- Centre d’étude de la forêt, Chaire en aménagement forestier durable Université du Québec en Abitibi-Témiscamingue – Université du Québec à Montréal (UQAT-UQAM), Département des sciences biologiques, Université du Québec à Montréal, Montréal, QC, Canada
Introduction: In eastern Canada, the boreal forest is associated with an important latitudinal shift in forest composition and structure, which occurs in the transition between the mixed southern boreal forest and the coniferous northern boreal forest. Along this transition, upland mixedwood stands with large deciduous trees (important for cavity-dependent vertebrate species) are gradually replaced by forests with smaller conifer trees, primarily black spruce (Picea mariana). Concomitantly, the availability of lowland forests flooded by the American beaver (Castor canadensis), which can provide adequate conditions for tree-cavity users, is also decreasing.
Methods: We hypothesized that this latitudinal gradient would bring important changes in the functional diversity and network structure of vertebrate cavity-using communities. Along this latitudinal gradient we used a nest web approach to analyze the structure and robustness of networks of cavity users in upland forests and in lowland forests flooded by beavers.
Results: Despite their low availability in the northern forest region, we found that mixedwood stands persisted throughout the boreal forest in being the main drivers of nest webs network structure of upland forests whereas old black spruce stands contribution was low. In lowland forests, beaver ponds harbored nest webs with a rich and complex structure in both forest regions. Species removal simulations revealed that across our latitudinal gradient upland and lowland forest nest webs responded differently. In upland forests, the removal of trembling aspen and the Pileated Woodpecker (Dryocopus pileatus) caused the highest proportions of secondary extinctions, showing low robustness of nest webs given that these two species were highly connected to the other species. Contrastingly, nest webs in beaver ponds were more robust mainly because excavator species used a higher diversity of tree species despite the removal of the Northern Flicker (Colaptes auratus) which induced numerous secondary extinctions. The Pileated Woodpecker remained the pivotal species across the two forest regions in upland forests whereas the Northern Flicker became the main large cavity provider in beaver ponds across the latitudinal gradient.
Discussion: We discuss how mixedwood forests and beaver ponds, which are key habitat types for the cavity-using vertebrate community across our latitudinal gradient, should be maintained and protected in landscapes under industrial timber harvesting.
1 Introduction
One of the most recognized patterns in ecology is the general decline in species diversity along latitudinal gradients from the tropics to the poles (Pianka, 1966; Rohde, 1992) or within continents (McCoy and Connor, 1980; Karanth et al., 2014). Along with this trend, a reduction in functional diversity is also observed (Kerkhoff, 2012). This pattern is also noticeable within the largest forest biome of the planet, the boreal forest, as avian diversity declines following the habitat type gradient from the south to the north (Kouki, 1999). In the eastern Canadian boreal forest this transition is characterized by a decrease in the availability of mixedwood stands with large diameter deciduous trees from the southern mixedwood forest region to the northern coniferous forest region, as the forest matrix becomes dominated by smaller-sized conifer trees (Gauthier et al., 2000; Drapeau et al., 2003; Bergeron et al., 2004).
This shift in tree species composition and tree-stem diameter, could likely affect species diversity of vertebrates, particularly those that use cavity-bearing trees in old forests since studies conducted so far in boreal and hemiboreal forests of North America have shown that cavity-using vertebrates are strongly linked to large old trembling aspens (Populus tremuloides) (Martin et al., 2004; Blanc and Martin, 2012; Ouellet-Lapointe et al., 2012; Cadieux et al., 2023). Moreover, from a functional diversity viewpoint, trembling aspen trees drive networks (hereafter called nest webs) of ecological interactions among cavity-bearing tree species, cavity formation agents (wood decay and excavating bird species) and non-excavator cavity users (Martin et al., 2004; Cooke and Hannon, 2011; Cadieux et al., 2023). The strong association between woodpeckers and aspens for cavity excavation is also well documented in European temperate, hemiboreal and boreal forests where intensive silviculture has considerably reduced the importance of key deciduous tree species such as Populus tremula (Remm and Lõhmus, 2011; Wesolowski and Martin, 2018), an important cavity-bearing tree species for several woodpecker species such as the endangered White-backed Woodpecker (Dendrocopos leucotos) (Carlson, 2000).
In a previous study, Cadieux and Drapeau (2017) found that rare and scattered mixedwood stands harbored the highest richness of birds associated with decaying and deadwood of all forest cover types in the northern coniferous region of the boreal forest in eastern Canada. This makes them habitat types of special concern for this functional group of birds in the region. However, they did not measure the structure of nest webs in this part of the boreal biome. Hence, the importance of mixedwood stands as regional drivers of functional diversity in vertebrate cavity-using communities in northern regions dominated by coniferous forest remains unknown.
Apart from natural senescence and wood decay processes associated with aging forest stands (Franklin et al., 1987; Jackson and Jackson, 2004; Bergeron and Fenton, 2012; Cadieux et al., 2023), the flooding of lowland forest stands by dams created by the American beaver (Castor canadensis) is another ecological process that allows the recruitment of decaying and dead standing trees in boreal ecosystems (Naiman et al., 1986). This process can provide adequate conditions for cavity-using vertebrates. Flooded trees typically have their roots and lower trunks submerged in water, whereas the top of the tree is usually in an open environment, exposed to direct sunlight, which hardens its exterior. In addition, the humid environment in flooded trees favors rapid development of fungi and decay organisms (Jackson and Jackson, 2004), thus facilitating their excavation by woodpeckers and other excavators. Numerous studies have shown that beaver ponds promote biodiversity by generating habitat for multiple terrestrial and aquatic species (Naiman et al., 1986; Grover and Baldassarre, 1995; Wright et al., 2002; Rosell et al., 2005; Aznar and Desrochers, 2008; Nummi and Kuuluvainen, 2013; Feldman et al., 2023). In a global review on beaver activity impacts, Grudzinski et al. (2022) found that studies within boreal areas are limited relative to other biomes. Whereas some work has also been conducted in beaver ponds on single species of woodpeckers and other cavity users (Lochmiller, 1979; Pietrasz et al., 2019; Orazi et al., 2022), to our knowledge no studies have documented the network structure of nest webs in these habitat types. In forest landscapes disturbed by beaver activities such as in our study region (Feldman et al., 2023), beaver ponds may provide an important source of cavity-bearing trees for the vertebrate cavity-using community. Moreover, in a context such as the black spruce-moss forest region, where old large aspens become rare and patchily distributed, beaver ponds could compensate for the limited sources of cavity-bearing trees of upland forests. In this paper, we analyze the structure of nest webs along a latitudinal gradient ranging from mixedwood to coniferous boreal forests in eastern Canada. We focus on specific habitat types rich in senescent and dead trees in both upland forests and lowland forests flooded by the American beaver. We analyze the persistence and changes in the structure and composition of nest webs along our latitudinal gradient. We test the robustness of nest webs, i.e., network’s tolerance to remain connected with a species removal analysis (Dunne et al., 2002). For upland forests, we hypothesize (H1) that the declining availability of large trees along our latitudinal gradient and the shift in tree species composition from mixedwood to coniferous stands will affect the cavity-using communities and change the network structure of nest webs at the tree and cavity excavator levels. As we move along this latitudinal gradient, we thus predict less diversified nest webs driven by a lower number of cavity excavators in coniferous forests and the decline in the occurrence of mixedwood excavator species, particularly the Pileated Woodpecker, a keystone large cavity provider in the boreal forest (Bonar, 2000; Bonar, 2001; Cooke and Hannon, 2011; Cadieux et al., 2023). We also predict that in the northern boreal conifer region this functional role will be played by the Northern Flicker, which has been shown to be more flexible in its nesting substrates (Wiebe and Moore, 2008). For lowland forested beaver ponds, we hypothesize (H2) that, when compared to upland forests, the smaller size of these habitat types will harbor less complex and less diversified nest webs across our latitudinal gradient.
2 Materials and methods
2.1 Study area
Our study area covers 12,000 km2 in the northern Clay Belt of northwestern Quebec and Ontario (Figure 1). It spans a 200 km latitudinal gradient across the continuous boreal forest which is divided into the southern boreal forest region (Abies balsamea–Betula papyrifera mixedwood zone) and the northern boreal forest region (Picea mariana-moss coniferous zone) (Robitaille and Saucier, 1998). The entire study region is characterized by extensive industrial timber harvesting (Drapeau et al. 2009a). The southern boreal region is characterized by clay soils and low rocky hills (Robitaille and Saucier, 1998). The area contains a complex natural forest mosaic formed by different forest cover types and driven by a variety of natural disturbances (Harvey, 1999). The northern boreal region has a generally flat topography (Robitaille and Saucier, 1998) and is known for its high vulnerability to paludification, a process of gradual conversion of a mesic forest to forested peatland through the accumulation of organic material and water table rise (Fenton et al., 2005; Simard et al., 2007). The forest mosaic is dominated by black spruce stands of different age and structure with marginal importance of mixedwood stands in this region (Gauthier et al., 2000).
2.2 Study design
We established sampling plots throughout the entire study region using a stratified semi-random design (Figure 1). The stratification was based on forest age and tree composition while the semi-random aspect was due to logistical constraints that necessitated the selection of accessible parcels of forests. In the southern boreal region, plots were in the Lake Duparquet Research and Teaching Forest (LDRTF) area at the southern fringe of the boreal mixedwood forest in Québec (48°30’ N, 79°22’ W; Figure 1). Research on cavity nesters has been undergoing since 2003 (Nappi, 2009; Cadieux, 2011; Nappi et al., 2015; Cadieux et al., 2023). Twelve sampling plots (24–40 ha) were distributed in an unmanaged continuous mature to old mixedwood forest tract (35 km2) of the LDRTF within which four forest age class categories (61–89, 135, 182–208 and 245 years after fire) determined from detailed studies on fire history reconstruction of last three centuries (Bergeron, 1991; Dansereau and Bergeron, 1993). These age categories, determined by time since last fire, represented the structural and compositional changes that occur in the mixedwood forest from early to late successional stages (Cadieux et al., 2023). Stands were composed of trembling aspen and paper birch (Betula papyrifera), white spruce (Picea glauca), balsam fir (Abies basamea), black spruce and eastern white cedar (Thuya occidentalis) (Bergeron, 2000). Furthermore, between 2008 and 2013, research projects were conducted within the managed area nearby the LDRTF. Fourteen plots totaling 31 hectares of linear strips of riparian buffers and cutblock separators were distributed in remnant upland mature to old mixedwood stands (> 90 years) surrounded by cutover areas in aggregated clearcut landscapes (Ouellet-Lapointe et al., 2012; Bédard, 2013). In 2015, we established 20 plots in beaver ponds (10 in mixedwoods and 10 in coniferous stands) of varying size (0.33 to 5.6 ha). Beaver ponds were selected in the field and had to be accessible by logging roads, be > 1,000 m2 in size and have at least one tree with a diameter > 25 cm. In addition, nests found in lowland portions of the twelve upland sampling plots were incorporated in nest web analyses of beaver ponds.
In the northern portion of our study area, stand composition was dominated by coniferous stands of black spruce of different ages. We used precise stand age data from fire history reconstruction studies based on dendrochronology analyses of trees and forest succession analyses (Gauthier et al., 2000; Bergeron et al., 2004; Bergeron et al., 2006) to established in 2012, a study design composed of 24 sampling plots (10 ha) in unharvested old forest stands dominated or co-dominated by trembling aspen (11 plots ranging from 96 to 105 years since fire) and by black spruce (13 plots ranging from 96 to 237 years since fire). These plots were selected by combining updated digital forest cover maps (MRNF, 2011) and fire history reconstruction maps (Bergeron et al., 2004; Bergeron et al., 2006) for the entire study region. To be selected, a forest patch had to be accessible by logging roads and be large enough to fit a 10-ha plot (250 m by 400 m). When possible, plots were located to avoid edge effects of logging roads. For lowland sampling sites flooded by beavers we used the same methodology as in the southern boreal region, 24 plots of varying size (0.1 to 3.2 ha) were established in beaver ponds in 2012 (12 in mixedwood ponds and 12 in coniferous ponds).
2.3 Nest search and cavity monitoring
Occupied cavities were systematically searched within designated plots. Additionally, during our travels, from one plot to another, active cavities were opportunistically found along logging roads and in forests near our plots. Observers were instructed to follow any hint that could indicate a nest or active cavity. This combined approach of systematic and opportunistic searches enhanced the detection of active cavities and improved the detection of interspecific interactions between cavity-users by increasing sample size. It also ensured a broader regional coverage of conditions used by cavity producers and users in both forest regions. To ensure that combining data from plots and opportunistic observations did not introduce significant biases, we compared key nest site and nest species characteristics between the two data sources. The results of this comparison are presented in supplementary material (Supplementary Table S1).
Nest searches were conducted between the beginning of May and the middle of July. In plots of upland forests, observers walked systematically along transects separated by 50 m and used visual and auditory cues that would indicate the presence of an occupied cavity: breeding-bird behavior, begging nestlings or wood chips on the ground. Observers also scanned trees for cavities. When possible, the excavator of the cavity was visually identified. If no excavator was present at the site, we indirectly estimated the excavator by using the size and characteristics of the cavity. If there was a doubt, the excavator remained unidentified (Cadieux et al., 2023). Two search surveys were conducted during the breeding season. In lowland forested beaver ponds, observers did a first cavity search survey by walking around the pond and spot mapping all cavity-nesting species, potential nests, and cavity trees. The second survey was done with high boots and a canoe in which every potential cavity tree mapped in the first survey was inspected. All trees with cavities were characterized by their tree species, diameter at breast height (DBH) and degradation (live or dead) and were also checked for the presence of fungal conks of species associated with heartwood decay. Cavities were inspected with a camera mounted on a telescopic pole to determine the state of the cavity: non-completed excavation, suitable cavity, or occupied cavity. A cavity was considered “occupied” if it contained at least one egg or nestling, or if a mammal was present inside the cavity (Martin et al., 2004; Cadieux et al., 2023).
2.4 Vegetation sampling
In upland forest study plots, vegetation sampling was conducted to characterize and compare cavity-bearing trees with available trees. Within each study plot, all available trees with a DBH >10 cm were measured and characterized by tree species, DBH, and tree degradation (live or dead and presence or absence of fungal conks). In the southern boreal mixedwood region, vegetation sampling was conducted in 112 rectangular shaped stations of 600 m2 (10 m × 60 m) and in 56 circular stations of 400 m2 that were distributed 100 to 200 m apart to cover each study plot. In the northern boreal coniferous region, the same variables for all trees were measured in the 24 upland forests plots by using a variable point sampling procedure with a Factor 2 wedge prism (Thompson et al., 2006) in 384 vegetation stations.
In lowland forest plots flooded by beaver, systematic or random placement of vegetation plots did not perform well to characterize the vegetation as plots often fell on empty areas given the openness of the habitat. Moreover, accessing certain areas in beaver ponds proved challenging due to fallen trees, underwater logs and branches, thick vegetation, and waterlogged terrain. For these reasons, vegetation sampling was centered on accessible trees. For beaver ponds with few trees, these were all characterized. For other beaver ponds we aimed at characterizing at least 30 trees representative of the tree population in the beaver pond.
At the landscape scale, we used digital forest cover maps of the 4th Quebec provincial forest inventory to measure the proportions of forest cover types and wetlands in each forest region (MRNF, 2011). At the time we conducted the fieldwork, these forest cover maps were the most recent available.
2.5 Statistical analyses
2.5.1 Local habitat characteristics of study plots
Characteristics of cavity-bearing trees, and available trees were compared between upland and lowland forests in the two forest regions by averaging values by plot. Northern boreal upland forests were split into mixedwood and coniferous forest cover types because of striking differences in available tree characteristics of these two forest cover types whereas in southern boreal mixedwood upland forests only mixedwood stands were characterized. For trees > 10 cm in diameter at breast height (DBH), we compared the mean DBH as well as the proportion of dead trees, coniferous trees, deciduous trees and trembling aspens. The same characteristics were measured in lowland forests flooded by beaver in both regions where mixedwood and coniferous ponds were grouped in each region. Kruskal-Wallis tests were conducted to compare available vs. used cavity-bearing trees for each habitat type in the two forest regions. P-values for statistical significance were set at P < 0.05.
2.5.2 Nest-web structure and robustness to the loss of keystone species
To compare interactions among cavity-bearing tree species, cavity producers and cavity users along the latitudinal gradient we built four nest webs in both regions for upland forests and flooded lowland forests by grouping all nests found in our study plots. Additionally, nests found opportunistically along logging roads were associated with a forest cover type (mixedwood or coniferous) with on-site verification and cross-referencing their location with a digital forest cover map (MRNF, 2011).
Strength of interactions was computed for each link between cavity-bearing tree species, cavity producing species and non-excavator users. It was calculated separately for two levels: the cavity-bearing tree to excavator level and the excavator to non-excavator cavity reuse level. Three categories of linkage strength were used to illustrate network structure of our nest webs: weak links (< 10%), medium links (10–50%) and strong links (> 50%) (Martin and Eadie, 1999; Cadieux et al., 2023).
Using Shannon-Weaver’s diversity index, nest webs were characterized with four different network characteristics including interaction diversity, cavity-bearing tree species diversity, excavator diversity and non-excavating user diversity. To compare nest web network structure among our nest webs, we used a bootstrap procedure with replacement of 10,000 permutations to calculate the means and 95% confidence intervals of the different network characteristics. To compute interaction diversity, every occupied cavity was categorized based on its interspecific interactions stemming from three different levels across our networks: the tree species level, the excavator level and the non-excavator level (Cadieux et al., 2023). Sampling size was set to the lowest network size (77 nest trees) to balance the number of occupied cavities by habitat type. Differences between habitat types were considered significant when the 95% confidence intervals did not overlap.
Using the same resampling method, we evaluated the robustness of nest webs to the loss of specific species by measuring the proportion of secondary extinctions (loss of other species) related to each species removal (sensu Dunne et al., 2002) in upland and lowland forests of both forest regions. Nest webs with low robustness will show high proportions of secondary extinctions whereas nest webs with high robustness will have lower proportions of secondary extinctions. In the case of this analysis for each permutation, we removed a specific species after having sampled the data. We removed excavator species (Downy Woodpecker – Picoides pubescens, Yellow-bellied Sapsucker – Sphyrapicus varius, Black-backed Woodpecker – Picoides arcticus, Northern Flicker, Pileated Woodpecker) and tree species (trembling aspen, black spruce, balsam fir, white birch, balsam poplar – Populus balsamifera). All statistical analyses were performed with R version 3.2.3 (R Core Team, 2015) and graphics were created with the R package: ggplot2 (Wickham, 2016).
3 Results
3.1 Forest cover types in the two forest regions
Digital forest cover maps depict contrasting differences between the southern and northern boreal regions where the proportions of wetlands vary from 12 to 48% along the transition from the southern boreal to the northern boreal region (Figure 2). Proportions of forest cover types on digital forest maps depict the longer industrial logging history under even-aged management with clearcuts of the southern boreal forest region and its northern progression whereas the age structure of both regions has shifted or is shifting towards higher proportions of regenerating and young forests (less than 70 years old) to the expanse of mature to old forests (over 70 years old). Hence, forests under 70 years cover 61% of the landscape in the southern boreal region and 20% in the northern boreal region whereas forest cover types older than 70 years were respectively represented by 27% and 32% of the stands in the southern and northern boreal regions (Figure 2). However, the proportion of deciduous and mixedwood stands over 70 years was only 4% in the northern boreal region compared to 12% in the southern boreal regions (Figure 2) and these forest cover types were fragmented within a matrix dominated by a coniferous forest cover.
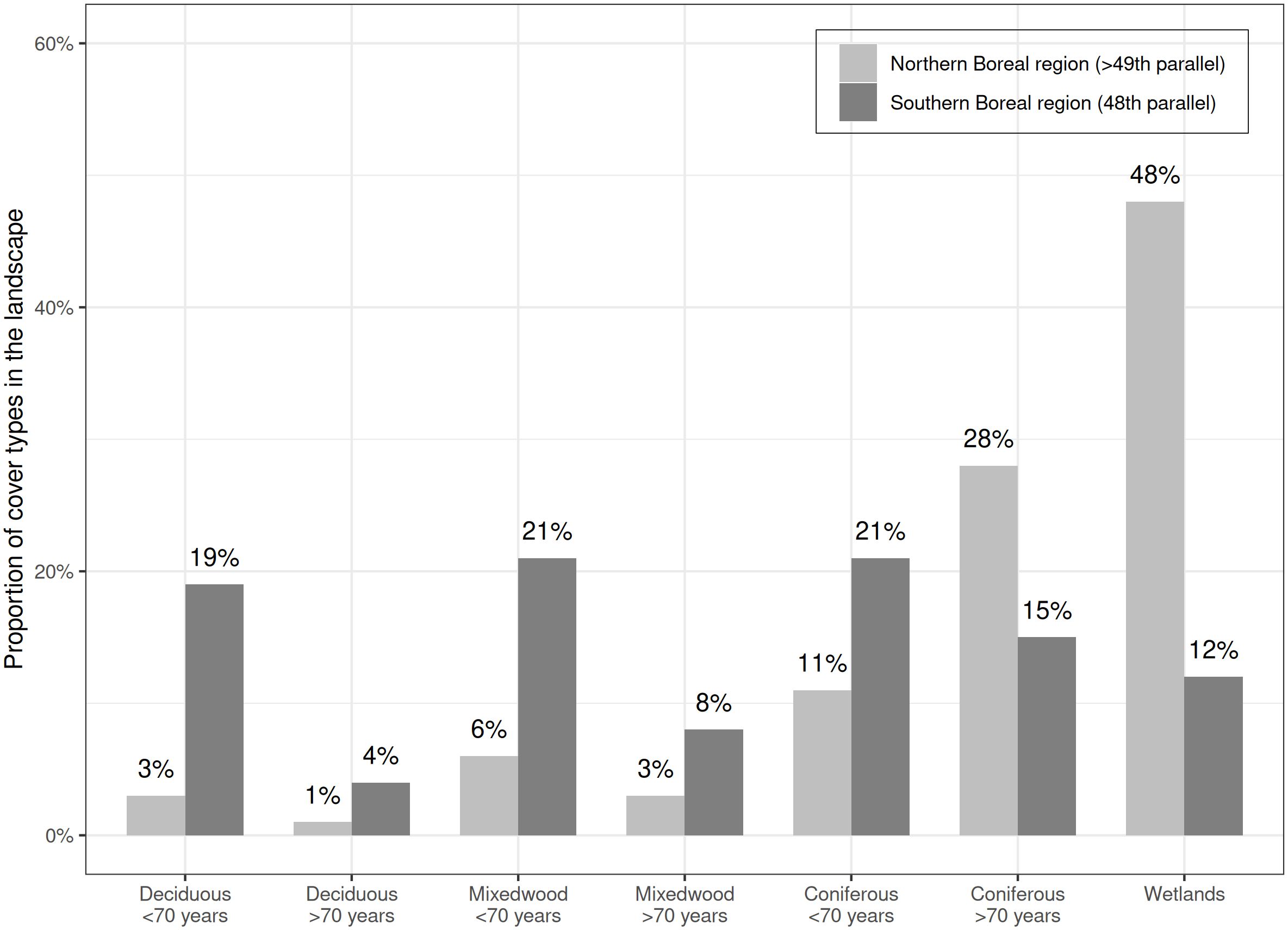
Figure 2 Proportion of forest cover types and wetlands for southern (48th parallel) and northern (49th parallel) boreal forest regions in northwestern Québec, Canada.
3.2 Local habitat characteristics of sampling plots
Comparisons between characteristics of cavity-bearing trees and available trees in the sampling plots investigated along our latitudinal gradient for upland and lowland forests show specific patterns (Table 1). In the northern boreal region very few cavity-bearing trees were found in upland coniferous forests plots (n=5) compared to mixedwood plots (n=58) or flooded lowlands (n=48) (Table 1). Selection patterns were observed when cavity-bearing trees characteristics were compared to those of available trees in upland and lowland forests in both forest regions. Cavity-bearing trees had a significantly higher DBH than available trees in all habitat types (Table 1). The proportion of cavities in standing dead trees was significantly higher than their availability in upland forests in both forest regions but this was not the case for beaver ponds since standing trees in ponds were all dead. Deciduous trees, mostly trembling aspen, were strongly selected as cavity-bearing trees in mixedwood upland forest plots in both regions. In southern beaver ponds, there was a significant selection for deciduous trees and a significant avoidance of conifers whereas in northern beaver ponds, there was no significant difference in characteristics of cavity-trees and available trees (Table 1).
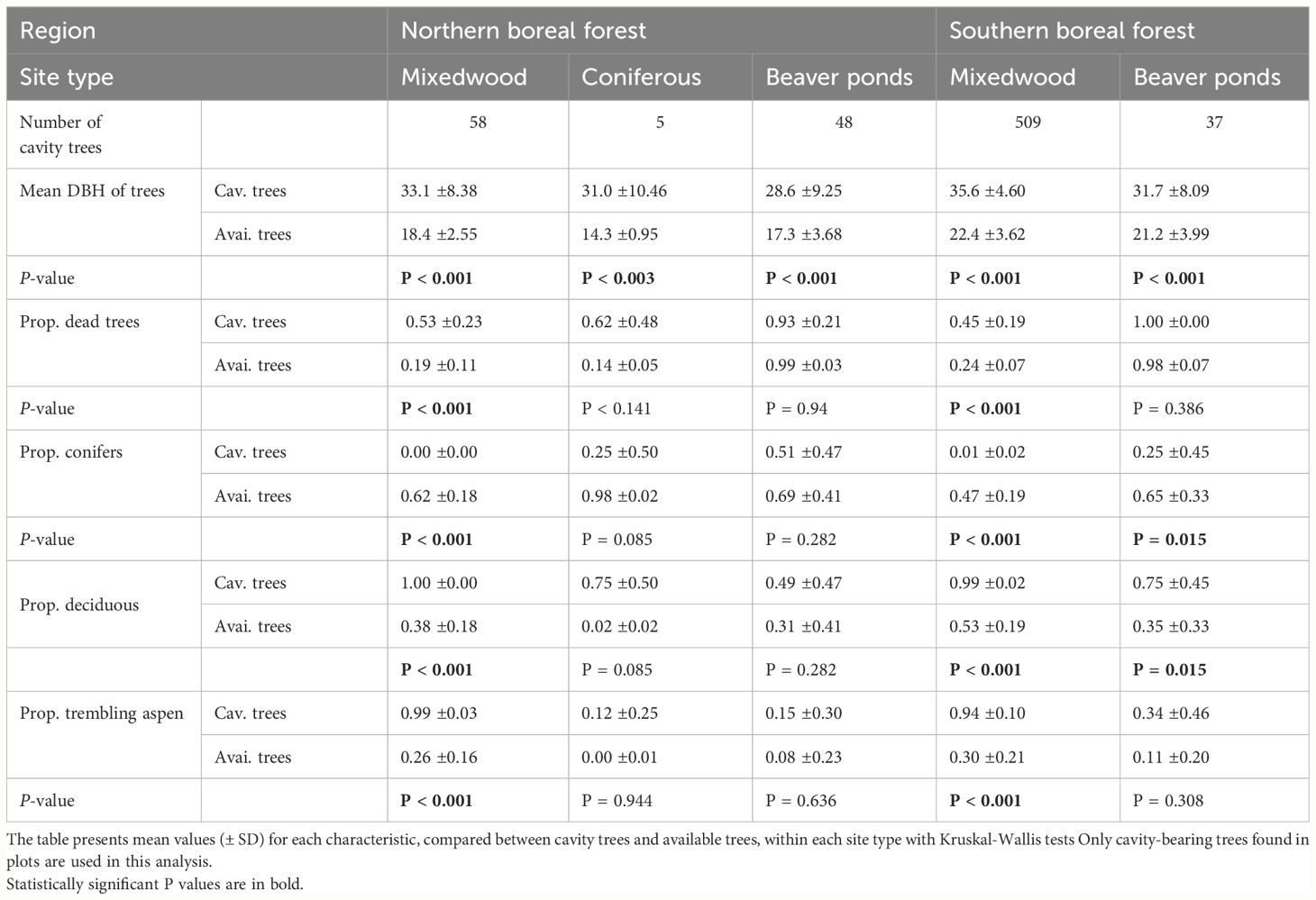
Table 1 Characteristics of cavity-bearing trees and available trees in plots sampled in southern and northern boreal forest regions on upland and lowland sites.
3.3 Nest webs network structure and robustness to the loss of key species
In the southern boreal region, throughout the 2003 to 2015 period, we found 851 nests and occupied cavities for 20 cavity-nesting species. Most occupied cavities were found inside of plots and 13% were found opportunistically outside of plots along the logging roads. In the northern boreal region, from 2012 to 2015, we found 249 nests and occupied cavities for 21 cavity-nesting species. Most occupied cavities were found inside plots and 42% were found opportunistically outside of plots next to logging roads.
Cavity users found in the southern boreal region were also found in the northern boreal region apart from the Wood Duck (Aix sponsa), the Common Raccoon (Procyon lotor) and the Northern Hawk Owl (Surnia ulula). However, the American Three-toed Woodpecker (Picoides dorsalis) and the Boreal Owl (Aegolius funerus), which were absent from our southern boreal region networks, were added in the northern boreal region nest webs. Across forest regions only 5% of nests were found in coniferous trees (n = 54), mostly in black spruce and 95% of nests were found in deciduous trees (n=1046), mostly in trembling aspen (85%; Figure 3 and Supplementary Figure S2).
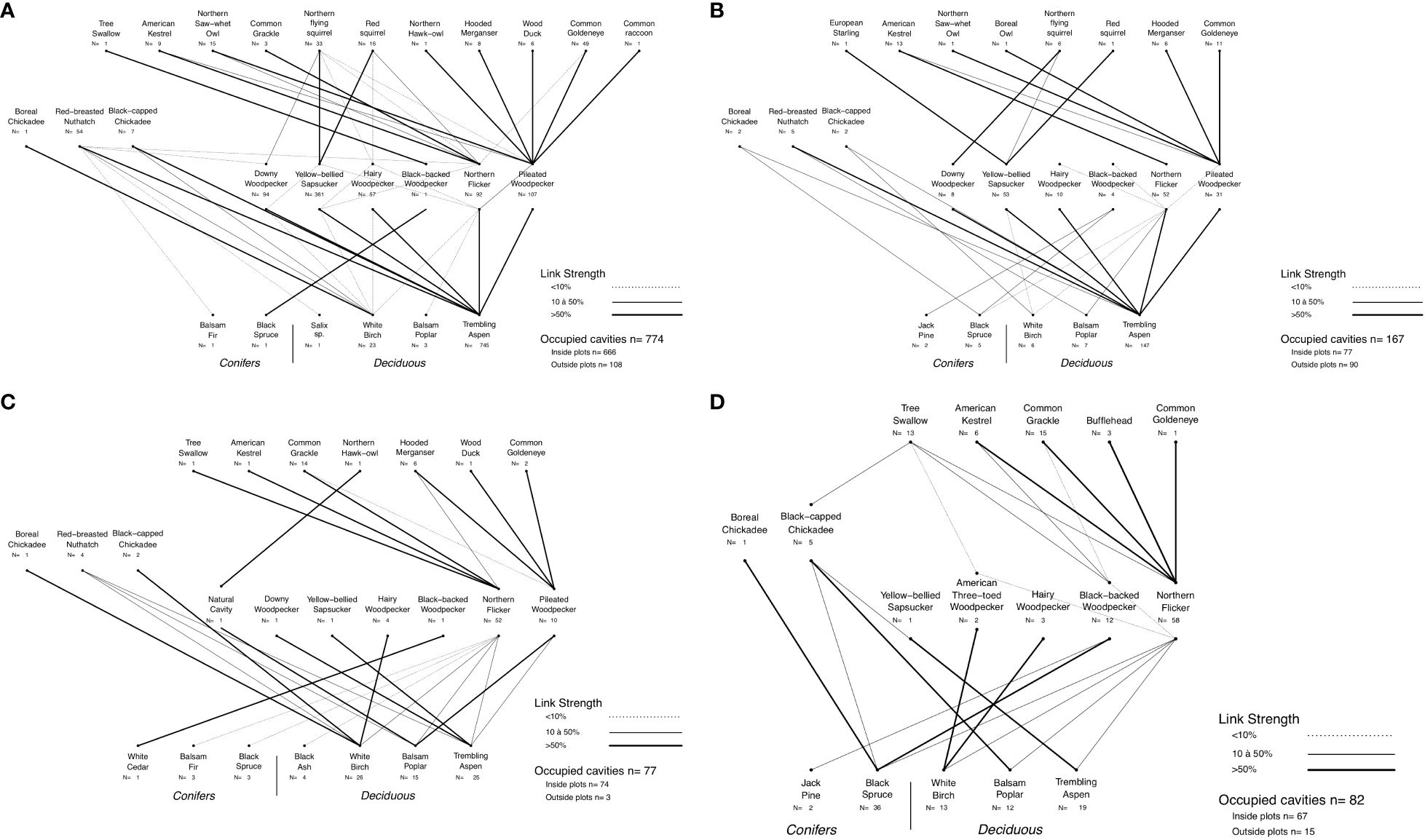
Figure 3 Nest webs for (A) southern boreal upland forests, (B) northern boreal upland forests, (C) southern boreal beaver ponds, (D) northern boreal beaver ponds in northwestern Québec, Canada. Nest data were collected from 2003 to 2015 for the southern boreal region and from 2012 to 2015 for the northern boreal region. Lines between species represent the use of cavity resources. N is the number of occupied cavities. At the excavator level, N represents the total number of cavities excavated that are used by this species or by a different species. Links between the excavator level and the tree level represent the proportion of occupied cavities found in the tree species whereas the links between non-excavator cavity users and excavators represent the proportion of nests or presence of mammals found in reused cavities. Reuse or re-excavation of cavities by excavators are indicated by lines between the base of the excavator that reused the cavity and the top of the excavator that created the cavity.
The fact that most cavity-bearing trees were in deciduous trees in upland forests of both regions and that nest webs shared a high number of excavators and non-excavators highlights their strong similarity (Figures 3A, B). Only a limited number of cavities were found in coniferous trees which were mainly excavated by the Black-backed Woodpecker and the Northern Flicker (Figure 3B). Another convergence is that in both nest webs, the network structure was centered on the trembling aspen as the main cavity-bearing tree species, the Yellow-bellied Sapsucker as the main cavity excavator and nester and the Pileated Woodpecker, which was the cavity excavator with the most cavities reused by non-excavator cavity users (Figures 3A, B).
Across our latitudinal gradient, most nests in coniferous trees were found in beaver ponds (83%). In this habitat type the Northern Flicker was the main cavity excavator and cavity nester. In addition, throughout the boreal forest, the Northern Flicker was the species that most often enlarged existing cavities created by other species (Figures 3A–D). Notably, in the northern boreal region, it enlarged existing Black-backed Woodpecker and American Three-toed Woodpecker cavities (Figure 3D). Following these enlargements, several non-excavator cavity users reused these cavities for denning or nesting.
The vertebrate communities of cavity-users in beaver ponds were generally similar in composition and structure in both regions. However, some distinctions were observed in the southern boreal region where sampled beaver ponds were often embedded in large mixedwood forest tracts. These environments favored vertebrate species typically associated with nest webs of upland mixedwood forests, which either excavated or used flooded dead trees (Figures 3C, D). This was particularly true for cavities excavated by the Pileated Woodpecker in southern boreal beaver ponds, which were absent in the northern beaver ponds.
Cavity-bearing tree species diversity was highest in beaver ponds and lowest in upland forests for both forest regions in subsampled nest webs (Figure 4). Nonetheless, diversity of primary cavity excavators (woodpeckers) was higher in upland forests than in beaver ponds (Figure 4). However, this difference was not significant for southern boreal upland forests. Diversity of non-excavators did not differ significantly between nest webs as all confidence intervals overlapped (Figure 4). Diversity of interspecific interactions within our nest webs was highest in beaver ponds across our latitudinal gradient because of the higher diversity of cavity-bearing tree species in this habitat type. The nest web of upland forests in the southern boreal region had significantly lower interaction diversity as links among cavity-bearing tree species and excavators were more concentrated around trembling aspen whereas in the northern boreal region the upland forest nest web did not differ significantly from the beaver pond nest webs (Figure 4).
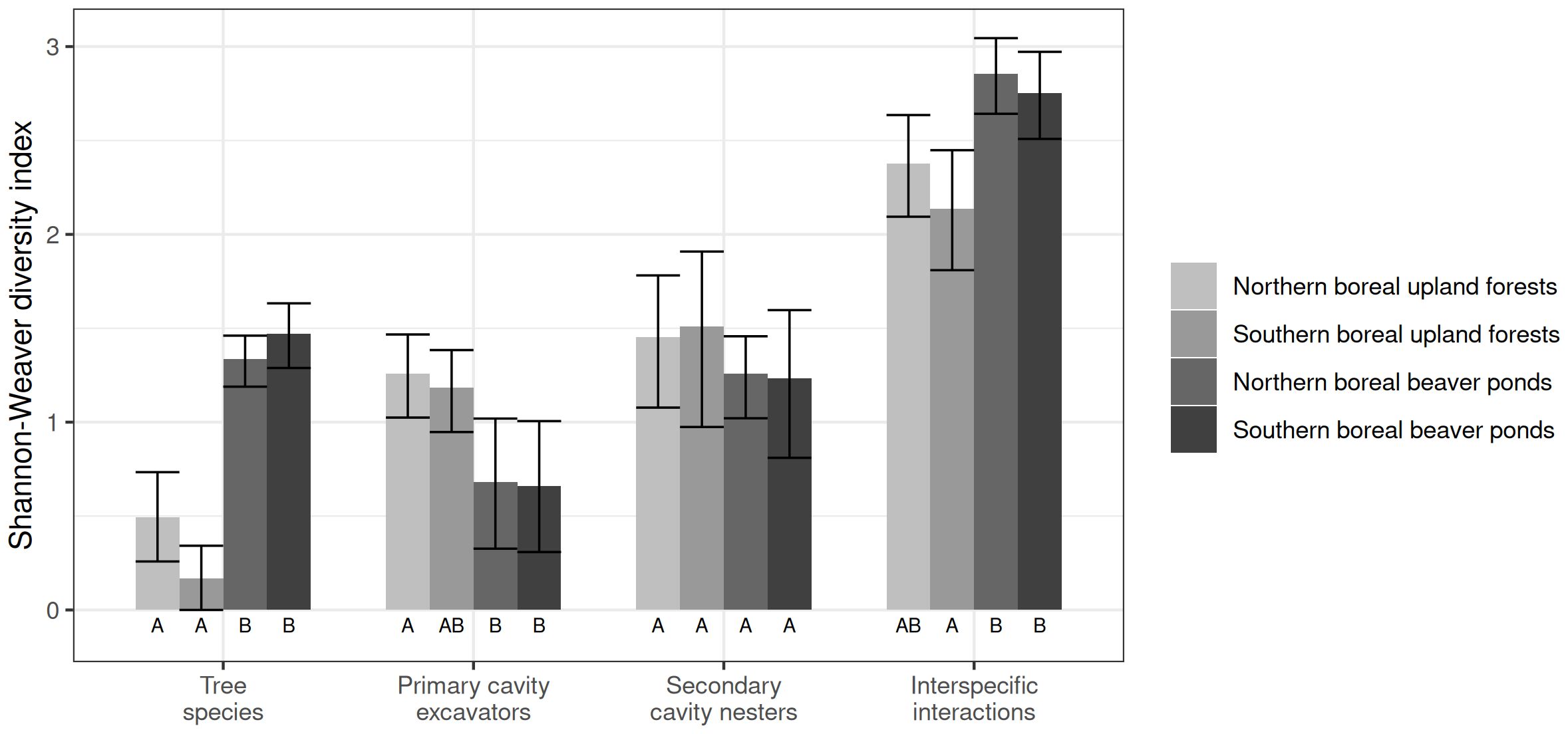
Figure 4 Means and 95% confidence intervals of Shannon-Weaver diversity index for characteristics of nest webs in upland forests and lowland beaver ponds of northern and southern boreal forest regions. We compared the diversity of cavity-bearing tree species, woodpecker species, non-excavator cavity users and interspecific interactions. The bootstrap procedure is described in section 2.5.2.
Our simulations of the removal of species had variable effects on the network robustness of cavity-nesting communities in upland and lowland forests in our two forest regions (Figures 5A, B). The removal of the Pileated Woodpecker caused a high proportion of secondary extinctions of non-excavator users with a loss ranging from 44% in upland forests of the southern boreal region to 50% in the northern boreal region (Figure 5A). The removal of the Northern Flicker had a strong impact in beaver ponds of the northern boreal region leading to a rate of secondary extinctions of 50% of non-excavator users (Figure 5A). The removal of other excavating species did not have a significant impact in all nest webs (Figure 5A). When simulating the removal of tree species in upland forests, the removal of trembling aspen caused the highest proportion of secondary extinctions reaching 81% in the southern boreal region and 73% in the northern boreal region forest region (Figure 5B). Beaver ponds displayed a higher robustness to tree species removal than upland forests (Figure 5B).
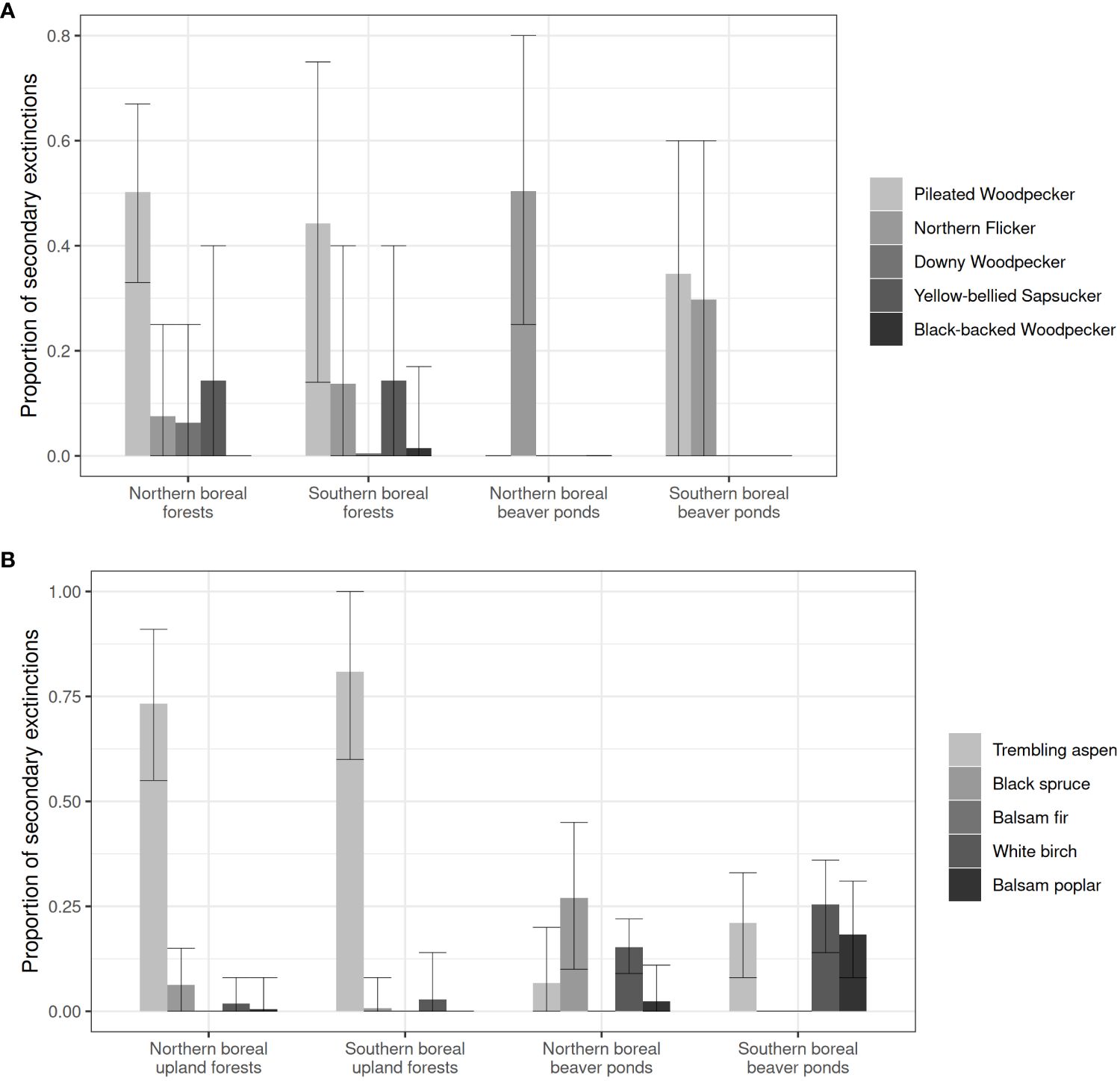
Figure 5 Evaluation of the robustness of nest webs to the loss of (A) excavator species and (B) tree species in upland forests and lowland beaver ponds of northern and southern boreal forest regions. Means and 95% confidence intervals of the proportion of species loss due to the simulation of targeted species removal are presented.
4 Discussion
To our knowledge, this study is the first to document and compare at a regional scale, nest webs of cavity-using vertebrate communities in upland and lowland forest cover types embedded in contrasted boreal forest landscapes. Along our latitudinal gradient ranging from the mixedwood to the coniferous boreal forest region, we observed changes but also persistence in the nest web structure of cavity-using vertebrate communities. The drastic reduction in the availability of mixedwood stands with large deciduous senescent and dead trees adequate for cavity excavation did not translate in the northern region into less complex network structure of nest webs. Therefore, it did not support our first hypothesis that a reduced availability of mixedwood forest cover types in the north would lead to simpler nest webs. Instead, the same cavity-using bird community was able to colonize isolated patches or tracts of mixedwood forests in the northern boreal forest region. In contrast, even though they were an important forest cover type in the northern boreal forest region old conifer stands contributed much less to upland forest nest webs (Figures 3A, B). Thus, our first prediction of a weak contribution of old conifer forest stands to nest webs in upland forests, due to a lower number of cavity excavators, was supported. The main excavators in old conifer forests, the American Three-toed Woodpecker and the Black-backed Woodpecker are known to have low densities and large home ranges (>150 ha for Black-backed Woodpecker; Tremblay et al., 2009; Tremblay et al., 2015) and tree retention of large old live and decaying ) that are strongly linked to forage substrate availability (senescent to recently dead trees) which is highly dispersed in old stands but becomes concentrated in recently burned stands (Nappi et al., 2015). Thus, the low number of nests found in our 10 ha sampling plots is likely to reflect the low densities of these two species in old black spruce forest stands.
Lowland forests flooded by beaver ponds were found to be highly important in maintaining diversified cavity-user communities across the latitudinal gradient of forest composition in this part of the Canadian boreal forest. The small size of these habitats (averaging 1.8 ha ± 1.4 SD for sampled beaver ponds) was not a limiting factor in harboring diversified nest webs with network structure as complex as what was found in upland forests for both forest regions. Moreover, the higher values of interspecific interaction diversity in nest webs in beaver ponds translated into more complex networks than in upland forests (Figure 4). Thus, our second hypothesis of less complex and less diversified nest webs due to the small size of beaver ponds was not supported across our latitudinal gradient.
4.1 Importance of mixedwood stands with trembling aspen across the latitudinal gradient
Although numerous studies conducted in forests of North America have shown that cavity-nesting birds are strongly reliant on trembling aspen (Li and Martin, 1991; Dobkin et al., 1995; Martin et al., 2004; Cooke and Hannon, 2012; Ouellet-Lapointe et al., 2012; Cadieux et al., 2023), the fact that in this study most cavities in the northern boreal forest region were in trembling aspen confirms the keystone functional role of this tree species even when the boreal forest becomes dominated by conifer stands. The strong selection by tree-hole using vertebrates for trembling aspen is thus not only consistent in the southern boreal mixedwood forest region (Cadieux et al., 2023) but also holds throughout the boreal forest biome, including the black-spruce–feather moss forest region, despite this region is harboring a very low proportion of old mixedwood forest stands (< 5%). Hence, contrary to our prediction, the declining availability of mixedwood stands with trembling aspens did not affect the occurrence of mixedwood excavator species and the overall structure of nest webs in the isolated upland mixedwood forest stands of the northern boreal forest region.
The association between cavity excavators and trembling aspen in the northern coniferous boreal forest region echoes the strong selection by woodpeckers for tree species of the genus Populus that are highly vulnerable to heart-rot fungi throughout the circumboreal forest biome (Remm and Lõhmus, 2011; Wesolowski and Martin, 2018). In the eastern Canadian boreal forest, it may also reflect an evolutionary adaptation between woodpeckers and trembling aspen that is driven by historical natural disturbance regimes where aspens persist through their root system in natural forests throughout their structural development. This phenomenon was observed in the southern boreal mixedwood forest region where we found that cavity excavators strongly selected trembling aspen trees even where their availability was at its lowest in very old mixedwood stands that become dominated by conifer trees (Cadieux et al., 2023). The same mechanism could operate in the northern boreal forest region where landscapes are dominated by conifer stands. Hence, trembling aspen could persist within conifer stands through its root system and regenerate by root suckers immediately following wildfires (Bergeron et al., 2004) or canopy gaps of small (single tree gap replacement) to medium size disturbances (insect outbreak) that affect the boreal forest structural development (Lavertu et al., 1994; Kneeshaw and Bergeron, 1998; Bergeron, 2000).
Our results also highlight the dispersal capacity and efficiency of woodpeckers, the main cavity formation agents in our nest webs, to find mixedwood forests with trembling aspen that are highly isolated within the coniferous forest matrix of black spruce stands in the northern boreal forest. For instance, Yellow-bellied sapsuckers, Downy Woodpeckers, Hairy Woodpeckers and Pileated Woodpeckers colonized mixedwood forest tracts located more than 185 km from the Lake Duparquet study sites (Cadieux et al., 2023) and contributed to nest webs with a similar network structure as those found in the southern boreal mixedwood region. However, more research is needed to assess whether habitat quality (measured by reproductive success of cavity nesters) in these isolated forests at the northern edge of distribution of most of these woodpecker species is as good as in the more continuous southern mixedwood forest tracts.
4.2 Importance of beaver ponds in lowland forests
Whereas tree senescence was a key process for upland forest nest webs, notably in mixedwood stands throughout our study region, forest flooding by beavers was an indisputable key disturbance agent in our study area where a tight complex of aquatic and terrestrial habitats provided key environmental conditions for this ecosystem engineer. Beaver ponds were used by cavity-using vertebrates across our latitudinal gradient even though the regional abundance of American beavers decreased northerly (Feldman et al., 2023). Beaver ponds were at the root of rich and complex nest webs for the cavity-using vertebrate community of the boreal forest. Contrary to our prediction and even though beaver ponds formed small habitats, the overall structure of links among cavity-bearing trees, cavity formation agents and non-excavator cavity-users in beaver ponds was as diversified as those found in upland forest nest webs. Moreover, unlike in upland forests, woodpeckers relied less on trembling aspen and excavated in a wider range of tree species including more coniferous trees, which were then used by non-excavating species. This contributed to the higher interaction diversity scores of beaver pond nest webs when compared to upland forest nest webs for both regions (Figure 4). Cavity users in this habitat type were mainly open forest species with the Northern Flicker as the main cavity excavator and the species with the highest number of occupied cavities. Another interesting result in beaver pond nest webs of both regions was the low mean height of cavities in forests flooded by beavers when compared with upland forests, that did not seem to deter the use of cavities (Supplementary Figure S1). Water might thus act as predation protection for cavity users in beaver ponds like what Roy Nielsen and Gates (2007) and Schmidt et al. (2023) found for river flood plains. More research on nesting success of cavity-nesting birds in beaver ponds could determine if predation risk is indeed reduced in this habitat type.
In the northern boreal forest region where large dead trees were not common, forests flooded by beavers provided large trees adequate for excavation that were readily used by cavity users. These areas also offered a wider range of tree species for cavity excavation which may have compensated for the lower availability of adequate trees for excavation in old upland conifer forests. For instance, more nests of Black-backed Woodpeckers were found in forests flooded by beavers where the synchronous death of trees produced higher availability of cavity trees for this species than in upland old conifer forests where tree mortality is asynchronous and dispersed. Synchronicity in dead trees recruitment, also explains why Black-backed Woodpeckers reach their highest nesting densities in recent burns (Nappi et al., 2003; Nappi and Drapeau, 2009; Nappi et al., 2010).
Beaver ponds produce important habitat changes in lowland forest habitats that may benefit biodiversity, even many years after abandonment by a beaver colony (Aznar and Desrochers, 2008; Levine and Meyer, 2019). Assessing over time the use of cavity-bearing trees by cavity users and measuring the network structure and flow of interactions among members of the cavity-using vertebrate community could increase our understanding of the regional contribution of beaver ponds to the cavity-using vertebrate community throughout the boreal forest.
4.3 Network structure and robustness of nest webs
In upland forests of both regions, network structure was centered around decaying and dead trembling aspens since mixedwood stands had a disproportionate importance in shaping nest webs in both forest regions. Hence, even though mixedwood forests were not abundant regionally in the northern portion of our study area (Drapeau et al., 2003; Cadieux and Drapeau, 2017), woodpeckers associated with mixedwoods were the main cavity producers; the Yellow-bellied Sapsucker was the main small cavity provider, and the Pileated Woodpecker was the excavator with the most reused large cavities by non-excavator users. These networks had a scale-free structure, i.e., in which few species forming network hubs (Barabási, 2009) were responsible for most links. This was the case for trembling aspen and Pileated Woodpecker. Upland forest nest webs in both regions thus showed low robustness to the loss of the trembling aspen. The removal of this tree species caused important losses in species triggering up to 80% of secondary extinctions in simulated networks. The removal of the Pileated Woodpecker caused a high proportion of secondary extinctions of non-excavator users ranging from 44% to 50% across our latitudinal gradient, also emphasizing the lower robustness (Albert et al., 2000) of nest webs in upland mixedwood stands in both regions.
Since most woodpecker species nested almost exclusively in upland mixedwood stands, old upland black spruce forests in both regions did not foster a complex cavity-using community as we found only a limited number of nests and cavity-bearing trees. Even though these forests were old (> 120–215 years), the number of large and dead trees was low. Black spruce stands in our study were indeed limited in diameter suggesting low site productivity resulting from paludification (Fenton et al., 2005; Cadieux and Drapeau, 2017). Further studies on cavity-nesting communities and nest webs in coniferous forests composed of other conifer species and/or with a higher productivity could either corroborate or provide new insights on the contribution of coniferous forests to nest webs in the black spruce forest region.
In lowland beaver ponds of both forest regions, nest webs had a higher complexity in terms of cavity-bearing tree species and a significantly higher interaction diversity than did nest webs in upland forests. They were also more robust to tree species removal than upland forests. In the southern boreal forest region they were less sensitive to the loss of trembling aspen than were upland forest nest webs. The simulated removal of Pileated Woodpecker and Northern Flicker caused a moderate level of secondary extinctions among non-excavator users suggesting the higher robustness of these networks. However, in the northern forest region, the nest web in beaver ponds had lower robustness than southern boreal beaver ponds due to a high connectedness of Northern Flicker cavities with many non-excavator users. The removal of this species induced the highest proportion of secondary extinctions (50%).
4.4 Persistence and changes in keystone excavators along the latitudinal gradient
Across our latitudinal gradient, the Pileated Woodpecker surprisingly remained a keystone species for providing large cavities that were the most reused by the largest non-excavator users, a result that shows the disproportionate importance of this species despite its very low regional occurrence in the northern portion of our study region (Cadieux and Drapeau, 2017). The absence of this keystone species in coniferous dominated forests and its removal along our latitudinal gradient in targeted removal simulations had a strong negative impact on the species diversity of non-excavator cavity nesters, particularly large cavity users who nested almost exclusively in Pileated Woodpecker cavities.
We found that the Northern Flicker, the second-largest cavity excavator of North American boreal forests was not dependent on trembling aspen. We predicted that the flexibility of this species in terms of breeding substrate selection (live senescent trees to dead trees of different decay stages) and its capacity to enlarge cavities excavated by other woodpeckers (Blanc and Walters, 2008; Wiebe and Moore, 2008), would lead to the replacement of the Pileated Woodpecker as the large cavity agent in northern boreal forest regions. The Northern Flicker did partly replace the Pileated in upland forest nest webs in which it was the only large cavity formation agent in old conifer forests but not in mixedwood stands. The keystone role of the Northern Flicker for large non-excavator species was, however, obvious for beaver pond nest webs where it was the only provider of large cavities that were used by a diverse range of non-excavator species of different sizes including a large cavity nester, the Common Goldeneye (Figure 3D). However, compared to the Pileated Woodpecker cavities which are typically excavated in senescent live trees that makes them relatively long-lasting, holes excavated by the Northern Flicker may have shorter longevity. In addition, these cavity-bearing trees are usually smaller in diameter, in dead trees, and are usually lower above ground, making them less secure and ultimately more prone to predation (Fisher and Wiebe, 2006). Conversely, Northern Flicker cavities may be the only possible choice for large-bodied cavity users in coniferous boreal forests since cavities excavated by Pileated Woodpecker or created by decay alone are extremely rare and mainly found in mixedwood stands (in agreement with Vaillancourt et al., 2009). The combination of two ecosystem engineers, the beaver and the Northern Flicker thus provide the last large nesting cavity option in northern boreal forest landscapes. Our results corroborate Robles and Martin (2014) suggestion that habitat type may mediate the interspecific associations between cavity excavators and non-excavator cavity users.
5 Conclusion
Our study has two important implications for the long-term sustainability of the cavity-using vertebrate community in the boreal forest. First, throughout our latitudinal gradient nest webs in upland forests remain strongly dependent by the persistence of old mixedwood forests even when these forests become marginal and when availability of large trembling aspen in the northern boreal region is highly reduced. Conservation of old upland mixedwood forests is thus critical given the overwhelming importance of this forest cover type for cavity-using vertebrates. The remaining large old mixedwood tracts in both forest regions need to be included in the existing protected areas network to maintain regional refuges for the cavity-user vertebrate community, as well as for other vertebrate species (Jacqmain et al., 2008; Cheveau et al., 2013). This, however, may not be enough in the context of extensive timber harvesting that is presently occurring in our study region in both upland mixedwood and coniferous forests (Bergeron et al., 2002; Drapeau et al., 2009a; Mackey et al., 2023). In addition to creating protected areas, it is necessary to adapt forestry practices to increase at the regional scale the proportion of old forest cover and reverse the trend of global decline of old large trees in managed forests (Lindenmayer et al., 2012). This can be achieved through combinations of longer rotations, continuous cover silviculture (Gauthier et al., 2009; Gauthier et al., 2015) and tree retention of large old live and decaying trees (Drapeau et al., 2009b; Edworthy and Martin, 2013). Diversifying forestry practices is not only likely to be sustainable for the cavity vertebrates but for all boreal birds associated with older forests (Drapeau et al., 2016).
Second, flooded lowland forests created by beaver activity were as important as upland old forests for the maintenance of the functional diversity and network structure of cavity-using vertebrates in the boreal forest. The flooding of trees in beaver ponds was an ecological process as critical as wood-decay processes of old trees in upland forests for facilitating excavation of cavities by woodpeckers for the recruitment of cavity-bearing trees that supported a diversified community of cavity-users with complex ecological interactions in the boreal biome. Therefore, while beaver ponds are well recognized for being hotspots for biodiversity (Grover and Baldassarre, 1995; Nummi and Holopainen, 2014; Romansic et al., 2020; Feldman et al., 2023), our study clearly showed how this habitat type is also important from a functional diversity standpoint. This new knowledge should be included to prioritize beaver ponds in the conservation planning of wetlands in the boreal biome.
Data availability statement
The raw data supporting the conclusions of this article will be made available by the authors, without undue reservation.
Ethics statement
The animal study was approved by Comite institutionnel de protection des animaux UQAM. The study was conducted in accordance with the local legislation and institutional requirements.
Author contributions
PC: Conceptualization, Data curation, Formal analysis, Investigation, Methodology, Software, Validation, Visualization, Writing – original draft, Writing – review & editing. AF: Conceptualization, Investigation, Methodology, Writing – review & editing. RD: Investigation, Methodology, Validation, Writing – review & editing. PD: Conceptualization, Data curation, Formal analysis, Funding acquisition, Methodology, Project administration, Resources, Supervision, Validation, Visualization, Writing – original draft, Writing – review & editing.
Funding
The author(s) declare financial support was received for the research, authorship, and/or publication of this article. Funding was provided by a Natural Sciences and Engineering Research Council of Canada (NSERC) graduate student scholarship to Cadieux, the Québec Fonds de la recherche nature et technologies (FRQNT) graduate student scholarship to Cadieux, the Center for forest research (CFR) scholarship for research communication, the NSERC CREATE program on Forest Complexity Modeling scholarship program with industrial and government agency partners, a NSERC Cooperative Research Development grant (RDCPJ 475301 - 14) to Drapeau and collaborators, a NSERC Discovery grant (RGPIN-2019-07075) to Drapeau, Tembec industries and Lake Duparquet Research and Teaching Forest.
Acknowledgments
We thank Antoine Plouffe Leboeuf, Hanna Brais Harvey, Louis-Joseph Drapeau, Simon Paradis, Vincent Poupart, Delphine Favorel, Jillian Slater, Sarah Wood and Derek Yarjeau for their assistance in the field. Special thanks to Mélanie Desrochers for her contributions to the map production. We are thankful to the three reviewers that provided thoughtful comments and suggestions to improve the clarity of this paper.
Conflict of interest
The authors declare that the research was conducted in the absence of any commercial or financial relationships that could be construed as a potential conflict of interest.
Publisher’s note
All claims expressed in this article are solely those of the authors and do not necessarily represent those of their affiliated organizations, or those of the publisher, the editors and the reviewers. Any product that may be evaluated in this article, or claim that may be made by its manufacturer, is not guaranteed or endorsed by the publisher.
Supplementary material
The Supplementary Material for this article can be found online at: https://www.frontiersin.org/articles/10.3389/fevo.2024.1392652/full#supplementary-material
References
Albert R., Jeong H., Barabási A. L. (2000). Error and attack tolerance of complex networks. Nature 406, 378–382. doi: 10.1371/journal.pone.0059613
Aznar J.-C., Desrochers A. (2008). Building for the future: abandoned beaver ponds promote bird diversity. Écoscience 15, 250–257http://www.jstor.org/stable/42901918
Barabási A. L. (2009). Scale-free networks: a decade and beyond. Science 325, 412. doi: 10.1126/science.1173299
Bédard S. (2013). Qualité des parcelles d’habitat résiduel des paysages aménagés en forêt boréale mixte pour la reproduction et l’alimentation du Pic Maculé (Sphyrapicus varius). M.Sc. Thesis (Montréal: Université du Québec à Montréal). Available at: https://archipel.uqam.ca/5517/.
Bergeron Y. (1991). The influence of island and mainland lakeshore landscapes on boreal forest fire regimes. Ecology 72, 1980–1992. doi: 10.2307/1941553
Bergeron Y. (2000). Species and stand dynamics in the mixed woods of Quebec’s southern boreal forest. Ecology 81, 1500–1516. doi: 10.1890/0012-9658(2000)081[1500:SASDIT]2.0.CO;2
Bergeron Y., Cyr D., Drever R., Flannigan M., Gauthier S., Kneeshaw D., et al. (2006). Past, current, and future fire frequencies in Quebec’s commercial forests: Implications for the cumulative effects of harvesting and fire on age-class structure and natural disturbance-based management. Can. J. For. Res. 36, 2737–2744. doi: 10.1139/x06-177
Bergeron Y., Fenton N. J. (2012). Boreal forests of eastern Canada revisited: Old growth, nonfire disturbances, forest succession, and biodiversity. Botany 90, 509–523. doi: 10.1139/b2012-034
Bergeron Y., Gauthier S., Flannigan M., Kafka V. (2004). Fire regimes at the transition between mixedwood and coniferous boreal forest in northwestern Quebec. Ecology 85, 1916–1932. doi: 10.1890/02-0716
Bergeron Y., Leduc A., Harvey B. D., Gauthier S. (2002). Natural fire regime: A guide for sustainable management of the Canadian boreal forest. Silva Fennica 36, 81–95. doi: 10.14214/sf.553
Blanc L. A., Martin K. (2012). Identifying suitabl woodpecker nest trees using decay selection profiles in trembling aspen (Populus tremuloides). For. Ecol. Manage. 286, 192–202. doi: 10.1016/j.foreco.2012.08.021
Blanc L. A., Walters J. R. (2008). Cavity-nest webs in a longleaf pine ecosystem. Condor 110, 80–92. doi: 10.1525/cond.2008.110.1.80
Bonar R. L. (2000). Availability of Pileated Woodpecker cavities and use by other species. J. Wildlife Manage. 64, 52–59. doi: 10.2307/3802974
Bonar R. L. (2001). Pileated Woodpecker habitat ecology in the Alberta foothills (Edmonton: University of Alberta). doi: 10.7939/r3-g9w6-yy27
Cadieux P. (2011). Dynamique de la faune cavicole le long d’un gradient d’âge en forêt boréale mixte de l’est de l’Amérique du Nord. M.Sc. Thesis (Montréal: Université du Québec à Montréal). Available at: https://archipel.uqam.ca/3995/.
Cadieux P., Drapeau P. (2017). Are old boreal forests a safe bet for the conservation of the avifauna associated with decayed wood in eastern Canada? For. Ecol. Manage. 385, 127–139. doi: 10.1016/j.foreco.2016.11.024
Cadieux P., Drapeau P., Ouellet-Lapointe U., Leduc A., Imbeau L., Deschênes R., et al. (2023). Old forest structural development drives complexity of nest webs in a naturally disturbed boreal mixedwood forest landscape. Front. Forests Global Change 6. doi: 10.3389/ffgc.2023.1084696
Carlson A. (2000). The effect of habitat loss on a deciduous forest specialist species: the White-backed Woodpecker (Dendrocopos leucotos). For. Ecol. Manage. 131, 215–231.
Cheveau M., Imbeau L., Drapeau P., Belanger L. (2013). Marten space use and habitat selection in managed coniferous boreal forests of eastern Canada. J. Wildlife Manage. 77, 749–760. doi: 10.1002/jwmg.511
Cooke H. A., Hannon S. J. (2011). Do aggregated harvests with structural retention conserve the cavity web of old upland forest in the boreal plains? For. Ecol. Manage. 261, 662–674. doi: 10.1016/j.foreco.2010.11.023
Cooke H. A., Hannon S. J. (2012). Nest-site selection by old boreal forest cavity excavators as a basis for structural retention guidelines in spatially-aggregated harvests. For. Ecol. Manage. 269, 37–51. doi: 10.1016/j.foreco.2011.12.042
Dansereau P., Bergeron Y. (1993). Fire history in the southern boreal forest of northwestern Quebec. Can. J. For. Res. 23, 25–32. doi: 10.1139/x93-005
Dobkin D. S., Rich A. C., Pretare J. A., Pyle W. H. (1995). Nest-site relationships among cavity-nesting birds of riparian and snowpocket aspen woodlands in the northwestern Great Basin. Condor 97, 694–704. doi: 10.2307/1369178
Drapeau P., Leduc A., Bergeron Y. (2009a). “Bridging ecosystem and multiple species approaches for setting conservation targets in managed boreal landscapes,” in Setting conservation targets in managed forest landscapes. Eds. Villard M. A., Jonsson B. G. (Cambridge University Press, New York, USA), 129–160. doi: 10.1017/CBO9781139175388.008
Drapeau P., Leduc A., Bergeron Y., Gauthier S., Savard J. P. (2003). Les communautés d’oiseaux des vieilles forêts de la pessière à mousses de la ceinture d’argile: Problèmes et solutions face à l’aménagement forestier. Forestry Chronicle 79, 531–540. doi: 10.5558/tfc79531-3
Drapeau P., Nappi A., Imbeau L., Saint-Germain M. (2009b). Standing deadwood for keystone bird species in the eastern boreal forest: Managing for snag dynamics. For. Chron. 85, 227–234. doi: 10.5558/tfc85227-2
Drapeau P., Villard M. A., Leduc A., Hannon S. J. (2016). Natural disturbance regimes as templates for the response of bird species assemblages to contemporary forest management. Diversity Distributions. 22, 385–399. doi: 10.1111/ddi.12407
Dunne J. A., Williams R. J., Martinez N. D. (2002). Network structure and biodiversity loss in food webs: Robustness increases with connectance. Ecol. Lett. 5, 558–567. doi: 10.1046/j.1461-0248.2002.00354.x
Edworthy A. B., Martin K. (2013). Persistence of tree cavities used by cavity-nesting vertebrates declines in harvested forests. J. Wildlife Manage. 77, 770–776. doi: 10.1002/jwmg.526
Feldman M. J., Mazerolle M. J., Imbeau L., Fenton N. J. (2023). Beaver activity and red squirrel presence predict bird assemblages in boreal Canada. Ornithology 140, ukad009. doi: 10.1093/ornithology/ukad009
Fenton N., Lecomte N., Légaré S., Bergeron Y. (2005). Paludification in black spruce (Picea mariana) forests of eastern Canada: Potential factors and management implications. For. Ecol. Manage. 213, 151–159. doi: 10.1016/j.foreco.2005.03.017
Fisher R. J., Wiebe K. L. (2006). Nest site attributes and temporal patterns of northern flicker nest loss: effects of predation and competition. Oecologia 147, 744–753. doi: 10.1007/s00442-005-0310-2
Franklin J. F., Shugart H. H., Harmon M. E. (1987). Tree death as an ecological process. BioScience 37, 550–556. doi: 10.2307/1310665
Gauthier S., Bernier P., Kuuluvainen T., Shvidenko A., Schepaschenko D. (2015). Boreal forest health and global change. Science 349, 819–822. doi: 10.1126/science.aaa9092
Gauthier S., De Grandpré L., Bergeron Y. (2000). Differences in forest composition in two boreal forest ecoregions of Quebec. J. Vegetation Sci. 11, 781–790. doi: 10.2307/3236548
Gauthier S., Vaillancourt M. A., Leduc A., De Grandpré L., Kneeshaw D., Morin H., et al. (2009). Ecosystem management in the boreal forest (Québec, QC, Canada: Presses de l’Université du Québec).
Grover A., Baldassarre G. (1995). Bird species richness within beaver ponds in south-central New York. Wetlands 15, 108–118. doi: 10.1007/BF03160664
Grudzinski B. P., Fritzb K., Goldenb H. E., Newcomer-Johnson T. A., Rechc J. A., Levyc J., et al. (2022). A global review of beaver dam impacts: Stream conservation implications across biomes. Glob Ecol. Conserv. 37, 1–15. doi: 10.1016/j.gecco.2022.e02163
Harvey B. D. (1999). The Lake Duparquet Research and Teaching Forest: Building a foundation for ecosystem management. Forestry Chronicle 75, 389–393. doi: 10.5558/tfc75389-3
Jackson J. A., Jackson B. J. S. (2004). Ecological relationships between fungi and woodpecker cavity sites. Condor 106, 37–49. doi: 10.1093/condor/106.1.37
Jacqmain H., Dussault C., Courtois R., Bélanger L. (2008). Moose–habitat relationships: Integrating local Cree native knowledge and scientific findings in northern Quebec. Can. J. For. Res. 38, 3120–3132. doi: 10.1139/X08-128
Karanth K., Nichols J., Sauer J., Hines J., Yackulic C. (2014). Latitudinal gradients in North American avian species richness, turnover rates, and extinction probabilities. Ecography 37, 626–636. doi: 10.1111/j.1600-0587.2013.00367.x
Kerkhoff A. J. (2012). The biogeography and filtering of woody plant functional diversity in North and South America. Global Ecol. Biogeography 21, 798–808. doi: 10.1111/j.1466-8238.2011.00727.x
Kneeshaw D. D., Bergeron Y. (1998). Canopy gap characteristics and tree replacement in the southeastern boreal forest. Ecology 79, 783–794. doi: 10.1890/0012-9658(1998)079[0783:CGCATR]2.0.CO;2
Kouki J. (1999). Latitudinal gradients in species richness in northern areas: some exceptional patterns. Ecol. Bulletins 47, 30–37.
Lavertu D., Mauffette Y., Bergeron Y. (1994). Effects of stand age and litter removal on the regeneration of Populus tremuloides. J. Vegetation Sci. 5, 561–568. doi: 10.2307/3235983
Levine R., Meyer G. (2019). Beaver-generated disturbance extends beyond active dam sites to enhance stream morphodynamics and riparian plant recruitment. Sci. Rep. 9, 8124. doi: 10.1038/s41598-019-44381-2
Li P., Martin T. E. (1991). Nest-site selection and nesting success of cavity-nesting birds in high elevation forest drainages. Auk 108, 405–418. doi: 10.1093/auk/108.2.405
Lindenmayer D. B., Laurance W. F., Franklin J. F. (2012). Global decline in large old trees. Science 338, 1305–1306.https://www.jstor.org/stable/20113224
Lochmiller R. L. (1979). Use of beaver ponds by southeastern woodpeckers in winter. J. Wildlife Manage. 43, 263–266. doi: 10.2307/3800670
Mackey B., Campbell C., Norman P., Hugh S., DellaSala D. A., Malcolm J. R., et al. (2023). Assessing the cumulative impacts of forest management on forest age structure development and woodland caribou habitat in boreal landscapes: A case study from two Canadian provinces. Land 13, 6. doi: 10.3390/land13010006
Martin K., Aitken K. E. H., Wiebe K. L. (2004). Nest sites and nest webs for cavity-nesting communities in interior British Columbia, Canada: Nest characteristics and niche partitioning. Condor 106, 5–19. doi: 10.1093/condor/106.1.5
Martin K., Eadie J. M. (1999). Nest webs: A community-wide approach to the management and conservation of cavity-nesting forest birds. For. Ecol. Manage. 115, 243–257. doi: 10.1016/S0378-1127(98)00403-4
McCoy E. D., Connor E. F. (1980). Latitudinal gradients in the species diversity of North American mammals. Evolution 34, 193–203. doi: 10.1111/j.1558-5646.1980.tb04805.x
MRNF (2011). Norme de stratification écoforestière, Quatrième inventaire écoforestier du Québec méridional (Québec: Ministère des Ressources naturelles et de la Faune, Gouvernement du Québec), 101.
Naiman R. J., Melillo J. M., Hobbie J. E. (1986). Ecosystem alteration of boreal forest streams by beaver (Castor canadensis). Ecology 67, 1254–1269. doi: 10.2307/1938681
Nappi A. (2009). Utilisation du bois mort et des forêts brûlées par le Pic à dos noir en forêt boréale. Ph.D. Thesis (Montréal: Université du Québec à Montréal), 220. Available at: https://archipel.uqam.ca/2283/.
Nappi A., Drapeau P. (2009). Reproductive success of the black-backed woodpecker (Picoides arcticus) in burned boreal forests: Are burns source habitats? Biol. Conserv. 142, 1381–1391. doi: 10.1016/j.biocon.2009.01.022
Nappi A., Drapeau P., Giroux J.-F., Savard J.-P. L. (2003). Snag use by foraging black-backed woodpeckers (Picoides articus) in a recently burned eastern boreal forest. Auk 120, 505–511. doi: 10.1093/auk/120.2.505
Nappi A., Drapeau P., Leduc A. (2015). How important is dead wood for woodpeckers foraging in eastern North American boreal forests? For. Ecol. Manage. 346, 10–21. doi: 10.1016/j.foreco.2015.02.028
Nappi A., Drapeau P., Saint-Germain M., Angers V. A. (2010). Effect of fire severity on long-term occupancy of burned boreal conifer forests by saproxylic insects and wood-foraging birds. Int. J. Wildland Fire 19, 500–511. doi: 10.1071/WF08109
Nummi P., Holopainen S. (2014). Whole-community facilitation by beaver: Ecosystem engineer increases waterbird diversity. Aquat. Conservation: Mar. Freshw. Ecosyst. 24, 623–633. doi: 10.1002/aqc.2437
Nummi P., Kuuluvainen T. (2013). Forest disturbance by an ecosystem engineer: beaver in boreal forest landscapes. Boreal Environ. Res. 18, 13–24.
Orazi V., Hagge J., Gossner M. M., Müller J., Heurich M. (2022). Biodiversity boost from the eurasian beaver (Castor fiber) in Germany’s oldest national park. Front. Ecol. Evol. 10. doi: 10.3389/fevo.2022.873307
Ouellet-Lapointe U., Drapeau P., Cadieux P., Imbeau L. (2012). Woodpecker excavations suitability for and occupancy by cavity users in the boreal mixedwood forest of eastern Canada. Ecoscience 19, 391–397. doi: 10.2980/19-4-3582
Pianka E. R. (1966). Latitudinal gradients in species diversity: A review of concepts. Am. Nat. 100, 33–46. doi: 10.1086/282398
Pietrasz K., Sikora D. J., Chodkiewicz T., Ślęzak M., Woźniak B. (2019). Keystone role of Eurasian beaver Castor fiber in creating the suitable habitat over the core breeding range for forest specialist species the three-toed woodpecker Picoides tridactylus. Baltic Forestry 25, 223–227. doi: 10.46490/vol25iss2pp223
Remm J., Lõhmus A. (2011). Tree cavities in forest – The broad distribution pattern of a keystone structure for biodiversity. For. Ecol. Manage. 262, 579–585.
R Core Team (2015). “R: A language and environment for statistical computing,” in R foundation for statistical computing, version 3.2.3 (Vienna: R Foundation for Statistical Computing).
Robitaille A., Saucier J. P. (1998). Paysages régionaux du Québec méridional (Québec: Les Publications du Québec), 220, ISBN: ISBN: 2551177375.
Robles H., Martin K. (2014). Habitat-mediated variation in the importance of ecosystem engineers for secondary cavity nesters in a nest web. PloS One 9, e90071. doi: 10.1371/journal.pone.0090071
Rohde K. (1992). Latitudinal gradients in species diversity: the search for the primary cause. Oikos 65, 514–527. doi: 10.2307/3545569
Romansic J., Nelson N., Moffett K., Piovia-Scott J. (2020). Beaver dams are associated with enhanced amphibian diversity via lengthened hydroperiods and increased representation of slow-developing species. Freshw. Biol. 66, 481–494. doi: 10.1111/fwb.13654
Rosell F., Bozser O., Collen P., Parker H. (2005). Ecological impact of beavers Castor fiber and Castor canadensis and their ability to modify ecosystems. Mammal Rev. 35, 248–276. doi: 10.1111/j.1365-2907.2005.00067
Roy Nielsen C. L., Gates R. J. (2007). Reduced nest predation of cavity-nesting wood ducks during flooding in a bottomland hardwood forest. Condor 109, 210–215. doi: 10.1093/condor/109.1.210.x
Schmidt S. M., Fournier A. M. V., Osborn J. M., Benson T. J. (2023). Water depth influences survival and predator-specific patterns of nest loss in three secretive marsh bird species. Ecol. Evol. 13, e10823. doi: 10.1002/ece3.10823
Simard M., Lecomte N., Bergeron Y., Bernier P. Y., Paré D. (2007). Forest productivity decline caused by successional paludification of boreal soils. Ecol. Appl. 17, 1619–1637. doi: 10.1890/06-1795.1
Thompson I. D., Ortiz D. A., Jastrebski C., Corbett D. (2006). Comparison of prism plots and modified point-distance sampling to calculate tree stem density and basal area. North. J. Appl. For. 23, 218–221. doi: 10.1093/njaf/23.3.218
Tremblay J. A., Ibarzabal J., Dussault C., Savard J. P. L. (2009). Habitat requirements of breeding black-backed woodpeckers (Picoides arcticus) in managed, unburned boreal forest. Avian Conserv. Ecol. 4, 3. doi: 10.5751/ACE-00297-040102
Tremblay J. A., Savard J.-P. L., Ibarzabal J. (2015). Structural retention requirements for a key ecosystem engineer in conifer-dominated stands of a boreal managed landscape in eastern Canada. For. Ecol. Manage. 357, 220–227. doi: 10.1016/j.foreco.2015.08.024
Vaillancourt M. A., Drapeau P., Robert M., Gauthier S. (2009). Origin and availability of large cavities for Barrow’s Goldeneye (Bucephala islandica), a species at risk inhabiting the eastern Canadian boreal forest. Avian Conserv. Ecol. 4, 6. doi: 10.5751/ACE-00295-040106
Wesolowski T., Martin K. (2018). “Tree holes and hole nesting birds in European and North American forests,” in Ecology and conservation of forest birds, Chap. 4. Eds. Mikusinski G., Roberge J., Fuller R. (Cambridge University Press, Cambridge), 79–134. doi: 10.1017/9781139680363
Wiebe K. L., Moore W. S. (2008). “Northern flicker (Colaptes auratus),” in The birds of north america online (Cornell lab of ornithology) Ithaca: Cornell Lab of Ornithology.
Keywords: upland mixedwood forests, upland conifer forests, beaver ponds, cavity-using vertebrate communities, nest webs, lowland forests, boreal forests
Citation: Cadieux P, Drapeau P, Fouillet A and Deschênes R (2024) Persistence, changes and robustness of nest webs along a latitudinal gradient in the Canadian boreal forest. Front. Ecol. Evol. 12:1392652. doi: 10.3389/fevo.2024.1392652
Received: 29 February 2024; Accepted: 25 June 2024;
Published: 17 July 2024.
Edited by:
Miguel Montoro Girona, Université du Québec en Abitibi Témiscamingue, CanadaReviewed by:
Brenda C. McComb, Oregon State University, United StatesMariano Feldman, Université du Québec en Abitibi Témiscamingue, Canada
Kathryn Aitken, Yukon University, Canada
Copyright © 2024 Cadieux, Drapeau, Fouillet and Deschênes. This is an open-access article distributed under the terms of the Creative Commons Attribution License (CC BY). The use, distribution or reproduction in other forums is permitted, provided the original author(s) and the copyright owner(s) are credited and that the original publication in this journal is cited, in accordance with accepted academic practice. No use, distribution or reproduction is permitted which does not comply with these terms.
*Correspondence: Pierre Drapeau, ZHJhcGVhdS5waWVycmVAdXFhbS5jYQ==