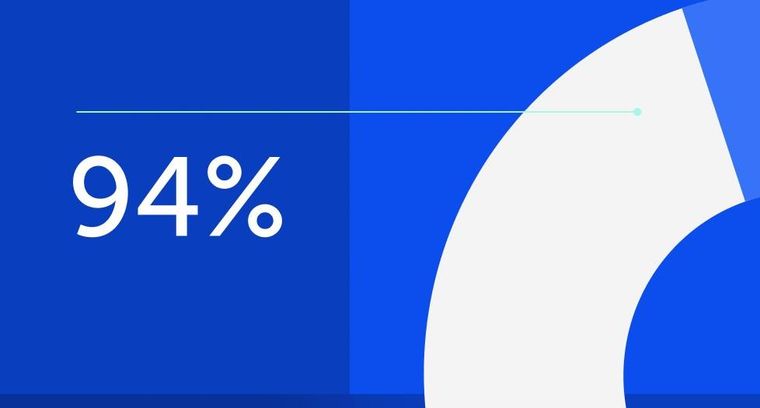
94% of researchers rate our articles as excellent or good
Learn more about the work of our research integrity team to safeguard the quality of each article we publish.
Find out more
ORIGINAL RESEARCH article
Front. Ecol. Evol., 14 May 2024
Sec. Conservation and Restoration Ecology
Volume 12 - 2024 | https://doi.org/10.3389/fevo.2024.1403602
This article is part of the Research TopicOpportunities and Challenges for Wild Bee ConservationView all 13 articles
Although the fire-maintained pine savannas of the southeastern U.S. Coastal Plain are recognized for their plant diversity, pollinators associated with these ecosystems remain comparatively understudied. Here we present the results from a season-long effort to record bee-flower interactions at a single site in Florida. We collected 93 bee species (out of an estimated 117) from 79 flower species, with a total of 446 unique interactions. Bee richness and the number of interactions exhibited a bimodal pattern, dipping in mid-summer before an estimated peak in October. The most important floral resources changed throughout the season as did the composition of bees, with the spring and fall periods being particularly distinct. We found that pollen specialists (that collect pollen from a single family of plants) and pollen generalists accounted for a similar proportion of bee species over the entire season. However, pollen generalists outnumbered pollen specialists in the spring and summer before reversing in the fall. Pollen specialists visited significantly fewer plant species and families than pollen generalists and many were collected exclusively from their host family. This was particularly the case for aster specialists active only during the fall. We estimate that between 18.3-25.8% of the local bee fauna depends directly on the overstory trees for nesting habitat including dead wood and resin. Two management recommendations can be made based on these results. First, because fall is the period of peak floral abundance and bee richness, including many late-season aster specialists, it is probably the least favorable time for prescribed fire. Second, considering that a significant proportion of native bees depend on dead wood for nesting, it is important to retain standing dead trees and fallen wood whenever possible.
The pine savannas of the southeastern U.S. support an outstanding diversity of endemic species. These ecosystems are highly fire-adapted, requiring a return interval of about two years to maintain pine dominance, open stand conditions, and diverse understory vegetation. Pine savanna ecosystems, dominated by longleaf pine (Pinus palustris Mill.) with smaller components of shortleaf pine (P. echinata Mill.), slash pine (P. elliottii Engelm.), and other fire-tolerant tree species, came to dominate the southeastern Coastal Plain 7500-5000 ybp in response to a warming climate, frequent lightning ignitions from convective thunderstorms, and anthropogenic burning (Van Lear et al., 2005). Whereas pine savanna ecosystems covered 370,000 km2 within this region prior to colonization by western European countries, they currently occupy a small fraction of their historical range (Frost, 2006) and many remain degraded from a history of agriculture and other soil disturbance, fire exclusion, logging, or invasion by exotic or off-site species. Ongoing efforts to preserve and restore native pine savannas are critical for conserving such iconic and vulnerable species as the red cockaded woodpecker, gopher tortoise, venus flytrap, and many others.
Southeastern pine savannas are recognized for their understory plant diversity which can reach up to 40 species per m2 and include many endemic taxa (Peet and Allard, 1993; Walker, 1993). Roughly three quarters of plant species in these systems are pollinated by insects (Folkerts et al., 1993). Pollinator communities of Coastal Plain savannas are distinct from those of other southeastern ecoregions (Ulyshen et al., in press) and have been the focus of numerous studies, ranging from the creation of species checklists (Bartholomew et al., 2006), efforts to better understand the life histories of focal species, comparisons among land use histories (Ulyshen et al., 2020; Dixon et al., 2022), and investigations into the effects of management activities (e.g., prescribed fire, thinning) (Breland, 2015; Moylett et al., 2019; Odanaka et al., 2020; Ulyshen et al., 2021, 2022). However, even some of the most basic questions about these communities remain unanswered. For example, although several studies have recorded pollinators visiting particular species of flowers (Deyrup and Menges, 1997; Hamon et al., 2018), efforts to document entire pollination networks are lacking. Similarly, the specific resource requirements of species comprising pollinator assemblages remain largely unestablished. Such basic descriptive information is of critical importance to fully understanding the nature of these communities and how best to conserve them.
Two topics concerning pollinator resource requirements are of particular relevance to managers. The first involves the diet breadth of bees. While Folkerts et al. (1993) suggested most bees associated with the longleaf pine ecosystem visit a wide range of floral hosts for either nectar or pollen (termed polytropic), the proportion of bees that specifically collect pollen from many vs. few hosts (polylectic vs. oligolectic, see Robertson, 1925) remains unknown. Observations of floral visitation alone may overestimate polylecty as some bees are known to visit more species for nectar than for pollen (Pekkarinen, 1997). Many bees are adapted to the pollen of particular plant lineages and cannot develop on pollen from unrelated plants (Praz et al., 2008). Efforts to estimate pollen specialization are challenged by the fact that sufficient data to make such designations are available for less than 5% of bee species globally (Wood et al., 2023). However, the North American fauna is better understood and predictive models that take into account flower visitation, occurrence, and phylogenetic data show promise (Smith et al., 2024). Because pollen specialists are inherently more sensitive to any alteration in resource availability, efforts to identify such species, and to understand which plant species they require and at what time of year, can be beneficial to managers. For example, pollinator species may be more sensitive to prescribed fire at certain times of year if it results in a phenological mismatch between when they are active and when their preferred floral resource is available.
The second topic of particular importance to mangers concerns utilization of tree-derived resources by bees. A large fraction of bees found in broadleaf forests are likely forest dependent (Smith et al., 2021), meaning they require a resource restricted to forests at some point during their life cycle. Such resources can include the nectar or pollen produced by broadleaf trees or dead wood used by many species for nesting. There is growing evidence that eastern deciduous forests of the U.S. provide important floral resources to bees in the canopy (Urban-Mead et al., 2021, 2023), and recent work from southeastern forests suggests that forest bee diversity increases as the diversity of flowering trees increases (Traylor et al., 2024). By contrast, forest bee diversity within the Piedmont has been shown to decrease as the amount of pine in the surrounding landscape or as a proportion of local basal area increases (Traylor et al., 2024; Ulyshen et al., in press). Such patterns raise questions about the value of pine trees to bee assemblages. Although pines probably do not provide useful floral resources to these insects (Pernal and Currie, 2000), they do provide nesting resources in the form of dead wood and resin. However, no previous effort has been made to estimate what proportion of the bee fauna in pine savannas depend on tree-derived nesting resources.
Here we characterize the bee community and pollination network (i.e., bee-flower interactions) based on direct sampling from flowers throughout the growing season in a native pine savanna in Florida. We aim to 1) identify which plant species support the greatest diversity of bees and how this varies throughout the year, 2) determine what proportion of native bee species are pollen specialists vs. generalists and how consistent observed floral visits are with pollen specialization, 3) document seasonal changes in pollinator networks, including changes in bee species richness, the number of interactions between bees and flowers, and the relative species richness of pollen specialists and generalists, and 4) estimate what proportion of bee species requires tree-derived resources for nesting.
The study area and sampling methods used in this study are described in Ulyshen et al. (2023). Briefly, we worked in an area of pine savanna measuring less than half a square kilometer on Tall Timbers Research Station in Leon County, Florida (Supplementary Figure S1). The site has no known history of plowing or other agricultural disturbance. The site has high plant species richness, with an average of 75 species per 100 m2 (KR and CD unpublished data). The open savanna-like conditions have been maintained by biennial prescribed fire since 1990 when the research station first acquired the property. Although the overstory trees consisted primarily of mature shortleaf pine (Pinus echinata), bee sampling mostly took place within eight 0.4 ha blocks where longleaf pine was planted in the 1990s and is mixed with shortleaf pine in the canopy. In 2019 each block was subdivided into four 0.1 ha square plots that had been randomly assigned to one of four season of fire treatments: winter (January), spring (March-April), summer (June), or fall (September-October). Although testing the effects of season of fire on bee communities was the original purpose of our sampling, the resulting dataset offers a special opportunity to study the pollinator network in a southeastern U.S. pine savanna.
As detailed in Ulyshen et al. (2023), two collectors followed a standardized protocol to net bees off flowers once a month from February to November 2022. During this period, prescribed fires were applied to the 0.1 ha plots according to the schedule described above, with winter plots being burned about one month before the study began. Nearly all plants in the community are perennial and rapidly resprout and often flower soon after burning in late winter through summer, so well-developed vegetation was always present in at least three of the four plots within each block. Only specimens judged to be actively foraging for pollen or nectar (based on observed behavior) were collected, and flowers were identified on site or from photographs and field notes. Sampling took place only during favorable weather (sunny or partly cloudy skies) between the hours of 9:30 am to 5:30 pm. Each collector spent 20 min (Feb, Mar, Aug, Oct, and Nov) or 25 min (Apr, May, Jun, Sep) sampling bees in each plot. In July, due to stormy weather, the plots within half the blocks were each sampled for a total of 40 min while the others were sampled for only 30 min. In November, only one collector visited the plots. Finally, some additional opportunistic sampling took place near the plots and we include those data in the current network analysis for completeness. In total, the data presented here resulted from more than 220 person-hours. Sampling took place on the following dates: February 22–24, March 20–21, April 19–29, May 16–18, June 13–15, July 13–14, August 6–10, September 12–15, October 19–21, and November 7–8. Bees were identified to species using published (Mitchell, 1960, 1962; Gibbs, 2011) and online (discoverlife.org) keys as well as an established reference collection. Voucher specimens are deposited in the first author’s research collection.
Bee species were classified as pollen specialists (i.e., species that consume pollen from only a single family of plants), generalists, or parasites, based on information from the literature when available. For other species, diet breadth was predicted using a random forest model based on trait, phylogenetic, and visitation data compiled for 682 bee species native to the United States (Smith et al., 2024).
All analyses were performed in R (Team, 2022). We pooled data by month to examine seasonal changes in bee-flower networks, including the number of interactions, the number of bee species, and the relative number of pollen specialist and generalist bees. Because our goal was to record the pollination network as completely as possible, data from the opportunistic sampling outside the main study plots were included in this analysis. To adjust for differences in sampling effort among months, we calculated the Chao1 richness estimator using the rareNMtests package (version 1.2) (Cayuela and Gotelli, 2014) for each month separately. We used the same method to estimate the total number of bee species present at our study site after combining data from all months. Chao1 adjusts the observed number of species based on the number of taxa represented by just one or two specimens. We used the bipartite package (version 2.19) to create figures showing interactions between the different bees and flowers for each month separately.
To investigate how bee community composition changed throughout the season, we performed non-metric multidimensional scaling (NMDS) on a Bray-Curtis distance matrix using the vegan package (version 2.6.4) (Oksanen et al., 2007). We only included the data collected from the season of fire plots in this analysis. We grouped data by month pairs (Feb-Mar, Apr-May, Jun-Jul, Aug-Sep, Oct-Nov), and bee abundance data were Hellinger-transformed (i.e., relativized by species maximum) prior to analysis. Then, to determine if any taxa were strongly associated with one or more of the month pairs, we performed indicator species analysis using the multipatt function in the package indicspecies (version 1.7.14) (De Caceres et al., 2016). This test produces values ranging from 0 (no association) to 1 (complete association).
Finally, we used the Wilcoxon ranked sum test to compare the number of hosts visited by pollen specialists and generalists. This test was performed separately for the number of flower species and families visited.
We collected a total of 93 bee species (Supplementary Table S1) from 79 species of flowers (Supplementary Table S2), with a total of 446 unique bee-flower interactions. Based on Chao1, the total bee richness at our study site was estimated to be 117 species with a 95% confidence range of 101-165. We observed a distinct mid-summer dip in the number of bee-flower interactions as well as bee richness, with peaks occurring in April and October (Figure 1). Chao1 estimates of bee richness are generally in agreement with a mid-summer dip and with bee richness reaching a low point in July (Figure 1). The most important floral resources, in terms of the number of visiting bee species, varied from month to month (Table 1, Figures 2–4). In February, for example, most of the collected bee species and individuals came from Gelsemium sempervirens (L.) J. St.-Hil. whereas Baptisia alba (L.) Vent. became the most important floral resource by March. Top species in April, May, and June were Rubus cuneifolius Pursh, Tephrosia virginiana (L.) Pers., and Callicarpa americana L., respectively. A variety of floral resources dominated later in the year, but, by October and November, members of Asteraceae (e.g., Helianthus angustifolius L., Chrysopsis mariana (L.) Elliot, Pityopsis aspera (Shuttlw. ex Small) Small) became the most visited flowers. Although the bee species observed visiting flowers of the most plant species also varied throughout the year (Table 1, Figures 2-4), several were commonly among the highest ranked species. For example, Ceratina sp. was either the first or second most interactive bee taxon from February through June. Similarly, Lasioglossum reticulatum (Robertson) was one of the top three most interactive species for five of the months.
Figure 1 Total number of observed bee-flower interactions and the richness of all bees, pollen generalists, and pollen specialists by month. Chao1 estimates of bee richness are also shown. The lower graph shows changes in relative sampling effort over the same period of time.
Table 1 Top three most interactive bee and plant species by month with the total number of partner species (and total number of observations).
Figure 2 Observed pollination networks for February, March, and April 2022. See Supplementary Tables S1, S2 for species abbreviations.
Figure 3 Observed pollination networks for May, June, and July 2022. See Supplementary Tables S1, S2 for species abbreviations.
Figure 4 Observed pollination networks for August, September, October, and November 2022. See Supplementary Tables S1, S2 for species abbreviations.
Our NMDS ordination (stress=0.18) revealed distinct seasonal changes in bee composition, with the early spring (Feb-Mar) and fall (Oct-Nov) faunas being particularly distinct (Figure 5). Based on indicator species analysis, 37 bee species were strongly associated with one or more of the month pairs (Supplementary Table S4). Three species were associated with the earliest months (Feb-Mar) (Eucera dubitata (Cresson), Habropoda laboriosa (Fabricius), and Osmia sandhouseae Mitchell), whereas eleven were associated with the fall months (Oct-Nov). These were Agapostemon splendens (Lepeletier), Andrena accepta Viereck, Andrena fulvipennis (Smith), Bombus bimaculatus Cresson, Melissodes boltoniae Robertson, M. dentiventris Smith, M. druriellus (Kirby), Paranthidium jugatorium (Say), Pseudopanurgus labrosiformis Cresson, P. solidaginus Robertson, and P. rugosus Robertson (Supplementary Table S4). All but three of these fall-associated species are known to be pollen specialists of Asteraceae, and the others were either collected exclusively (B. bimaculatus and P. jugatorium) or mostly (A. splendens) from this family (Supplementary Table S3).
Based on the literature and model predictions, 38 and 37 of the species collected in this study are pollen specialists and generalists, respectively. Of these classifications, 23 are model predictions. Another six species are parasitic and the remaining 12 species remain unclassified. Among the 25 species with known pollen specialization, 80% are specialists of Asteraceae (Supplementary Table S1). On average, species classified as generalists and specialists visited 6.2 ± 1.3 and 3.0 ± 0.6 species of flowers, respectively, a significant difference based on the Wilcoxon ranked sum test (W=920, p=0.02). The numbers of plant families visited by generalists and specialists were 3.7 ± 0.6 and 1.7 ± 0.2, respectively, also a significant difference (W=1025.5, p<0.001). Of the 50 most frequently captured bee species (represented by at least five specimens), twelve are known to be pollen specialists. Ten of these were captured exclusively from their known host family (Supplementary Table S3). Only one of these specialist species, Svastra atripes (Cresson), was captured more often on non-host families. We caught a greater number of pollen generalist species than specialists throughout the spring and much of the summer, but more specialist species were collected than generalists beginning in October (Figure 1).
Nearly three quarters of the sampled bee species nest either within the soil or at the ground surface (Supplementary Table S1). It is not possible to determine exactly how many species depend on trees for nesting, but at least 15 species (including a species of Heriades, ten species of Megachile, two species of Osmia, and two species of Xylocopa), and perhaps as many as 22, nest in dead wood. Additionally, two species of Anthidiellum are known to build nests out of resin collected from trees. Thus, between 18.3-25.8% of the local bee species collected in this study can be considered dependent on overstory trees for nesting.
To our knowledge, this study represents the first effort to record species-level interactions between bees and flowers over an entire season in a southeastern pine savanna. We recorded 93 bee species (out of an estimated total of 117 species) within a sampling area measuring no more than half a square kilometer, showing that such savannas can support a high diversity of bees. Our observations likely represent only a small fraction of the bee-flower interactions that occur at our small study site (Chacoff et al., 2012), and network interactions are likely to vary considerably from year to year. For example, researchers previously reported that even species that appear to be restricted to a single host one year can function more like generalists over larger periods of time (Petanidou et al., 2008). Such findings suggest that many bees respond opportunistically to available resources and, as a consequence, pollinator networks may be less sensitive to disturbances than once thought. Given such variability, the networks presented here should be viewed only as snapshots in time from a single location. However, several important insights into southeastern pine bee communities can be gleaned from these observations and are discussed below.
The seasonality of southeastern pine savanna bee communities appears to be more complicated than previously understood, with the number of bee species and network interactions exhibiting peaks in both the spring and fall, with a dip in mid-summer. This bimodal pattern contrasts with the single June peak reported across multiple Coastal Plain forests and savannas by Ulyshen et al. (in press). There are several possible explanations for this discrepancy. First, sampling effort was not consistent across months in the current study and was somewhat lower in July and August than in the months before or after. This may have contributed to the observed mid-summer dip in the number of bee species and interactions (Figure 1). However, it should be noted that this dip was corroborated by our Chao1 richness estimates, which are less sensitive to differences in sampling effort. Another possibility is that our sampling under-represented bee diversity in mid-summer when high temperatures may have acted as a filter on the foraging bee assemblage. Although we did notice a marked reduction in mid-day bee activity on the hottest days in July and August, the fact that the downward trend began in May during milder conditions indicates that weather conditions alone cannot explain these patterns. Differences in sampling method may also have resulted in dissimilar observed seasonality patterns between studies. For example, the study by Ulyshen et al. (in press) involved pan traps which are known to under-sample certain taxa (Cane et al., 2000) and to be less effective during periods of high floral abundance (Baum and Wallen, 2011). Finally, that previous study did not extend beyond September and thus missed the peak fall blooming period. Whatever the explanation, the fact that October had the highest Chao1 richness estimate of all months, and eleven bee species were strongly associated with October and November, shows that fall is a particularly important time of year for bees in southeastern U.S. pine savannas.
Our findings suggest that pollen specialists may make up a larger proportion of bee species in pine savannas than previously thought (Folkerts et al., 1993). Based on published information as well as model predictions, we found bee species at our study site to be about equally divided between pollen specialists and generalists, with pollen specialists accounting for 50.7% of classified species. Although pollen specialists are known to often visit non-host flowers for nectar, they visited flowers of significantly fewer plant species and families than pollen generalists in the current study. Moreover, 83.3% of the most frequently captured pollen specialists were captured exclusively from their known host family (Supplementary Table S3). Consistent with previous studies (Pelletier and Forrest, 2023), we found the relative number of pollen specialists to increase later in the year. Of the eleven species found to be strongly associated with October and November, eight were pollen specialists. All of these species are specialists of Asteraceae, which underscores the key role this family plays in shaping the fall bee community in southeastern U.S. pine savannas.
This study represents the first effort to quantify the importance of the pine overstory to savanna bee assemblages. Although the openness of pine savannas creates an almost grassland-like understory, and floral resources are almost entirely confined to this layer, it is clear from our results that the pine overstory is necessary for a large proportion of savanna bee species. We estimate that 18.3-25.8% of the bee species collected in this study depend on trees for nesting habitat (e.g., cavities in dead wood) or for resin used in nest construction. The upper end of this range is not much below the 32% of forest-associated bees reported from the deciduous forests of the northeastern U.S (Smith et al., 2021). Thus, even though pine trees do not provide floral resources beneficial to bees, they do provide many species with critical nesting resources. While beyond the scope of the current study, another potential benefit of the pine overstory to bees concerns the role pine needles play as fuel for the fires so important to the maintenance of savanna plant diversity (Kirkman et al., 2007). It is probable that broadleaf trees, when present, provide further benefit to bees in this system. For example, the flowers of many broadleaf tree genera (including Acer, Liriodendron, Quercus, etc.) are known to be visited by bees, and bee diversity has been shown to be positively correlated with flowering tree diversity (Traylor et al., 2024). It is likely that riparian forests and other corridors or patches of hardwood trees provide an additional benefit to bees within Coastal Plain landscapes.
The results from this study have important implications for managers. First, it is clear from our results that fall is a particularly important time of year for bees including many late-season Asteraceae specialists. Thus, in terms of impacts on pollinators, fall may be the least favorable time of year for implementing prescribed burns, as suggested by a previous analyses of these data (Ulyshen et al., 2023). Our results also highlight the value of pine trees to savanna bee assemblages. We estimate that as much as one quarter of bee species may depend on trees for nesting purposes. Because most of these nest in dead wood, ensuring an adequate availability of standing and downed woody debris will benefit this fauna.
The raw data supporting the conclusions of this article will be made available by the authors, without undue reservation.
The manuscript presents research on animals that do not require ethical approval for their study.
MU: Conceptualization, Data curation, Formal analysis, Investigation, Methodology, Writing – original draft, Writing –review & editing.KR: Conceptualization, Investigation, Writing – review & editing. SH: Investigation, Writing – review & editing. CD: Investigation, Writing – review & editing.
The author(s) declare financial support was received for the research, authorship, and/or publication of this article. This research was funded by the USDA Forest Service.
We are grateful to Colleen Smith for using her model to predict pollen specialization for species with unknown diet breadth.
The authors declare that the research was conducted in the absence of any commercial or financial relationships that could be construed as a potential conflict of interest.
All claims expressed in this article are solely those of the authors and do not necessarily represent those of their affiliated organizations, or those of the publisher, the editors and the reviewers. Any product that may be evaluated in this article, or claim that may be made by its manufacturer, is not guaranteed or endorsed by the publisher.
The findings and conclusions in this publication are those of the authors and should not be construed to represent any official USDA or U.S. Government determination or policy.
The Supplementary Material for this article can be found online at: https://www.frontiersin.org/articles/10.3389/fevo.2024.1403602/full#supplementary-material
Bartholomew C. S., Prowell D., Griswold T. (2006). An annotated checklist of bees (Hymenoptera: apoidea) in longleaf pine savannas of Southern Louisiana and Mississippi. J. Kansas Entomol. Soc 79, 184–198. doi: 10.2317/0410.27.1
Baum K. A., Wallen K. E. (2011). Potential bias in pan trapping as a function of floral abundance. J. Kansas Entomol. Soc 84, 155–159, 155. doi: 10.2317/JKES100629.1
Breland S. (2015). Bee assemblage and vegetation across a suite of restoration conditions in a fire-maintained longleaf pine savanna (Athens, Georgia: University of Georgia).
Cane J. H., Minckley R. L., Kervin L. J. (2000). Sampling bees (Hymenoptera: apiformes) for pollinator community studies: pitfalls of pan-trapping. J. Kansas Entomol. Soc 73, 225–231.
Cayuela L., Gotelli N. J. (2014). rareNMtests: Ecological and Biogeographical Null Model Tests for Comparing Rarefaction Curves. R Package v1 (Vienna: The R Foundation for Statistical Computing).
Chacoff N. P., Vázquez D. P., Lomáscolo S. B., Stevani E. L., Dorado J., Padrón B. (2012). Evaluating sampling completeness in a desert plant–pollinator network. J. Anim. Ecol. 81, 190–200. doi: 10.1111/j.1365-2656.2011.01883.x
Deyrup M., Menges E. S. (1997). Pollination ecology of the rare scrub mint Dicerandra frutescens (Lamiaceae). Florida Scientist 60, 143–157.
Dixon C. M., Robertson K. M., Ulyshen M. D., Sikes B. A. (2022). Pine savanna restoration on agricultural landscapes: The path back to native savanna ecosystem services. Sci. Total Environ. 818, 151715. doi: 10.1016/j.scitotenv.2021.151715
Folkerts G. W., Deyrup M. A., Sisson D. (1993). “Arthropods associated with xeric longleaf pine habitats in the southeastern United States: a brief overview,” in Proceedings of the Tall Timbers Fire Ecology Conference (Tall Timbers Research Station, Tallahassee, Florida Tallahassee, FL).
Frost C. (2006). “History and future of the longleaf pine ecosystem,” in The longleaf pine ecosystem: ecology, silviculture, and restoration. Eds. Jose S., Jokela E. J., Miller D. L. (Springer, New York), pgs 9–pgs48.
Gibbs J. (2011). Revision of the metallic Lasioglossum (Dialictus) of eastern North America (Hymenoptera: Halictidae: Halictini). Zootaxa 3073, 1–216. doi: 10.11646/zootaxa.3073.1
Hamon L. E., Youngsteadt E., Irwin R. E., Sorenson C. E. (2018). Pollination Ecology and morphology of venus flytrap in sites of varying time since last fire. Ann. Entomol. Soc Am. 112, 141–149. doi: 10.1093/aesa/say032
Kirkman L. K., Mitchell R. J., Melanie J. K., Pecot S. D., Kimberly L. C. (2007). The perpetual forest: using undesirable species to bridge restoration. J. Appl. Ecol. 44, 604–614. doi: 10.1111/j.1365-2664.2007.01310.x
Mitchell T. B. (1960). Bees of the Eastern United States Volume 1 (The North Carolina Agricultural Experiment Station).
Mitchell T. B. (1962). Bees of the eastern United States Volume 2 (The North Carolina Agriculture Experiment Station).
Moylett H., Youngsteadt E., Sorenson C. (2019). The impact of prescribed burning on native bee communities (Hymenoptera: Apoidea: Anthophila) in longleaf pine savannas in the North Carolina sandhills. Environ. Entomol. 49, 211–219. doi: 10.1093/ee/nvz156
Odanaka K., Gibbs J., Turley N. E., Isaacs R., Brudvig L. A. (2020). Canopy thinning, not agricultural history, determines early responses of wild bees to longleaf pine savanna restoration. Restor. Ecol. 28, 138–146. doi: 10.1111/rec.13043
Oksanen J., Kindt R., Legendre P., O’Hara B., Stevens M. H. H., Oksanen M. J. (2007). The vegan package. Community Ecol. Package 10, 719.
Peet R. K., Allard D. J. (1993). “Longleaf pine vegetation of the southern Atlantic and eastern Gulf Coast regions: a preliminary classification,” in Proceedings of the Tall Timbers Fire Ecology Conference. Ed. Hermann S. M. (Tallahassee, FL. Tall Timbers Research Station, Tallahassee, Florida.), 45–81.
Pekkarinen A. (1997). Oligolectic bee species in Northern Europe (Hymenoptera, Apoidea). Entomol. Fennica 8, 205–214. doi: 10.33338/ef.83945
Pelletier D., Forrest J. R. K. (2023). Pollen specialisation is associated with later phenology in Osmia bees (Hymenoptera: Megachilidae). Ecol. Entomol. 48, 164–173. doi: 10.1111/een.13211
Pernal S. F., Currie R. W. (2000). Pollen quality of fresh and 1-year-old single pollen diets for worker honey bees (Apis mellifera L.). Apidologie. 31, 387–409.
Petanidou T., Kallimanis A. S., Tzanopoulos J., Sgardelis S. P., Pantis J. D. (2008). Long-term observation of a pollination network: fluctuation in species and interactions, relative invariance of network structure and implications for estimates of specialization. Ecol. Lett. 11, 564–575. doi: 10.1111/j.1461-0248.2008.01170.x
Praz C. J., Müller A., Dorn S. (2008). Specialized bees fail to develop on non-host pollen: Do plants chemically protect their pollen? Ecology 89, 795–804. doi: 10.1890/07-0751.1
Smith C., Bachelder N., Russell A. L., Morales V., Mosher A. R., Seltmann K. C. (2024). Pollen specialist bee species are accurately predicted from visitation, occurrence, and phylogenetic data. preprint. doi: 10.21203/rs.3.rs-3851958/v1
Smith C., Harrison T., Gardner J., Winfree R. (2021). Forest-associated bee species persist amid forest loss and regrowth in eastern North America. Biol. Conserv. 260, 109202. doi: 10.1016/j.biocon.2021.109202
Team R. C. (2022). R: A language and environment for statistical computing (Vienna, Austria: R Foundation for Statistical Computing).
Traylor C. R., Ulyshen M. D., Bragg D. C., McHugh J. V. (2024). Forest bees need compositionally diverse canopies. Preprint. doi: 10.21203/rs.3.rs-4110135/v1
Ulyshen M., Adams C., Adams J., Adams S. B., Bland M., Bragg D. C., et al. (in press). Spatiotemporal patterns of forest pollinator diversity across the southeastern United States. Diversity Distrib.
Ulyshen M. D., Hiers J. K., Pokswinksi S. M., Fair C. (2022). Pyrodiversity promotes pollinator diversity in a fire-adapted landscape. Front. Ecol. Environ. 20, 78–83. doi: 10.1002/fee.2436
Ulyshen M. D., Pokswinski S., Hiers J. K. (2020). A comparison of bee communities between primary and mature secondary forests in the longleaf pine ecosystem. Sci. Rep. 10, 2916. doi: 10.1038/s41598-020-59878-4
Ulyshen M., Robertson K., Horn S., Dixon C. (2023). Effects of season of fire on bee-flower interaction diversity in a fire-maintained pine savanna. Ecol. Evol. 13, e10450. doi: 10.1002/ece3.10450
Ulyshen M. D., Wilson A. C., Ohlson G. C., Pokswinksi S. M., Hiers J. K. (2021). Frequent prescribed fires favour ground-nesting bees in southeastern U.S. forests. Insect Conserv. Diver. 14, 527–534. doi: 10.1111/icad.12484
Urban-Mead K. R., Muñiz P., Gillung J., Espinoza A., Fordyce R., van Dyke M., et al. (2021). Bees in the trees: Diverse spring fauna in temperate forest edge canopies. For. Ecol. Manage. 482, 118903. doi: 10.1016/j.foreco.2020.118903
Urban-Mead K. R., van Dyke M., Muniz P., Young A., Danforth B. N., McArt S. H. (2023). Early spring orchard pollinators spill over from resource-rich adjacent forest patches. J. Appl. Ecol. 60, 553–564. doi: 10.1111/1365-2664.14350
Van Lear D. H., Carroll W. D., Kapeluck P. R., Johnson R. (2005). History and restoration of the longleaf pine-grassland ecosystem: Implications for species at risk. For. Ecol. Manage. 211, 150–165. doi: 10.1016/j.foreco.2005.02.014
Walker J. (1993). “Rare vascular plant taxa associated with the longleaf pine ecosystem: patterns in taxonomy and ecology,” in Proceedings of the Tall Timbers Fire Ecology Conference. Ed. Hermann S. M. (Tall Timbers Research Station, Tallahassee, Florida., Tallahassee, FL), 105–125.
Keywords: Apoidea, flower visitors, fire-adapted, forest-dependent, saproxylic, shortleaf pine
Citation: Ulyshen MD, Robertson K, Horn S and Dixon C (2024) Characterization of the bee community and pollination network in a southeastern U.S. pine savanna. Front. Ecol. Evol. 12:1403602. doi: 10.3389/fevo.2024.1403602
Received: 19 March 2024; Accepted: 16 April 2024;
Published: 14 May 2024.
Edited by:
Pedro Gonçalves Vaz, University of Lisbon, PortugalReviewed by:
Gabor Pozsgai, University of the Azores, PortugalCopyright © 2024 Ulyshen, Robertson, Horn and Dixon. This is an open-access article distributed under the terms of the Creative Commons Attribution License (CC BY). The use, distribution or reproduction in other forums is permitted, provided the original author(s) and the copyright owner(s) are credited and that the original publication in this journal is cited, in accordance with accepted academic practice. No use, distribution or reproduction is permitted which does not comply with these terms.
*Correspondence: Michael D. Ulyshen, bWljaGFlbC5kLnVseXNoZW5AdXNkYS5nb3Y=
Disclaimer: All claims expressed in this article are solely those of the authors and do not necessarily represent those of their affiliated organizations, or those of the publisher, the editors and the reviewers. Any product that may be evaluated in this article or claim that may be made by its manufacturer is not guaranteed or endorsed by the publisher.
Research integrity at Frontiers
Learn more about the work of our research integrity team to safeguard the quality of each article we publish.