emissions associated with different degrees of subsea permafrost disintegration implies substantial and potent emission enhancement in the East Siberian arctic seas as the process of subsea permafrost thawing progresses with time (Leifer et al., 2017; Shakhova et al., 2017).
In the western part of the Arctic Ocean, methane seeps have been studied in some detail, including local benthic communities (Levin et al., 2000; Gebruk et al., 2003; Dando, 2010). Methane seep communities are different from common deep-sea communities. Benthic diversity at the cold seeps compared to the areas not affected by methane seepage, i.e., the background sites, is very different. Generally, the diversity index is lower within the seep areas (Levin, 2005). However, locally, the increased habitat heterogeneity can increase the overall benthic diversity (Gebruk et al., 2003; Levin, 2005; Sen et al., 2019). In the vicinity of deep-sea methane seeps, there are endemic species and taxonomic groups; in addition to them, the species diversity of common allochthonous invertebrate species increases (Gebruk et al., 2003; Baranov et al., 2020; Vedenin et al., 2020). At shallow depths, the inhabitants of methane seeps do not have obligate and seep-specific features (Dando, 2010; Kokarev et al., 2023).
Several seeps are known in the eastern part of the Arctic Ocean (Shakhova et al., 2010; Lobkovsky et al., 2022), and studies of the composition of benthic communities and endemic species have been conducted on them (Vedenin et al., 2020). However, benthic communities of the Laptev and East Siberian seas have not been sufficiently studied yet (Sirenko, 1998; Petryashev and Novozhilov, 2004; Sirenko et al., 2004; Sirenko and Denisenko, 2010; Kokarev et al., 2017; Vedenin et al., 2018; Kokarev et al., 2021). The previous surveys at the Siberian shelf and slope with no reference to the methane seepages show that diversity gradient was observed ascending from the coastal areas to the shelf edge (Petryashev and Novozhilov, 2004; Vedenin et al., 2018). The coastal shelf community, mostly inhabited by the bivalves species, corresponded to Portlandia arctica, Ennucula tenuis, and Astarte montagui communities, described in several surveys (Petryashev and Novozhilov, 2004; Sirenko and Denisenko, 2010; Kokarev et al., 2017). The community the Laptev Sea shelf previously described as the Ophiocten sericeum community, identified in the shelf areas of the Kara, Laptev, and East Siberian seas, revealed by the trawl samples (Zenkevich, 1963; Sirenko, 1998; Petryashev and Novozhilov, 2004; Sirenko and Denisenko, 2010). At the slope of the Laptev Sea, the community was described by Sirenko (1998) as the Polychaeta community, encircling the upper continental slope area of the entire Siberian Arctic. Although the abundance and biomass values were low within this community, the extrapolated species diversity was the highest within the Laptev slope, corresponding to recent data summarized from the central Arctic Ocean, where diversity maximum was observed at ~200 m to 300 m around the shelf edge (Vedenin et al., 2018, 2021b).
The current work examines the communities of previously studied seepages in the Laptev Sea in more detail; for the first time, the seepage area communities of the East Siberian Sea are studied, as well as a large-scale and accurate benthic survey of background shelf stations. All the seeps were discovered in the Eastern-Siberian arctic seas during the International Siberian Shelf Studies (Semiletov and Gustafsson, 2009). The general properties of massive methane release from these seepage areas and their isotopic signatures were described previously (Shakhova et al., 2015; Sapart et al., 2017; Shakhova et al., 2019; Steinbach et al., 2021).
During the selection, other characteristics of ecosystems were also recorded (bottom salinity, sediments type, concentration of organic matter, and methane in bottom water), so we were able to find out which parameters most influence the distribution of benthic communities on the shelf of the Laptev and East Siberian seas. We hypothesized that the depth (i.e., different depth strata) and the presence of active methane seepage are major factors influencing the structure of benthic communities within the study area.
Materials and methods
A total of 23 stations were obtained in the Laptev and East Siberian seas during the 82d cruise of RV “Akademik Mstislav Keldysh” in October 2020 (Figure 1). Field samplings and experiments were approved by the Ministry of Education and Science of the Russian Federation. The following information was supplied relating to field study approvals: DN-09–54/52 of 29.06.2020.
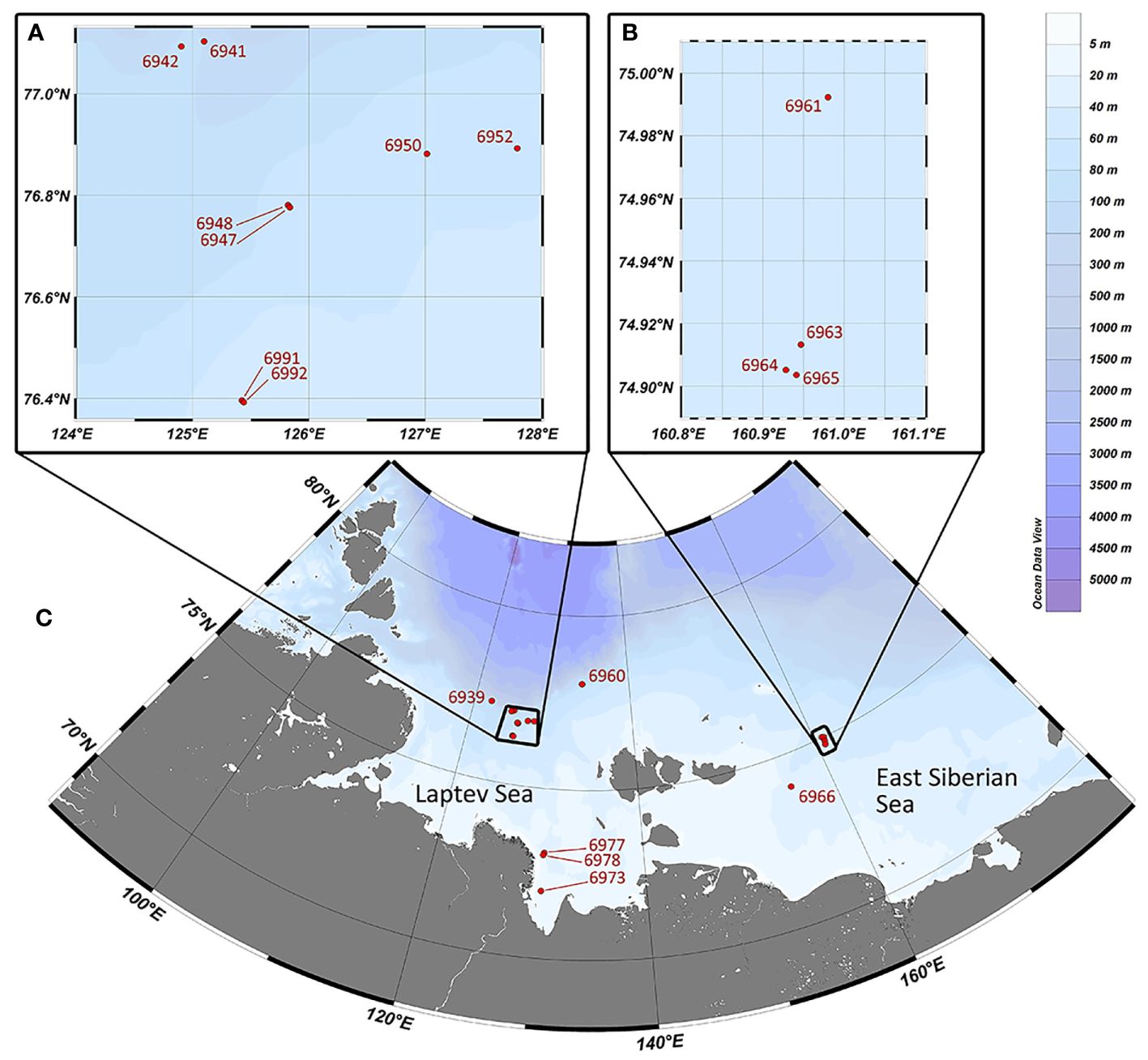
Figure 1 Study area and location of sampling stations (C). Enclosed areas show closely located stations at the Laptev Sea shelf (A) and the East Siberian Sea shelf (B).
From one to four “Okean” grabs (0.25 m2) taken at each station, the coordinates were chosen according to the detected locations of the methane seepages revealed by the acoustic flares (14 stations); control stations were taken in the background areas not affected by methane seepage (nine stations) (Eleftheriou and McIntyre, 2005). Station data with coordinates and depth are shown in Table 1.
All samples were washed onboard through the sieves of 0.5-mm mesh size and later fixed with 4% buffered formalin or with 96% ethanol. All organisms were sorted and identified in the laboratory to the lowest possible taxonomical level, counted, and weighed (wet weight and without decalcification). Polychaetes with calcareous (Spirorbidae) and mucous tubes (Chaetopteridae and Siboglinidae) were weighed with tubes. For the individuals fixed with ethanol, the biomass values were corrected according to coefficients introduced by Brotskaya and Zenkevich (1939).
Species abundance and biomass were calculated to square meters. Data on each species abundance is presented in the Supplementary Files. The similarity among the stations was estimated using the quantitative index of Bray–Curtis (square root transformed data, due to high dominance of certain taxa). Dendrogram was built on the basis of similarity matrices (Unweighted Pair Group Method with Arithmetic Mean (UPGMA) method); the cluster analysis was supplemented by non-metric multidimensional scaling (n-MDS). Clusters revealed by these methods were defined as separate benthic communities in terms of quantitative taxonomical similarity. Shade plot was used to visualize the species abundance differences between the stations and species in clusters (McCune et al., 2002; Clarke and Gorley, 2015).
Diversity parameters were estimated by the Simpson index (1 − λ) (McCune et al., 2002). In addition, due to low abundance and species number in some samples, the extrapolated diversity using Hill numbers (q = 0) for 100 individuals was calculated (further referred to as “Hill 100 extrapolated”). Detailed algorithms of extrapolation can be found in the work of Chao et al. (2014). Hill numbers were calculated using original non-transformed number of individuals in each sample. The difference between the benthic communities identified by cluster analysis was verified by the non-parametric Kruskal–Wallis test followed by the Dunn’s post-hoc test for abundance, biomass, and diversity indices (Lockwood, 1985). The contribution of individual taxa to the Bray–Curtis similarity between the communities was tested using the similarity percentages (SIMPER) analysis. For multiple groups (>2), individual pairwise comparisons were merged with mean dissimilarity and contribution calculated (Clarke and Warwick, 1994).
Environmental measurements were obtained immediately before sampling. Temperature and salinity values were measured in the near-bottom water with the Conductivity-Temperature-Depth (CTD) instrument (SBE 911plus). Methane content and pH values were measured in the sediment at different depths sampled by multicorer instrument. Samples of bottom sediments for determining methane concentrations were taken from the multicorer liner through preliminarily prepared holes with a resolution of 5 cm. The sediment in a volume of 5 cm3 was placed in hermetically split glass flasks with a volume of 20 mL. Extraction was performed according to the method of static headspace analysis. Thermostating took place at temperatures up to 25°C for at least 30 min. Sample analysis was performed on an SRI-8610c gas chromatograph equipped with a module with Helium Ionization Detector/Thermal Conductivity Detector (HID/TCD) and Flame Ionization Detector (FID) detectors. Methane concentrations were calculated according to the method of Yamamoto et al. (1976) modified by Wiesenburg and Guinasso (1979) using the calculated methane solubility constants. Weight control was additionally carried out for each sediment sample. Under laboratory conditions (20°C), the moisture content and specific gravity required in the calculations were determined.
The determination of pH in bottom sediments is carried out with a portable pH meter (Hanna, HI 991300, UK). Measurements were made according to the manufacturer method. The electrodes were washed with ionized water before measurements, and the instruments were calibrated every 24 h. The measurement error is pH ±0.02.
All resulting values are shown in Table 1. Spearman ranked correlation was calculated between the environmental values and values of total abundance, biomass, diversity indices, and individual species abundances. The p-values were adjusted by the Bonferroni correction (α = 0.05/#comparisons) to avoid the likelihood of type 1 error. The distance-based redundancy analysis (db-RDA) based on the Bray–Curtis dissimilarity matrix followed by Principal Coordinate Analysis (PCoA) was performed to visualize station distribution along the environmental vectors (Borcard et al., 2011). Taxa significantly correlated with the methane content were plotted as with general additive models (GAMs) (Hastie and Tibshirani, 1990).
Statistics was performed using Microsoft Excel 2010 software and original Python 3.8 scripts using NumPy, Pandas, Scipy, Scikit, Math, Matplotlib, and PyGAM libraries (https://www.python.org/downloads/release/python-380/). Maps were built using Ocean Data View software (Schlitzer, 2020).
Results
Description of samples and benthic communities
A total of 11,591 individuals from 209 taxa were obtained from the samples. Total abundance and biomass varied from 16 ind. m−2 and 1.03 g ww m−2 [station (st.) 6964] to 1,924 ind. m−2 and 188.37 g m−2 (st. 6977). The macrotaxa contributing most to the total abundance were the polychaetes (the majority of stations) or mollusks (sts. 6965–6981) (Figure 2A). At some stations, polychaetes were dominant in terms of the biomass (sts. 6939 and 6960); mollusks were dominating at sts. 6966–6981 and 6985; at a few stations, the echinoderms were contributing over 50% (st. 6950) or sipunculids (sts. 6961 and 6983) (Figure 2B).
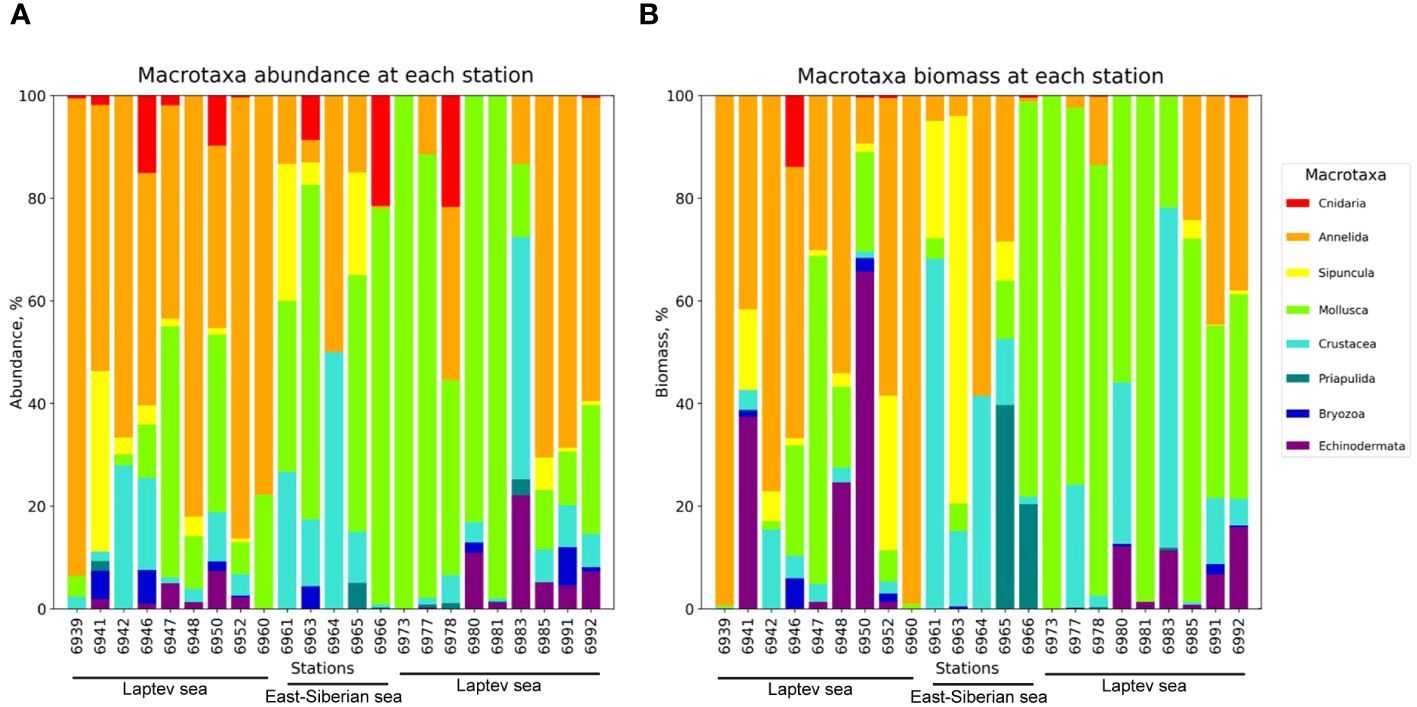
Figure 2 Percentage values of macrotaxa at each station. (A) Abundance percentage. (B) Biomass percentage.
Cluster analysis revealed four groups of stations (Figure 3). The four clusters that we chose were significantly supported by the PERMANOVA with p-values of <0.01. The clusters were named according to their approximate geographic position as the Laptev Slope, the Lower Shelf, the Upper Shelf, and the Estuarine communities. Each community contained the stations from both the methane seepage areas and from the background areas. Multidimensional scaling plot demonstrated similar results; however, the Kruskal stress level was rather low indicating poor ordination results (Figure 4). According to the SIMPER analysis, different taxa dominated each of the revealed communities (Table 2). Full pairwise comparisons are available in the Supplementary Files. Kruskal–Wallis test followed by the pairwise Dunn’s post-hoc comparison demonstrated no significant difference between the revealed communities in the total abundance and biomass. However, significant difference in the Hill 100 extrapolated diversity index was found (Table 3). Below is a brief description of each benthic community.
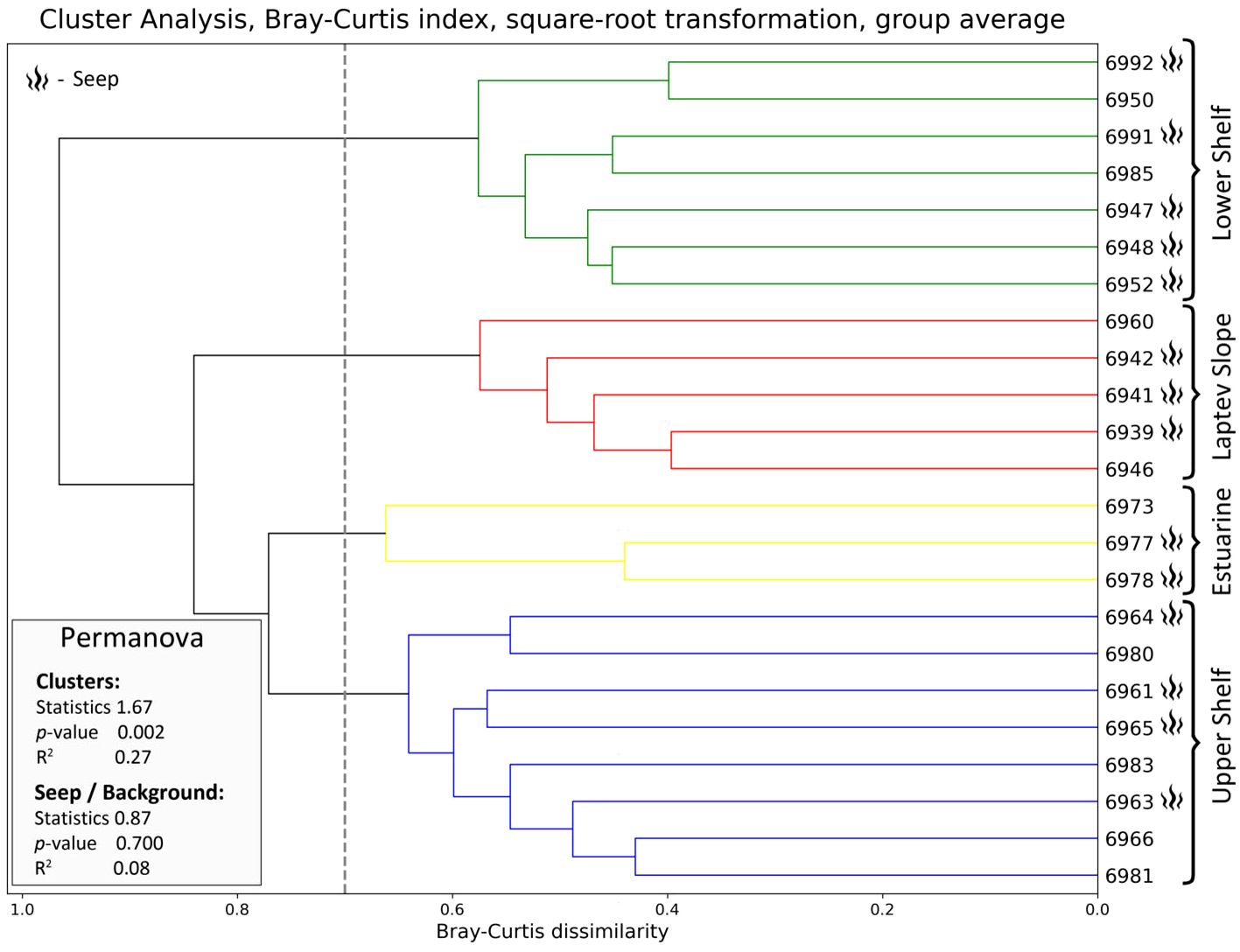
Figure 3 Cluster dendrogram of the stations based on the Bray–Curtis similarity index (square root transformed data). Each cluster further referred to as the benthic community is marked with corresponding color; active methane seepage areas are marked with the flame symbol. PERMANOVA results for marked clusters and for active seep/background samples are shown enclosed (sample size, 23; number of permutations, 1,000; number of groups, 4 and 2, correspondingly).
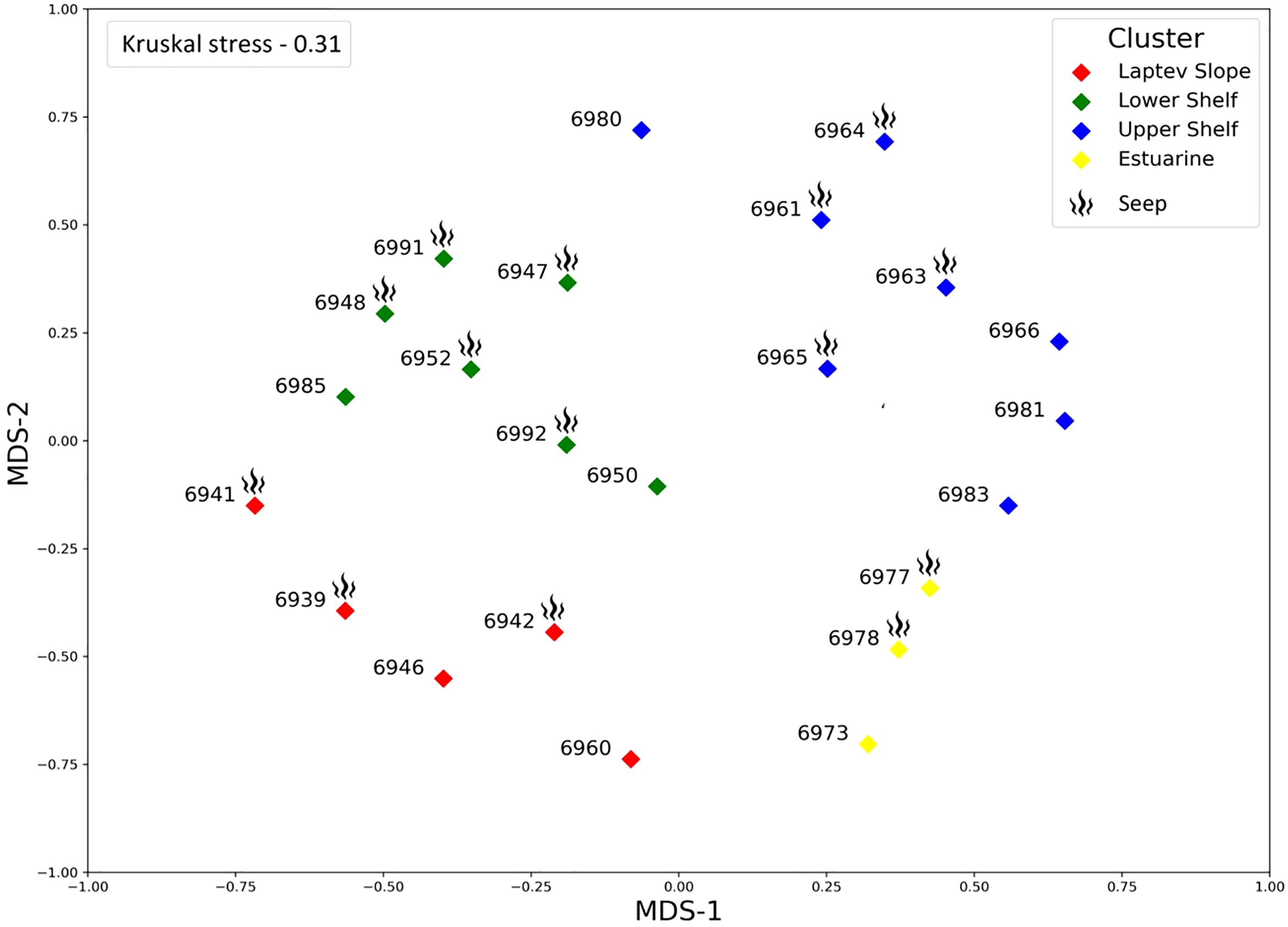
Figure 4 Non-metric multidimensional scaling plot of stations, based on the Bray–Curtis similarity index (square root transformed data). Colors and symbols as in Figure 3.
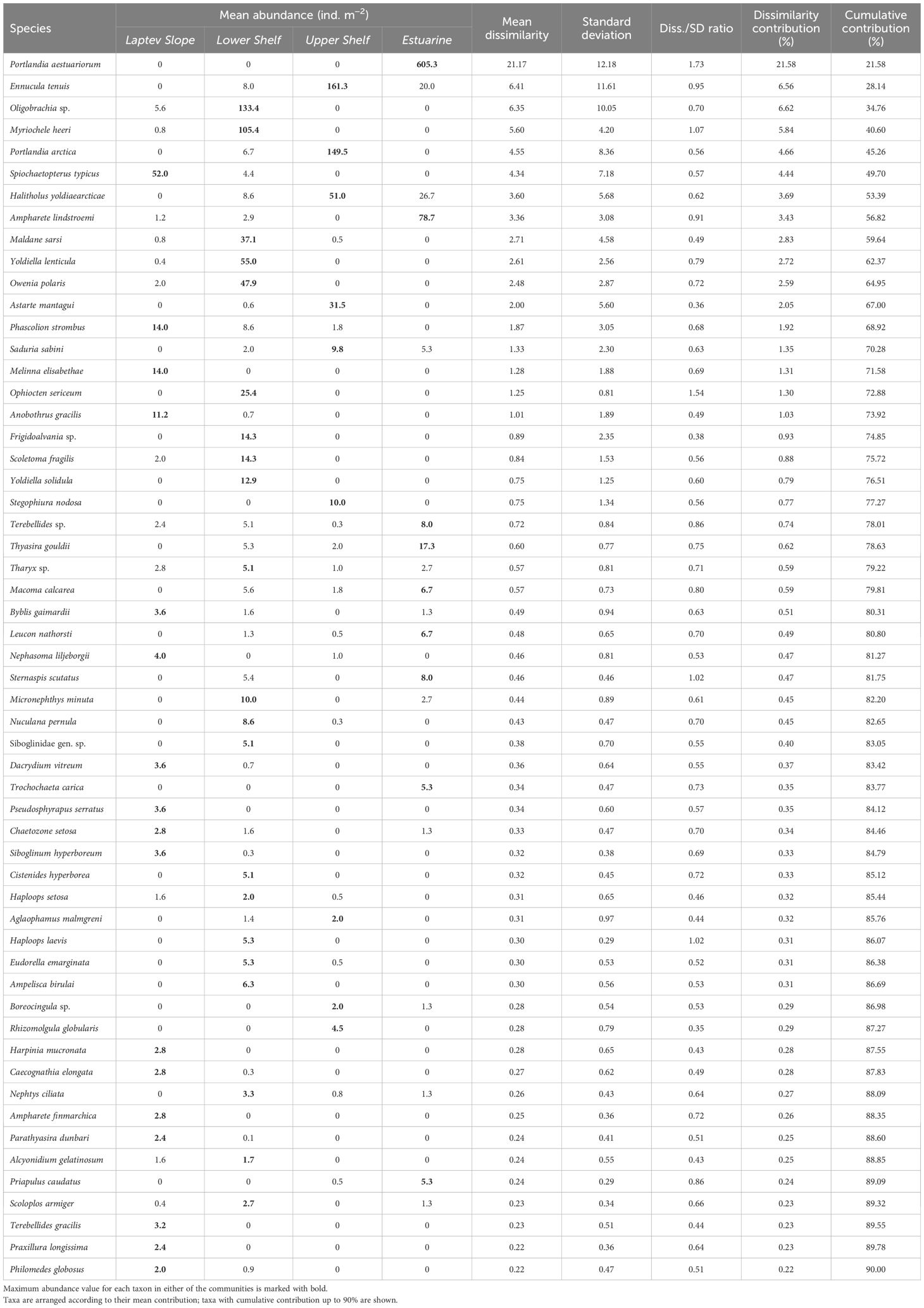
Table 2 Results of the SIMPER analysis with mean values of individual taxon abundance in each of the inferred communities (abundance data).
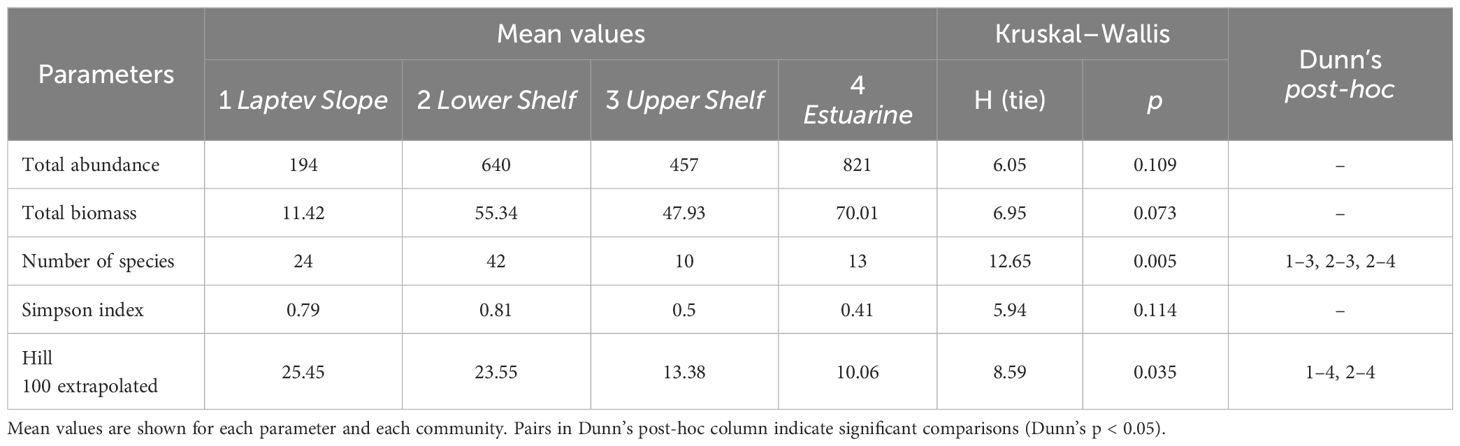
Table 3 Results of the Kruskal–Wallis and Dunn’s post-hoc tests for total abundance, biomass, and diversity indices in different benthic communities.
The Laptev Slope community consisted of five stations, located at the upper slope of the Laptev Sea within the 193-m to 364-m-depth range (Figures 3, 4). Values of the total abundance and biomass were the lowest for this community (mean values of <200 ind. m−2 and 11 g ww m−2; see Table 3). However, the extrapolated diversity was the highest (mean values over 25 taxa per 100 individuals; see Figures 5, 6). Main dominants in terms of abundance and biomass were the polychaete Spiochaetopterus typicus; other abundant taxa included Phascolion strombus sipunculids, Melinna elisabethae and Anobothrus gracilis polychaetes, siboglinids, and Pseudosphyrapus serratus tanaid crustaceans (Table 2, Figure 7).
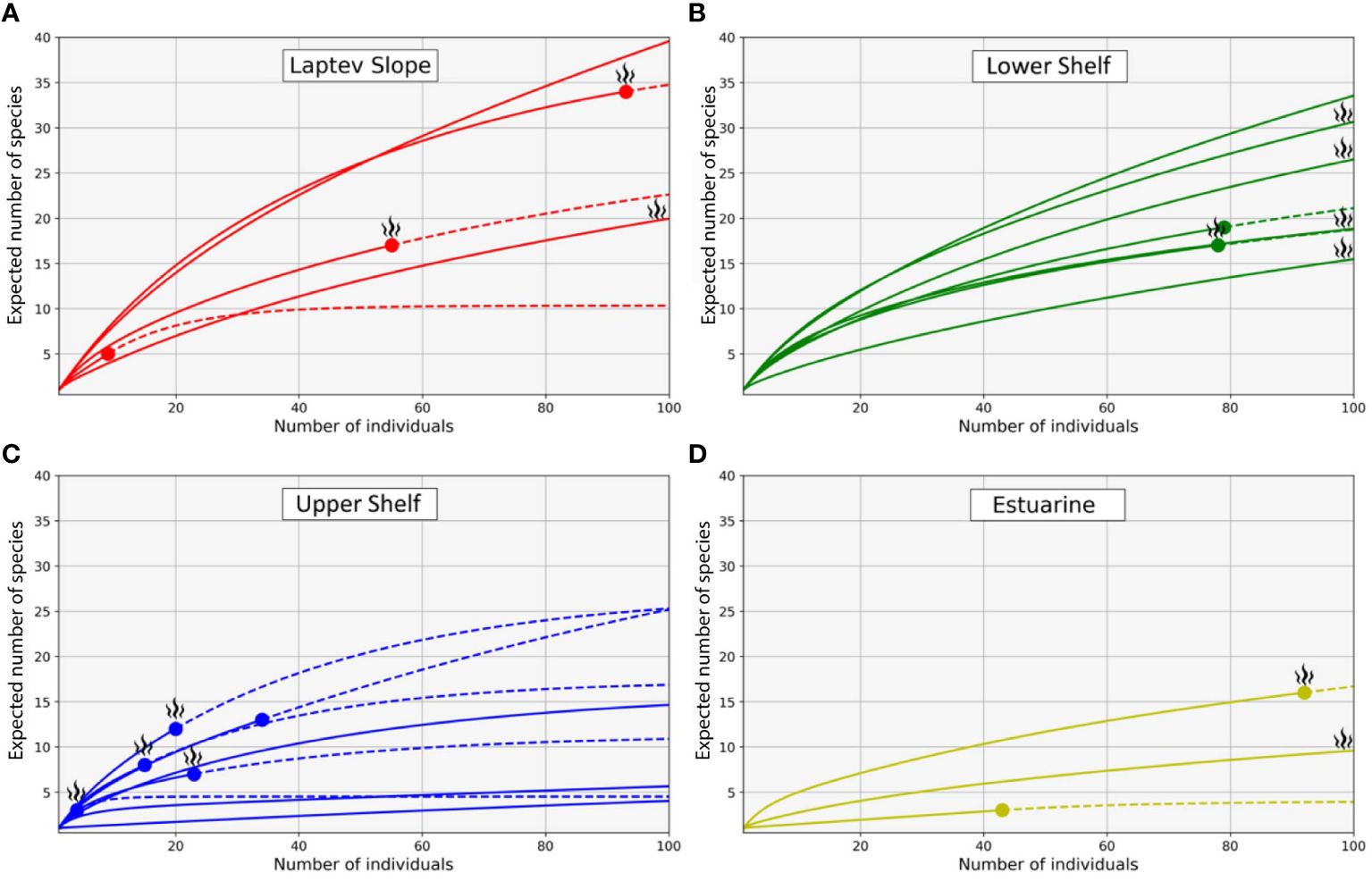
Figure 5 Rarefaction curves for the quantitative samples up to 100 individuals with extrapolation based on the Hill numbers (q = 0). (A) Laptev Slope community; (B) Lower Shelf community; (C) Upper Shelf community; (D) Estuarine community; color corresponds to benthic communities as in Figures 3, 4; active methane seepage areas are marked with flame symbol. Continuous lines indicate true (sample-sized) rarefaction; circles indicate the end of sample; dashed lines indicate the extrapolated rarefaction.
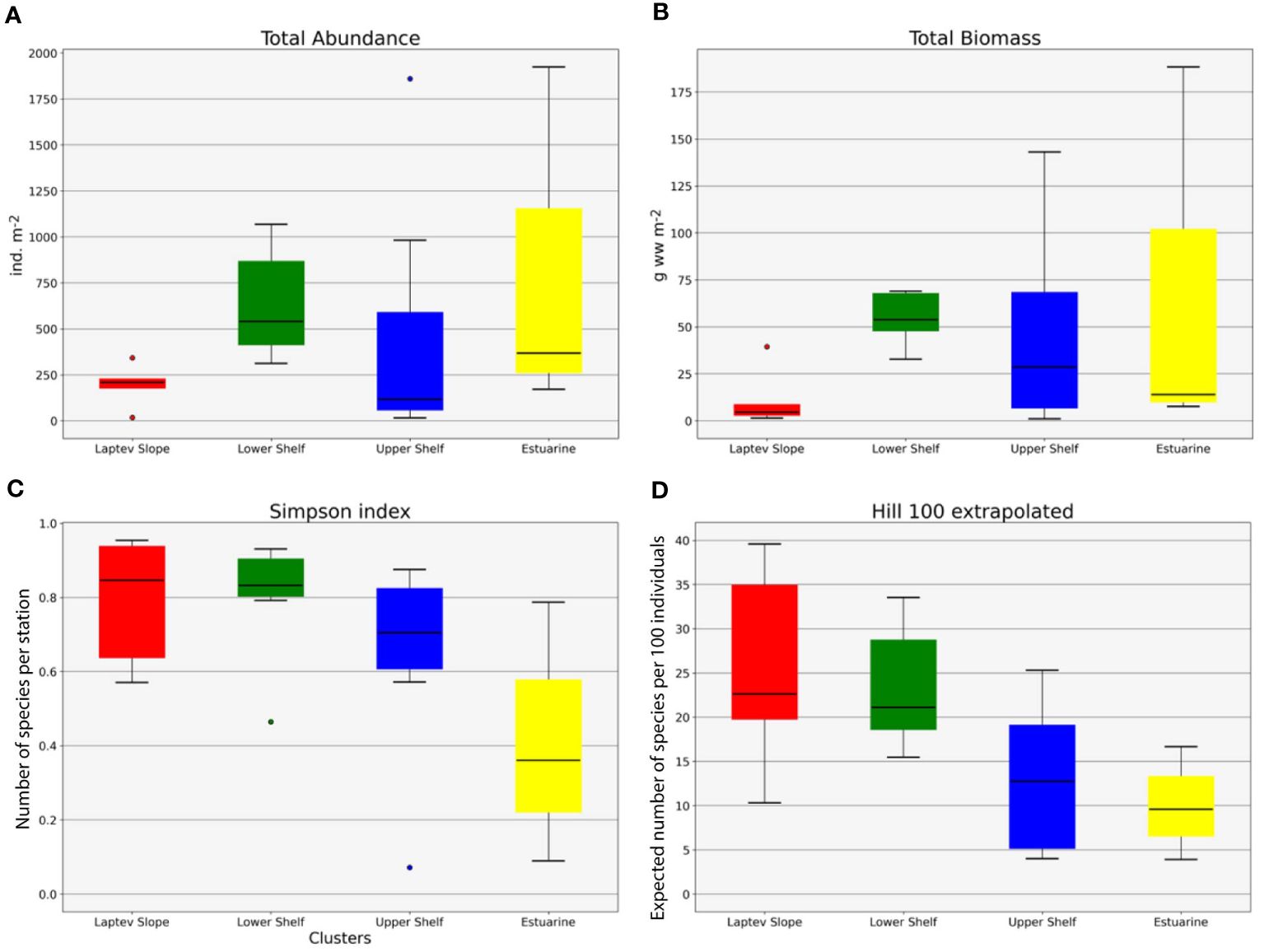
Figure 6 Univariative characteristics of identified communities expressed in standard box plots. Values of total abundance (A), biomass (B), Simpson index (C), and Hill 100 extrapolated (D) are shown. Each graph contains interquartile ranges (colored boxes), median values (horizontal line inside each box), and minimum and maximum values (lines outside the boxes). Colors are the same as in Figures 3–5.
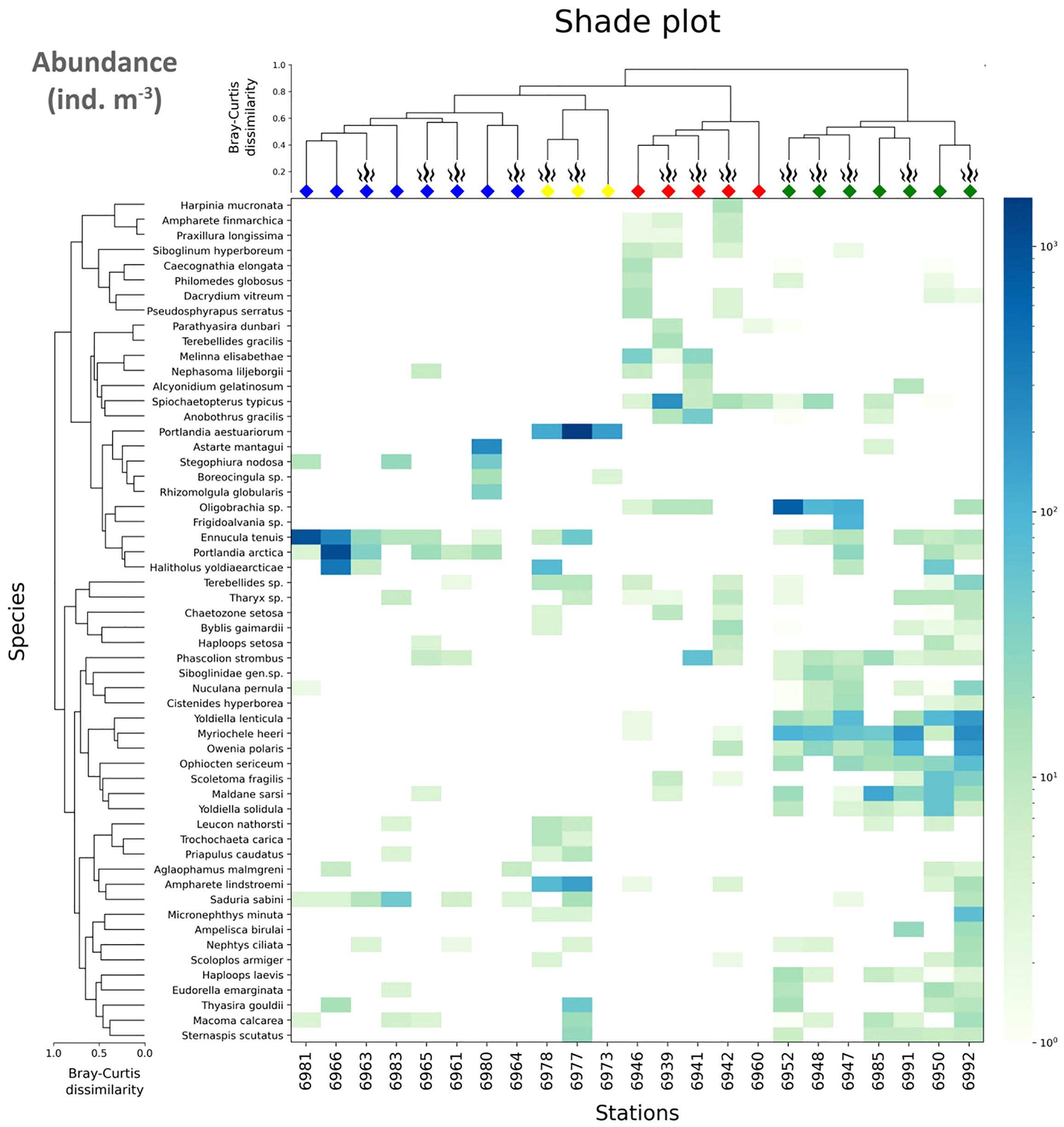
Figure 7 Shade plot of species square root transformed abundance at stations. The species list is reduced to 56 taxa presented in the SIMPER table. Order of stations is the same as in Figure 3; symbols and colors for station dendrogram are the same as in Figures 3, 4; taxa are grouped using the UPGMA algorithm based in the index of association. Abundance scale (color bar) is logarithmic.
The Lower Shelf community contained seven stations, located in the outer shelf of the Laptev Sea at the depth range of 51 m to 73 m (Figures 3, 4). Values of total abundance and biomass were very high (mean values over 600 ind. m−2 and 55 g ww m−2; see Table 3). Diversity was also very high with the mean species number of 42 and Hill 100 extrapolated of 24 (Figures 5, 6). Oweniidae polychaetes were dominant at all the Lower Shelf stations. At some of them, siboglinids Oligobrachia sp. were extremely dominant (over 700 ind. m−2 at st. 6952). Among other abundant species, Maldane sarsi polychaetes, Yoldiella lenticula mollusks, and Ophiocten sericeum ophiuroids should be mentioned (Table 2, Figure 7).
The Upper Shelf community contained eight stations, being the largest and the most widespread cluster in the sample set. Part of the stations was taken at the Laptev Sea shelf and part of them at the East Siberian Sea shelf; the depth range was 17 m to 46 m (Figures 3, 4). Abundance and biomass values were intermediate for this community (mean: 460 ind. m−2, 48 g ww m−2; Table 3). Diversity values were low (10 species per sample in average, 13 extrapolated taxa per 100 individuals; see Figures 5, 6). Bivalves were dominant by both abundance and biomass, including Ennucula tenuis, Portlandia arctica, and Astarte montagui species. High abundance was also recorded for hydroids Halitholus yoldiaearcticae symbiotic with P. arctica and for Stegophiura nodosa ophiuroids (Table 2, Figure 7).
The Estuarine community consisted of only three stations, all located near the Lena River Delta at depths of 17 m to 23 m (Figures 3, 4). This was the poorest community in terms of diversity with high values of abundance and biomass (over 800 ind. m−2 and 70 g ww m−2; only 10 species per 100 individuals; see Table 3, Figures 5, 6) due to a few species: bivalves Portlandia aestuariorum and polychaetes Ampharete lindstroemii (Table 2, Figure 7).
Seep vs. background species composition
Each of the inferred benthic communities consisted of both seep and background stations (Figures 3, 4). The overall community structure was not divided by the presence or absence of the active seepages, the corresponding PERMANOVA analysis showed p-values >0.0 5 (Figure 3). Integral community characteristics including the total abundance, biomass, and diversity indices did not differ significantly between the seep and background stations, except for the abundance and biomass within the Upper Shelf stations (Table 4). However, additional SIMPER analysis demonstrated certain differences in species composition between the seep and background stations within the Laptev Slope, Lower Shelf, and Upper Shelf communities; the Estuarine community consisted of only three stations and was, therefore, too small to run the SIMPER test (Table 5).
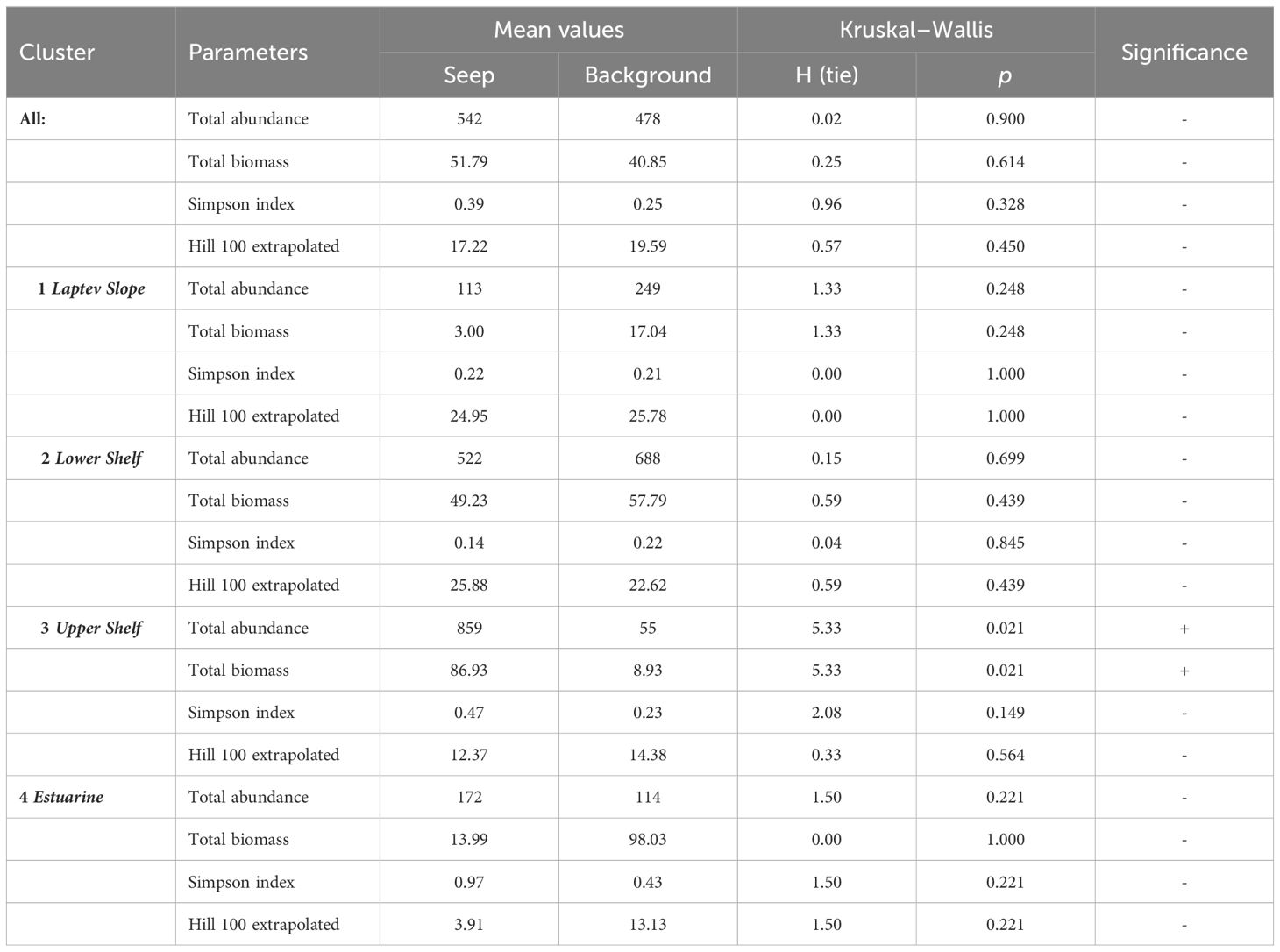
Table 4 Results of the Kruskal–Wallis and Dunn’s for total abundance, biomass, and diversity indices between the seep and background areas.
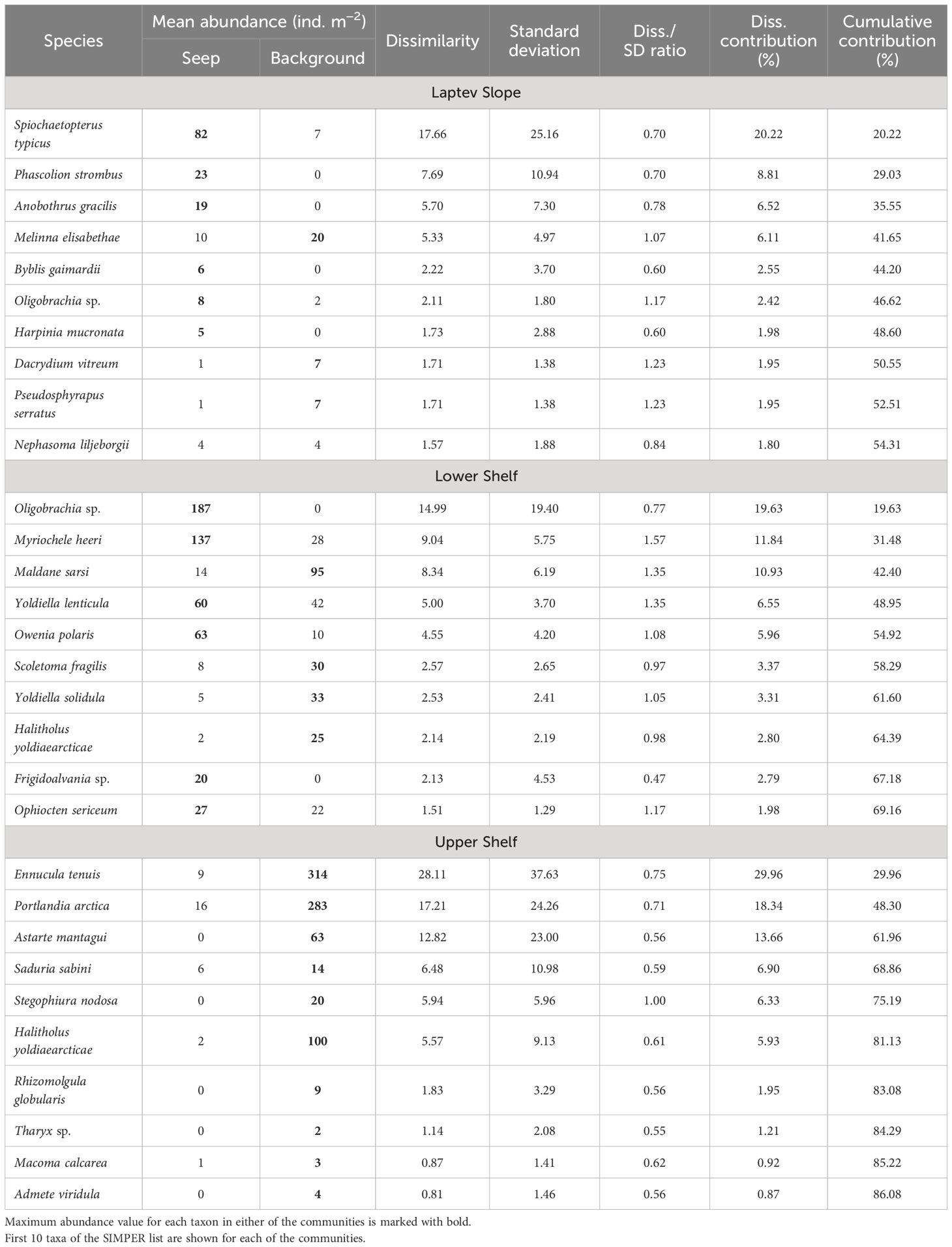
Table 5 Results of the SIMPER analysis between the seep and background stations within the Laptev Slope, Lower Shelf, and Upper Shelf benthic communities (abundance data).
In the Upper Shelf community, the majority of the taxa showed higher abundance values at the background stations than at the seep stations. In the Laptev Slope and Lower Shelf communities, most of the species either demonstrated similar abundances at the seep and background station or were less abundant at the seep stations (Table 5). However, several species, including the polychaetes Spiochaetopterus typicus and Anobothrus gracilis, sipunculid Phascolion strombus in the Laptev Slope community, and siboglinid Oligobrachia sp., polychaete Oweniidae, and gastropod Frigidalvania sp., were significantly more abundant at the seep stations (Table 5).
Environmental parameters and benthic communities
The environmental parameters including the temperature, salinity, depth, methane content, and pH were available for not all the stations (Table 1). For the distant-based redundancy analysis, we reduced the amount of stations from 23 to 15 in order to include the methane data to the plot (Figure 8). All stations were grouped along two vectors: the Depth (sts. 6939, 6941, and 6946) and more co-directional Salinity + Temperature + CH4. RDA1 and RDA2 eigenvalues explained 57.8% results, making the ordination acceptable.
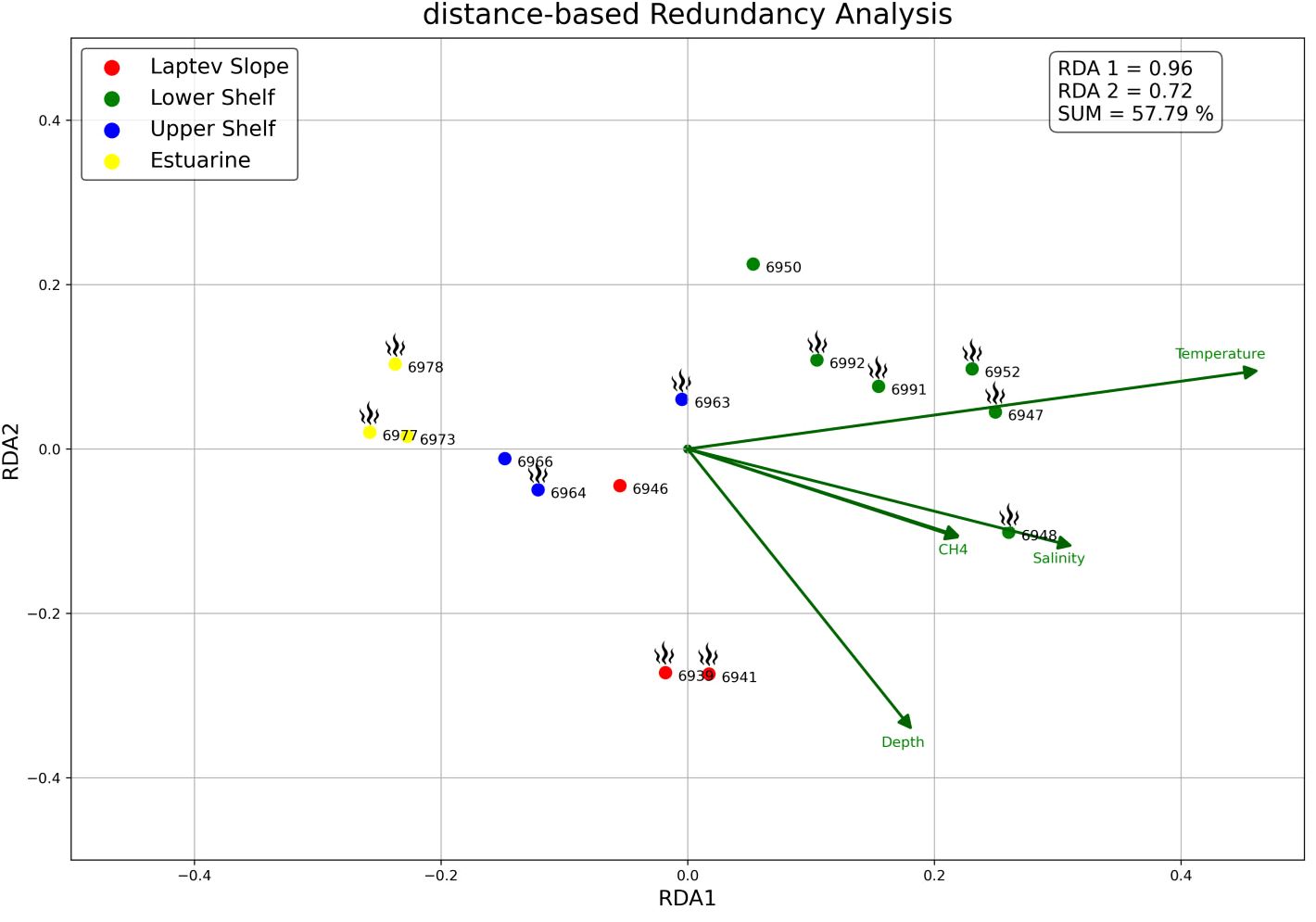
Figure 8 db-RDA plot of stations along the depth, temperature, salinity, and methane content (green vectors). Active methane seepage areas are marked with flame symbol. Colors of stations the same as in Figures 3, 4, 7. Response data are calculated by PCoA based on the square-root Bray–Curtis dissimilarity; scaling, 1.
Spearman ranked correlation was performed for diversity indices including the Simpson index and Hill 100 extrapolated (Figure 9). According to p-values, these parameters were significantly correlated with depth (after the Bonferroni correction) and, to a lesser degree, with temperature and salinity. Total abundance and biomass were not correlated with any of the environmental parameters measured; methane content and pH were also not correlated with any of the community integral parameters (Figure 9).
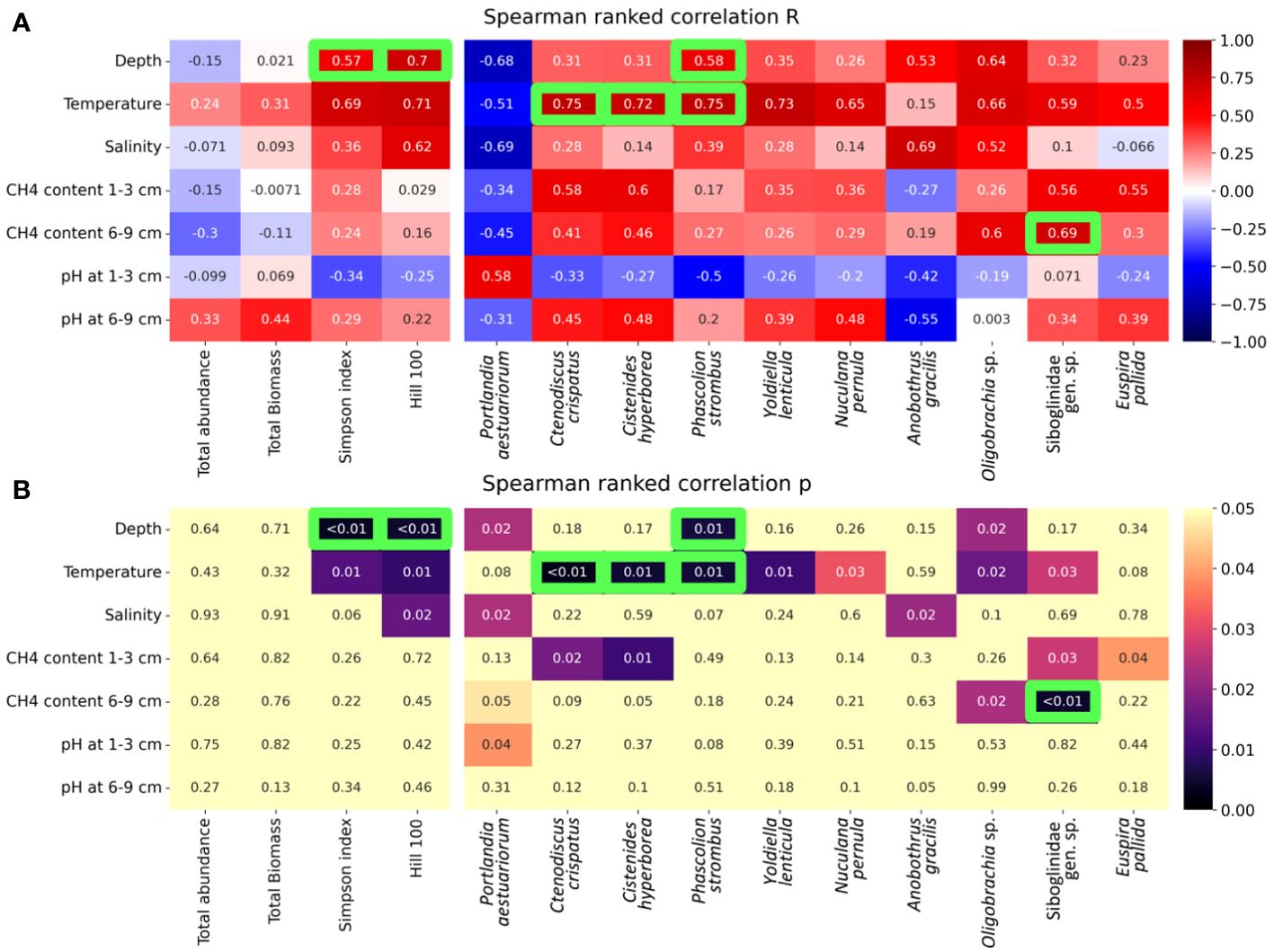
Figure 9 Heat maps of Spearman ranked correlation values between the environmental parameters and integral community parameters (total abundance, biomass, and diversity indices) and individual taxon abundances. (A) R-values; (B) p-values. Ten taxa with lowest mean p-values are shown. Green boxes mark the correlations that remained significant after the Bonferroni correction.
Over 10 taxa were significantly correlated with either of the environmental factors considered, although only four of them had the significant p-values after the Bonferroni correction (Figure 9). Most of them demonstrated positive correlation with temperature and/or depth (three taxa). A few taxa were positively correlated with the methane content: polychaetes Cistenides hyperborea and asteroids Ctenodiscus crispatus were most correlated with methane concentration at 1-cm to 3-cm sediment depth, and siboglinids Oligobrachia sp. and Siboglinidae gen. sp. were most correlated with methane concentration at 6-cm to 9-cm sediment depth (although only Siboglinidae gen. sp. showed significant p-values after the Bonferroni correction) (Figure 9).
The abundance of four taxa significantly correlated with the methane content was plotted in relation to methane concentration at different sediment layers; GAMs with Poisson distribution were calculated (Figure 10). For the siboglinids, GAM showed highest values of taxa abundance at methane concentration of ~104 to 105 µM L−1 at sediment depth of 6 cm to 9 cm. For polychaetes, Cistenides hyperborea maximum values of abundance, according to GAM, were at ~105 µM L−1 of methane at sediment depth of 1 cm to 3 cm; for asteroids, Ctenodiscus crispatus, the same was observed at methane concentration of ~10 µM L−1. The summary of the GAM models showed significant p-values for all the fitted models except for the C. crispatus vs. untransformed CH4 (Table 6).
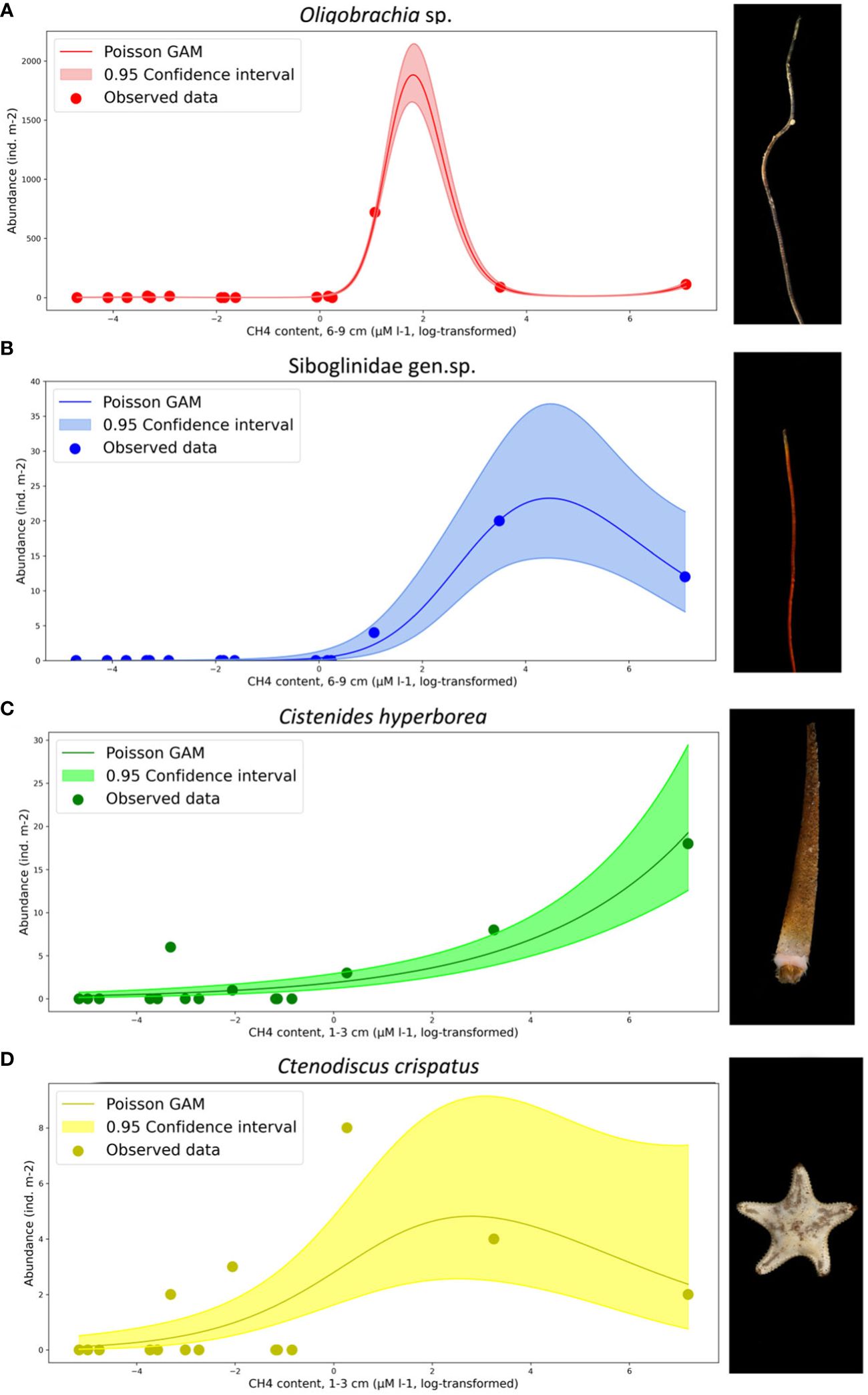
Figure 10 Abundance of four taxa in relation to methane concentration (log-log transformed data) with Poisson GAM (general additive model) and confidence GAM interval added. (A) Oligobrachia sp.; (B) Siboglinidae gen. sp.; (C) Cistenides hyperborea; (D) Ctenodiscus crispatus. Photos by A. Vedenin.
Discussion
Benthic abundance, biomass, and diversity
Methane seeps are known to influence surrounding benthic communities in various ways from slight changes in benthic abundance and biomass to major altering the species composition (Levin et al., 2000; Dando, 2010). Within the Arctic Ocean, significant increase of the benthic abundance and biomass was reported from several areas of the Atlantic sector of the Arctic, including the Vestnesa Ridge (e.g., five-fold increase in biomass from 0.5 to 3.0 g ww m−2 at 1,200 m to 1,500 m; Åström et al., 2018) and cold seeps south off Svalbard (two-fold increase in biomass from ~100 g to ~200 g ww m−2 at 200 m to 350 m; Åström et al., 2016). Increased biomass was also reported from the Håkon Mosby mud volcano, although no numbers are currently available (Gebruk et al., 2003). The mentioned cold seeps are located at depths of 200 m to 1,300 m, which is mostly deeper than the samples analyzed in this study. Within the Laptev Shelf, an increase of abundance was previously observed at two geographically compact shallow-water (63 m to 73 m) seep sites named C15 and Oden compared to the background control area (Vedenin et al., 2020). Specifically, a four-fold increase in abundance was observed at the C15 (from ~2,000 ind. m−2 to ~8,000 ind. m−2) and a less significant increase at the Oden (from ~2,000 ind. m−2 to ~3,000 ind. m−2); biomass changes were statistically insignificant (Vedenin et al., 2020). In this study, sts. 6947 and 6948 were geographically very close to the described C15 seep site, whereas st. 5952 was close to the Oden seep site (Table 1; Vedenin et al., 2020) and demonstrated high values of abundance and biomass (524 ind. m−2, 312 ind. m−2, and 1,018 ind. m−2 and 23.9 g ww m−2, 59.0 g ww m−2, and 58.2 g ww m−2, respectively; see the Supplementary Files). However, adding more stations from different areas (both seep and background, including those from the neighboring shelf areas) probably blurred the effect of two previously described sites, likely due to varying activity of the numerous small cold seeps around the C15 and Oden. Overall, across the entire study area from the Lena River Delta to the upper Laptev Sea slope, the differences in abundance and biomass (including those between the seep and background areas) were insignificant (see Table 3, Figures 6A, B).
Benthic diversity at the cold seeps compared to the background is very different. Generally, the diversity expressed in ES-100 or Shannon–Wiener index is lower within the seep areas (Levin, 2005). However, locally, the increased habitat heterogeneity caused, e.g., by the presence of carbonate crusts, can increase the overall benthic diversity (Gebruk et al., 2003; Levin, 2005; Sen et al., 2019). Previously, very high values of the ES-100 and Shannon–Wiener index within the Oden seep site were reported with >30 taxa per 100 individuals compared to ~20–25 taxa per 100 individuals in the background (Vedenin et al., 2020). According to our data, this is not correct for the all seep sites used in this study; the species diversity was comparable at seep and non-seep sites varying from 3.9 to 39.6 taxa per 100 individuals and from 4.5 to 34.8 taxa per 100 individuals, correspondingly (Table 3, Figure 5). The diversity was significantly different, however, in different benthic communities, being the lowest near the Lena River Delta and the highest at the Laptev Sea upper continental slope or at the outer shelf, depending on the diversity index used (Table 3, Figure 6). This corresponds with the previous surveys at the Siberian shelf and slope with no reference to the methane seepages, where diversity gradient was observed from the coastal areas to the shelf edge (Petryashev and Novozhilov, 2004; Vedenin et al., 2018).
Benthic communities’ characteristics
Four benthic communities revealed in this study (Laptev Slope, Lower Shelf, Upper Shelf, and Estuarine) correspond with the previously reported from the Laptev and East Siberian seas during multiple ecological surveys (Sirenko, 1998; Petryashev and Novozhilov, 2004; Sirenko et al., 2004; Sirenko and Denisenko, 2010; Kokarev et al., 2017; Vedenin et al., 2018; Kokarev et al., 2021). Specifically, the Laptev Slope community corresponds with the Polychaeta community identified by Sirenko (1998), encircling the upper continental slope area of the entire Siberian Arctic. Sirenko et al. (2004) reported polychaete community dominated by Spiochaetopterus typicus with high amount of ophiuroids Ophiopleura borealis at the depths of ~200 m to 500 m in the Laptev Sea. In our samples, several polychaete species were dominant at 193 m to 354 m including S. typicus, Anobothrus gracilis, and Melinna elisabethae (Table 2, Figure 7). Although the abundance and biomass values were low within this community, the extrapolated species diversity was the highest within the Laptev Slope, corresponding together to the common community ecology paradigm (Weiher and Keddy, 1999; Piraino et al., 1999) and to recent data summarized from the central Arctic Ocean, where diversity maximum was observed at ~200 m to 300 m around the shelf edge (Vedenin et al., 2018, 2021b). This community is influenced (and probably conditioned) by the warm Atlantic waters, enriched with organic carbon and flowing eastward at these depths (Wassmann et al., 2019; Bluhm et al., 2020).
The Lower Shelf community described in this study apparently corresponds with the Ophiocten sericeum community, identified in the shelf areas of the Kara, Laptev, and East Siberian seas, revealed by the trawl samples (Zenkevich, 1963; Sirenko, 1998; Petryashev and Novozhilov, 2004; Sirenko and Denisenko, 2010). Grab samples, containing fewer members of megafauna, demonstrated the dominance of the bivalves, including Yoldiella solidula and Yoldiella lenticula and, sometimes, polychaetes Maldanidae (Kokarev et al., 2017; Vedenin et al., 2018). In our samples, species composition was similar, although shifted significantly to the polychaete dominance at seep stations, mostly Oweniidae and Siboglinidae (Table 5).
The Upper Shelf community, mostly inhabited by the bivalves species, corresponded to Portlandia arctica, Ennucula tenuis, and Astarte montagui communities, described in several surveys (Petryashev and Novozhilov, 2004; Sirenko and Denisenko, 2010; Kokarev et al., 2017). So far, no direct comparison between the Laptev and East Siberian shelves was published. In our samples, community composition at three stations from the Laptev Sea and at five stations from the East Siberian Sea was surprisingly similar, intermixed at the cluster dendrogram (Figure 3). Located at depths of 17 m to 46 m within the inner Siberian shelf, this community is influenced by the freshwater runoff (Kokarev et al., 2017; Kokarev et al., 2021).
The Estuarine community, the poorest by diversity but with high values of abundance and biomass, is directly influenced by the Lena River runoff in terms of lower salinity and higher sedimentation rate values (Drits et al., 2021). Dominated by bivalves, Portlandia aestuariorum, this community corresponds to estuarine communities, formed near large Siberian rivers, including Ob, Yenisei, Lena, Kolyma, Khatanga, Mackenzie, and other rivers (Jørgensen et al., 1999; Deubel et al., 2003; Aitken et al., 2008; Sirenko and Denisenko, 2010; Vedenin et al., 2015).
Seep vs. background
Throughout the study area, the differences between the Estuarine, Upper Shelf, Lower Shelf, and Laptev Slope communities exceeded the differences between the seep and background areas. Total abundance, biomass, and diversity showed no significant differences between the seep and non-seep samples (Table 4). However, within each of the benthic communities (except for the Estuarine), certain differences in taxonomical composition were revealed (Table 5). The Estuarine community consisted of only three stations, therefore making statistically insignificant SIMPER results (Table 4).
The Laptev Slope stations were located at the depth range, where significant peculiarities in species composition are known from the cold seeps worldwide (>200 m; Levin, 2005; Dando, 2010). In the Arctic cold seeps, high abundances of chemosymbiotrophic taxa were reported (mainly different species of Siboglinidae, all obligatory symbiotrophic) from depths exceeding 200 m as it was in the Barents Sea cold seeps (Åström et al., 2016), Vestnesa Ridge (Åström et al., 2018; Sen et al., 2020), Håkon Mosby mud volcano (Gebruk et al., 2003), mud volcanoes of the Beaufort Sea (Paull et al., 2015), and Lofoten-Vesterålen canyons (Sen et al., 2019). Apart from siboglinids, several species of molluscs are known to inhabit cold seeps, including probably symbiotrophic recently extinct Thyasiridae bivalves (west off Svalbard; Åström et al., 2018) and Rissoidae gastropods, grazing at bacterial colonies [at Håkon Mosby mud volcano (Gebruk et al., 2003) and in Lofoten-Vesterålen canyons (Sen et al., 2019)]. Another taxon, sometimes associated with the Arctic cold seeps, is Tanaidacea crustaceans. For the Arctic Ocean, several species were described from the Håkon Mosby mud volcano (Błażewicz-Paszkowycz and Bamber, 2011), although it is not known whether they are restricted to the cold seeps environment. In our samples, only a low amount of Oligobrachia sp. and Siboglinum hyperboreum siboglinids was found in the seep samples from 193-m to 364-m-depth range (Table 2; Supplementary 1), whereas most of the taxa demonstrated similar densities within outside the active methane seepages. In addition, comparing to the background stations, an incredibly high amount of Spiochaetopterus typicus polychaetes was observed at the seep stations (Table 5). Nothing is known about S. typicus ability to symbiotrophy; however, certain members of Chaetopteridae family are known to inhabit reduced habitats, e.g., deep-sea hydrothermal vents or whale falls (Nishi and Rouse, 2007; Kiel and Dando, 2009; Nishi and Rouse, 2014). Anyway, S. typicus is an extremely widespread species, inhabiting vast areas of the Arctic Ocean shelf and slope (Vedenin et al., 2021a).
The Lower Shelf stations were located shallower than the usual faunal response to the methane seepage that occurs. Previously, large quantities of Oligobrachia sp. siboglinids were reported from the C15 and Oden seep sites as the shallowest known localities for this species (Baranov et al., 2020; Vedenin et al., 2020). Furthermore, shallow-water localities of different siboglinids were recently discovered in the Kara Sea (Smirnov et al., 2020; Karaseva et al., 2021a) and in the East Siberian Sea (Karaseva et al., 2021b). In our samples (all taken in the Laptev Sea shelf at 51 m to 73 m), significantly higher densities of Oligobrachia sp. siboglinids, Oweniidae polychaetes, and Frigidalvania sp. gastropods were found at seep stations compared to the background ones, which is in accordance with previously published species lists for the C15 and Oden seep sites (Vedenin et al., 2020). As noted previously, the overall difference between the seep and non-seep Lower Shelf samples in this study was less significant than described for the C15 and Oden (see first subsection of the Discussion). A possible explanation is due to larger area covered by stations in this study, therefore, sampling more cold seeps and more background samples. Compared to C15 and Oden, other seep sites could be smaller in area or lower in activity, although this is yet to be investigated in future.
The Upper Shelf stations (both seep and background ones) were located even shallower and closer to the coast than the Lower Shelf stations. In this community, the seep samples demonstrated significantly lower abundance of most of species, compared to the background samples (Table 5). This corresponds with the known distribution patterns of macrobenthos at the shallow coastal cold seeps, like the described for the North Sea pockmarks (Dando et al., 1991), with little or no faunal response to methane presence, or the Baltic Sea pockmarks (Pimenov et al., 2008; Ezhova et al., 2012), where significant depletion of fauna was observed. Many authors agree that the chemosynthesis-derived organic (e.g., from cold seeps or hydrothermal vents) is a major source for benthic consumers only at depths, where the photosynthesis-derived organic is depleted (Powell et al., 1986; Levin, 2005; Tarasov et al., 2005; Dando, 2010). At the shallow-water areas, the chemosynthesis-derived organic input to the macrofauna is usually low or absent, probably due to unfavorable environment (methane or sulfides, toxic for many benthic organisms), as confirmed for the North Sea pockmarks and for Northern California (Dando et al., 1991; Levin et al., 2000); in both areas, the signatures of the chemosynthetic contribution to the macrofaunal diet were little or even absent.
Several taxa demonstrated correlations with different environmental factors, including the latitude, depth, temperature, salinity, pH, and methane content (Figure 9), not depending on the revealed benthic community. The methane content was higher at the seep stations (see Table 1), although the seep/non-seep stations were determined by the acoustic flares, not by the sediment chemical parameters. Eight taxa demonstrated correlations with the methane content measured at different sediment depths with Spearman p-values of <0.03 observed for four species: siboglinids Oligobrachia sp., Siboglinidae gen. sp., polychaetes Cistenides hyperborea, and asteroids Ctenodiscus crispatus. Two latter taxa, although not caught by SIMPER analysis, showed higher abundance within the stations with high methane content near the sediment surface (Figures 10C, D). Based on Figure 10, highest densities of C. hyperborea corresponded to methane concentration of ~105 µM L−1. C. crispatus reached largest quantities at much lower methane concentration of ~10 µM L−1. Both taxa could possibly act as grazers at the seep sites, consuming sediment with chemoautotrophic organic matter. For polychaetes, such behavior was reported for many species in various cold seeps and hydrothermal vents (Sibuet et al., 1988; Levin, 2005). For echinoderms, higher quantities of juvenile specimens were observed within or near the seep sites, e.g., ophiuroids on the continental slope of Chile (Quiroga and Sellanes, 2009). Similar aggregations were reported for holothurians, echinoids, and asteroids of Oregon, California, and Gulf of Mexico (MacDonald et al., 2004; Levin, 2005). The phenomenon of increased abundance of certain widespread taxa around the cold seeps was described by many authors (summarized by Levin, 2005; Dando, 2010).
In contrast to polychaetes and ophiuroids described, two siboglinids taxa (Oligobrachia sp. and Siboglinidae gen. sp.) demonstrated high abundances at stations with highest methane content deep in the sediment, measured at 6 cm to 9 cm from the surface (Figures 10A, B). The number of data point for the siboglinids is also small, but both species reached maximum densities at methane concentration of ~104 to 105 µM L−1. A few correlations between Siboglinidae species have been published so far. For the Antarctic cold seeps in Bransfield Strait, Sclerolinum genus was found at methane concentrations of <25 µM L−1 (Sahling et al., 2005). In the Skagerrak, siboglinid Siboglinum poseidoni reached high densities within the methane concentrations of 300 µM L−1 to 3,300 µM L−1 (Dando et al., 1994). In the Sea of Okhotsk, Siboglinum caulleryi and Oligobrachia dogieli siboglinids were found at extremely high concentrations of 4 × 106 µM L−1 (Karaseva et al., 2020). In the Arctic Ocean, high densities of Sclerolinum contortum and Oligobrachia haakonmosbiensis siboglinids were found at methane concentration of >2.103 µM L−1 (Pimenov et al., 1999; Lein et al., 2000; Smirnov, 2000). At the Vestnesa Ridge, over 2,000 ind. m−2 of unidentified siboglinids (belonging to Oligobrachia genus; see Sen et al., 2020) were found within the methane concentration of ~104 µM L−1 measured at 5 cm to 15 cm below the seafloor surface (Åström et al., 2018). At the Siberian shelf, our geochemical data for siboglinid habitats are the first to be published so far.
Although an increase in benthic diversity in the cold seep areas has previously been described, this was not confirmed in our studies. This may to be significant for deep seeps communities, which have few sources of organic matter. In addition, this result may be explained by the fact that a larger number of samples in our studies “blurred” the effect of increasing diversity in the area of seeps.
The diversity gradient of benthic communities rather confirmed the previously described patterns of increasing the number of species from coast to continental slope. However, several species, including the polychaetes Spiochaetopterus typicus and Anobothrus gracilis, sipunculid Phascolion strombus in the Laptev Slope community, siboglinid Oligobrachia sp., polychaete Oweniidae, and gastropod Frigidalvania sp., were significantly more abundant at the seep stations.
Further research is planned for studying the trophic features and possible symbiotrophy of individual species together with the microbiome of benthic invertebrates, as well as studying the biodiversity of benthic communities in the East Siberian Sea.
Author’s Note
The following information was supplied relating to field study approvals (i.e., approving body and any reference numbers): DN-09–54/52 of 29.06.2020.
Field samplings and experiments were approved by the Ministry of Education and Science of the Russian Federation.
Data availability statement
The original contributions presented in the study are included in the article/Supplementary Material. Further inquiries can be directed to the corresponding author.
Ethics statement
The manuscript presents research on animals that do not require ethical approval for their study.
Author contributions
OK: Conceptualization, Funding acquisition, Investigation, Project administration, Supervision, Writing – original draft, Writing – review & editing. NR-K: Investigation, Writing – original draft, Writing – review & editing, Conceptualization, Funding acquisition, Methodology. PK: Investigation, Writing – original draft, Writing – review & editing, Data curation, Validation. AO: Data curation, Investigation, Writing – original draft, Writing – review & editing, Formal analysis, Funding acquisition. MF: Formal analysis, Investigation, Writing – original draft, Writing – review & editing. IM: Funding acquisition, Investigation, Methodology, Writing – original draft, Writing – review & editing. DP: Funding acquisition, Investigation, Methodology, Writing – original draft, Writing – review & editing. DK: Funding acquisition, Investigation, Methodology, Writing – original draft, Writing – review & editing. IS: Funding acquisition, Project administration, Supervision, Writing – original draft, Writing – review & editing.
Funding
The author(s) declare financial support was received for the research, authorship, and/or publication of this article. AO was supported by the Moscow Institute of Physics and Technology development program Priority-2030. NR-K was supported by the Russian Science Foundation (grant # 20–74-10011). OK, DP, JM, and IS were supported by the Ministry of Science and Higher Education of the Russian Federation (grant “Priority -2030” to TSU), DK was supported by Russian Scientific Foundation (grant # 21–77-30001 to POI). The charter of the RV Academician Mstislav Keldysh was funded by grant 021–2021-0010 from the Ministry of Science and Higher of the Russian Federation.
Acknowledgments
We thank Andrey Vedenin for performing statistical analysis and first draft of the manuscript and also the crew and participants of the 82nd cruise of R/V “Akademik Mstislav Keldysh” for successful sampling in the Laptev and East Siberian Sea.
Conflict of interest
The authors declare that the research was conducted in the absence of any commercial or financial relationships that could be construed as a potential conflict of interest.
Publisher’s note
All claims expressed in this article are solely those of the authors and do not necessarily represent those of their affiliated organizations, or those of the publisher, the editors and the reviewers. Any product that may be evaluated in this article, or claim that may be made by its manufacturer, is not guaranteed or endorsed by the publisher.
Supplementary material
The Supplementary Material for this article can be found online at: https://www.frontiersin.org/articles/10.3389/fevo.2024.1406680/full#supplementary-material
References
Aitken A. E., Conlan K., Renaud P. E., Hendrycks E., McClelland C., Archambault P., et al. (2008). “The Benthic environment, Chapter 9,” in On thin ice: A synthesis of the Canadian Arctic Shelf Exchange Study (CASES). Eds. Fortier L., Barber D., Michaud J. (Vinnipeg, USA: Aboriginal Issues Press, University of Mani-toba), 159–199.
Åström E. K., Carroll M. L., Ambrose W. G. Jr., Carroll J. (2016). Arctic cold seeps in marine methane hydrate environments: impacts on shelf macrobenthic community structure offshore Svalbard. Mar. Ecol. Prog. Ser. 552, 1–18. doi: 10.3354/meps11773
Åström E. K., Carroll M. L., Ambrose W. G. Jr., Sen A., Silyakova A., Carroll J. (2018). Methane cold seeps as biological oases in the high-Arctic deep sea. Limnol. Oceanogr. 63, S209–S231. doi: 10.1002/lno.10732
Baranov B., Galkin S., Vedenin A., Dozorova K., Gebruk A., Flint M. (2020). Methane seeps on the outer shelf of the Laptev Sea: characteristic features, structural control, and benthic fauna. Geo-Marine Lett. 40, 541–557. doi: 10.1007/s00367-020-00655-7
Błażewicz-Paszkowycz M., Bamber R. N. (2011). Tanaidomorph Tanaidacea (Crustacea: Peracarida) from mud-volcano and seep sites on the Norwegian Margin. Zootaxa 3061, 1–35. doi: 10.11646/zootaxa.3061.1
Bluhm B. A., Janout M. A., Danielson S. L., Ellingsen I., Gavrilo M., Grebmeier J. M., et al. (2020). The pan-Arctic continental slope: sharp gradients of physical processes affect pelagic and benthic ecosystems. Front. Mar. Sci. 7. doi: 10.3389/fmars.2020.544386
Borcard D., Gillet F., Legendre P. (2011). Numerical ecology with R Vol. 2 (New York: Springer), 688. doi: 10.1007/978-1-4419-7976-6
Brotskaya V., Zenkevich L. (1939). Quantitative account of the Barents Sea benthic fauna Vol. 4 (Moscow, Russia: Trudy VNIRO), 55–120.
Chao A., Gotelli N. J., Hsieh T. C., Sander E. L., Ma K. H., Colwell R. K., et al. (2014). Rarefaction and extrapolation with Hill numbers: a framework for sampling and estimation in species diversity studies. Ecol. Monogr. 84, 45–67. doi: 10.1890/13-0133.1
Clarke K. R., Gorley R. N. (2015). Getting started with PRIMER v7. PRIMER-E Vol. 20 (Plymouth: Plymouth Marine Laboratory).
Clarke K. R., Warwick R. M. (1994). Similarity-based testing for community pattern: the two-way layout with no replication. Mar. Biol. 118, 167–176. doi: 10.1007/BF00699231
Dando P. R. (2010). “Biological communities at marine shallow-water vent and seep sites,” in The vent and seep biota. Ed. Kiel S. (Springer, Dordrecht), 333–378.
Dando P. R., Austen M. C., Burke R. A. Jr., Kendall M. A., Kennicutt M. C., Judd A. G., et al. (1991). Ecology of a North Sea pockmark with an active methane seep. Mar. Ecol. Prog. Ser. 70 (1), 49–63. doi: 10.3354/meps070049
Dando P. R., Bussmann I., Niven S. J., O’hara S. C. M., Schmaljohann R., Taylor L. J. (1994). A methane seep area in the Skagerrak, the habitat of the pogonophore Siboglinum poseidoni and the bivalve mollusc Thyasira sarsi. Mar. Ecol. Prog. Ser. 107 (1/2), 157–167. doi: 10.3354/meps107157
Deubel H., Engel M., Fetzer I., Gagaev S., Hirche H. J., Klages M., et al. (2003). “The southern Kara Sea ecosystem: Phyto-plankton, zooplankton and benthos communities influenced by river run-off,” in Siberian River Runoff in the Kara Sea: characterization, quantification variability and envi-ronmental significance. Proceedings in Marine Science, vol. 6 . Ed. Ruediger S.(Elsevier, Amsterdam: Elsevier Science), 237–275.
Drits A. V., Pasternak A. F., Arashkevich E. G., Sukhanova I. N., Sergeeva V. M., Flint M. V. (2021). Influence of riverine discharge and timing of ice retreat on particle sedimentation patterns on the Laptev Sea shelf. J. Geophys. Res.: Oceans. 126, e2021JC017462. doi: 10.1029/2021JC017462
Eleftheriou A., McIntyre A. (2005). Methods for the study of marine benthos (Oxford, UK: Blackwell Science), 418. doi: 10.1002/9780470995129
Ezhova E., Dorokhov D., Sivkov V., Zhamoida V., Ryabchuk D., Kocheshkova O. (2012). “Benthic habitats and benthic communities in Southeastern Baltic Sea, Russian sector,” in Seafloor Geomorphology as Benthic Habitat (Elsevier), 613–621. doi: 10.1016/B978-0-12-385140-6.00043-8
Gebruk A. V., Krylova E. M., Lein A. Y., Vinogradov G. M., Anderson E., Pimenov N. V., et al. (2003). Methane seep community of the Håkon Mosby mud volcano (the Norwegian Sea): composition and trophic aspects. Sarsia 88, 394–403. doi: 1080/00364820310003190
Hastie T. J., Tibshirani R. J. (1990). Generalized Additive Models (New York, USA: Chapman & Hall/CRC), ISBN: ISBN 978-0-412-34390-2.
Jørgensen L. L., Pearson T. H., Anisimova N. A., Gulliksen B., Dahle S., Denisenko S. G., et al. (1999). Environmental influences on benthic fauna associations of the Kara Sea (Arctic Russia). Polar Biol. 22, 395–416. doi: 10.1007/s003000050435
Karaseva N., Gantsevich M., Obzhirov A., Shakirov R., Starovoitov A., Smirnov R., et al. (2020). Correlation of the siboglinid (Annelida: Siboglinidae) distribution to higher concentrations of hydrocarbons in the Sea of Okhotsk. Mar. pollut. Bull. 158, 111448. doi: 10.1016/j.marpolbul.2020.111448
Karaseva N. P., Rimskaya-Korsakova N. N., Ekimova I. A., Gantsevich M. M., Kokarev V. N., Kremnyov S. V., et al. (2021a). A new genus of frenulates (Annelida: Siboglinidae) from shallow waters of the Yenisey River estuary, Kara Sea. Invertebrate Systematics 35, 857–875. doi: 10.1071/IS20075
Karaseva N. P., Rimskaya-Korsakova N. N., Ekimova I. A., Kokarev V. N., Simakov M. I., Gantsevich M. M. (2021b). The first discovery of pogonophores (Annelida, Siboglinidae) in the East Siberian Sea coincides with the areas of methane seeps. Doklady Biol. Sci. 501, 517–521. doi: 10.1134/S0012496621060016
Kiel S., Dando P. R. (2009). Chaetopterid tubes from vent and seep sites: Implications for fossil record and evolutionary history of vent and seep annelids. Acta Palaeontologica Polonica 54, 443–448. doi: 10.4202/app.2009.0022
Kokarev V. N., Vedenin A. A., Basin A. B., Azovsky A. I. (2017). Taxonomic and functional patterns of macrobenthic communities on a high-Arctic shelf: a case study from the Laptev Sea. J. Sea Res. 129, 61–69. doi: 10.1016/j.seares.2017.08.011
Kokarev V. N., Vedenin A. A., Polukhin A. A., Basin A. B. (2021). Taxonomic and functional patterns of macrobenthic communities on a high Arctic shelf: A case study from the East Siberian Sea. J. Sea Res. 174, 102078. doi: 10.1016/j.seares.2021.102078
Kokarev V., Zalota A. K., Zuev A., Tiunov A., Kuznetsov P., Konovalova O., et al. (2023). Opportunistic consumption of marine pelagic, terrestrial, and chemosynthetic organic matter by macrofauna on the Arctic shelf: a stable isotope approach. PeerJ 11, e15595. doi: 10.7717/peerj.15595
Leifer I., Chernykh D., Shakhova N., Semiletov I. (2017). Sonar gas flux estimation by bubble insonification: application to methane bubble fluxes from the east Siberian arctic shelf seabed. Cryosphere 11, 1333–1350. doi: 10.5194/tc-11-1333-2017
Lein A. Y., Pavlova G. A., Bogdanov Y. A., Sagalevich A. M., Pimenov N. V., Savvichev A. S., et al. (2000). Methane as a source of organic matter and carbon dioxide of carbonates at a cold seep in the Norway Sea. Geochem. Int. 38, 232–245.
Levin L. A. (2005). “Ecology of cold seep sediments: interactions of fauna with flow, chemistry and microbes,” in Oceanography and marine biology (CRC Press, Boca Raton), 11–56.
Levin L. A., James D. W., Martin C. M., Rathburn A. E., Harris L. H., Michener R. H. (2000). Do methane seeps support distinct macrofaunal assemblages? Observations on community structure and nutrition from the northern California slope and shelf. Mar. Ecol. Prog. Ser. 208, 21–39. doi: 10.3354/meps208021
Lobkovsky L. I., Baranov A. A., Ramazanov M. M., Vladimirova I. S., Gabsatarov Y. V., Semiletov I. P., et al. (2022). Trigger mechanisms of gas hydrate decomposition, methane emissions, and glacier breakups in polar regions as a result of tectonic wave deformation. Geosciences 12, 372. doi: 10.3390/geosciences12100372
Lockwood E. (1985). An Introduction to linear regression and correlation, 2nd edition. Ed. Edwards A. L., 206. doi: 10.1017/S0025557200105583
MacDonald I. R., Bohrmann G., Escobar E., Abegg F., Blanchon P., Blinova V., et al. (2004). Asphalt volcanism and chemosynthetic life in the Campeche Knolls, Gulf of Mexico. Science 304, 999–1002. doi: 10.1126/science.1097154
McCune B., Grace J. B., Urban D. L. (2002). Analysis of ecological communities (vol. Mjm software design (Oregon: Gleneden Beach), 28.
Nishi E., Rouse G. W. (2007). A new species of Phyllochaetopterus (Chaetopteridae: Annelida) from near hydrothermal vents in the Lau Basin, western Pacific Ocean. Zootaxa 1621, 55–64. doi: 10.11646/zootaxa.1621.1
Nishi E., Rouse G. W. (2014). First whale fall chaetopterid; a gigantic new species of Phyllochaetopterus (Chaetopteridae: Annelida) from the deep sea off California. Proc. Biol. Soc. Washington 126, 287–298. doi: 10.2988/0006-324X-126.4.287
Paull C. K., Dallimore S. R., Caress D. W., Gwiazda R., Melling H., Riedel M., et al. (2015). Active mud volcanoes on the continental slope of the Canadian Beaufort Sea. Geochem. Geophysics Geosystems 16, 3160–3181. doi: 10.1002/2015GC005928
Petryashev V. V., Novozhilov A. V. (2004). Influence of hydrological regime on distribution of macrobenthos in the Laptev Sea, Issled. Fauny Morei. 54 (62), 74–85.
Pimenov N., Savvichev A., Rusanov I., Lein A., Egorov A., Gebruk A., et al. (1999). Microbial processes of carbon cycle as the base of food chain of Håkon Mosby Mud Volcano benthic community. Geo-Marine Lett. 19, 89–96. doi: 10.1007/s003670050097
Pimenov N. V., Ul’yanova M. O., Kanapatskii T. A., Sivkov V. V., Ivanov M. V. (2008). Microbiological and biogeochemical processes in a pockmark of the Gdansk Depression, Baltic Sea. Microbiology 77, 579–586. doi: 10.1134/S0026261708050111
Piraino S., Fanelli G., Boero F. (1999). Variability of species’ roles in marine communities: change of paradigms for conservation priorities. Mar. Biol. 140, 1067–1074. doi: 10.1007/s00227-001-0769-2
Powell E. N., Bright T. J., Brooks J. M. (1986). The effect of sulfide and an increased food supply on the meiofauna and macrofauna at the East Flower Garden brine seep. Helgoländer Meeresuntersuchungen 40, 57–82. doi: 10.1007/BF01987289
Quiroga E., Sellanes J. (2009). Growth and size-structure of Stegophiura sp.(Echinodermata: Ophiuroidea) on the continental slope off central Chile: a comparison between cold seep and non-seep sites. J. Mar. Biol. Assoc. United Kingdom 89, 421–428. doi: 10.1017/S0025315408002786
Sahling H., Wallmann K., Dählmann A., Schmaljohann R., Petersen S. (2005). The physicochemical habitat of Sclerolinum sp. at Hook Ridge hydrothermal vent, Bransfield Strait, Antarctica. Limnol. Oceanogr. 50, 598–606. doi: 10.4319/lo.2005.50.2.0598
Sapart C. J., Shakhova N., Semiletov I., Jansen J., Szidat S., Kosmach D., et al. (2017). The origin of methane in the East Siberian Arctic Shelf unraveled with triple isotope analysis. Biogeosciences 14, 2283–2292. doi: 10.5194/bg-14-2283-2017
Schlitzer R. (2020). Ocean Data View. Available online at: https://odv.awi.de/.
Semiletov I. P., Gustafsson O. (2009). East Siberian shelf study alleviates scarcity of observations. Eos Transactions AGU 90, 145–146. doi: 10.1029/2009EO170001
Sen A., Chitkara C., Hong W. L., Lepland A., Cochrane S., Di Primio R., et al. (2019). Image based quantitative comparisons indicate heightened megabenthos diversity and abundance at a site of weak hydrocarbon seepage in the southwestern Barents Sea. PeerJ 7, e7398. doi: 10.7717/peerj.7398
Sen A., Didriksen A., Hourdez S., Svenning M. M., Rasmussen T. L. (2020). Frenulate siboglinids at high Arctic methane seeps and insight into high latitude frenulate distribution. Ecol. Evol. 10, 1339–1351. doi: 10.1002/ece3.5988
Shakhova N., Semiletov I., Salyuk A., Joussupov V., Kosmach D., Gustafsson O. (2010). Extensive methane venting to the atmosphere from sediments of the East Siberian Arctic Shelf. Science 327, 1246–1250. doi: 10.1126/science.1182221
Shakhova N., Semiletov I., Leifer I., Salyuk A., Rekant P., Kosmach D. (2010a). Geochemical and geophysical evidence of methane release over the East Siberian Arctic Shelf. J. Geophys. Res.: Oceans 115, 14. doi: 10.1029/2009JC005602
Shakhova N., Semiletov I., Leifer I., Sergienko V., Salyuk A., Kosmach D., et al. (2014). Ebullition and storm-induced methane release from the East Siberian Arctic Shelf. Nat. Geosci. 7, 64–70. doi: 10.1038/ngeo2007
Shakhova N., Semiletov I., Sergienko V., Lobkovsky L., Yusupov V., Salyuk A., et al. (2015). The East Siberian Arctic Shelf: towards further assessment of permafrost-related methane fluxes and role of sea ice. Philos. Trans. R. Soc. A 373, 20140451. doi: 10.1098/rsta.2014.0451
Shakhova N., Semiletov I., Gustafsson O., Sergienko V., Lobkovsky L., Dudarev O., et al. (2017). Current rates and mechanisms of subsea permafrost degradation in the East Siberian Arctic Shelf. Nat. Commun. 8, 15872. doi: 10.1038/ncomms15872
Shakhova N., Semiletov I., Chuvilin E. (2019). Understanding the permafrost–hydrate system and associated methane releases in the east Siberian arctic shelf. Geosci. (Switzerland). 9 (6), 251. doi: 10.3390/geosciences9060251
Sibuet M., Juniper K. S., Pautot G. (1988). Cold-seep benthic communities in the Japan subduction zones: geological control of community development. J. Mar. Res. 46, 333–348. doi: 10.1357/002224088785113595
Sirenko B. I. (1998). Marine fauna of the Arctic (after the expeditions of the Zoological Institute, Russian Academy of Sciences). Russian J. Mar. Biol. 24, 353–364.
Sirenko B. I., Denisenko S. G. (2010). Fauna of the East-Siberian Sea, distribution patterns and structure of bottom communities. Explorations of the fauna of the Seas Vol. 66 (Saint-Petersburg: Zoological Institute RAS), 248.
Sirenko B., Denisenko S., Deubel H., Rachor E. (2004). Deep water communities of the Laptev Sea and adjacent parts of the Arctic Ocean. Fauna and the ecosystems of the Laptev Sea and adjacent deep waters of the Arctic Ocean. Explorations of the fauna of sea Vol. 54 (St. Petersburg: Zoological Institute of Russian Academy of Sciences), 28–73.
Smirnov R. V. (2000). Two new species of Pogonophora from the arctic mud volcano off northwestern Norway. Sarsia 85, 141–150. doi: 10.1080/00364827.2000.10414563
Smirnov R. V., Zaitseva O. V., Vedenin A. A. (2020). A remarkable pogonophoran from a desalted shallow near the mouth of the Yenisey River in the Kara Sea, with the description of a new species of the genus Galathealinum (Annelida: Pogonophora: Frenulata). Zoosystematica Rossica 29, 138–154. doi: 10.31610/zsr/
Steinbach J., Holmstrand H., Scherbakova K., Kosmach D., Bruchrt V., Shakhova N., et al. (2021). Source apportionment of methane escaping the subsea permafrost system in the outer Eurasian arctic shelf. Proc. Natl. Acad. Sci. (PNAS) 118, e2019672118. doi: 10.1073/pnas.2019672118
Tarasov V. G., Gebruk A. V., Mironov A. N., Moskalev L. I. (2005). Deep-sea and shallow-water hydrothermal vent communities: two different phenomena? Chem. Geol. 224, 5–39. doi: 10.1016/j.chemgeo.2005.07.021
Vedenin A. A., Galkin S. V., Gebruk A. V. (2021a). List of macrobenthic species: Data from the Siberian seas and the adjacent area of the deep-sea central arctic. Data Brief 36, 107115. doi: 10.1016/j.dib.2021.107115
Vedenin A. A., Galkin S. V., Kozlovskiy V. V. (2015). Macrobenthos of the Ob Bay and adjacent Kara Sea shelf. Polar Biol. 38, 829–844. doi: 10.1007/s00300-014-1642-3
Vedenin A., Galkin S., Mironov A. N., Gebruk A. (2021b). Vertical zonation of the Siberian Arctic benthos: bathymetric boundaries from coastal shoals to deep-sea Central Arctic. PeerJ 9, e11640. doi: 10.7717/peerj.11640
Vedenin A., Gusky M., Gebruk A., Kremenetskaia A., Rybakova E., Boetius A. (2018). Spatial distribution of benthic macrofauna in the Central Arctic Ocean. PloS One 13, e0200121. doi: 10.1371/journal.pone.0200121
Vedenin A. A., Kokarev V. N., Chikina M. V., Basin A. B., Galkin S. V., Gebruk A. V. (2020). Fauna associated with shallow-water methane seeps in the Laptev Sea. PeerJ 8, e9018. doi: 10.7717/peerj.9018
Wassmann P. F., Slagstad D., Ellingsen I. (2019). Advection of mesozooplankton into the northern Svalbard shelf region. Front. Mar. Sci. 6. doi: 10.3389/fmars.2019.00458
Weiher E., Keddy P. A. (1999). Relative abundance and evenness patterns along diversity and biomass gradients. Oikos 87, 355–361. doi: 10.2307/3546751
Wiesenburg D. A., Guinasso N. L. (1979). Equilibrium solubility of methane, carbon monoxide, and hydrogen in water and sea water. J. Chem. Eng. Data 24, 356–360. doi: 10.1021/je60083a006
Yamamoto S., Alcauskas J. B., Crozier T. E. (1976). Solubility of methane in Distilled water and Seawater. J. Chem. Eng. Data 21, 78–80. doi: 10.1021/je60068a029
Keywords: benthic communities, methane seeps, sea shelf, siboglinids, Oligobrachia, benthic diversity, Laptev Sea, East Siberian Sea
Citation: Konovalova O, Rimskaya-Korsakova N, Kuznetsov P, Osadchiev A, Fedyaeva M, Moiseeva I, Purgina D, Kosmach D and Semiletov I (2024) Benthic communities under methane gradient in the Laptev and East Siberian seas. Front. Ecol. Evol. 12:1406680. doi: 10.3389/fevo.2024.1406680
Received: 25 March 2024; Accepted: 28 June 2024;
Published: 23 July 2024.
Edited by:
Robert Klinger, Belize Foundation for Research and Environmental Education, BelizeReviewed by:
Chih-Lin Wei, National Taiwan University, TaiwanMichael Vecchione, National Oceanic and Atmospheric Administration (NOAA), United States
Copyright © 2024 Konovalova, Rimskaya-Korsakova, Kuznetsov, Osadchiev, Fedyaeva, Moiseeva, Purgina, Kosmach and Semiletov. This is an open-access article distributed under the terms of the Creative Commons Attribution License (CC BY). The use, distribution or reproduction in other forums is permitted, provided the original author(s) and the copyright owner(s) are credited and that the original publication in this journal is cited, in accordance with accepted academic practice. No use, distribution or reproduction is permitted which does not comply with these terms.
*Correspondence: Olga Konovalova, oljakonovalova@gmail.com
†ORCID: Olga Konovalova, orcid.org/0000-0002-9023-7938
Nadezhda Rimskaya-Korsakova, orcid.org/0000-0001-9576-2435
Petr Kuznetsov, orcid.org/0000-0001-7214-1732
Alexander Osadchiev, orcid.org/0000-0002-6659-0934
Maria Fedyaeva, orcid.org/0000-0002-3153-9450
Iuliia Moiseeva, orcid.org/0000-0002-8166-1040
Daria Purgina, orcid.org/0000-0001-7169-8264
Denis Kosmach, orcid.org/0000-0002-2276-324X
Igor Semiletov, orcid.org/0000-0003-1741-6734